Abstract
1. The single gap voltage clamp technique (Kovács & Schneider, 1978) was used to monitor membrane charge movement in tendon-terminated short segments of cut frog skeletal muscle fibres.
2. Experiments were performed both on fibres able to contract and on fibres in which contraction was eliminated by exposing the open end to a solution containing 20 mm-EGTA. In both cases ionic conductances were minimized by using a predominantly caesium glutamate solution at the open end and a predominantly tetraethyl-ammonium sulphate solution with tetrodotoxin at the closed end.
3. Modifications of previously used charge movement analysis procedures included synthesis of a `mean linear' ON and OFF capacitative transient from the OFFs of several different hyperpolarizing pulses and use of only the first 35 msec of the `mean linear' transient so that base lines could be fitted to unaltered latter parts of ON and OFF currents for depolarizing pulses.
4. Simultaneous two-micro-electrode and gap current recording from gap-clamped fibres with blocked contraction established the validity of gap-recorded charge movement currents.
5. For pulses to below about 0 mV in non-contracting fibres the charges QON and QOFF moved by the non-linear transient currents at pulse ON and OFF were approximately equal. For pulses to between about 0 and +50 mV QOFF exceeded QON, with the charge inequality increasing with both pulse amplitude and pulse duration.
6. Use of 20 mm-cobalt in the solution at the closed end eliminated the ON:OFF charge inequality for large depolarizations by decreasing QOFF.
7. The charge inequality and cobalt effect indicate that, in the absence of cobalt, ionic conductance was being slowly activated during depolarizations to between 0 and +50 mV and that inward calcium current tails were contributing to the measured QOFF values. The small and slowly developing ionic current during large depolarizations was probably removed with the straight sloping base line so that QON was minimally affected by conductance activation.
8. Average Q vs. V results for pulses to at most 0 mV in eighteen non-contracting fibres were well fitted by the two-state Boltzmann model where Q = Qmax/[1+exp-(V - ¯V)/k] with Qmax = 26·7±0·6 nC/μF, k = 16·7±0·6 mV and ¯V = -32·9±1·0 mV (least-squares values±s.d. obtained from fit).
9. In contracting fibres the only apparent artifact produced by contraction in the IQ records for pulses to at most 0 mV was a `bowing' of the OFF base lines for the larger pulses. The ON records appeared to be unaffected by contraction artifacts.
