Abstract
1. Cell membrane potentials were measured with intracellular 3 M-CKl microelectrodes in isolated, perfused segments of the straight portion of the rabbit proximal tubule. 2. Under in vitro conditions simulating the in vivo situation, the transepithelial potential difference was about 1.6 mV, lumen-positive, and the basolateral membrane potential was 61 mV, cell negative. 3. Isomolar single ion substitutions in the bath (K+ for Na+, isethionate for Cl-, N-methyl-D-glucamine (NMDG+) for Na+, and Cl- for HCO3-) resulted in significant basolateral membrane potential changes only when [K+] was increased and [HCO3-] was reduced; in both cases the basolateral membrane depolarized. Cl- and Na+ substitutions with large monovalent ions did not change basolateral membrane potential. 4. Transepithelial potential changes in substitution experiments suggest that, at the paracellular pathway, PK greater than PNa greater than PNMDG, and PCl greater than Pisethionate. 5. It is concluded that the basolateral membrane of these cells is mainly K+-conductive and that electrodiffusional PNa and PCl are undetectable by this technique. 6. Addition of 1 mM-Ba2+ to the bath reduced basolateral membrane electro-diffusional PK, as evidenced by depolarization and by a reduction of the magnitude of the change in membrane potential produced by increasing bath [K+]. 