Abstract
1. Miniature excitatory junctional currents (m.e.j.c.s) were examined in conditions where inward current was carried mainly by Na+ (i.e. in normal medium, Ca2+-free medium and Cl--free medium). M.e.j.c.s were also examined in isotonic Ca2+ where the inward post-synaptic current was carried mainly by Ca2+.
2. In normal medium, mean m.e.j.c. amplitude = 2.34±0.05 nA. The decay time constant of m.e.j.c.s (excluding a small percentage with abnormal shapes) was τm.e.j.c. = 2.62±0.11 msec (Vm = -80 mV, T = 22 °C). Decay-time was not markedly changed in Ca2+-free or Cl--free medium. τm.e.j.c. approaches the life-time of glutamate activated junctional channels.
3. Excitatory junctional currents, evoked by nerve impulses, decayed slightly faster than m.e.j.c.s obtained in the same fibres. Extracellularly recorded m.e.j.c.s and voltage-clamped m.e.j.c.s were similar in time course.
4. τm.e.j.c. decreased exponentially with membrane hyperpolarization. An e-fold change was produced by 182.±24.8 mV change in Vm.
5. The dependence of mean m.e.j.c. amplitude on clamp potential showed a slight non-linearity at hyperpolarized levels. The equilibrium potential for transmitter