10. The average Q vs. V relationship for pulses to at most 0 mV in contracting fibres was virtually identical to the one obtained from fibres in which contraction was blocked.
11. The ON portions of IQ records for pulses to between about -50 and -25 mV exhibited prolonged tails, plateaux or secondary rising phases whereas the OFF portions decayed smoothly. IQ time courses were not noticeably different with or without blockage of contraction by internal EGTA.
Full text
PDF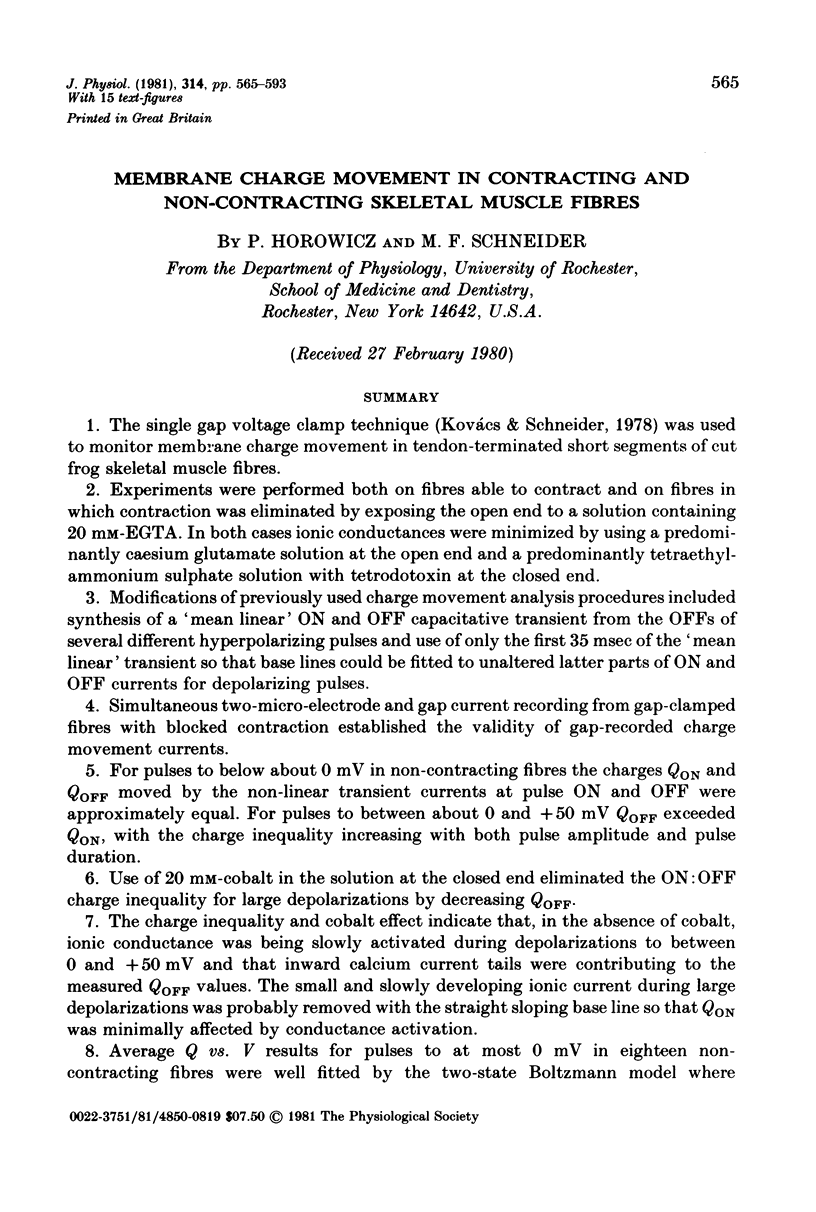
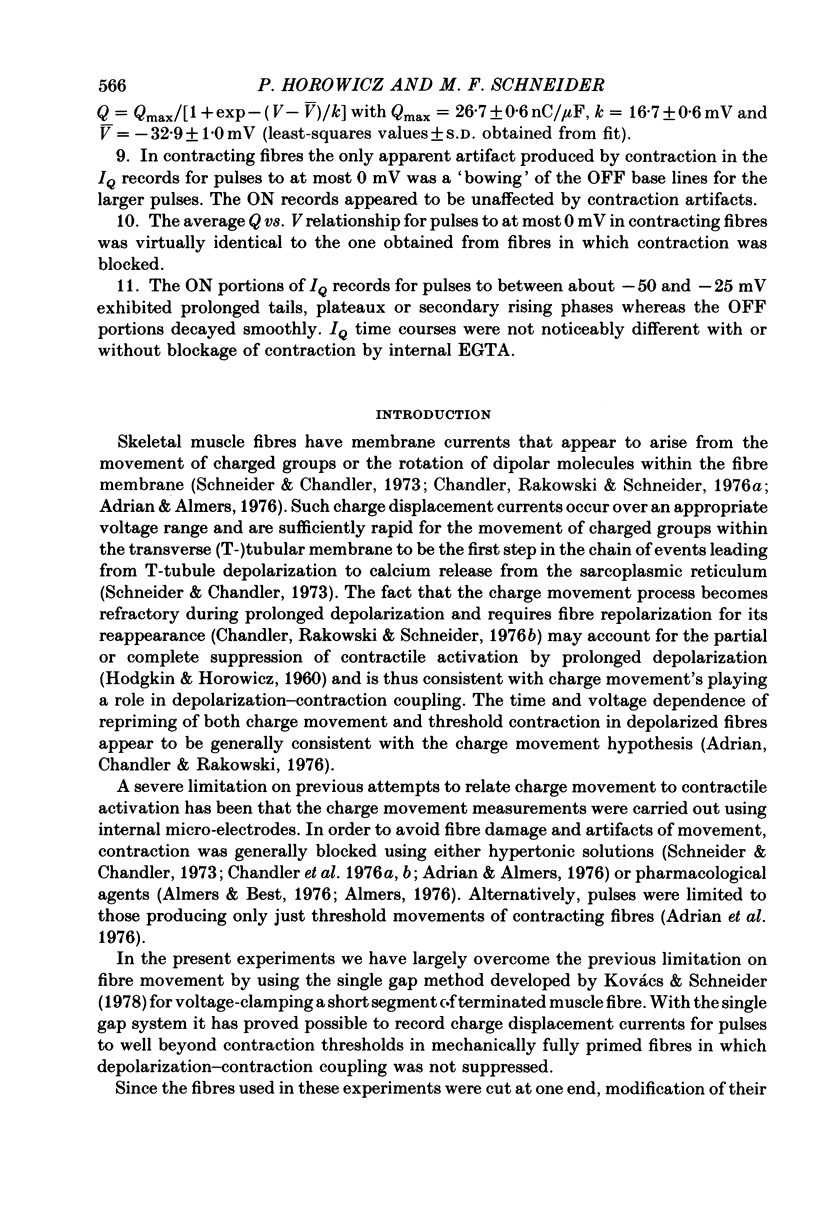
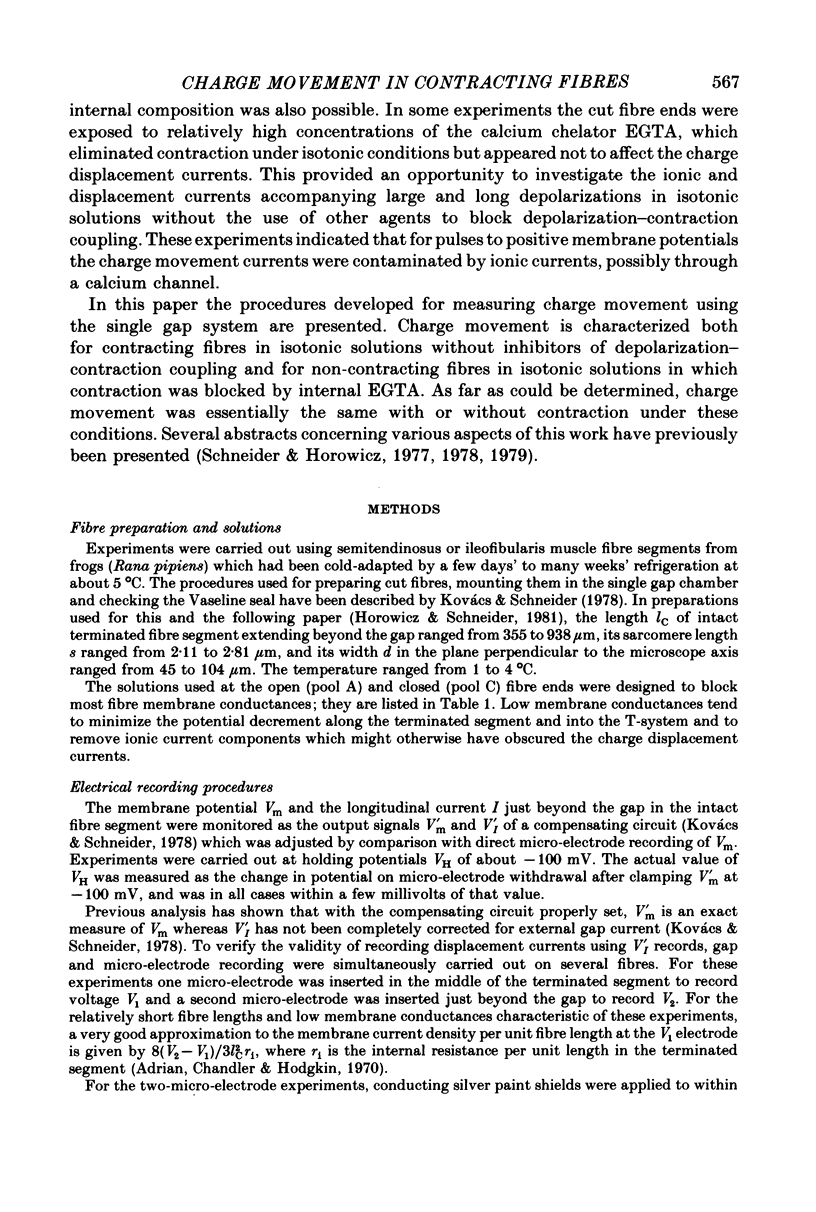
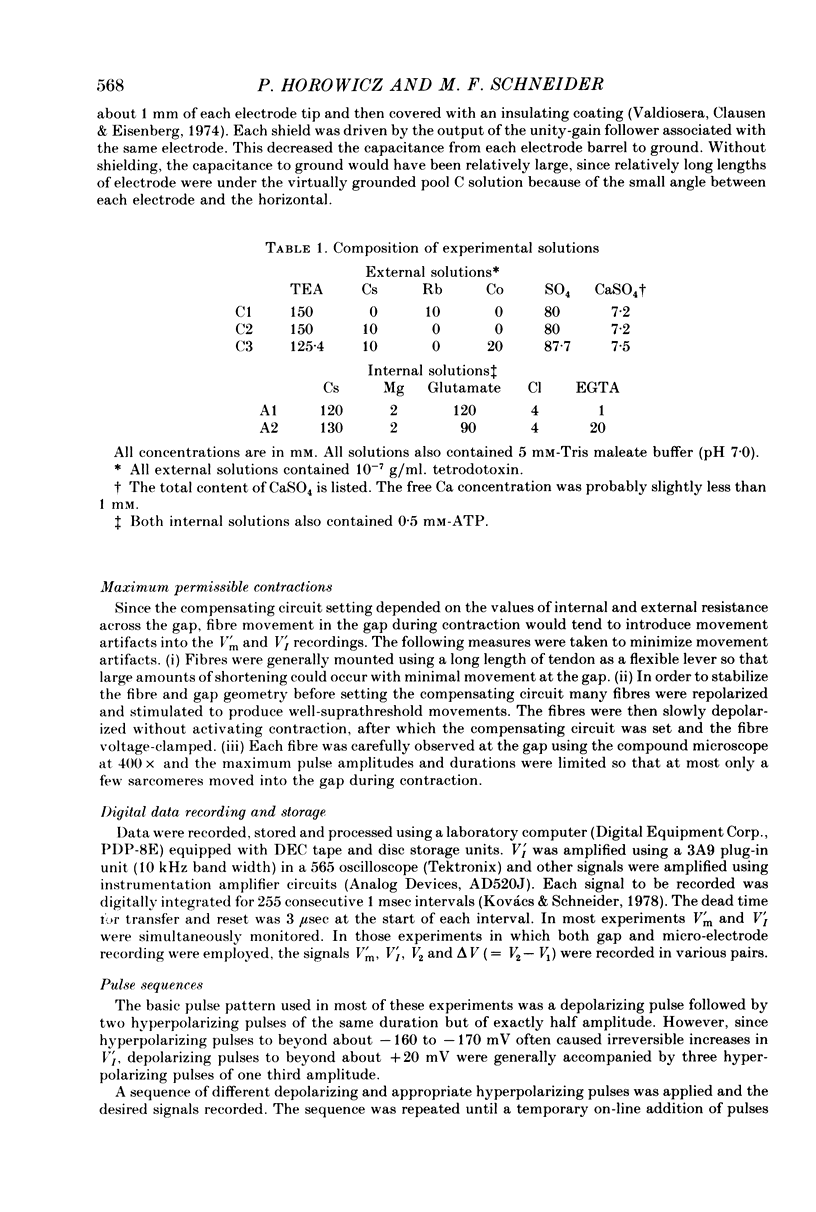
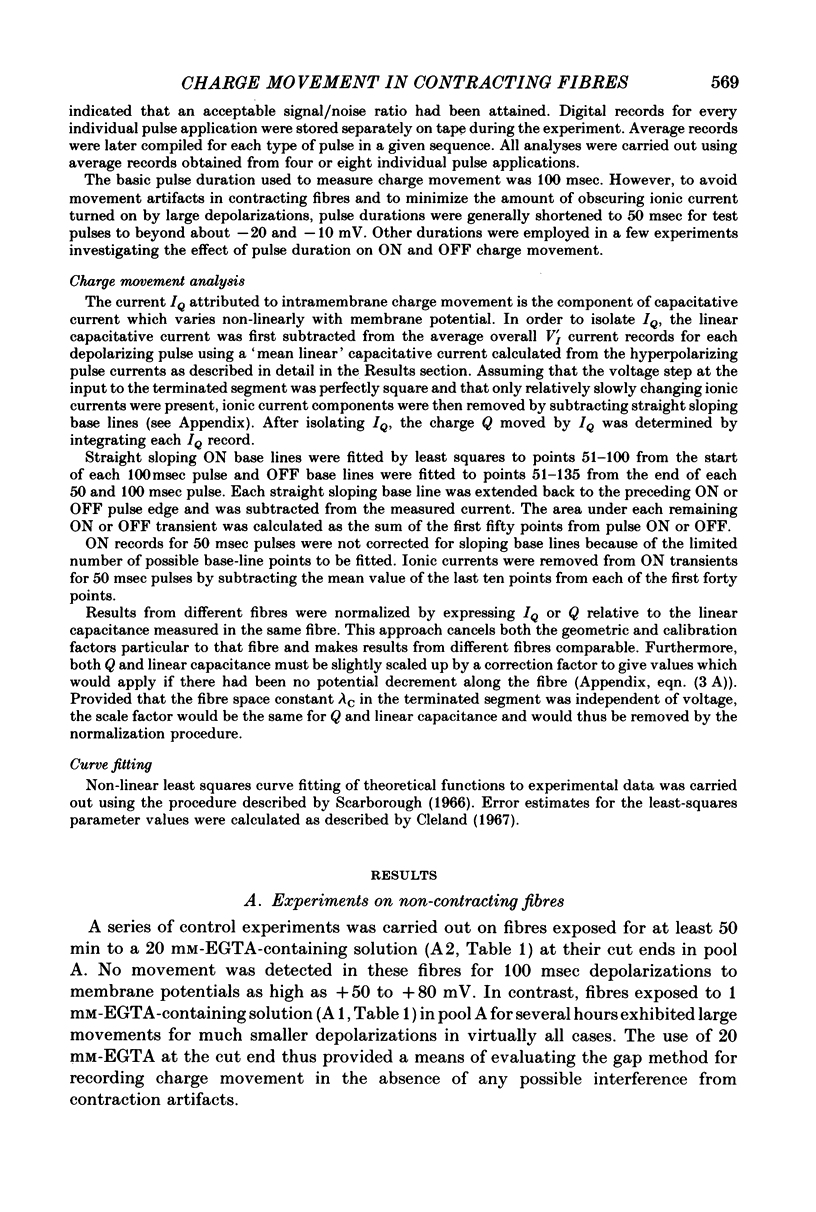
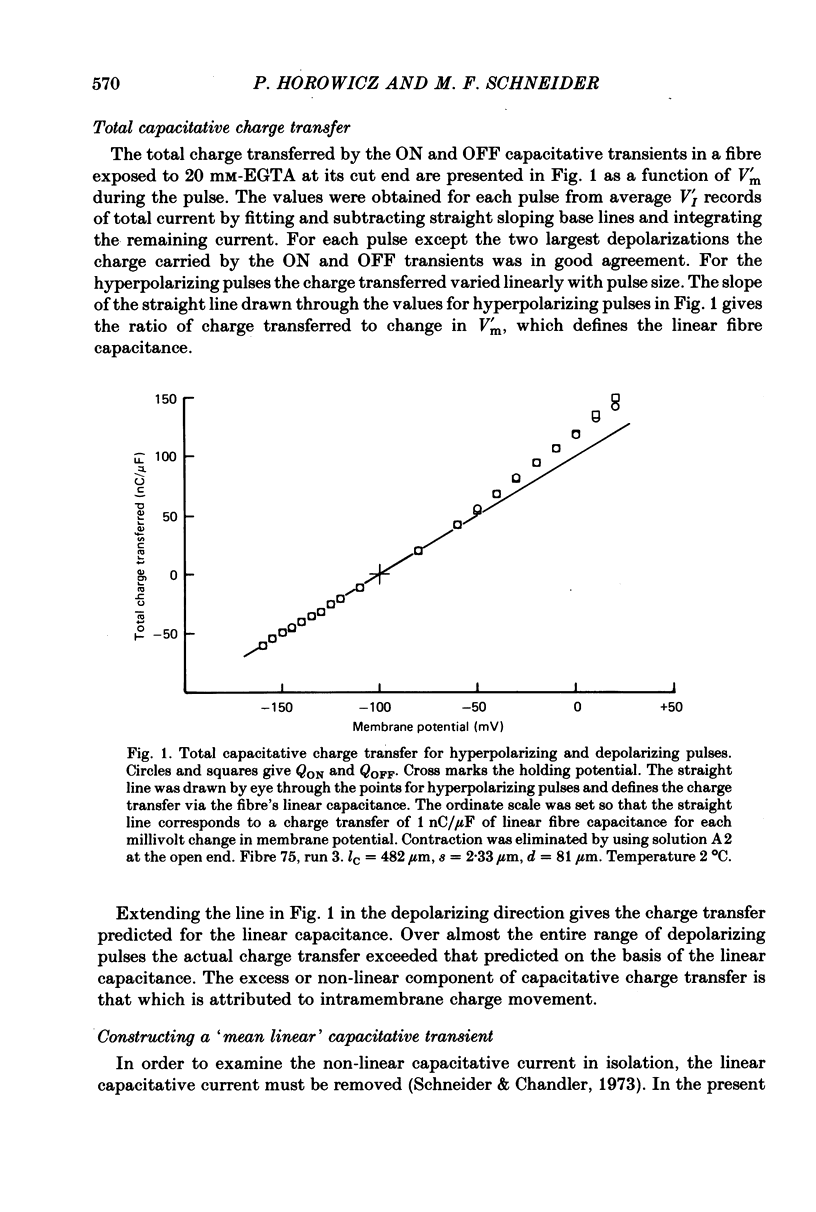
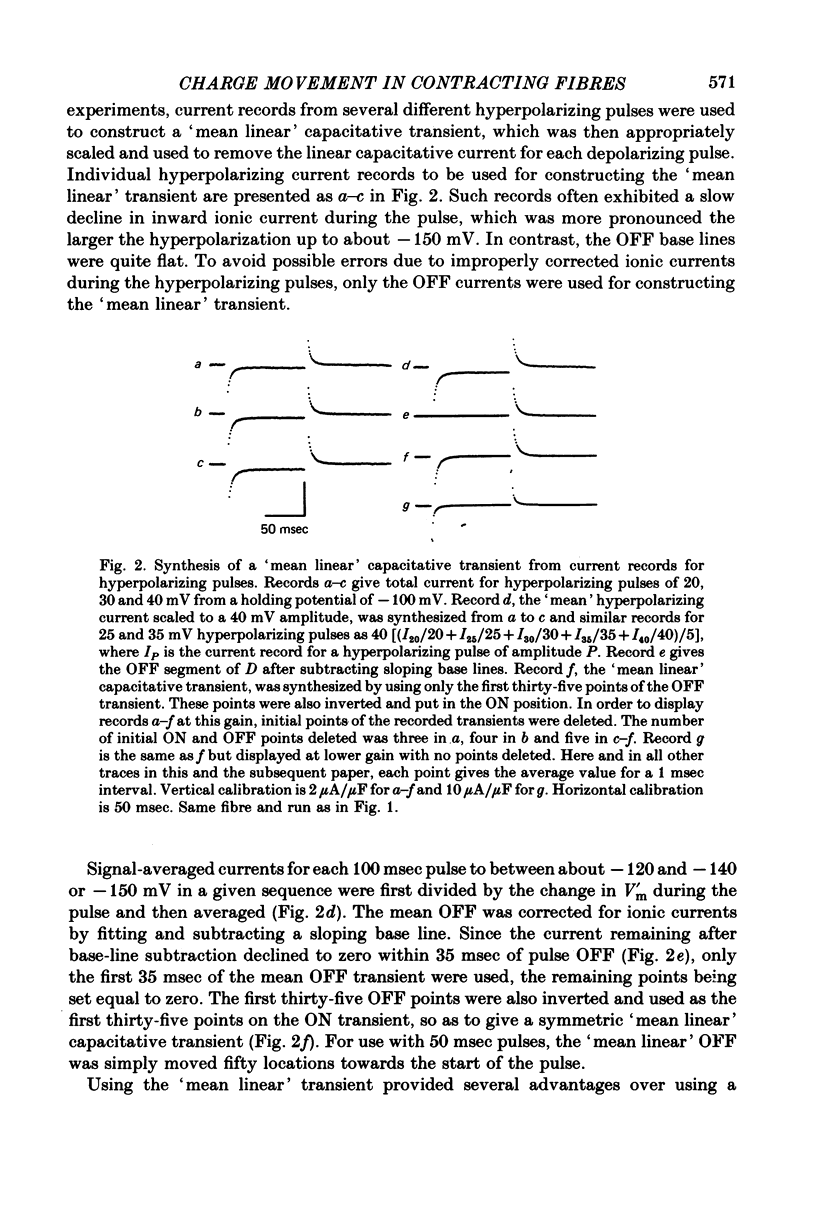
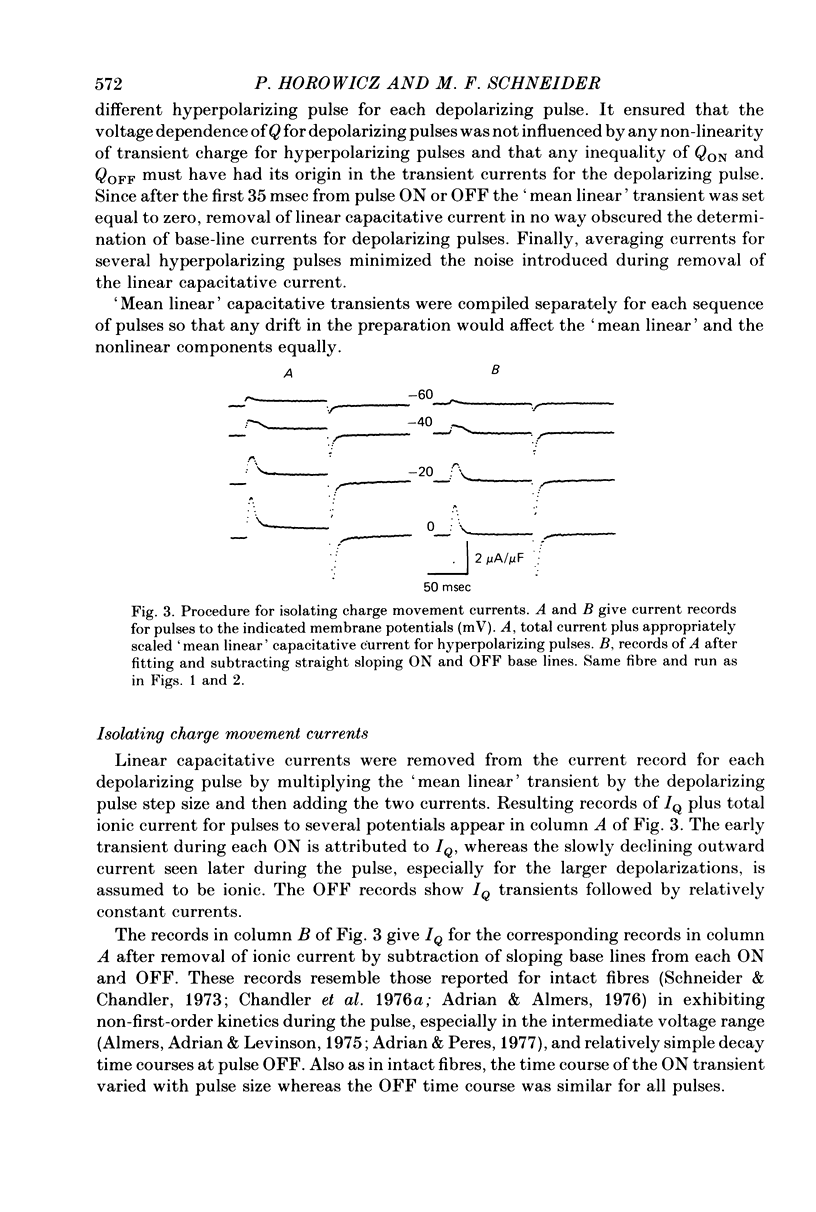
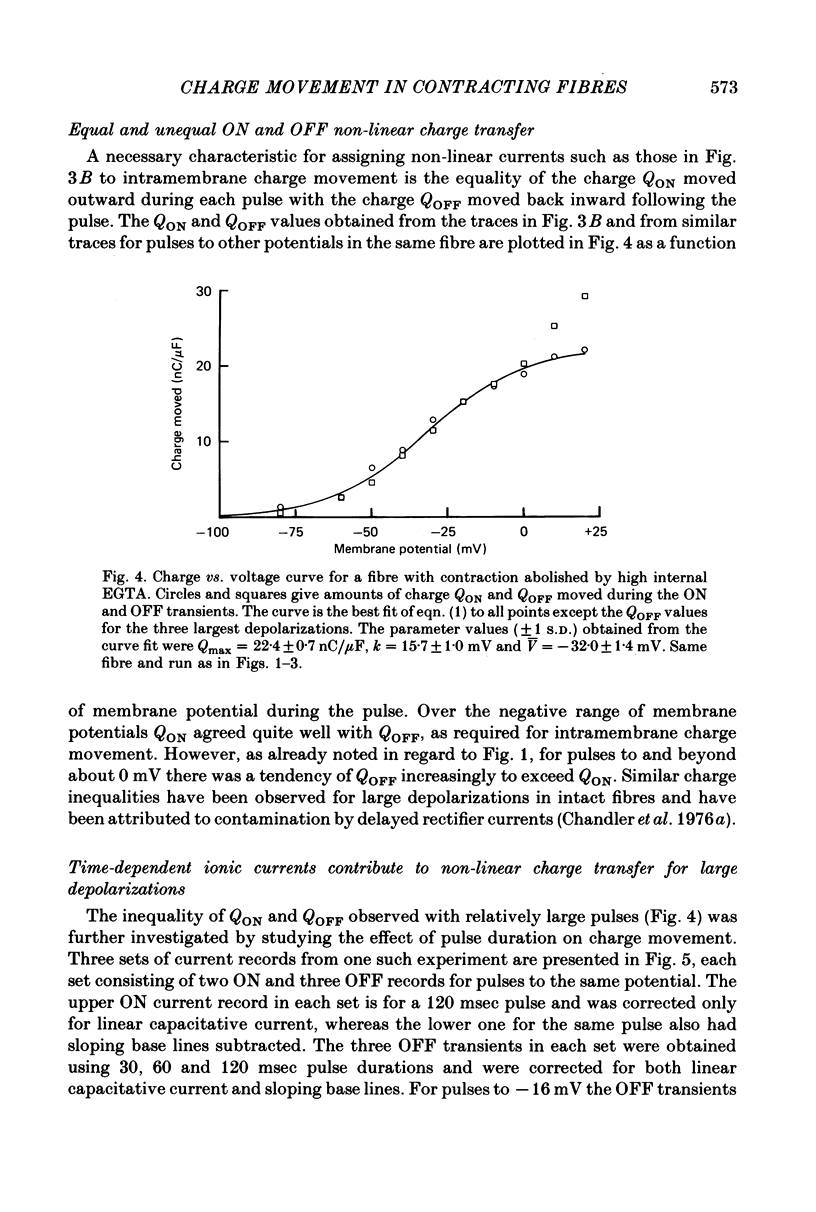
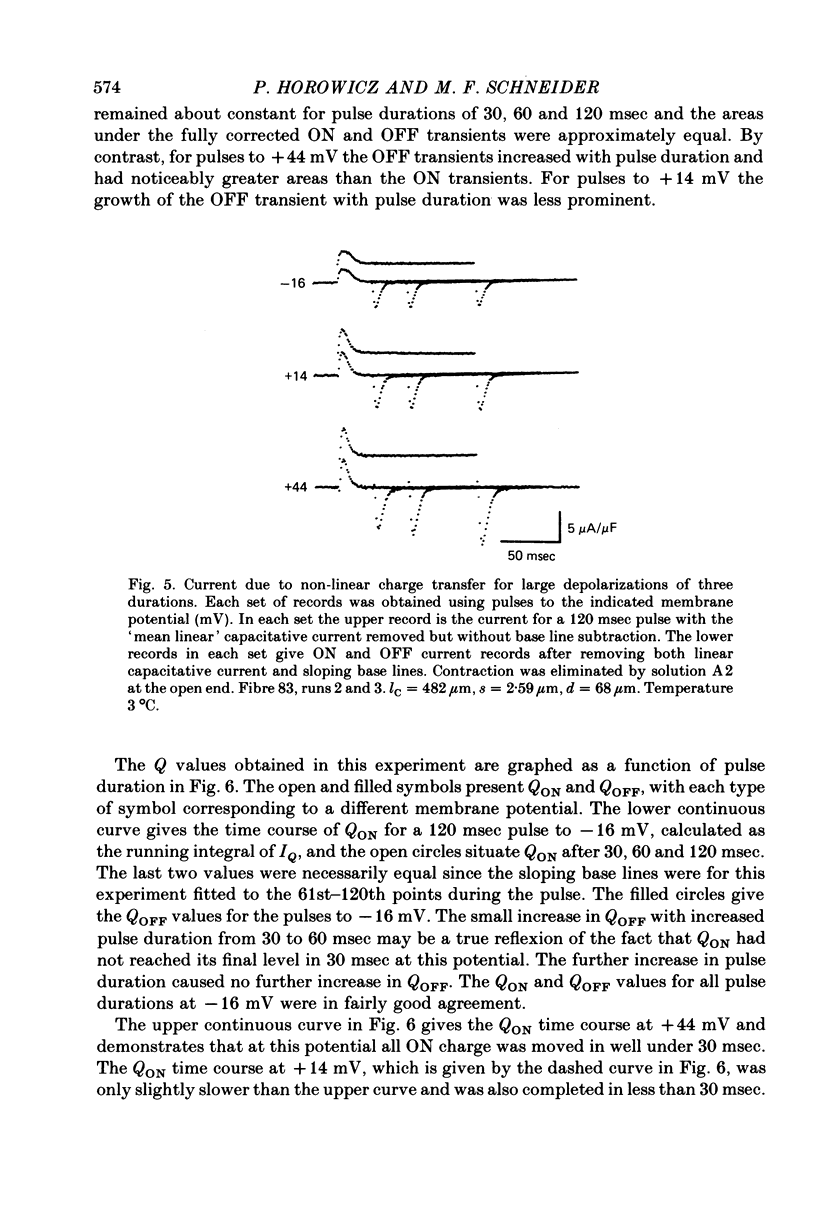
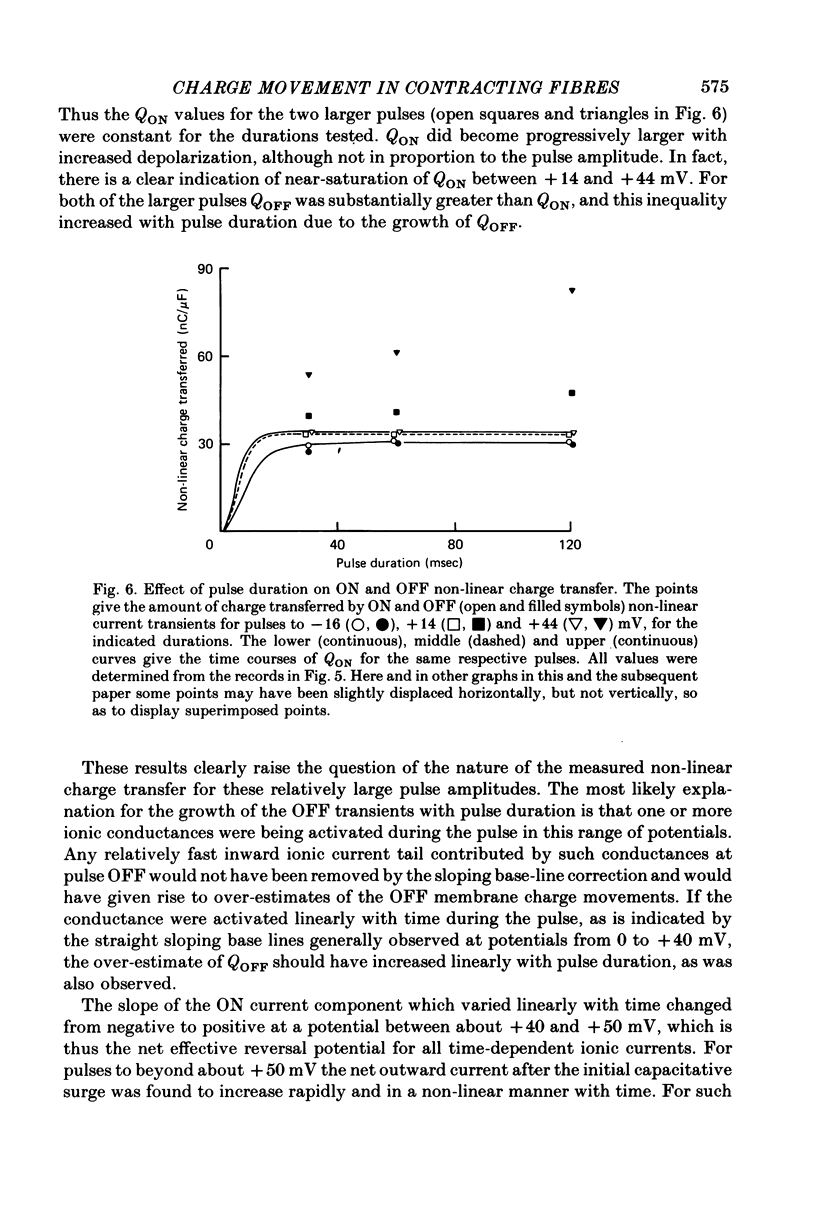
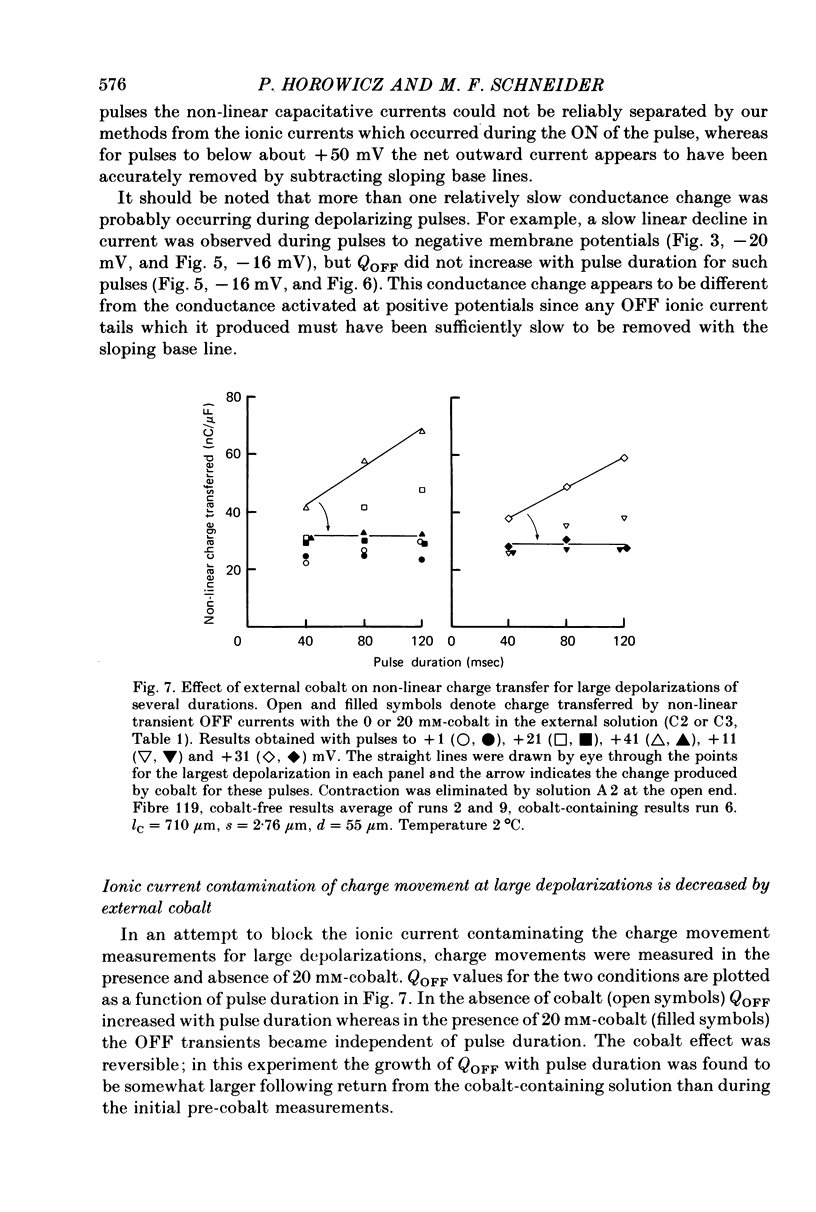
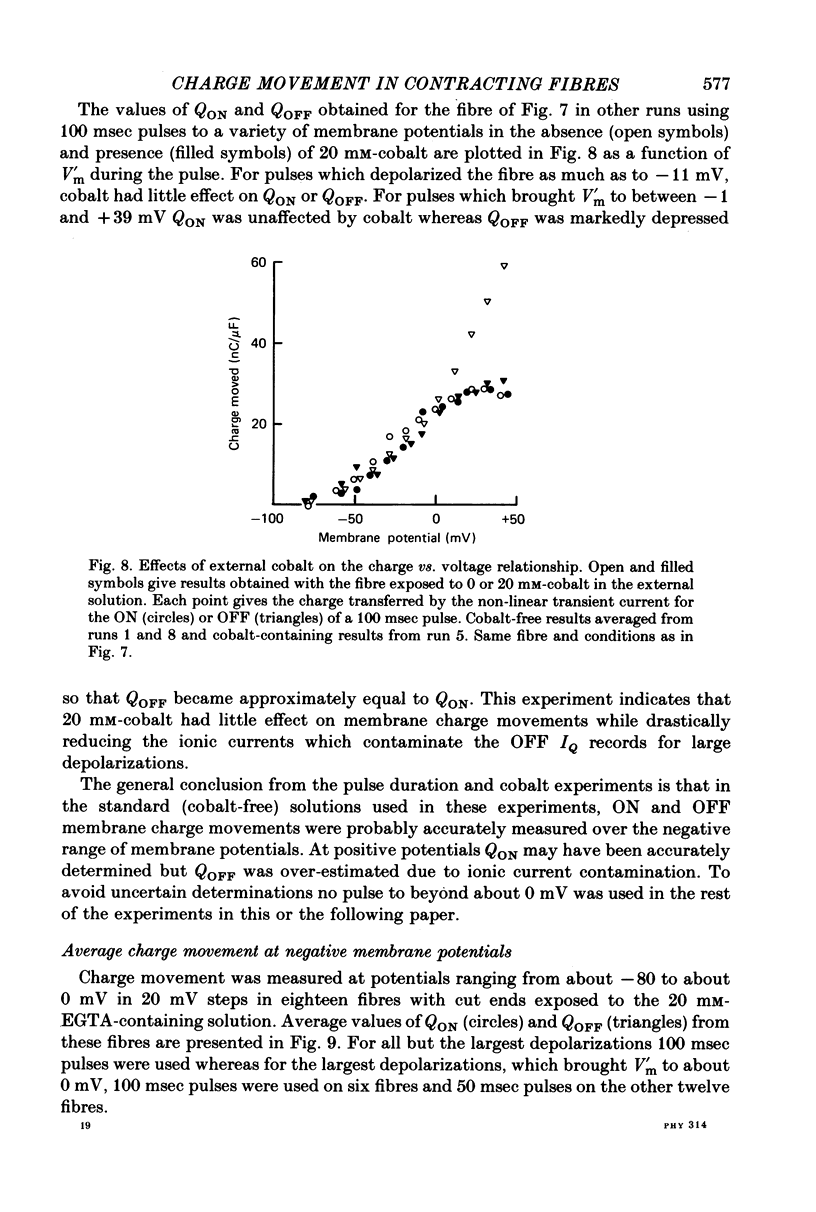
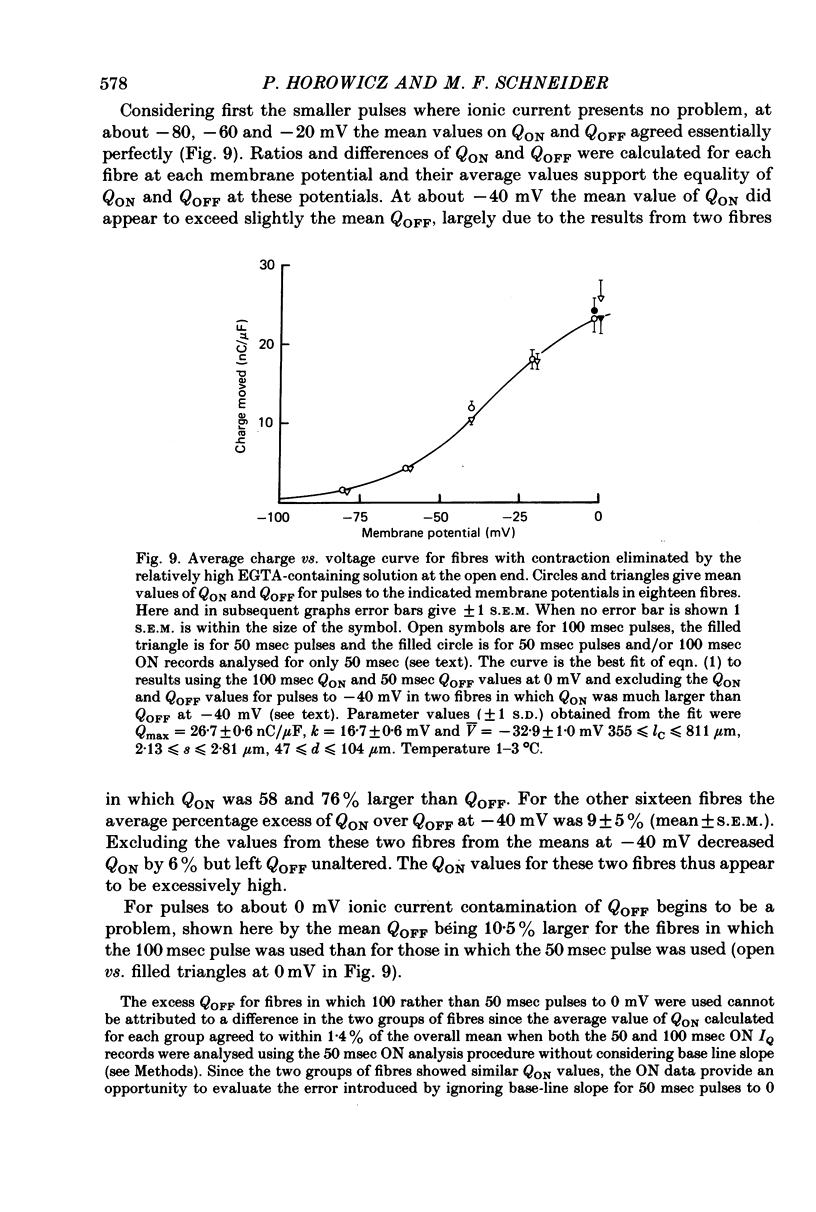
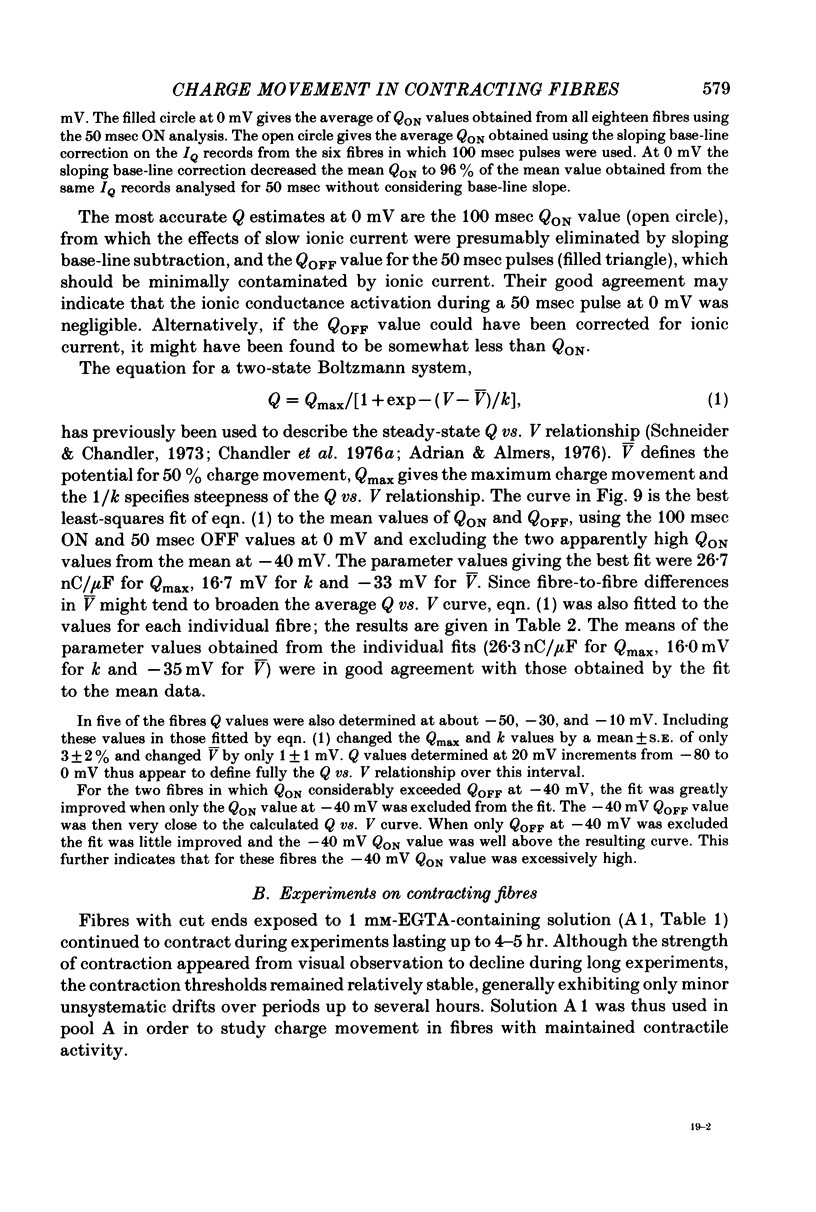
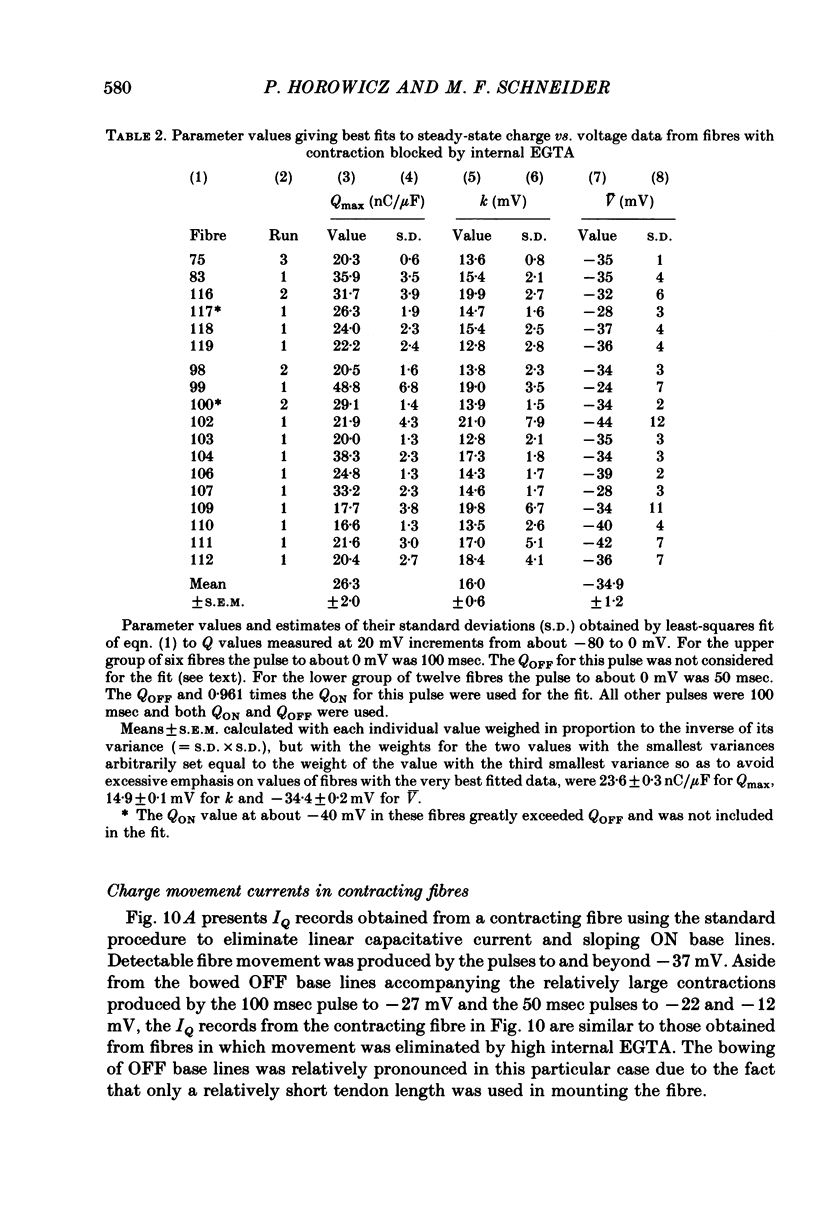