7. The depolarization produced by lowering bath [HCO3-] appears to result from a reduction of electrodiffusional PK, since it is blocked by Ba2+. There is no need to postulate a conductive pathway for HCO3- or a related species.
Full text
PDF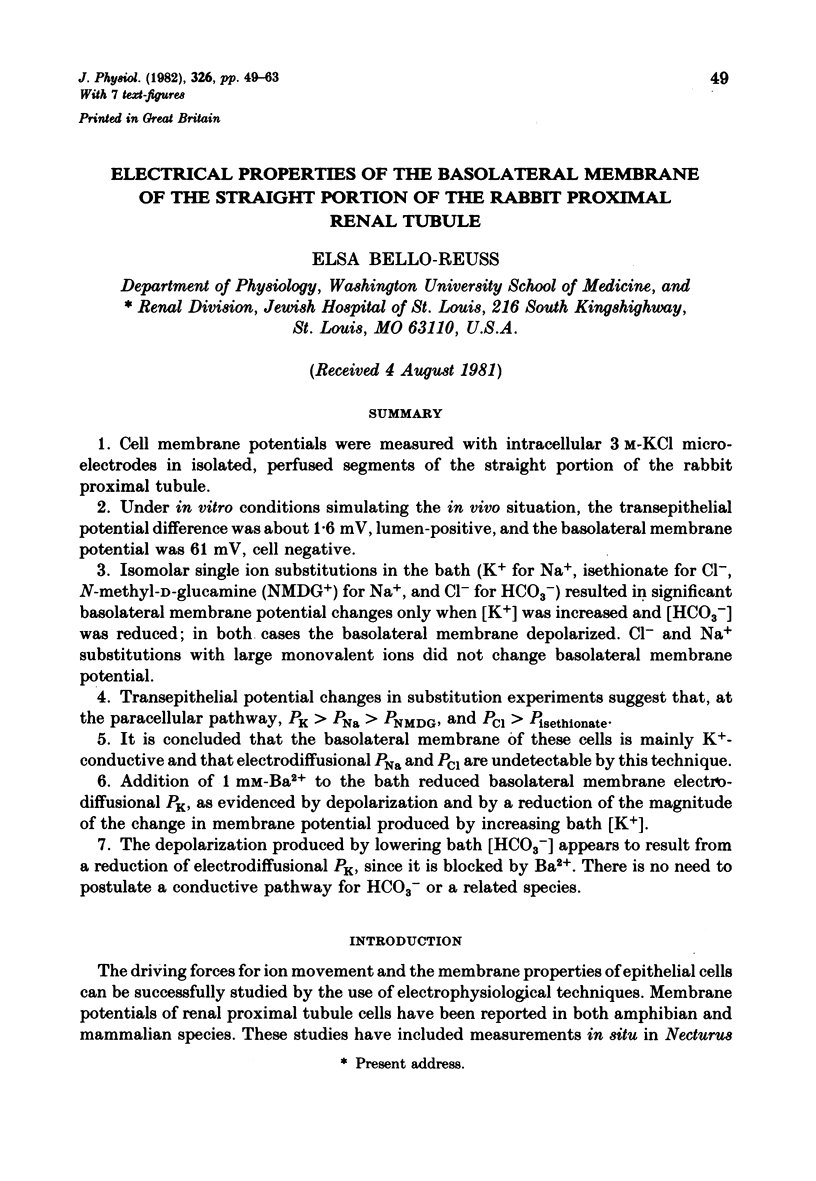
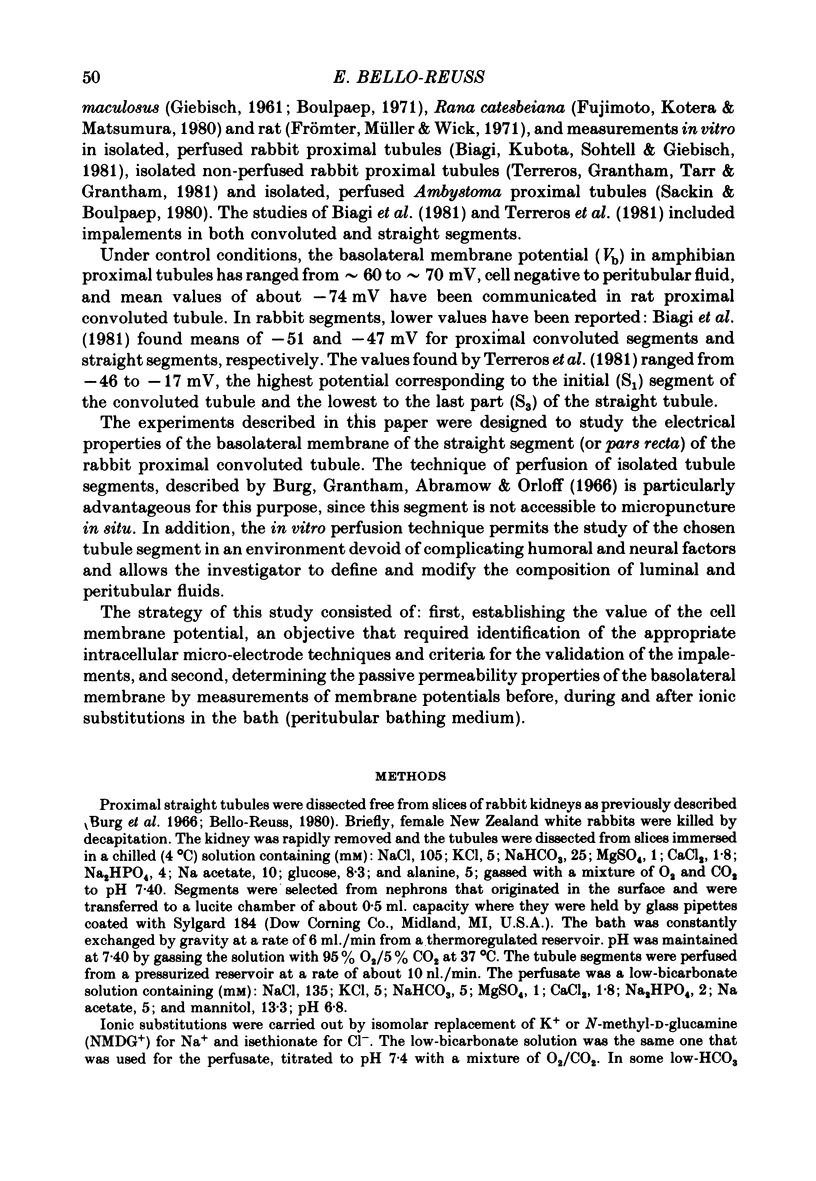