action was close to 0 mV in normal solution as well as in Ca2+-free and Cl--free solutions.
6. The kinetics of junctional channels are altered in isotonic Ca2+. M.e.j.c. amplitude was reduced to about one-third normal size; mean m.e.j.c. = 0.74±0.03 nA. The decay time becomes markedly briefer, τm.e.j.c. = 1.01±0.08 msec, indicating a reduction in mean channel life-time (Vm = -80 mV, T = 22 °C).
7. A population of slow time course and composite m.e.j.c.s appear when muscle fibres are hyperpolarized in isotonic Ca2+, thus producing a prolongation in mean τm.e.j.c.. This results from an influence of post-synaptic membrane potential on presynaptic transmitter release. If such m.e.j.c.s are ignored the voltage dependence of τm.e.j.c. of the remaining events is abolished or even reversed indicating that voltage sensitivity of channel life-time is altered in isotonic Ca2+. The equilibrium potential for transmitter action may be slightly more positive than normal.
8. We estimate that a single packet of neurally released transmitter normally opens, on average, 250 ion channels at these junctions.
Full text
PDF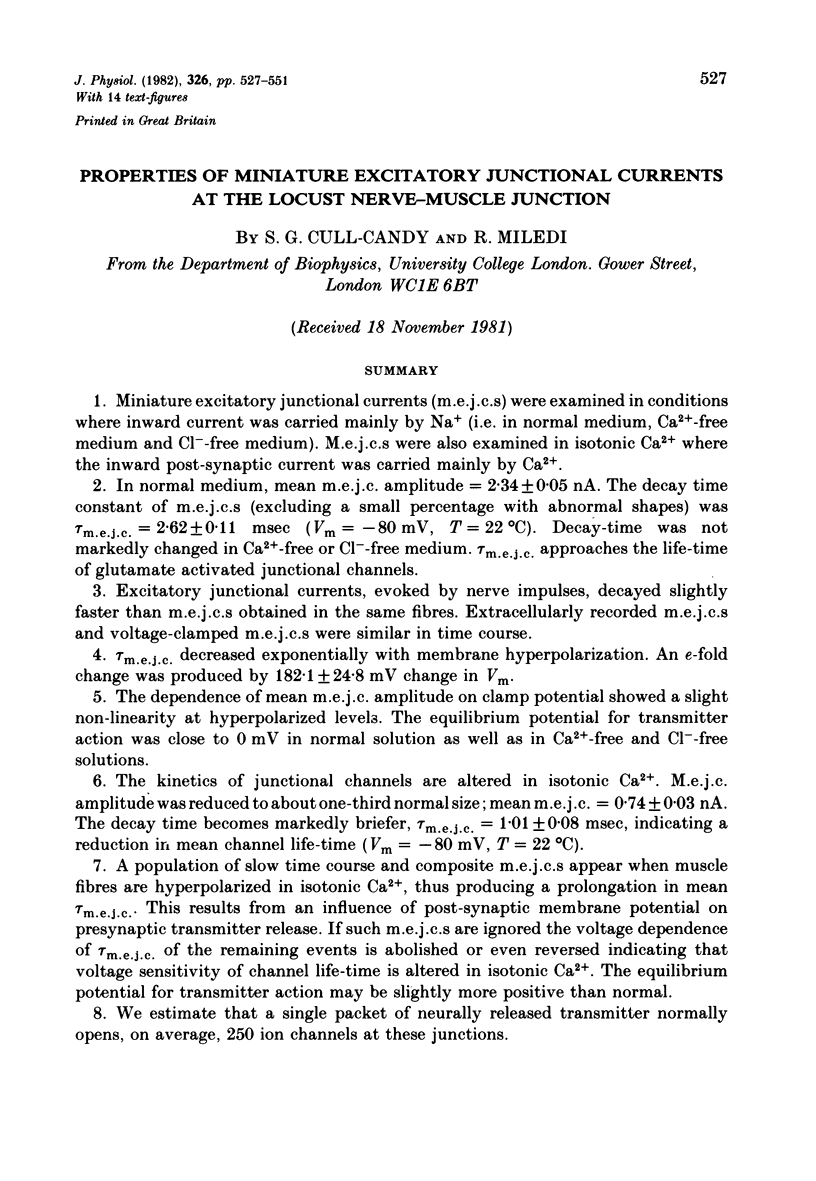
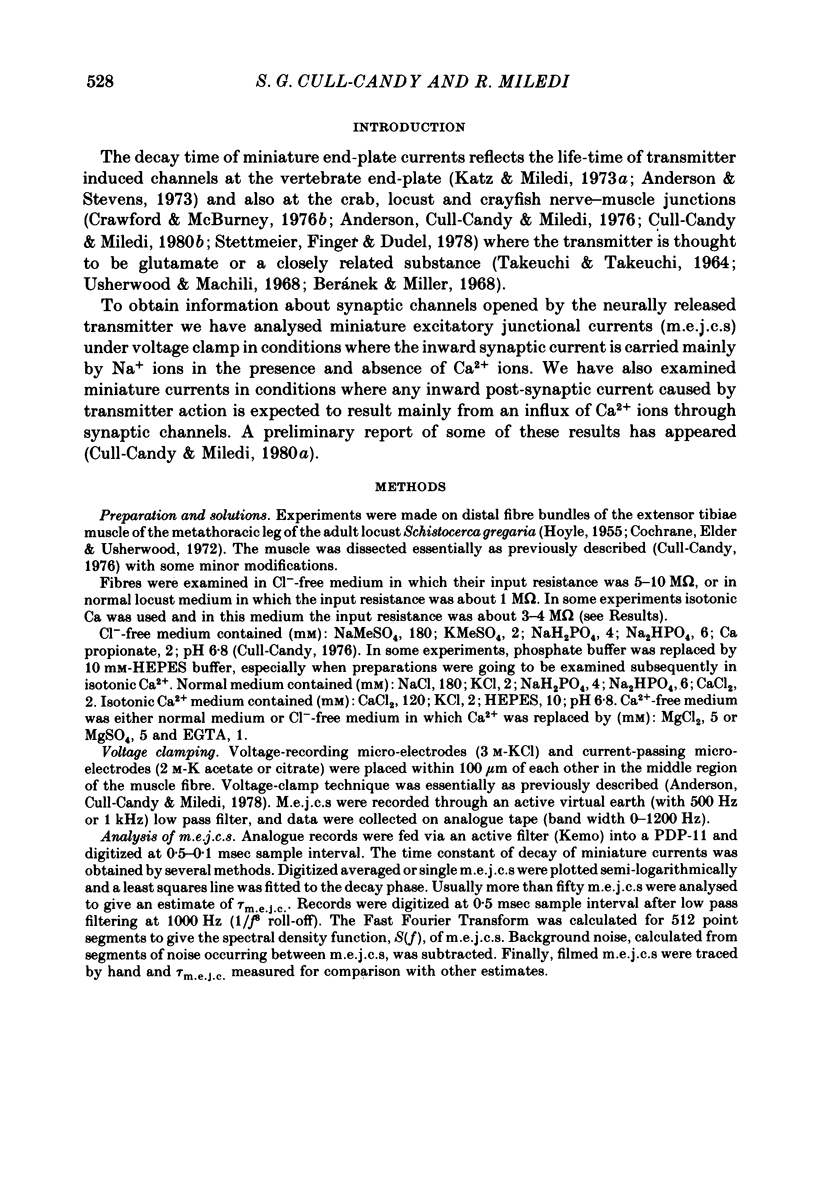