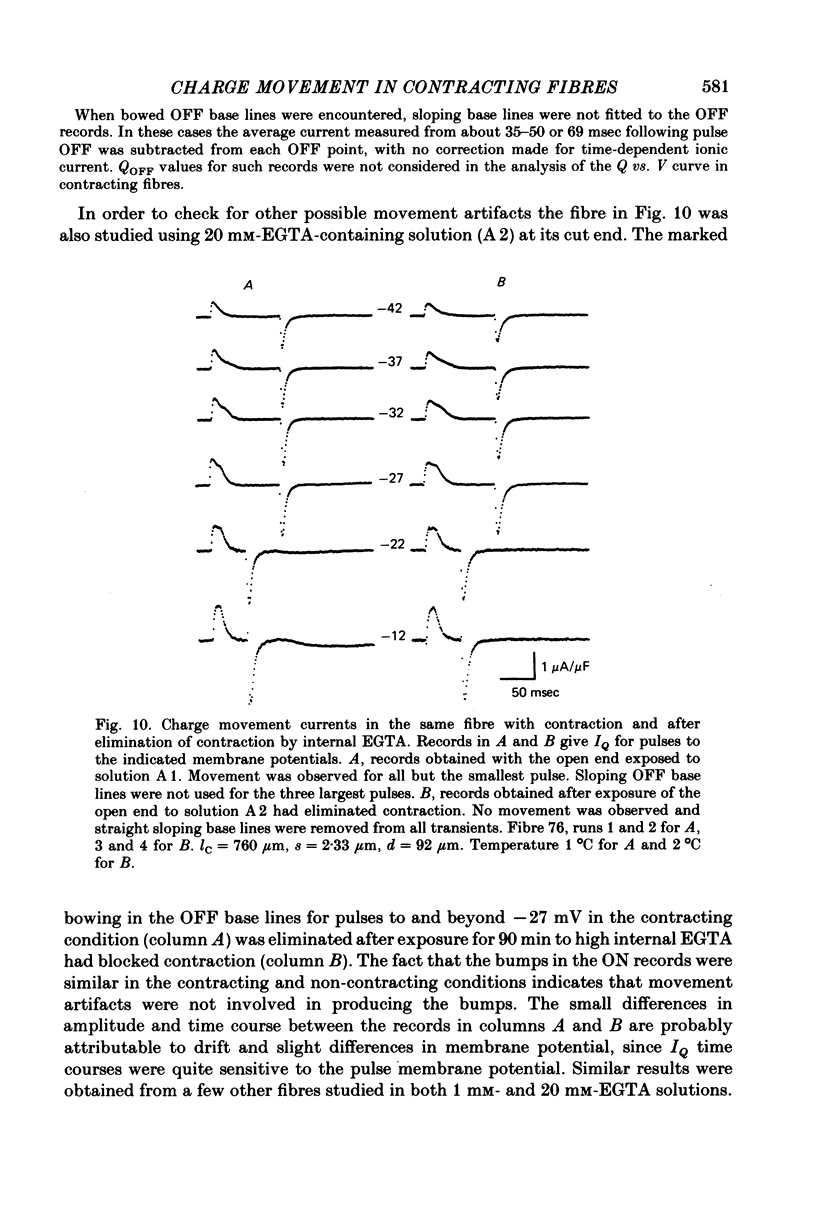
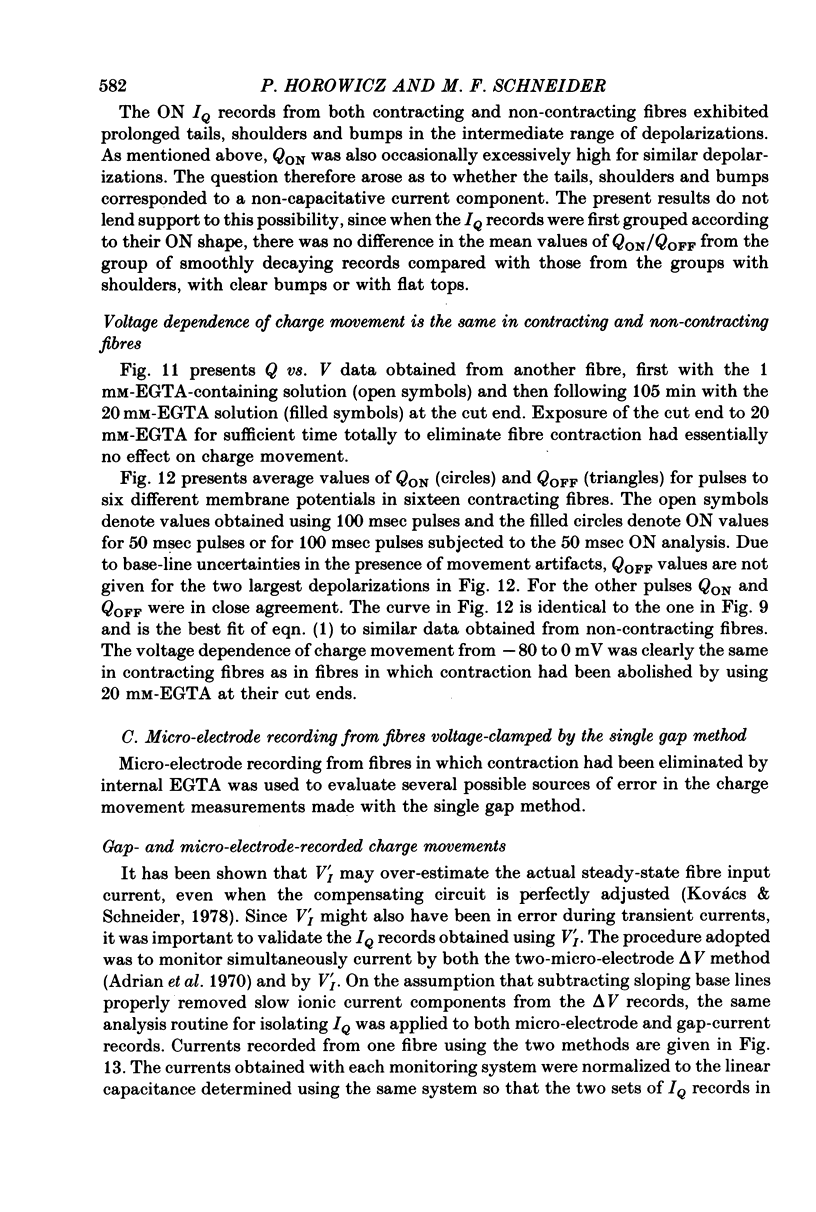
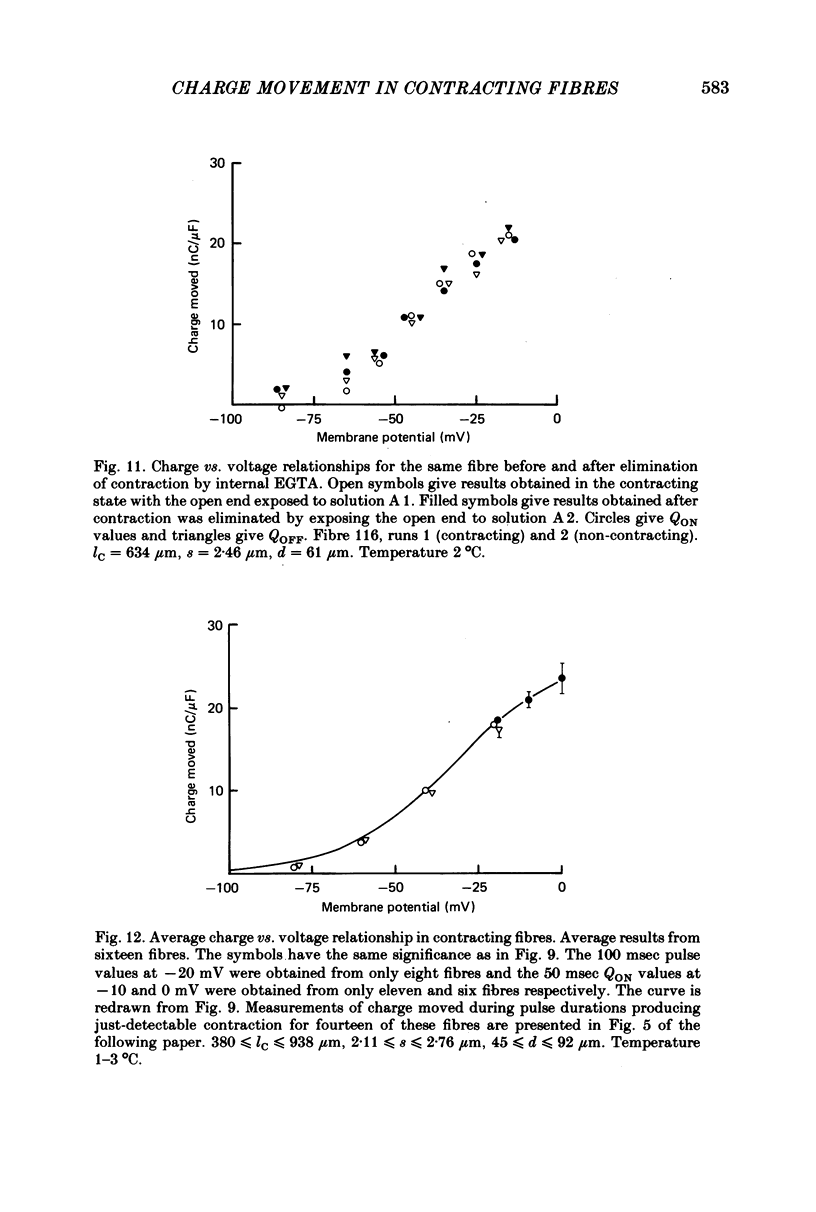
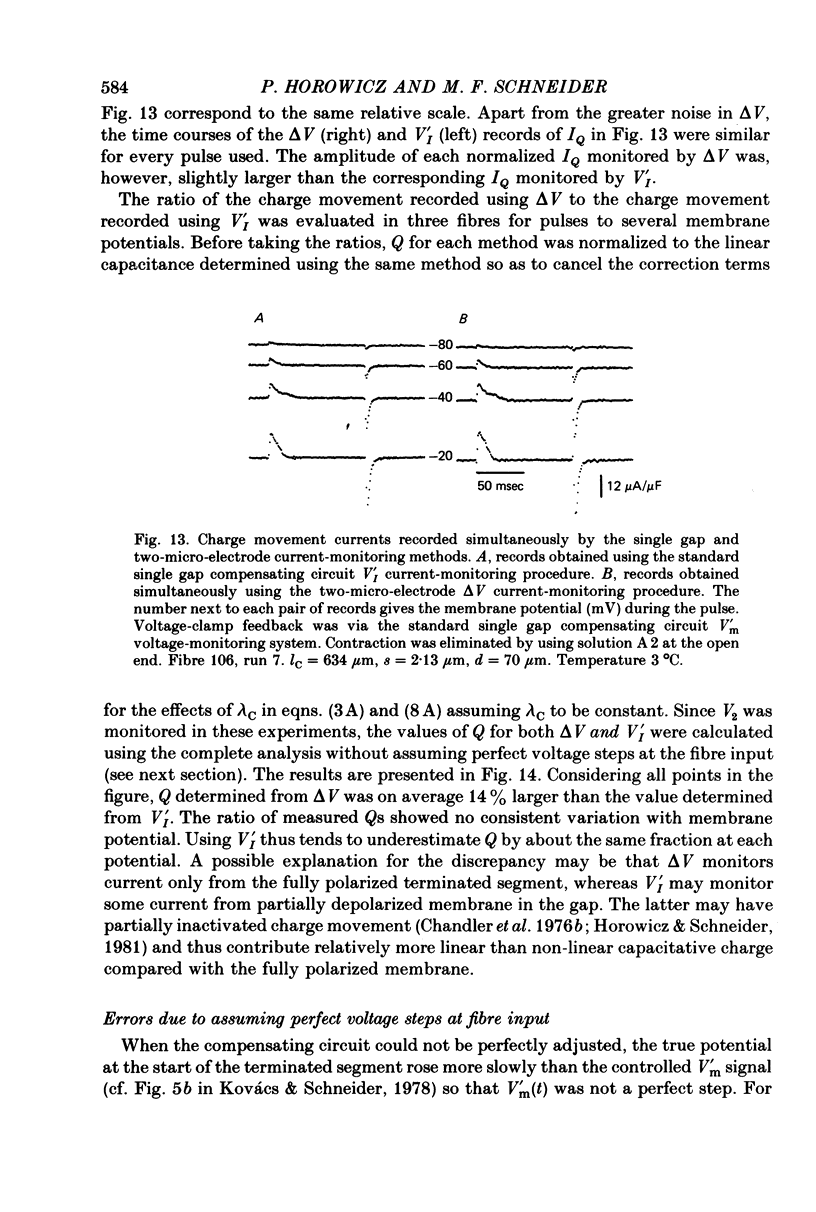
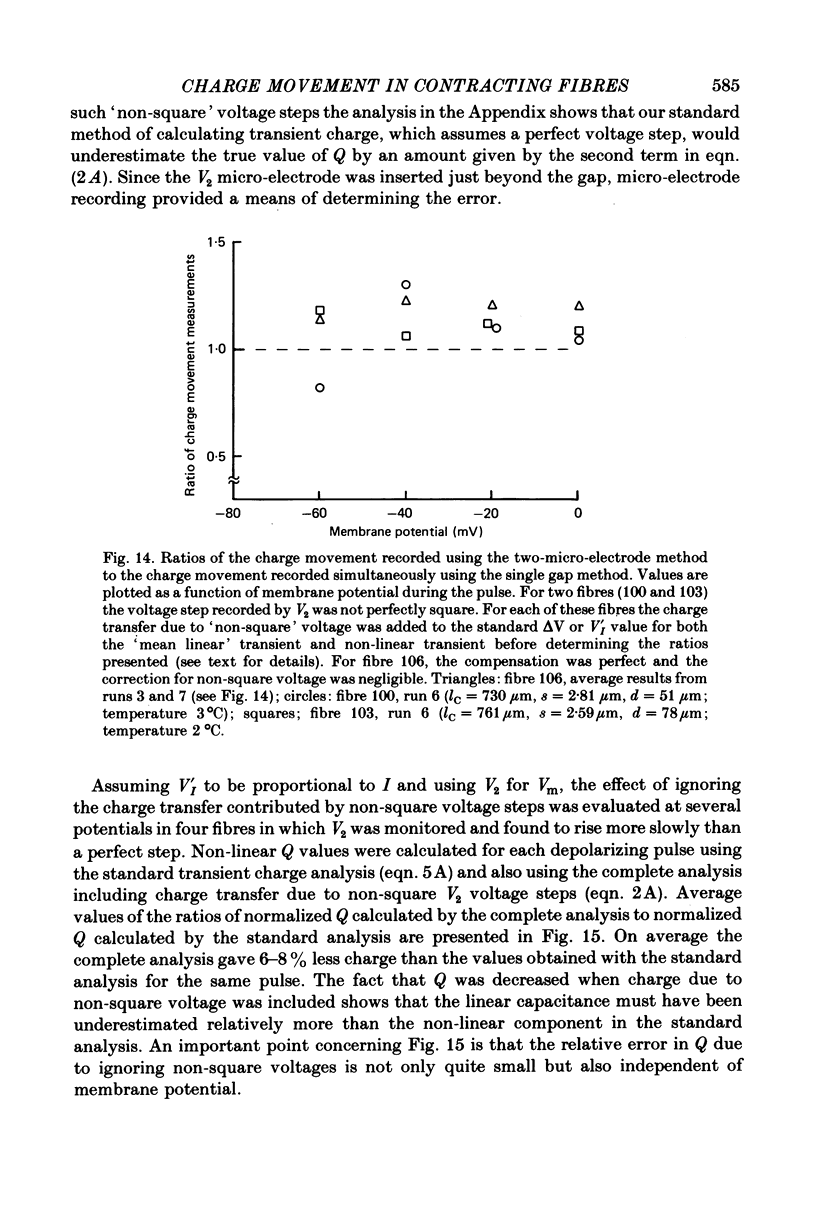
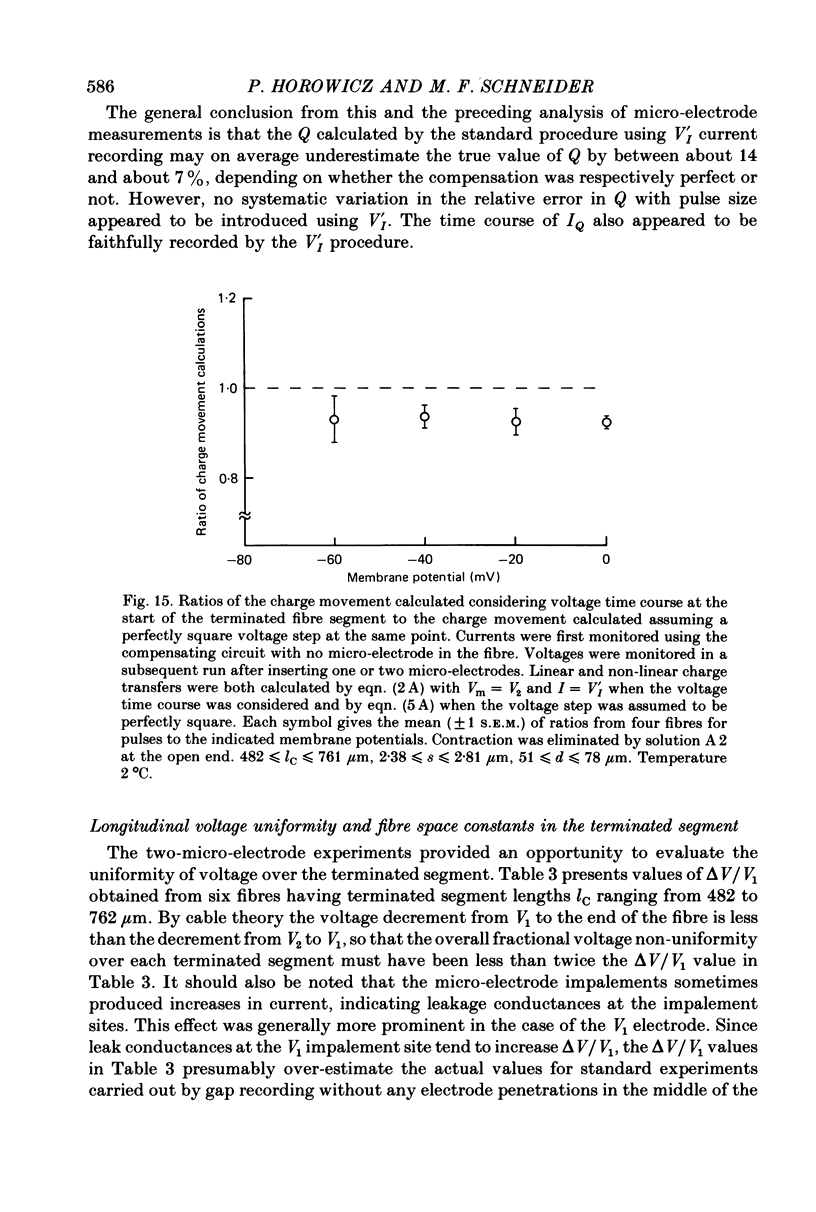
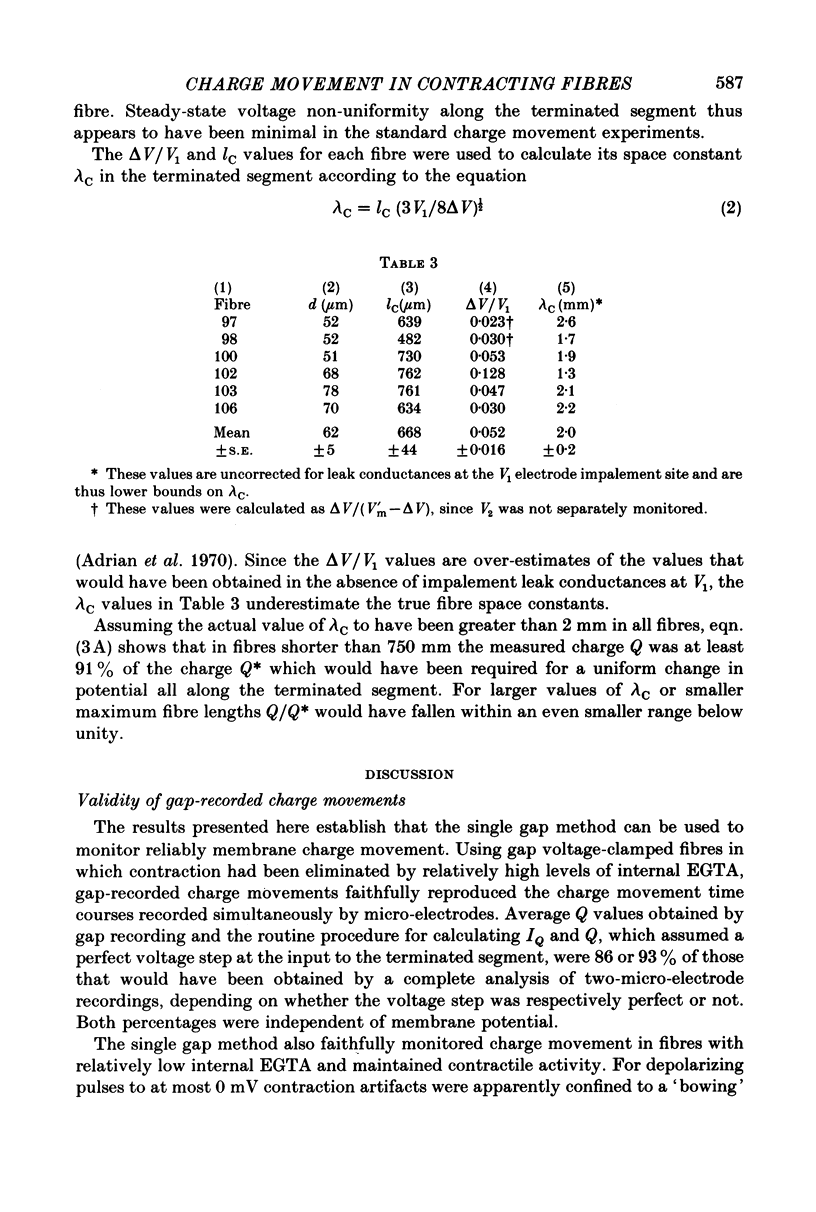
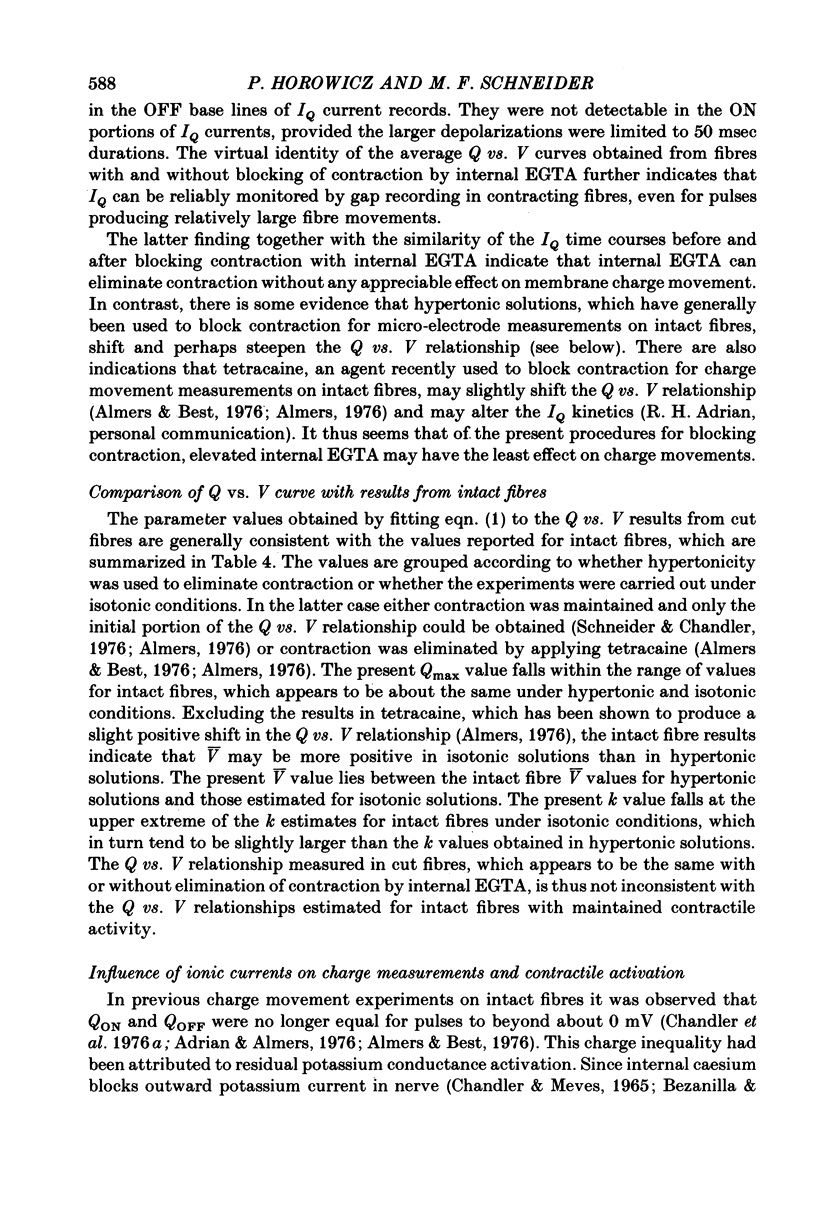
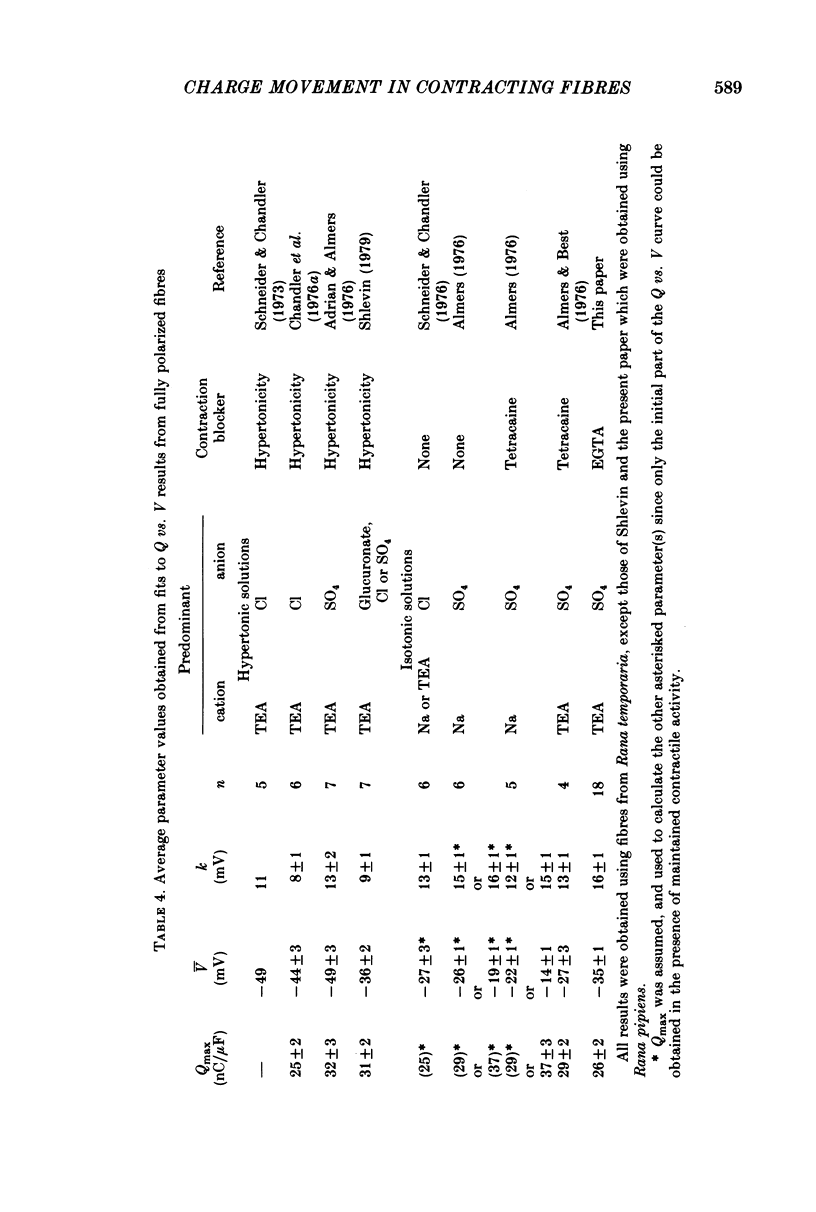
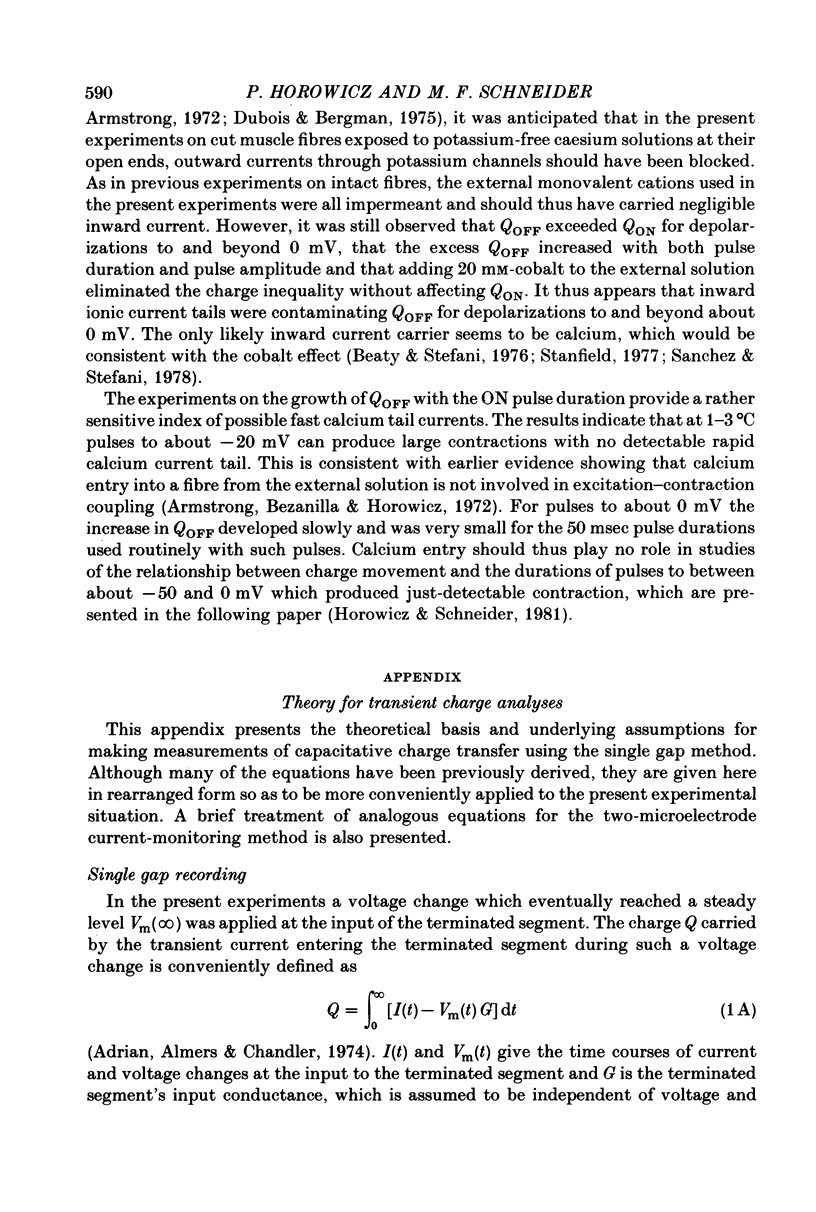
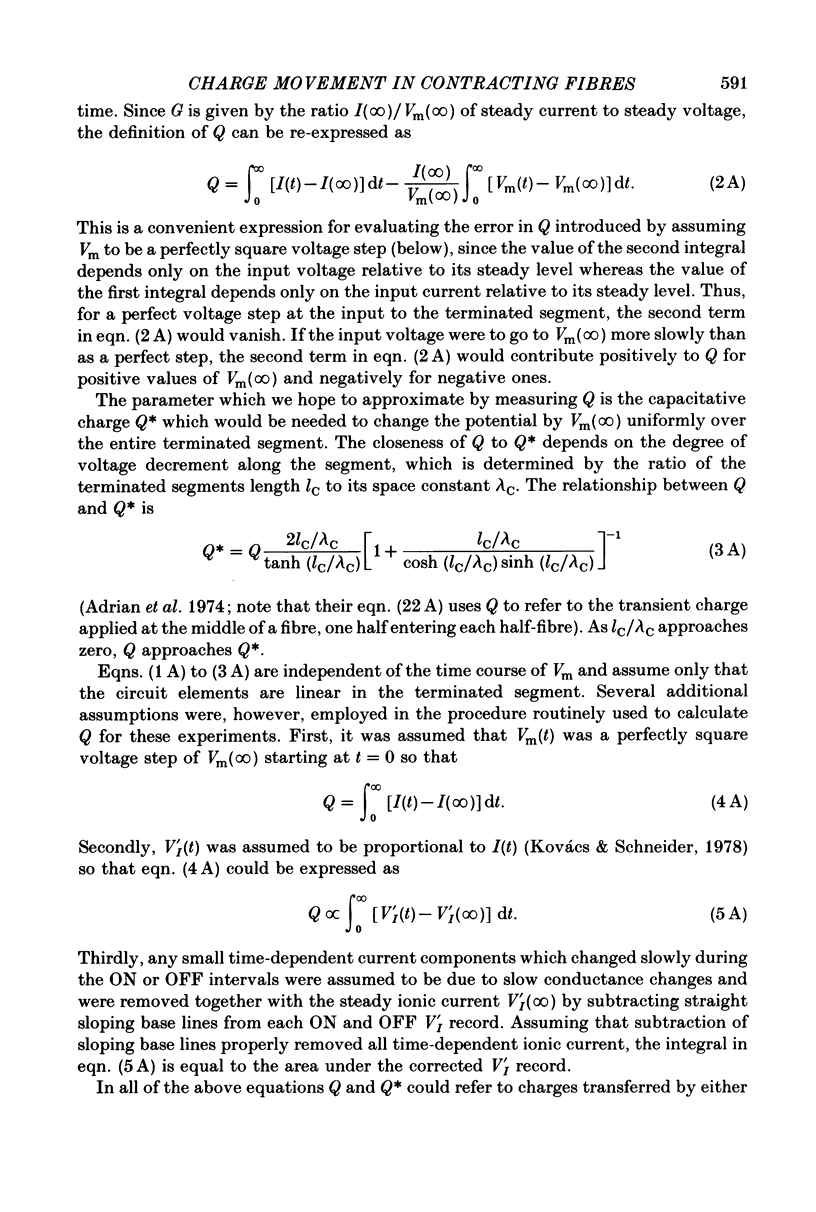
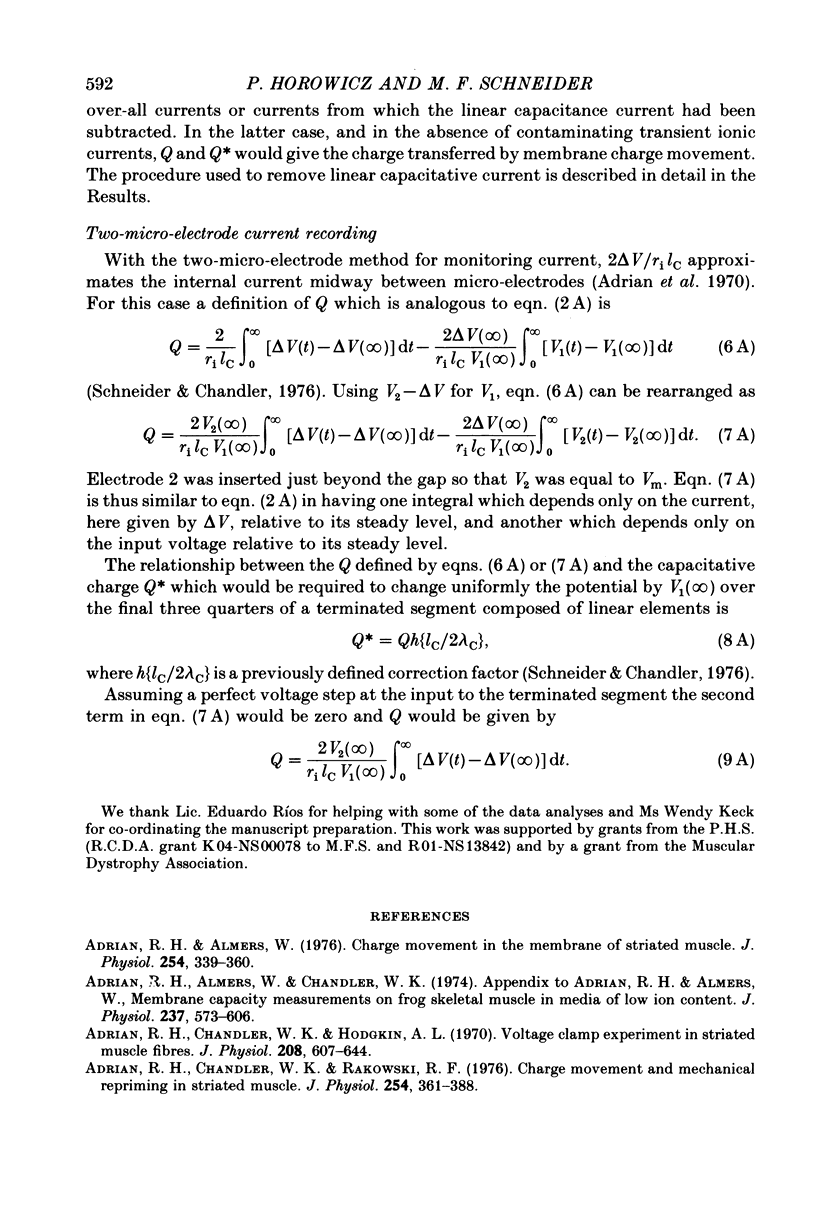
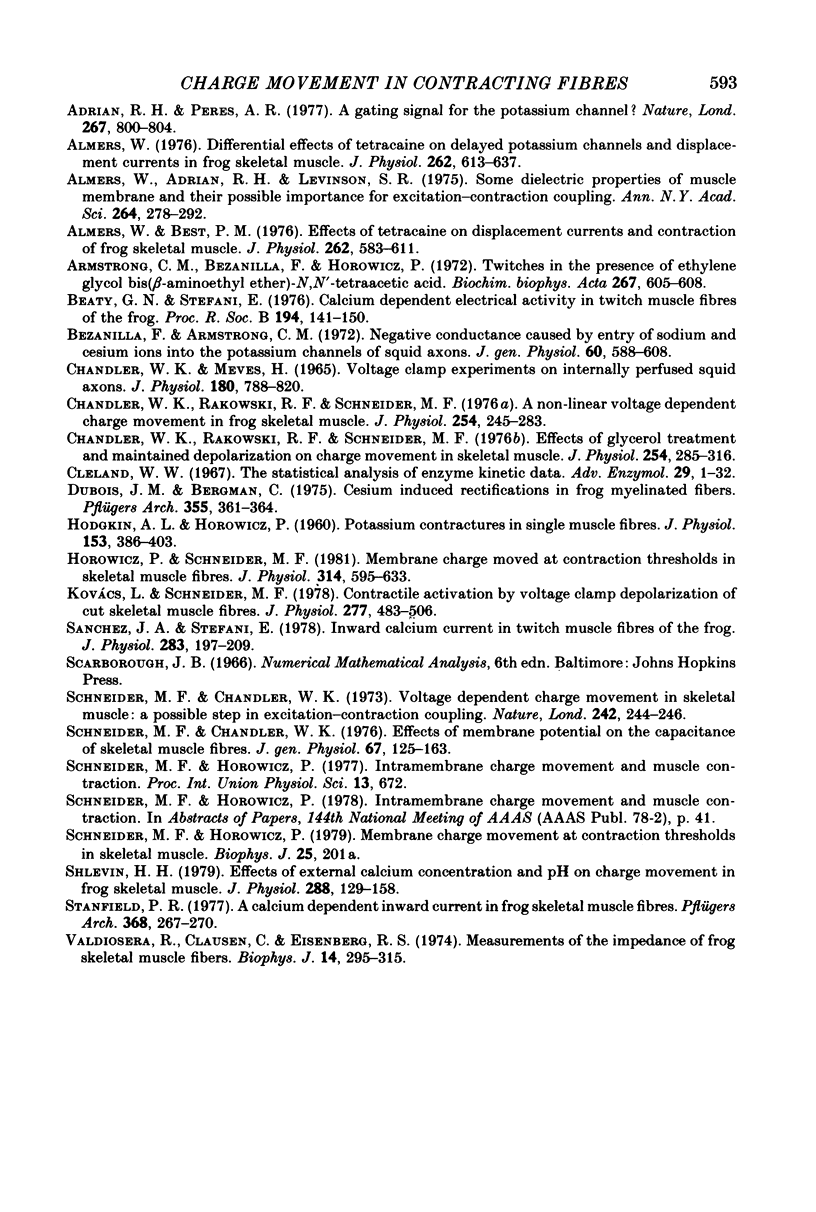
Selected References
These references are in PubMed. This may not be the complete list of references from this article.
- Adrian R. H., Almers W. Charge movement in the membrane of striated muscle. J Physiol. 1976 Jan;254(2):339–360. doi: 10.1113/jphysiol.1976.sp011235. [DOI] [PMC free article] [PubMed] [Google Scholar]
- Adrian R. H., Almers W. Membrane capacity measurements on frog skeletal muscle in media of low ion content. J Physiol. 1974 Mar;237(3):573–605. doi: 10.1113/jphysiol.1974.sp010499. [DOI] [PMC free article] [PubMed] [Google Scholar]
- Adrian R. H., Chandler W. K., Hodgkin A. L. Voltage clamp experiments in striated muscle fibres. J Physiol. 1970 Jul;208(3):607–644. doi: 10.1113/jphysiol.1970.sp009139. [DOI] [PMC free article] [PubMed] [Google Scholar]