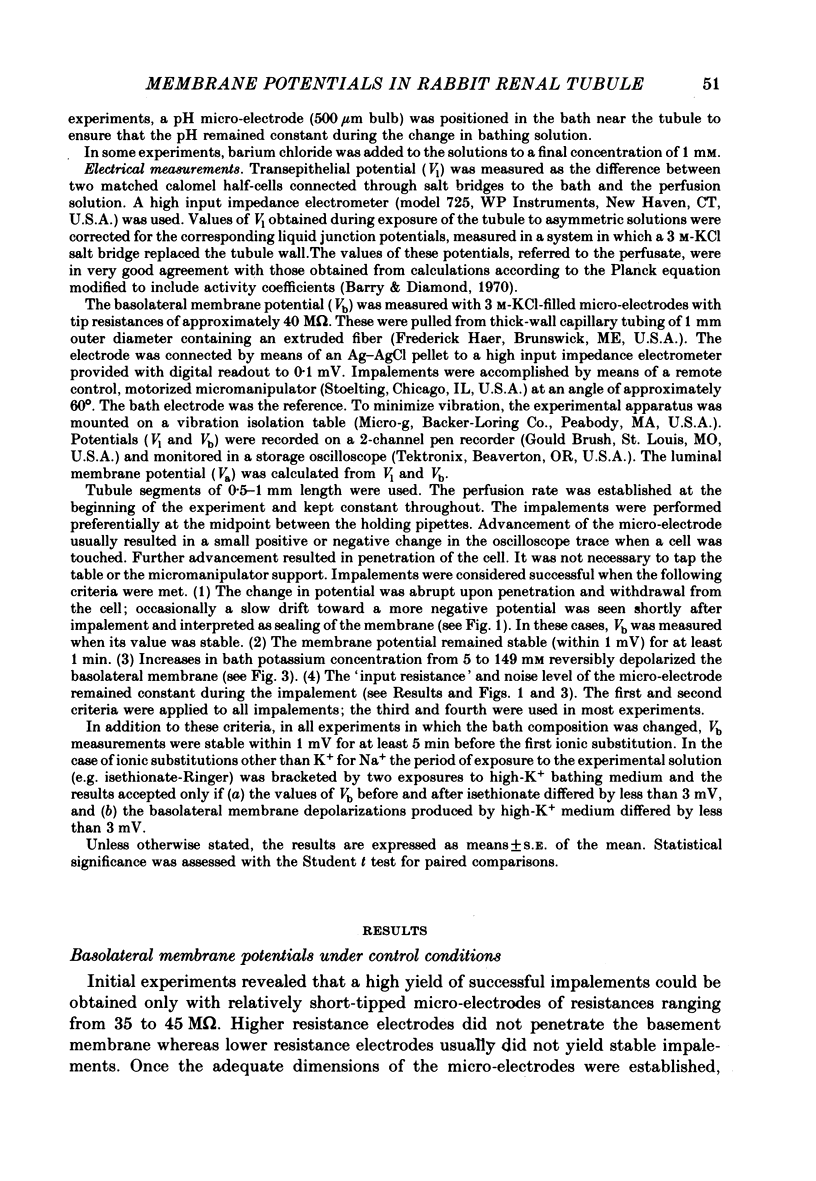
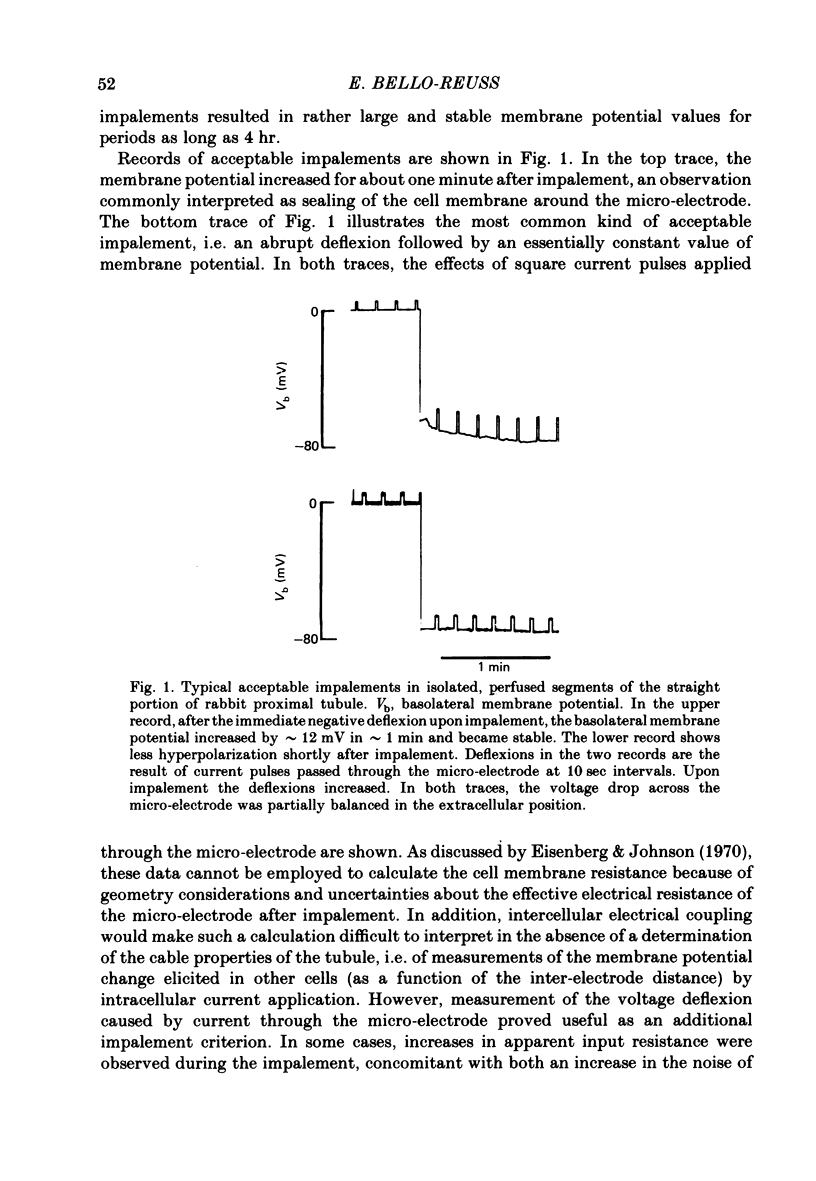
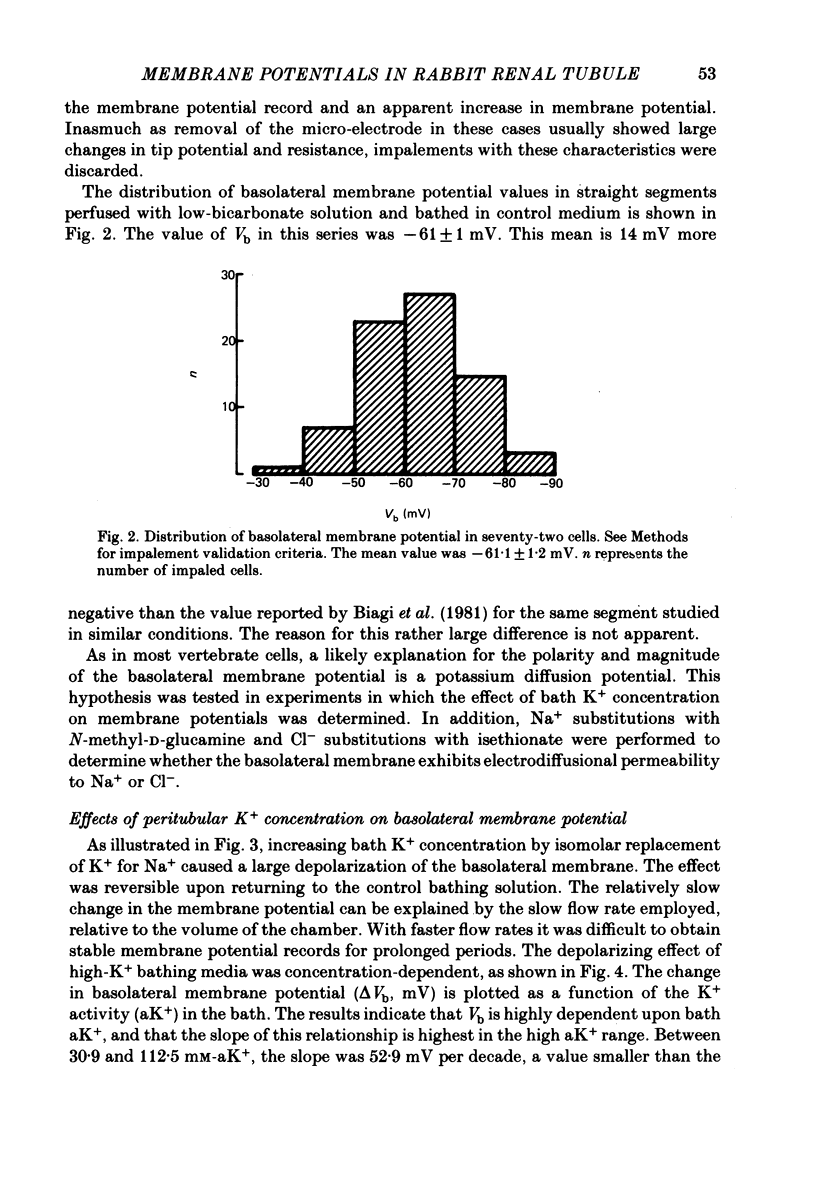
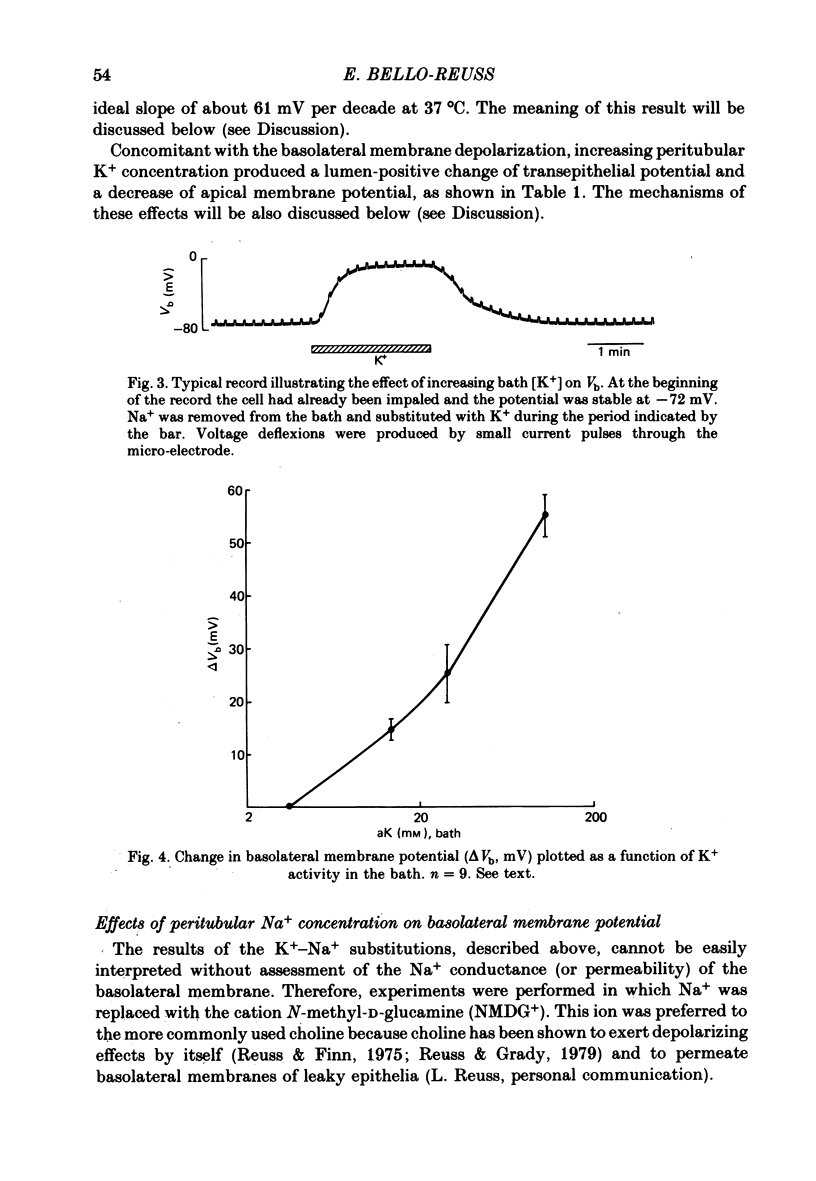
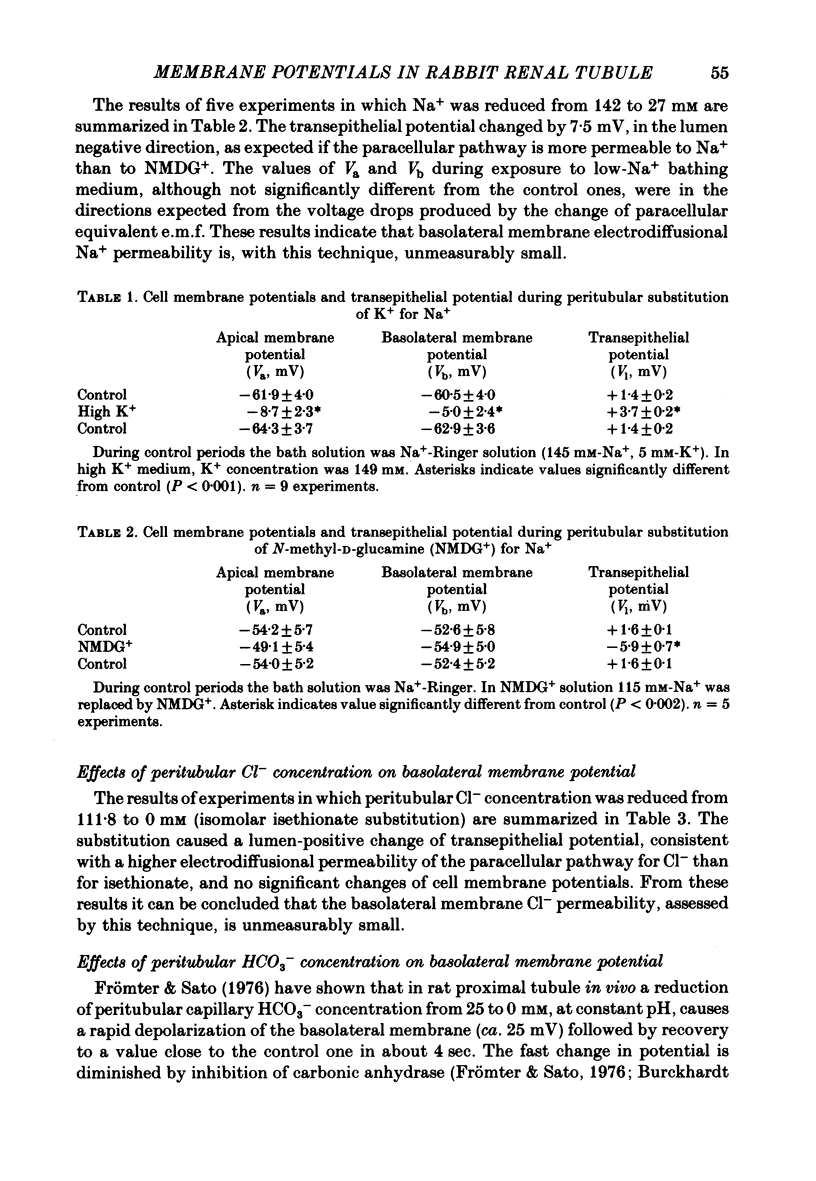
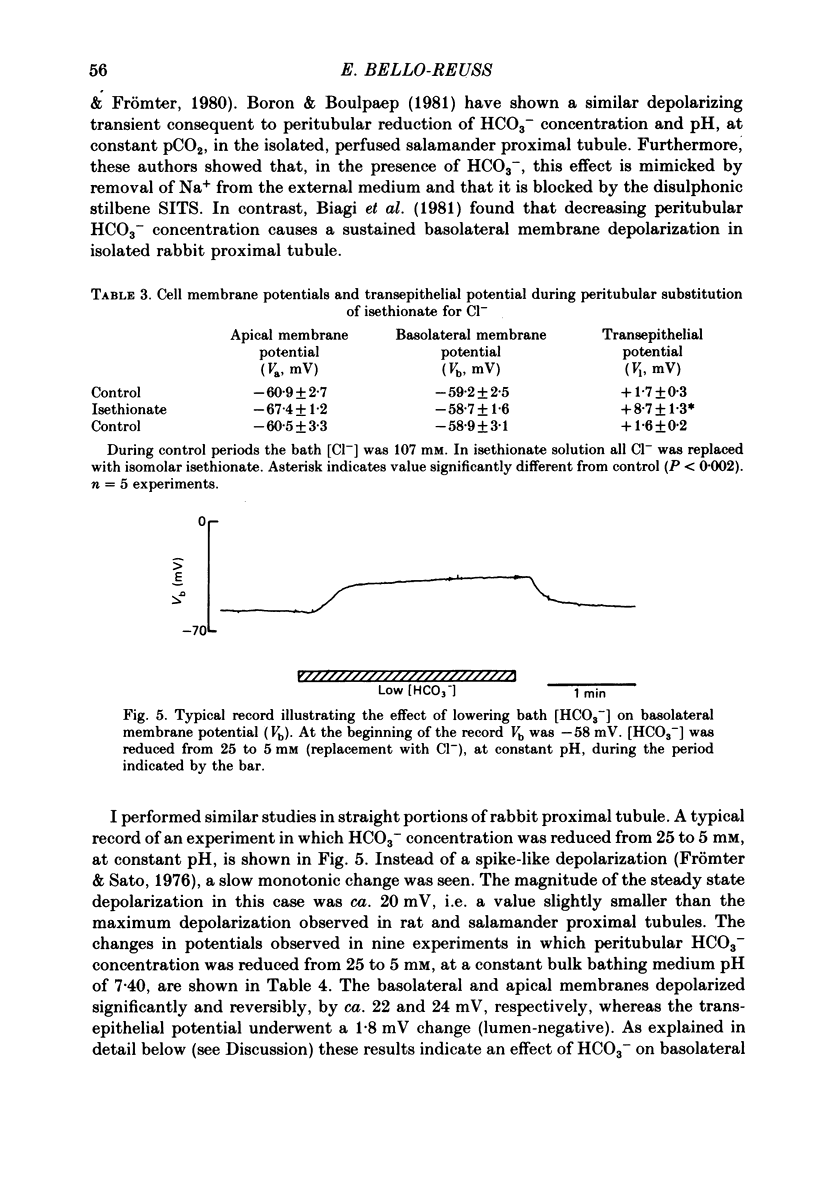
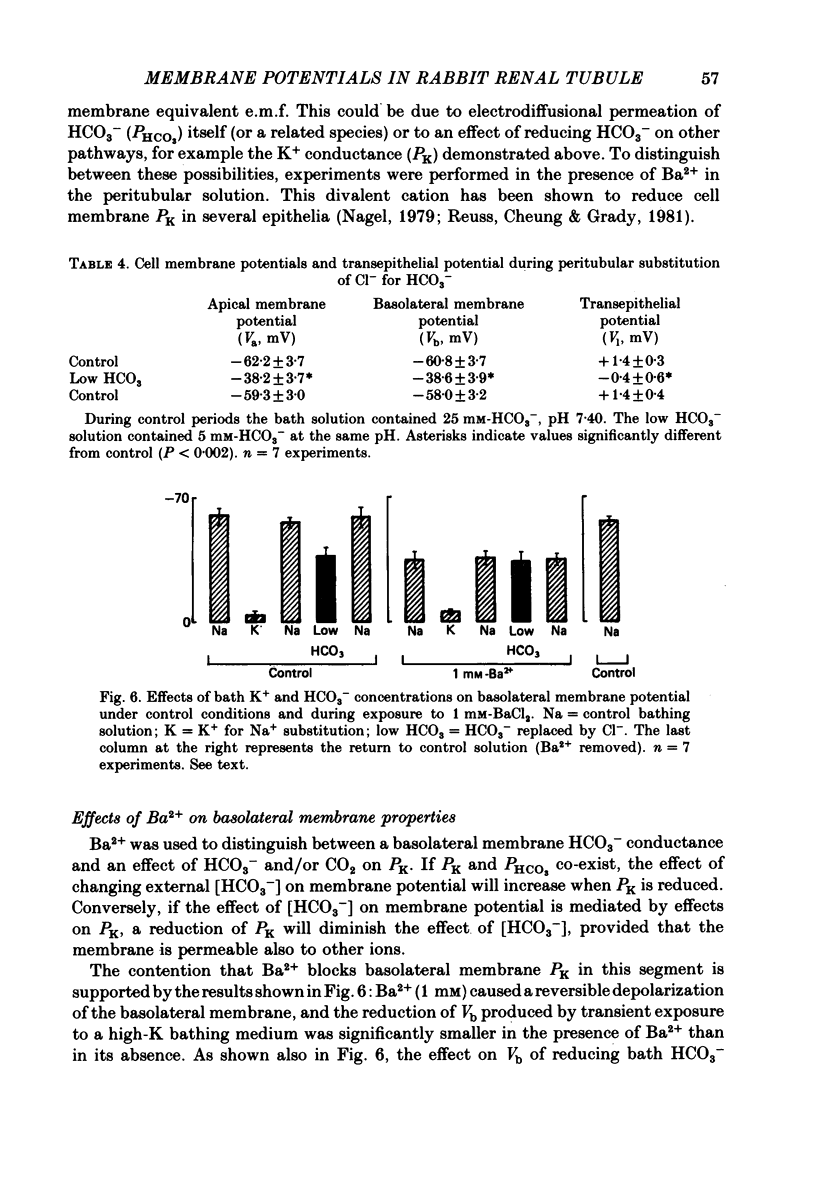
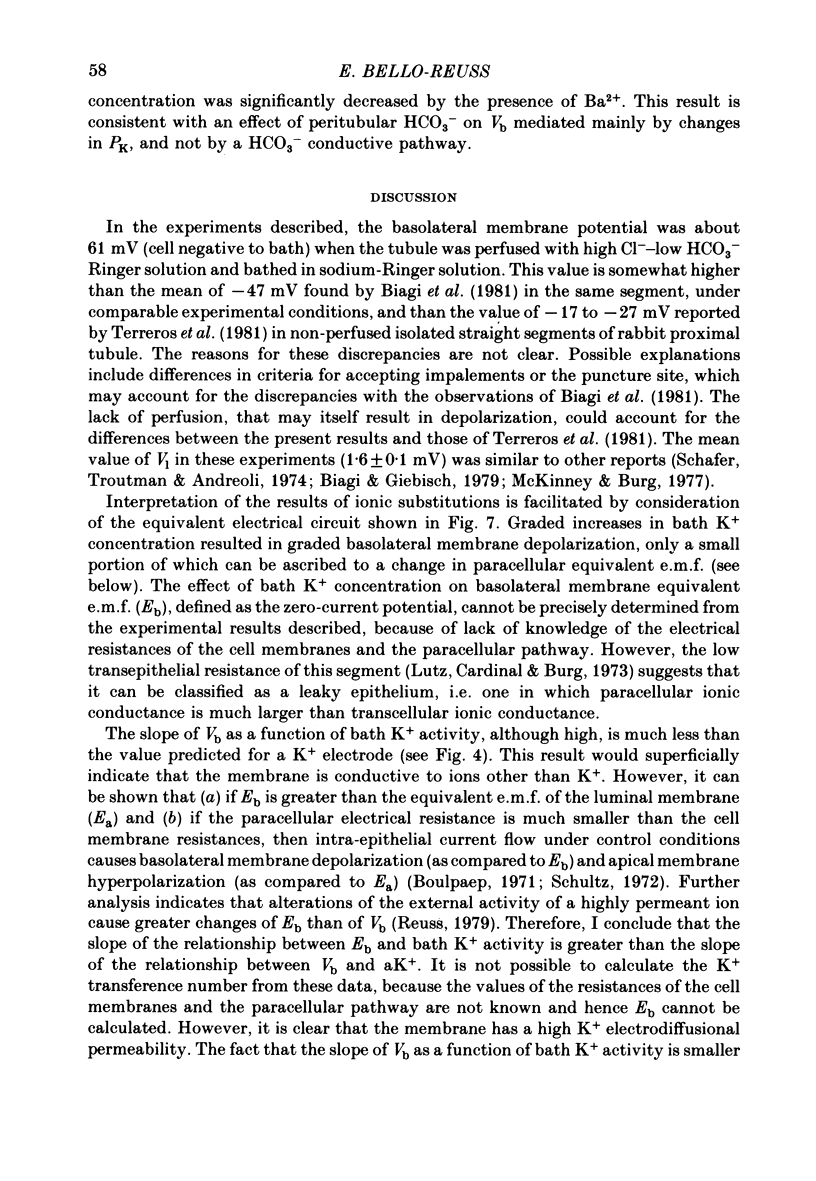