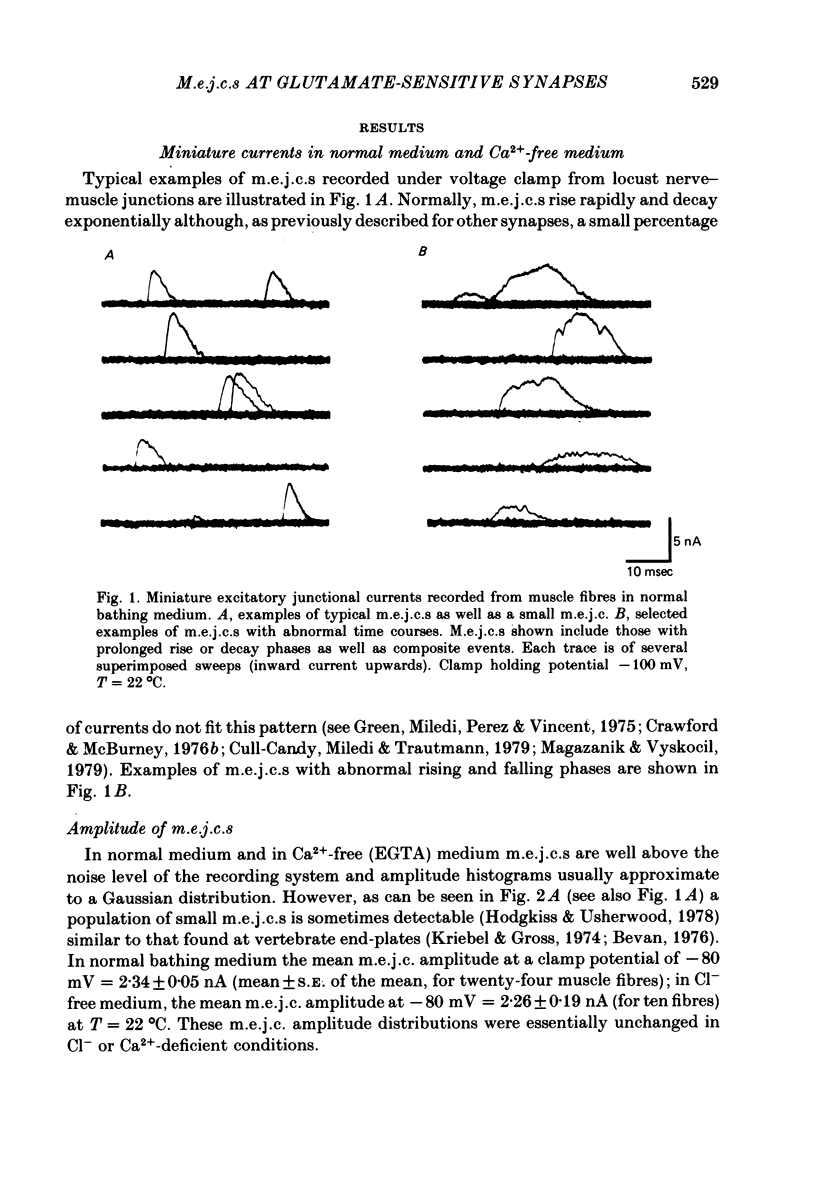
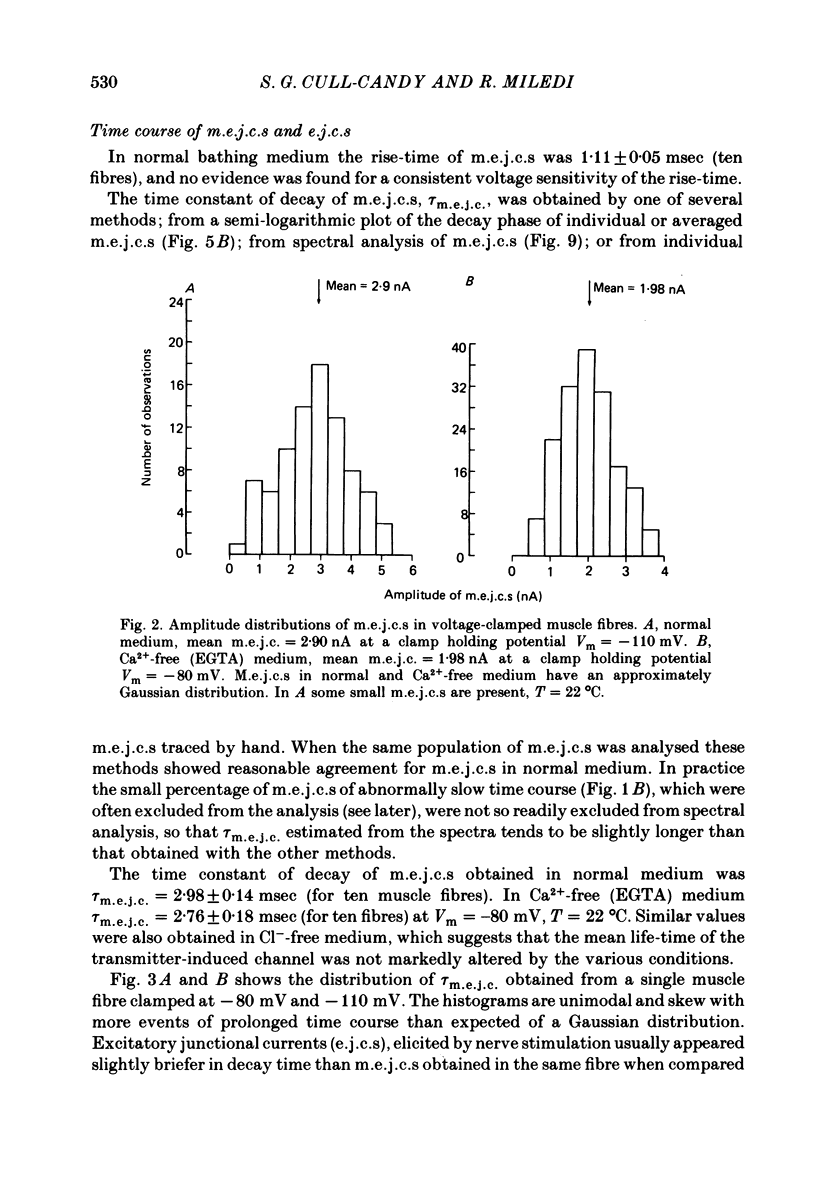
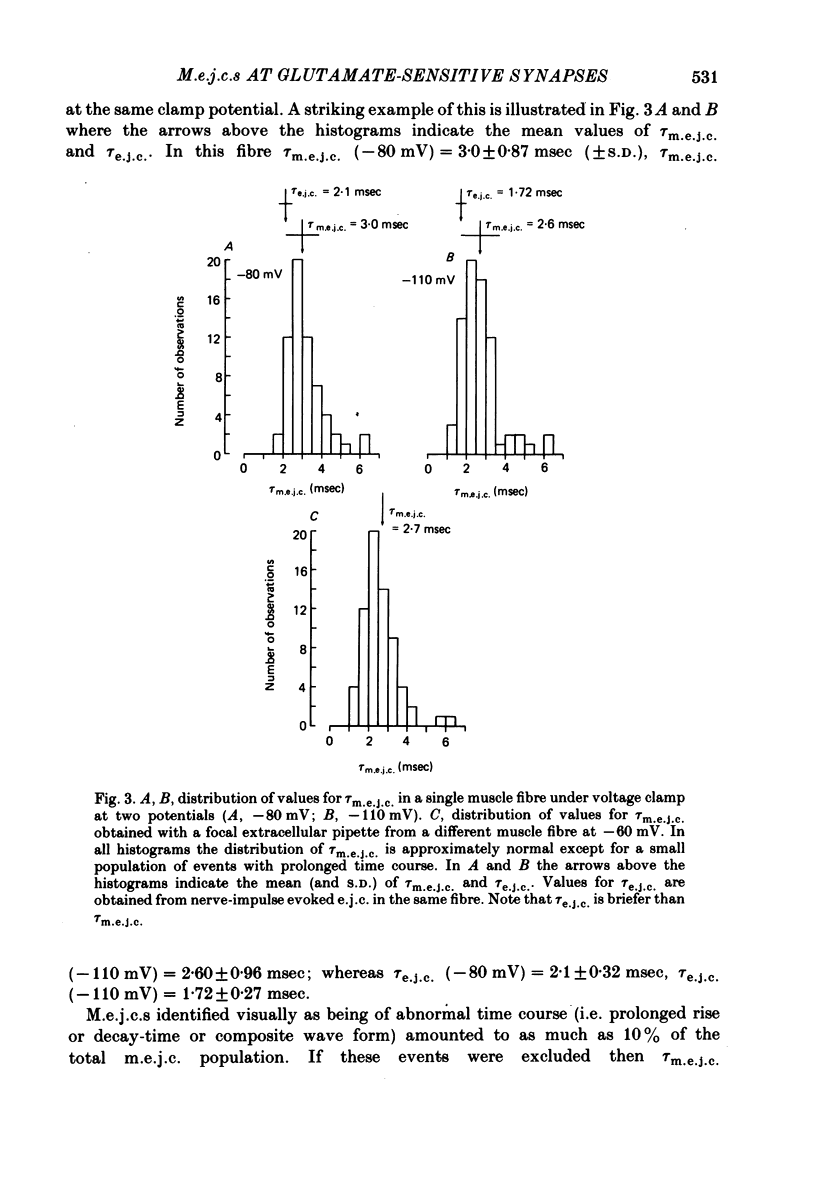
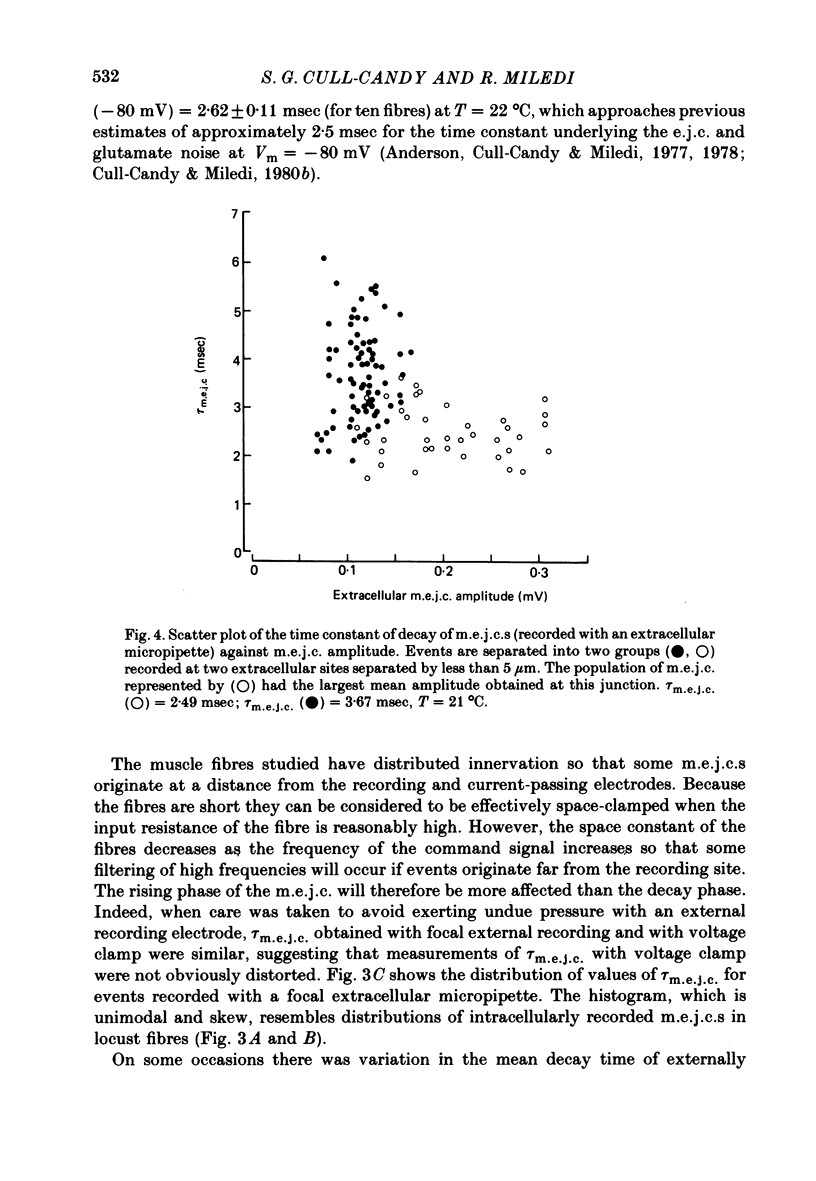
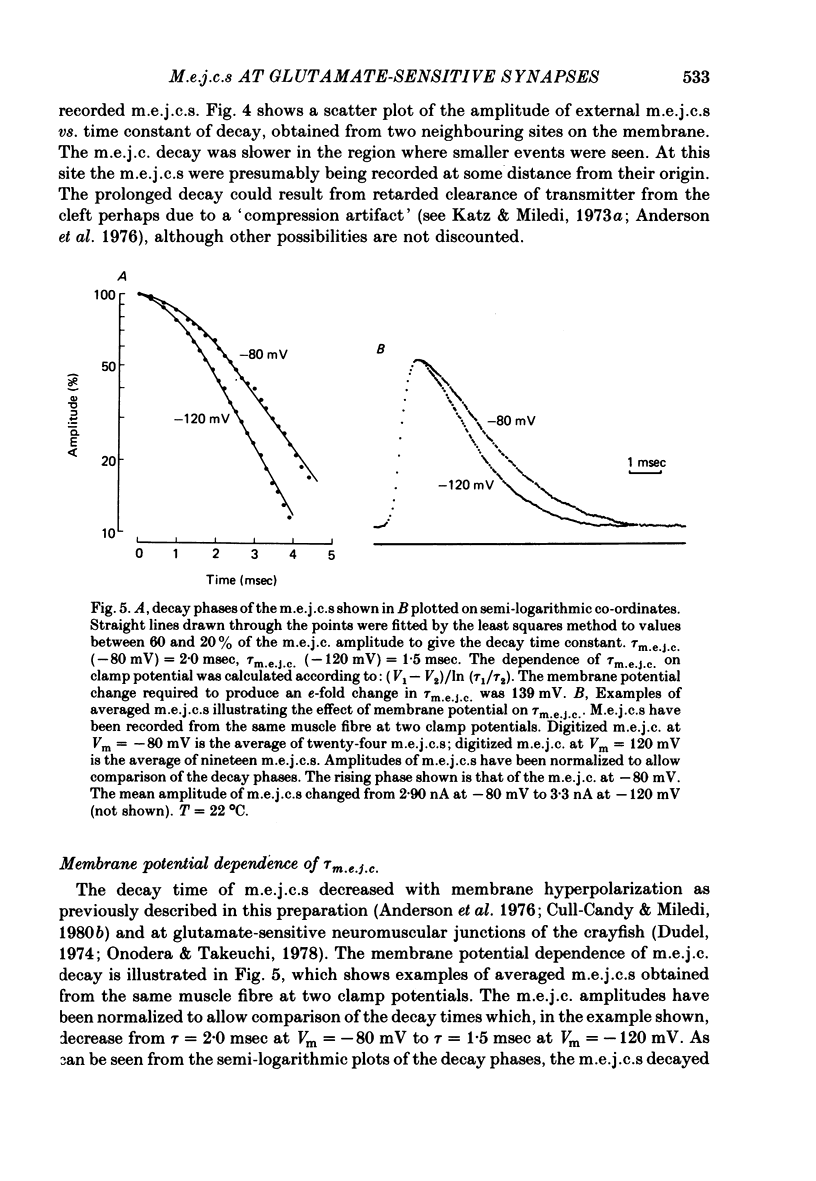
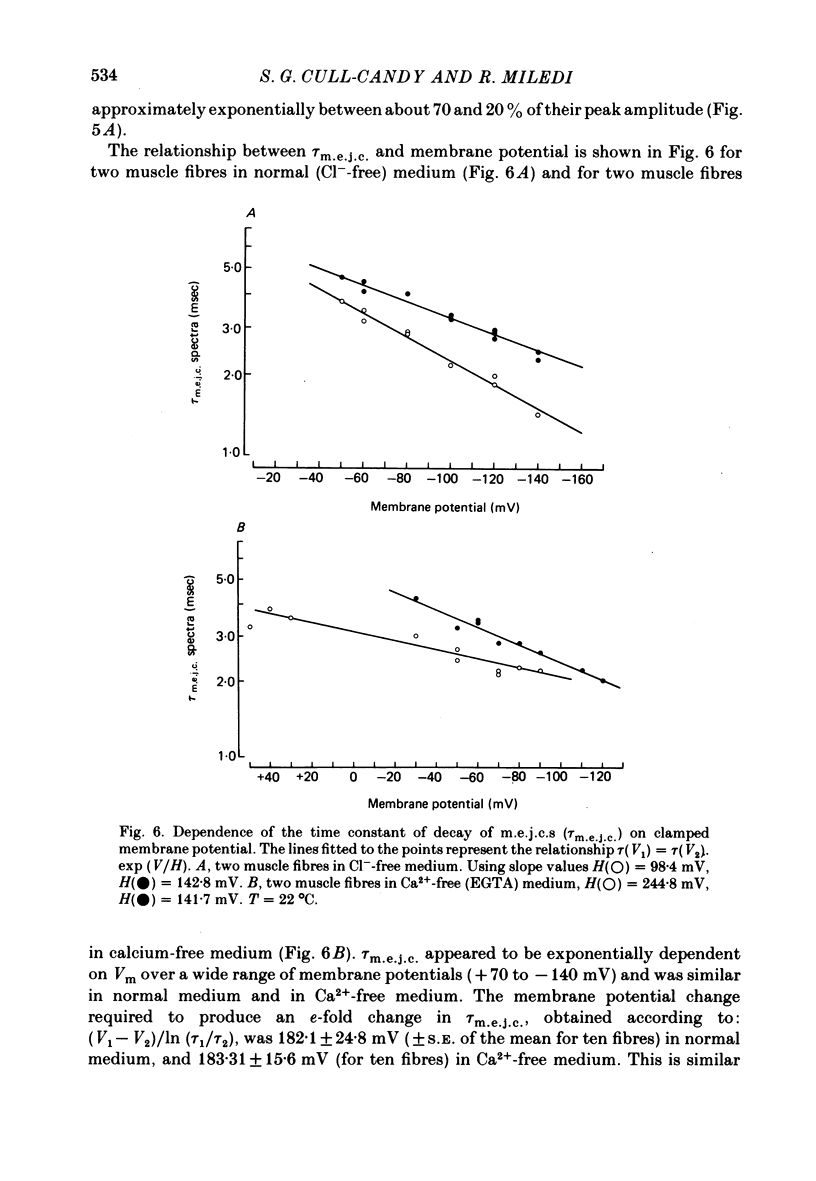
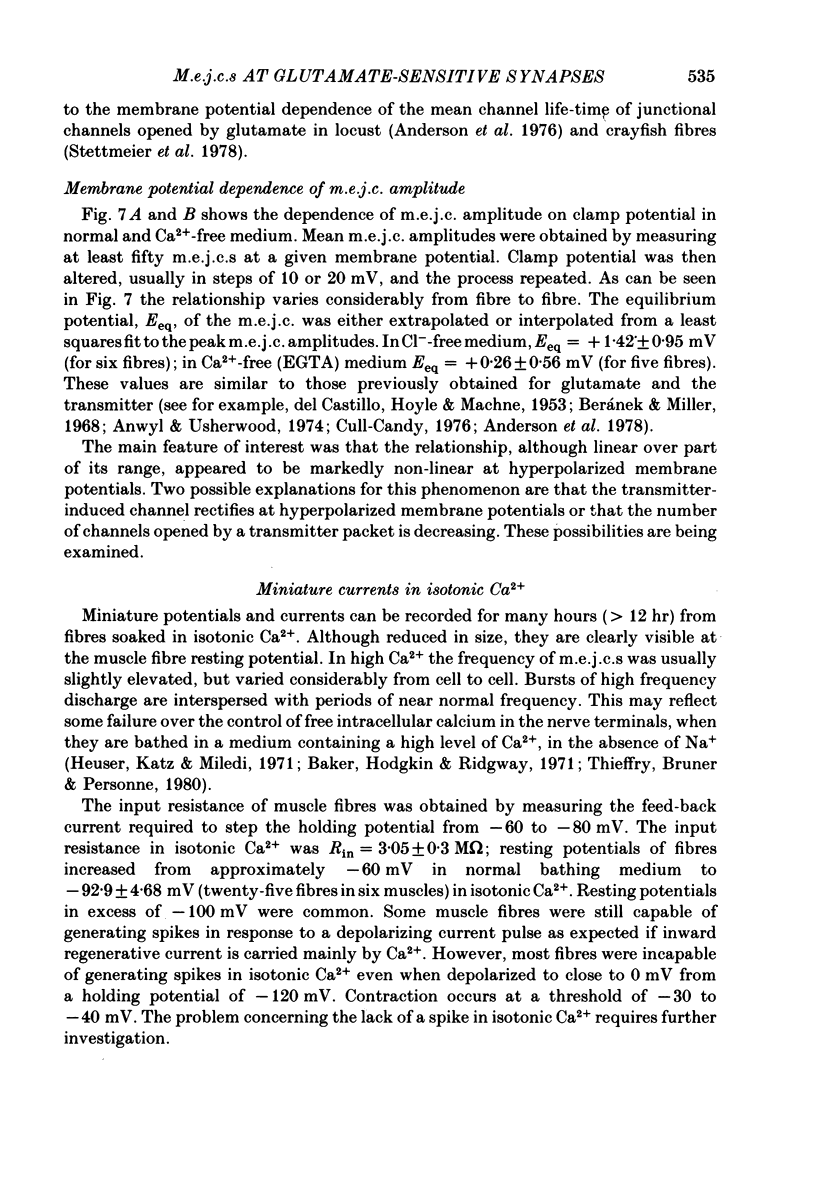
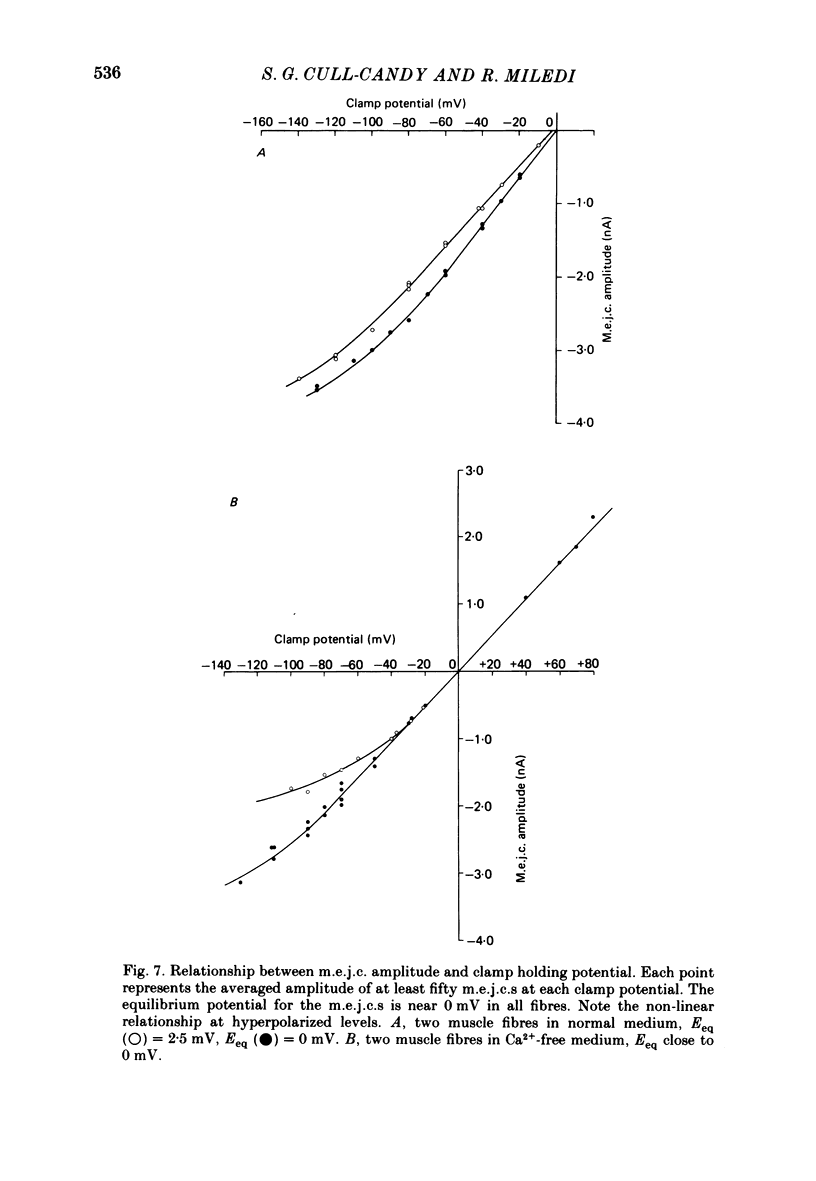
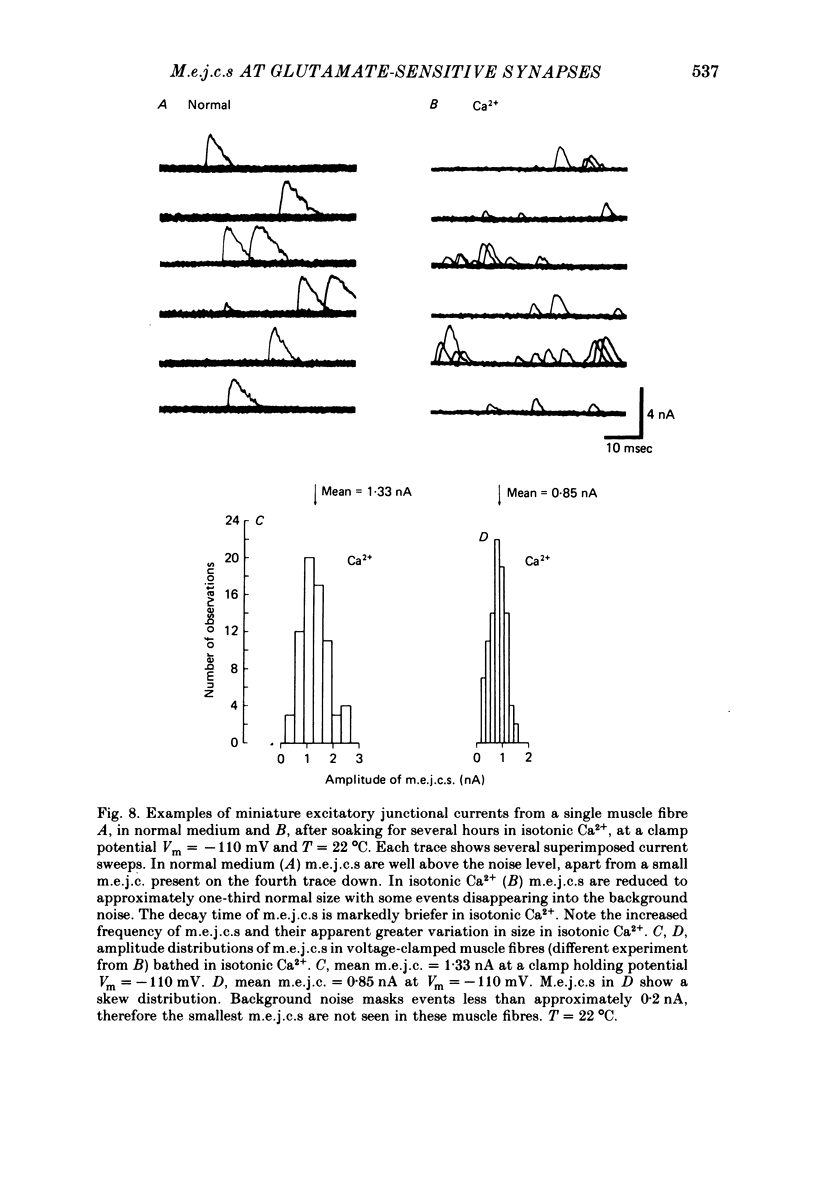