- Adrian R. H., Chandler W. K., Rakowski R. F. Charge movement and mechanical repriming in skeletal muscle. J Physiol. 1976 Jan;254(2):361–388. doi: 10.1113/jphysiol.1976.sp011236. [DOI] [PMC free article] [PubMed] [Google Scholar]
- Adrian R. H., Peres A. R. A gating signal for the potassium channel? Nature. 1977 Jun 30;267(5614):800–804. doi: 10.1038/267800a0. [DOI] [PubMed] [Google Scholar]
- Almers W., Best P. M. Effects of tetracaine on displacement currents and contraction of frog skeletal muscle. J Physiol. 1976 Nov;262(3):583–611. doi: 10.1113/jphysiol.1976.sp011611. [DOI] [PMC free article] [PubMed] [Google Scholar]
- Almers W. Differential effects of tetracaine on delayed potassium channels and displacement currents in frog skeletal muscle. J Physiol. 1976 Nov;262(3):613–637. doi: 10.1113/jphysiol.1976.sp011612. [DOI] [PMC free article] [PubMed] [Google Scholar]
- Almers W. Some dielectric properties of muscle membrane and their possible importance for excitation-contraction coupling. Ann N Y Acad Sci. 1975 Dec 30;264:278–292. doi: 10.1111/j.1749-6632.1975.tb31489.x. [DOI] [PubMed] [Google Scholar]