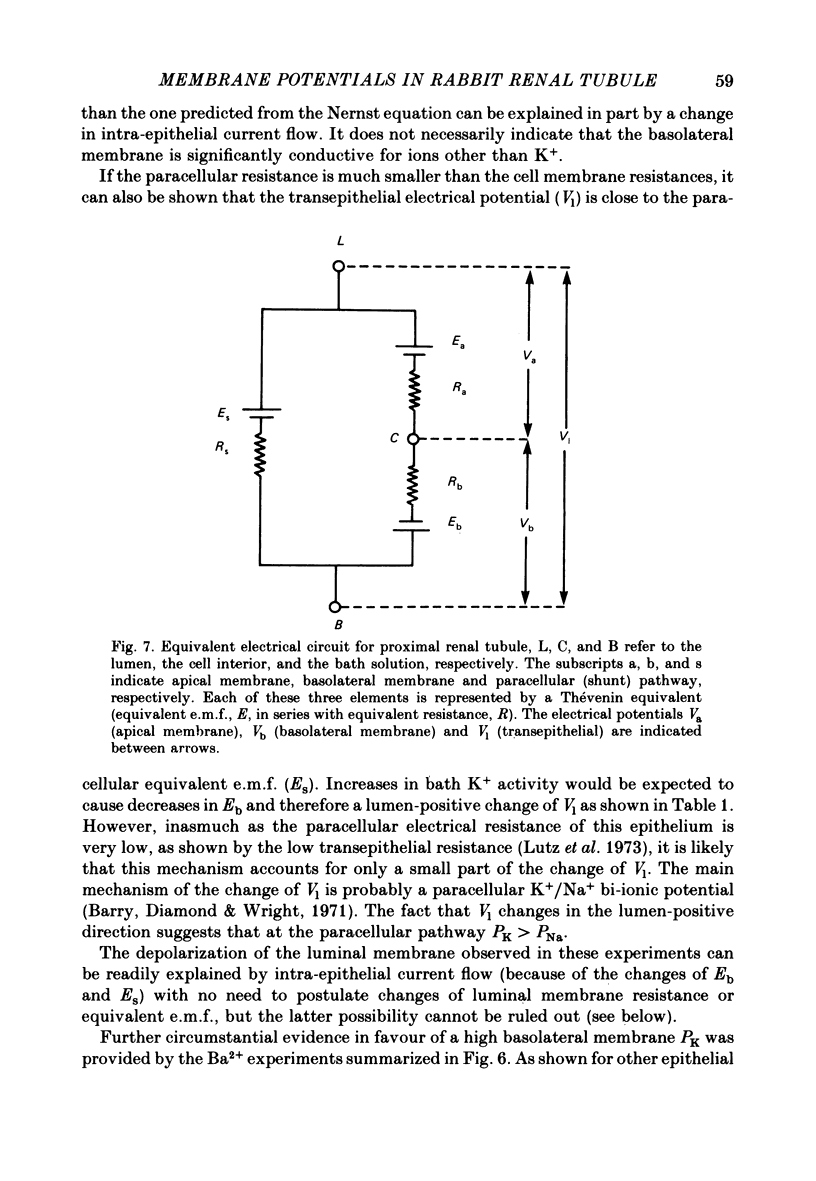
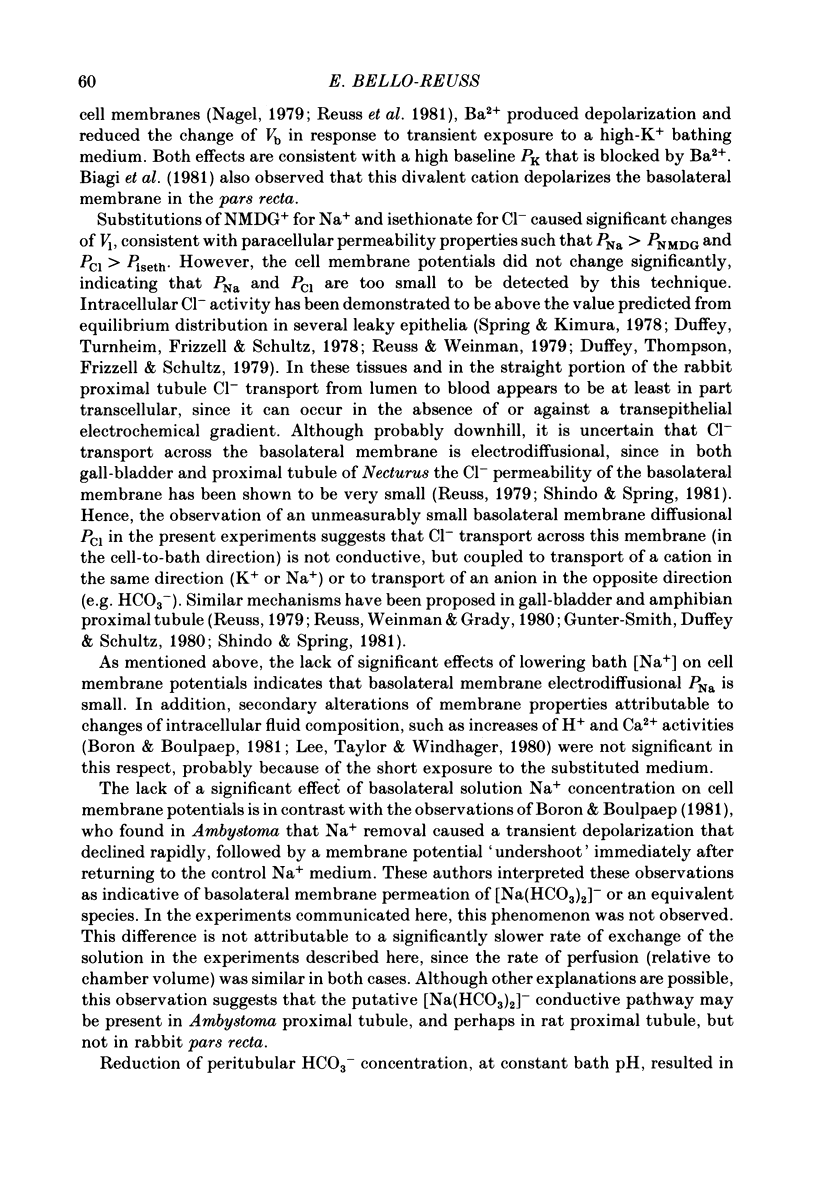
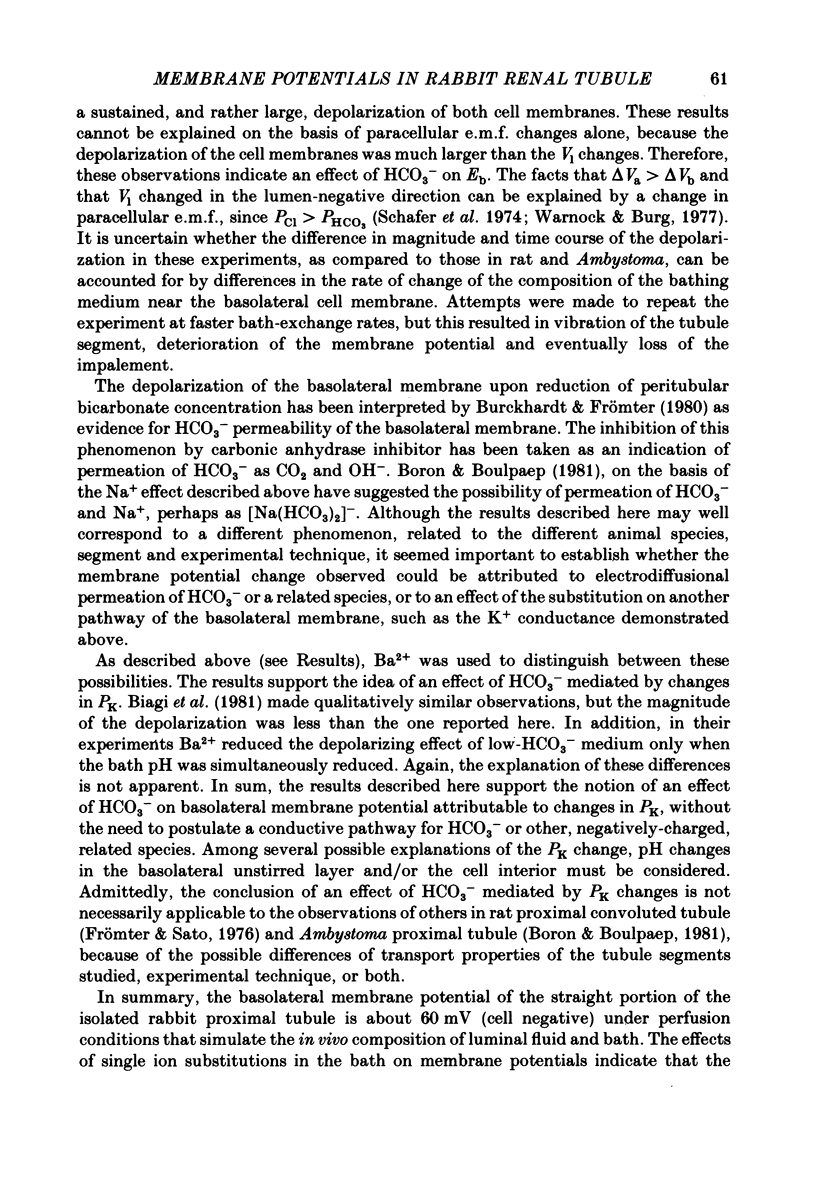
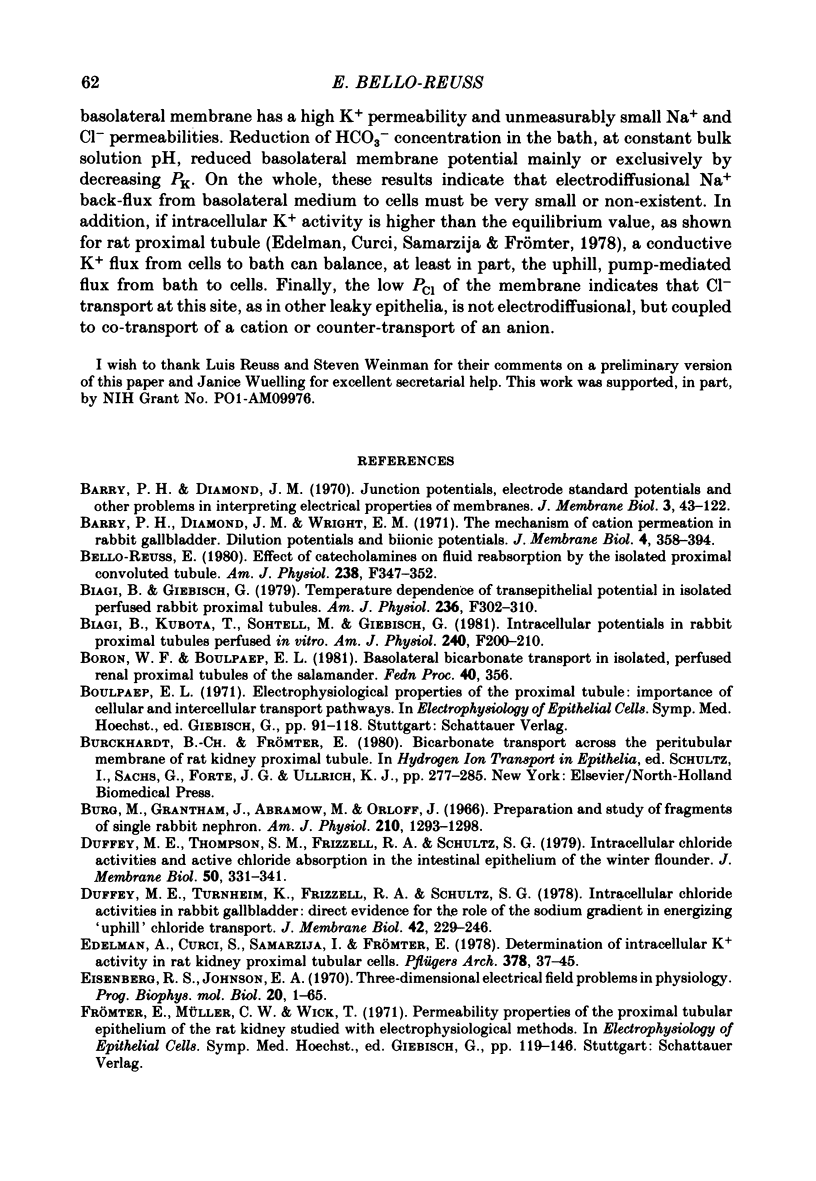
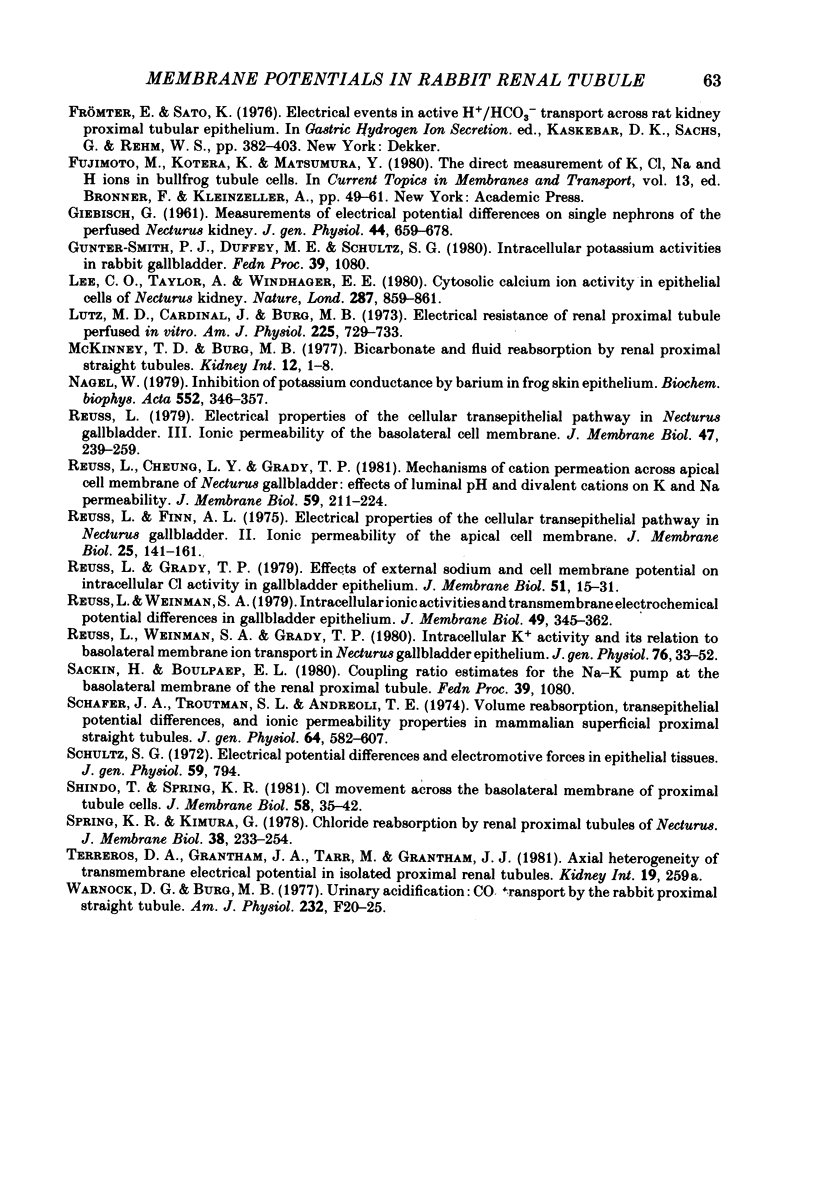
Selected References
These references are in PubMed. This may not be the complete list of references from this article.