- Armstrong C. M., Bezanilla F. M., Horowicz P. Twitches in the presence of ethylene glycol bis( -aminoethyl ether)-N,N'-tetracetic acid. Biochim Biophys Acta. 1972 Jun 23;267(3):605–608. doi: 10.1016/0005-2728(72)90194-6. [DOI] [PubMed] [Google Scholar]
- Beaty G. N., Stefani E. Calcium dependent electrical activity in twitch muscle fibres of the frog. Proc R Soc Lond B Biol Sci. 1976 Aug 27;194(1114):141–150. doi: 10.1098/rspb.1976.0070. [DOI] [PubMed] [Google Scholar]
- Bezanilla F., Armstrong C. M. Negative conductance caused by entry of sodium and cesium ions into the potassium channels of squid axons. J Gen Physiol. 1972 Nov;60(5):588–608. doi: 10.1085/jgp.60.5.588. [DOI] [PMC free article] [PubMed] [Google Scholar]
- Chandler W. K., Meves H. Voltage clamp experiments on internally perfused giant axons. J Physiol. 1965 Oct;180(4):788–820. doi: 10.1113/jphysiol.1965.sp007732. [DOI] [PMC free article] [PubMed] [Google Scholar]
- Chandler W. K., Rakowski R. F., Schneider M. F. A non-linear voltage dependent charge movement in frog skeletal muscle. J Physiol. 1976 Jan;254(2):245–283. doi: 10.1113/jphysiol.1976.sp011232. [DOI] [PMC free article] [PubMed] [Google Scholar]
- Chandler W. K., Rakowski R. F., Schneider M. F. Effects of glycerol treatment and maintained depolarization on charge movement in skeletal muscle. J Physiol. 1976 Jan;254(2):285–316. doi: 10.1113/jphysiol.1976.sp011233. [DOI] [PMC free article] [PubMed] [Google Scholar]
- Cleland W. W. The statistical analysis of enzyme kinetic data. Adv Enzymol Relat Areas Mol Biol. 1967;29:1–32. doi: 10.1002/9780470122747.ch1. [DOI] [PubMed] [Google Scholar]
- Dubois J. M., Bergman C. Cesium induced rectifications in frog myelinated fibres. Pflugers Arch. 1975 Apr 2;355(4):361–364. doi: 10.1007/BF00579857. [DOI] [PubMed] [Google Scholar]
- HODGKIN A. L., HOROWICZ P. Potassium contractures in single muscle fibres. J Physiol. 1960 Sep;153:386–403. doi: 10.1113/jphysiol.1960.sp006541. [DOI] [PMC free article] [PubMed] [Google Scholar]
- Horowicz P., Schneider M. F. Membrane charge moved at contraction thresholds in skeletal muscle fibres. J Physiol. 1981 May;314:595–633. doi: 10.1113/jphysiol.1981.sp013726. [DOI] [PMC free article] [PubMed] [Google Scholar]
- Kovács L., Schneider M. F. Contractile activation by voltage clamp depolarization of cut skeletal muscle fibres. J Physiol. 1978 Apr;277:483–506. doi: 10.1113/jphysiol.1978.sp012286. [DOI] [PMC free article] [PubMed] [Google Scholar]