- Bello-Reuss E. Effect of catecholamines on fluid reabsorption by the isolated proximal convoluted tubule. Am J Physiol. 1980 May;238(5):F347–F352. doi: 10.1152/ajprenal.1980.238.5.F347. [DOI] [PubMed] [Google Scholar]
- Biagi B. A., Giebisch G. Temperature dependence of transepithelial potential in isolated perfused rabbit proximal tubules. Am J Physiol. 1979 Mar;236(3):F302–F310. doi: 10.1152/ajprenal.1979.236.3.F302. [DOI] [PubMed] [Google Scholar]
- Biagi B., Kubota T., Sohtell M., Giebisch G. Intracellular potentials in rabbit proximal tubules perfused in vitro. Am J Physiol. 1981 Mar;240(3):F200–F210. doi: 10.1152/ajprenal.1981.240.3.F200. [DOI] [PubMed] [Google Scholar]
- Burg M., Grantham J., Abramow M., Orloff J. Preparation and study of fragments of single rabbit nephrons. Am J Physiol. 1966 Jun;210(6):1293–1298. doi: 10.1152/ajplegacy.1966.210.6.1293. [DOI] [PubMed] [Google Scholar]
- Duffey M. E., Thompson S. M., Frizzell R. A., Schultz S. G. Intracellular chloride activities and active chloride absorption in the intestinal epithelium of the winter flounder. J Membr Biol. 1979 Nov 30;50(3-4):331–341. doi: 10.1007/BF01868896. [DOI] [PubMed] [Google Scholar]
- Duffey M. E., Turnheim K., Frizzell R. A., Schultz S. G. Intracellular chloride activities in rabbit gallbladder: direct evidence for the role of the sodium-gradient in energizing "uphill" chloride transport. J Membr Biol. 1978 Sep 19;42(3):229–245. doi: 10.1007/BF01870360. [DOI] [PubMed] [Google Scholar]
- Edelman A., Curci S., Samarzija I., Frömter E. Determination of intracellular K+ activity in rat kidney proximal tubular cells. Pflugers Arch. 1978 Dec 15;378(1):37–45. doi: 10.1007/BF00581956. [DOI] [PubMed] [Google Scholar]
- GIEBISCH G. Measurements of electrical potential differences on single nephrons of the perfused Necturus kidney. J Gen Physiol. 1961 Mar;44:659–678. doi: 10.1085/jgp.44.4.659. [DOI] [PMC free article] [PubMed] [Google Scholar]
- Lee C. O., Taylor A., Windhager E. E. Cytosolic calcium ion activity in epithelial cells of Necturus kidney. Nature. 1980 Oct 30;287(5785):859–861. doi: 10.1038/287859a0. [DOI] [PubMed] [Google Scholar]
- Lutz M. D., Cardinal J., Burg M. B. Electrical resistance of renal proximal tubule perfused in vitro. Am J Physiol. 1973 Sep;225(3):729–734. doi: 10.1152/ajplegacy.1973.225.3.729. [DOI] [PubMed] [Google Scholar]
- McKinney T. D., Burg M. B. Biocarbonate and fluid absorption by renal proximal straight tubules. Kidney Int. 1977 Jul;12(1):1–8. doi: 10.1038/ki.1977.72. [DOI] [PubMed] [Google Scholar]
- Nagel W. Inhibition of potassium conductance by barium in frog skin epithelium. Biochim Biophys Acta. 1979 Apr 4;552(2):346–357. doi: 10.1016/0005-2736(79)90289-x. [DOI] [PubMed] [Google Scholar]
- Reuss L., Cheung L. Y., Grady T. P. Mechanisms of cation permeation across apical cell membrane of Necturus gallbladder: effects of luminal pH and divalent cations on K+ and Na+ permeability. J Membr Biol. 1981 Apr 30;59(3):211–224. doi: 10.1007/BF01875426. [DOI] [PubMed] [Google Scholar]
- Reuss L. Electrical properties of the cellular transepithelial pathway in Necturus gallbladder: III. Ionic permeability of the basolateral cell membrane. J Membr Biol. 1979 May 25;47(3):239–259. doi: 10.1007/BF01869080. [DOI] [PubMed] [Google Scholar]
- Reuss L., Finn A. L. Electrical properties of the cellular transepithelial pathway in Necturus gallbladder. II. Ionic permeability of the apical cell membrane. J Membr Biol. 1975 Dec 4;25(1-2):141–161. doi: 10.1007/BF01868572. [DOI] [PubMed] [Google Scholar]
- Reuss L., Grady T. P. Effects of external sodium and cell membrane potential on intracellular chloride activity in gallbladder epithelium. J Membr Biol. 1979 Dec 12;51(1):15–31. doi: 10.1007/BF01869341. [DOI] [PubMed] [Google Scholar]
- Reuss L., Weinman S. A., Grady T. P. Intracellular K+ activity and its relation to basolateral membrane ion transport in Necturus gallbladder epithelium. J Gen Physiol. 1980 Jul;76(1):33–52. doi: 10.1085/jgp.76.1.33. [DOI] [PMC free article] [PubMed] [Google Scholar]
- Reuss L., Weinman S. A. Intracellular ionic activities and transmembrane electrochemical potential differences in gallbladder epithelium. J Membr Biol. 1979 Sep 14;49(4):345–362. doi: 10.1007/BF01868991. [DOI] [PubMed] [Google Scholar]
- Schafer J. A., Troutman S. L., Andreoli T. E. Volume reabsorption, transepithelial potential differences, and ionic permeability properties in mammalian superficial proximal straight tubules. J Gen Physiol. 1974 Nov;64(5):582–607. doi: 10.1085/jgp.64.5.582. [DOI] [PMC free article] [PubMed] [Google Scholar]
- Schultz S. G. Electrical potential differences and electromotive forces in epithelial tissues. J Gen Physiol. 1972 Jun;59(6):794–798. doi: 10.1085/jgp.59.6.794. [DOI] [PMC free article] [PubMed] [Google Scholar]
- Shindo T., Spring K. R. Chloride movement across the basolateral membrane of proximal tubule cells. J Membr Biol. 1981 Jan 30;58(1):35–42. doi: 10.1007/BF01871032. [DOI] [PubMed] [Google Scholar]
- Spring K. R., Kimura G. Chloride reabsorption by renal proximal tubules of Necturus. J Membr Biol. 1978 Jan 18;38(3):233–254. doi: 10.1007/BF01871924. [DOI] [PubMed] [Google Scholar]
- Warnock D. G., Burg M. B. Urinary acidification: CO2 transport by the rabbit proximal straight tubule. Am J Physiol. 1977 Jan;232(1):F20–F25. doi: 10.1152/ajprenal.1977.232.1.F20. [DOI] [PubMed] [Google Scholar]