- Sanchez J. A., Stefani E. Inward calcium current in twitch muscle fibres of the frog. J Physiol. 1978 Oct;283:197–209. doi: 10.1113/jphysiol.1978.sp012496. [DOI] [PMC free article] [PubMed] [Google Scholar]
- Schneider M. F., Chandler W. K. Effects of membrane potential on the capacitance of skeletal muscle fibers. J Gen Physiol. 1976 Feb;67(2):125–163. doi: 10.1085/jgp.67.2.125. [DOI] [PMC free article] [PubMed] [Google Scholar]
- Schneider M. F., Chandler W. K. Voltage dependent charge movement of skeletal muscle: a possible step in excitation-contraction coupling. Nature. 1973 Mar 23;242(5395):244–246. doi: 10.1038/242244a0. [DOI] [PubMed] [Google Scholar]
- Shlevin H. H. Effects of external calcium concentration and pH on charge movement in frog skeletal muscle. J Physiol. 1979 Mar;288:129–158. [PMC free article] [PubMed] [Google Scholar]
- Stanfield P. R. A calcium dependent inward current in frog skeletal muscle fibres. Pflugers Arch. 1977 Apr 25;368(3):267–270. doi: 10.1007/BF00585206. [DOI] [PubMed] [Google Scholar]
- Valdiosera R., Clausen C., Eisenberg R. S. Measurement of the impedance of frog skeletal muscle fibers. Biophys J. 1974 Apr;14(4):295–315. doi: 10.1016/S0006-3495(74)85917-5. [DOI] [PMC free article] [PubMed] [Google Scholar]