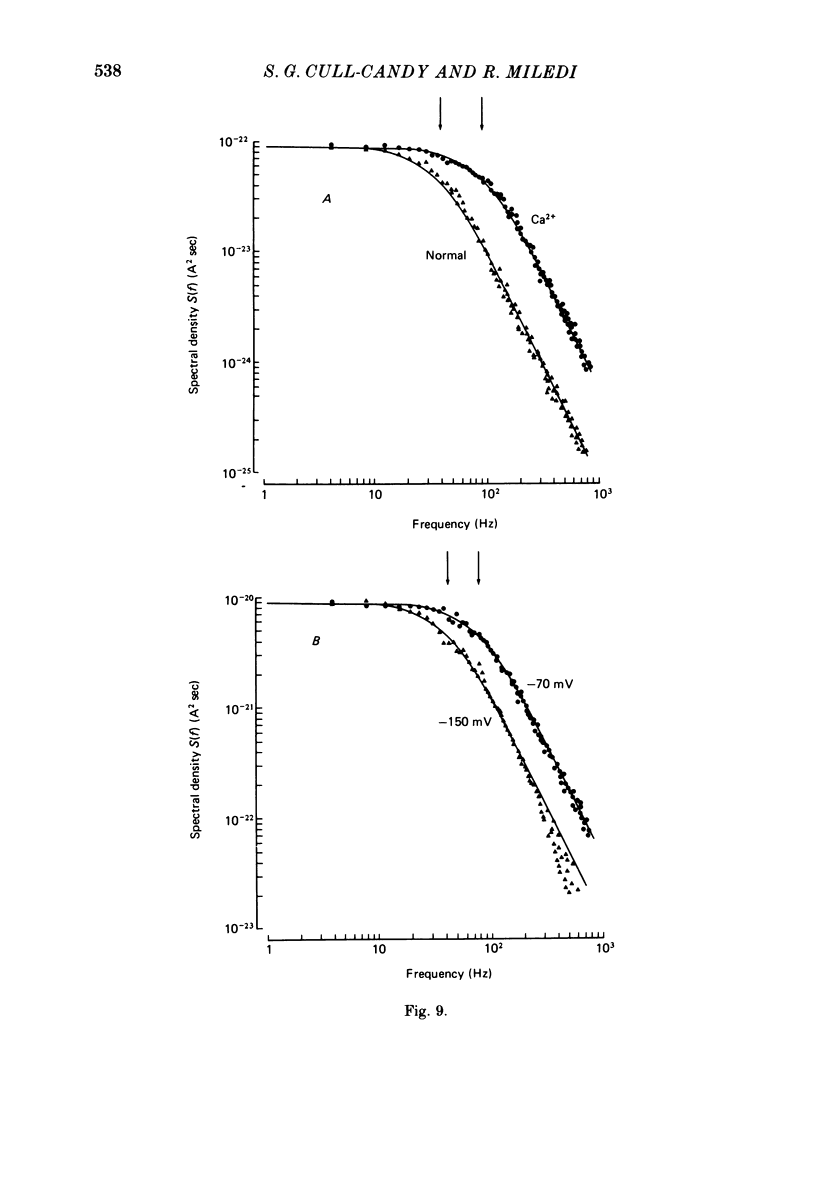
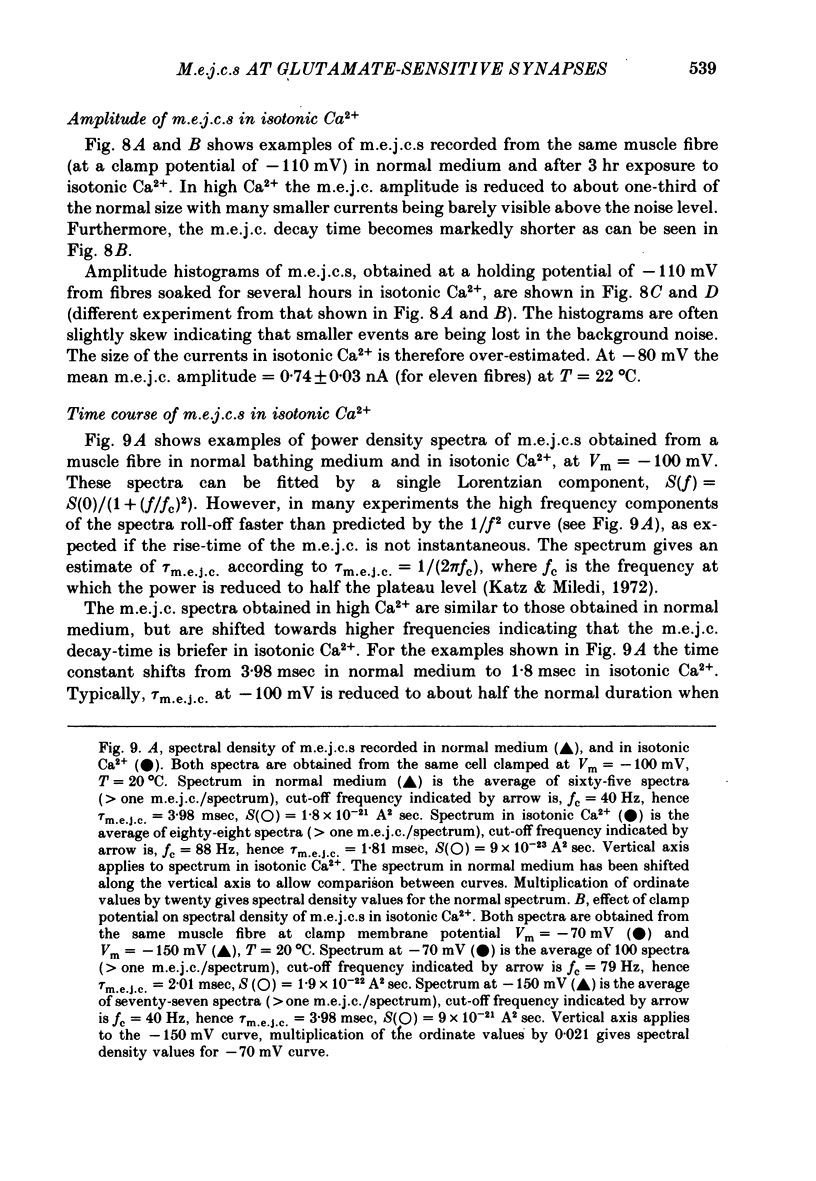
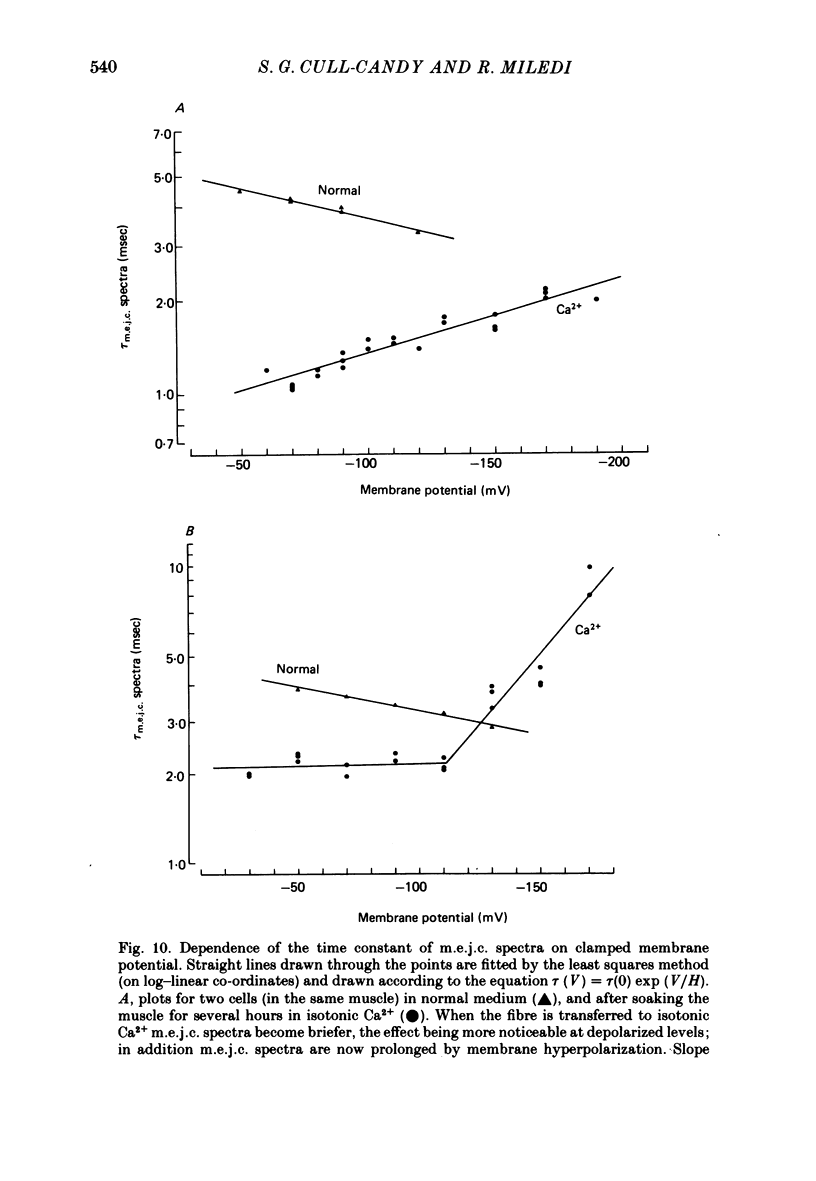
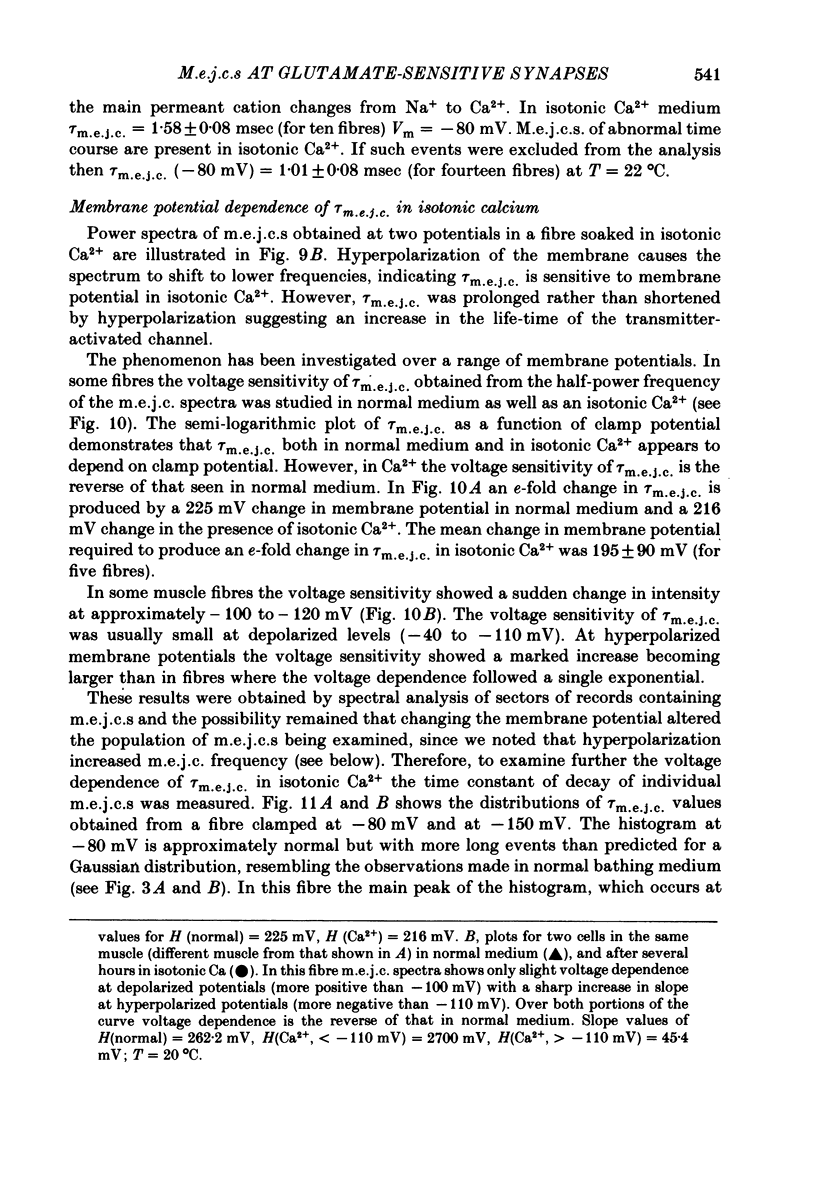
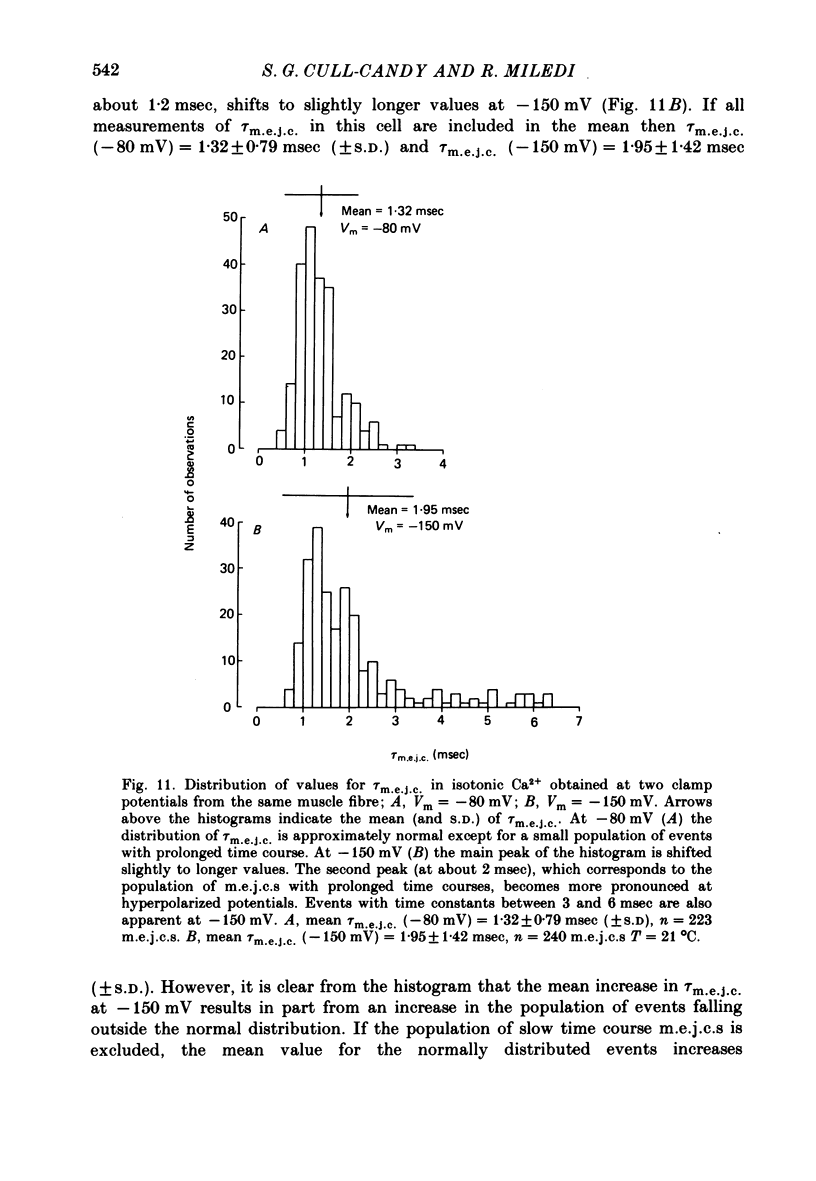
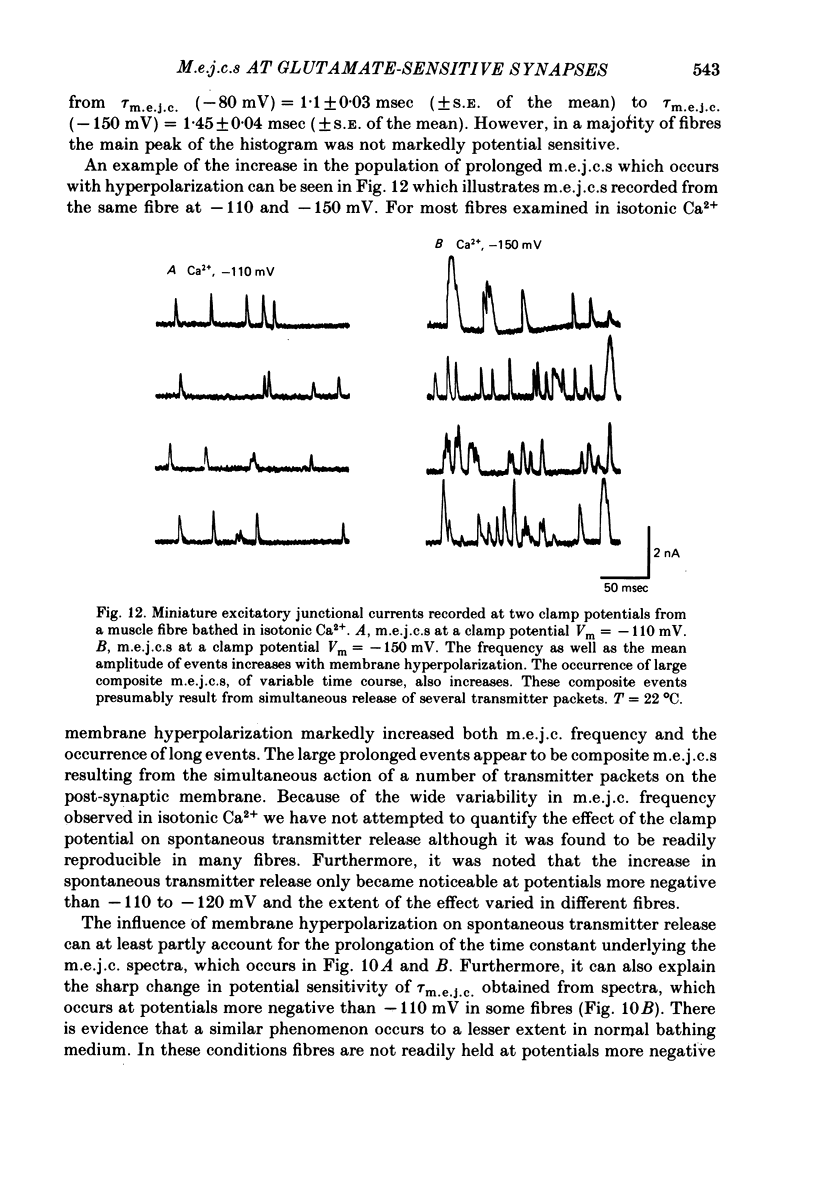
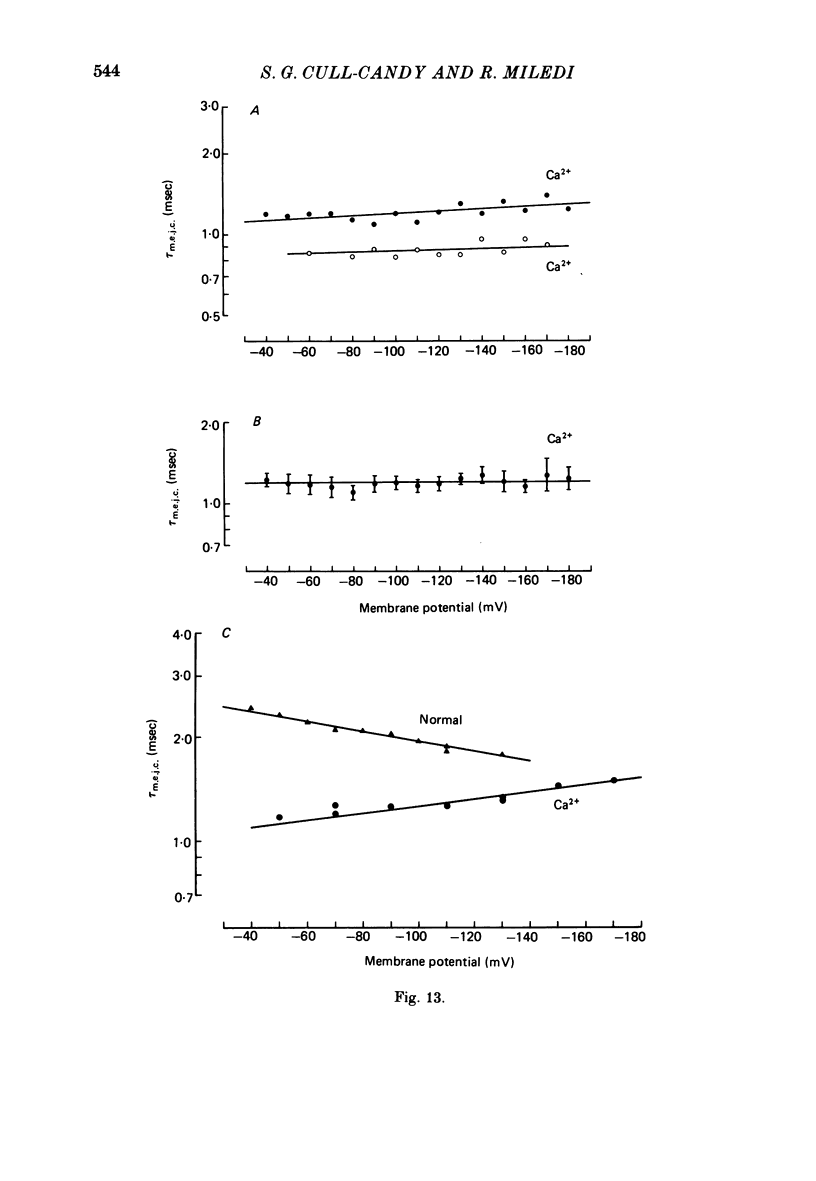
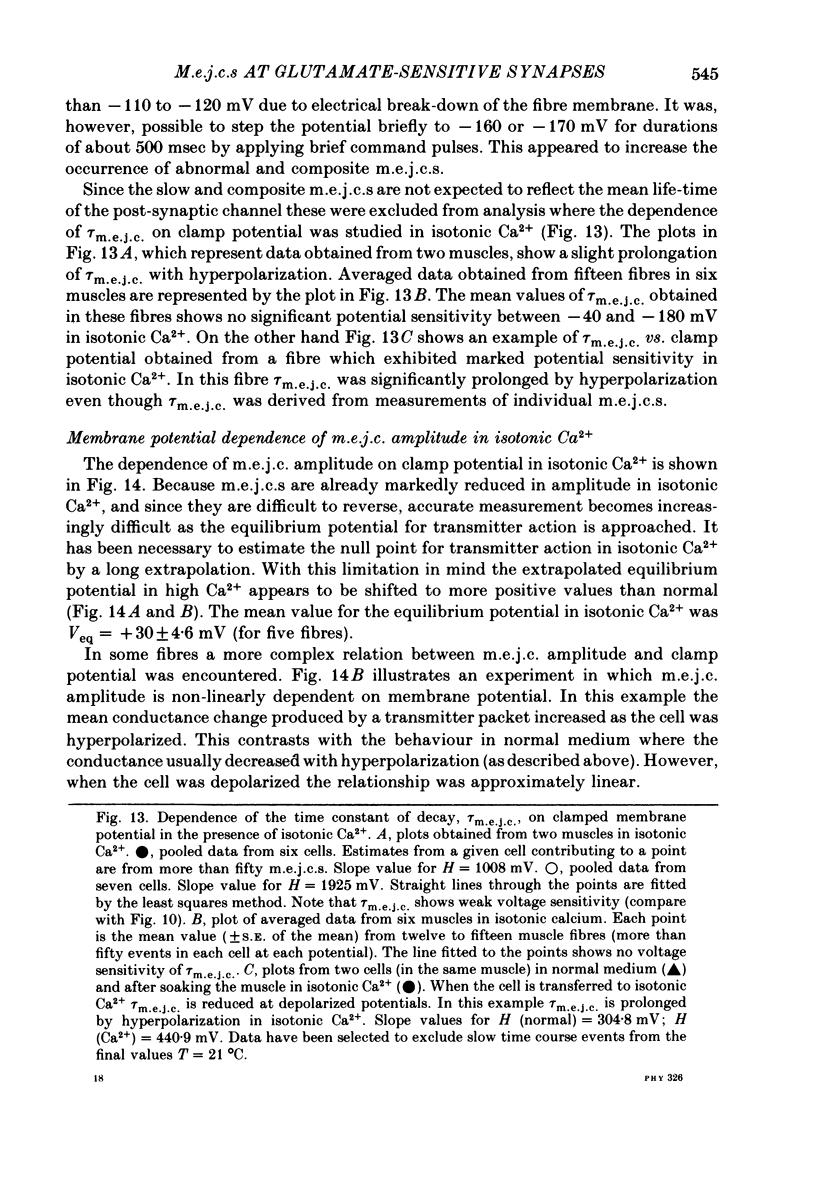
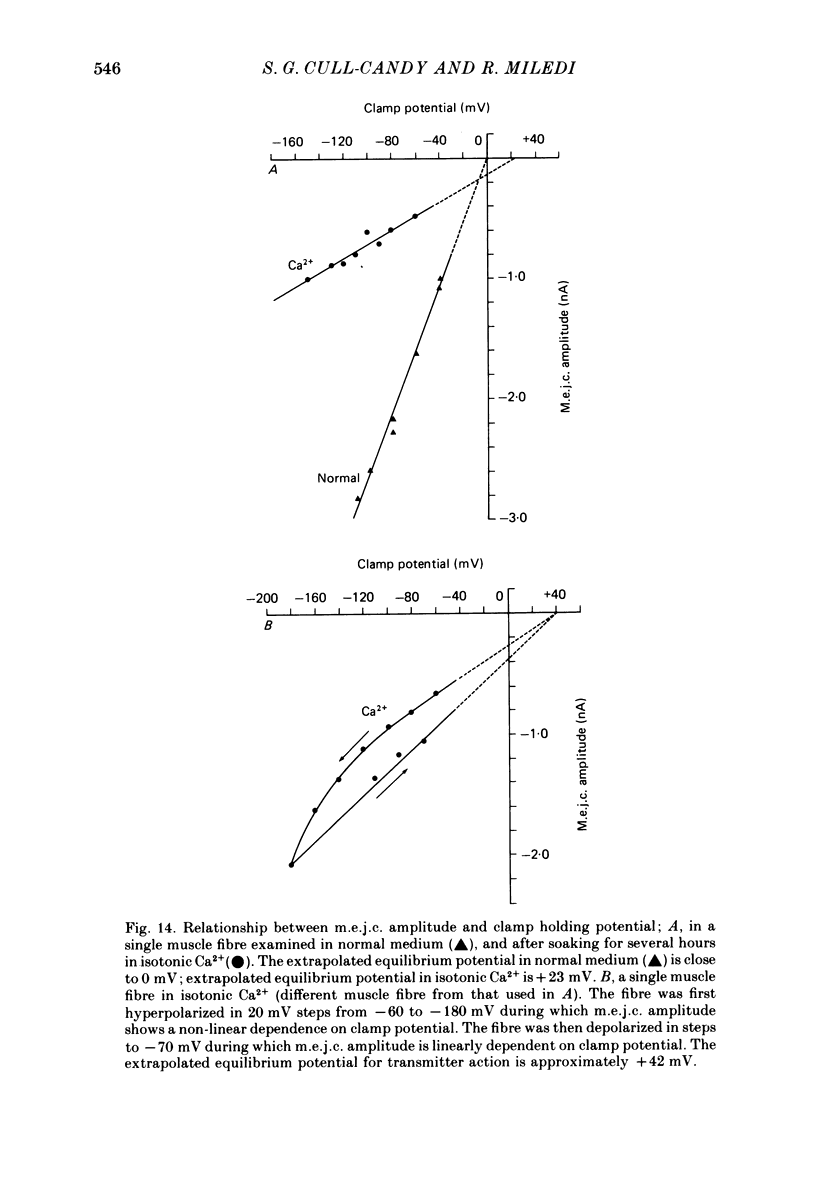
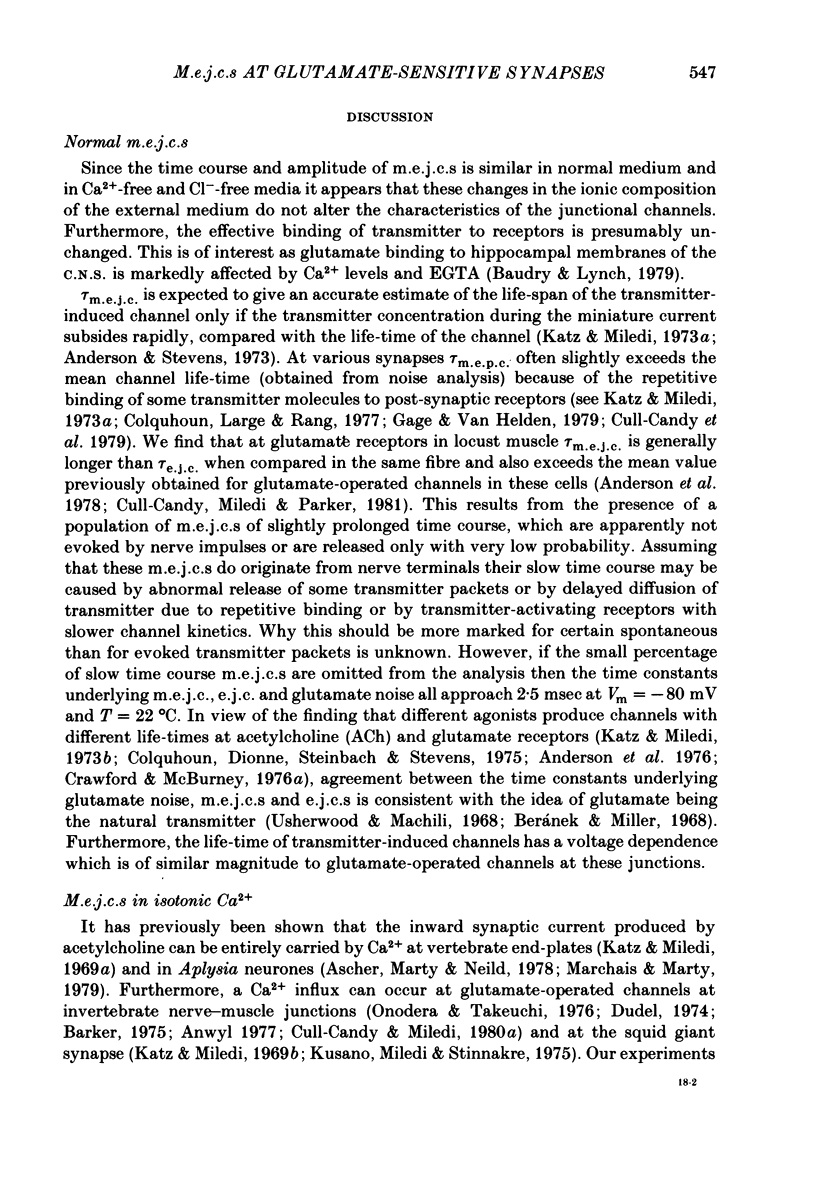
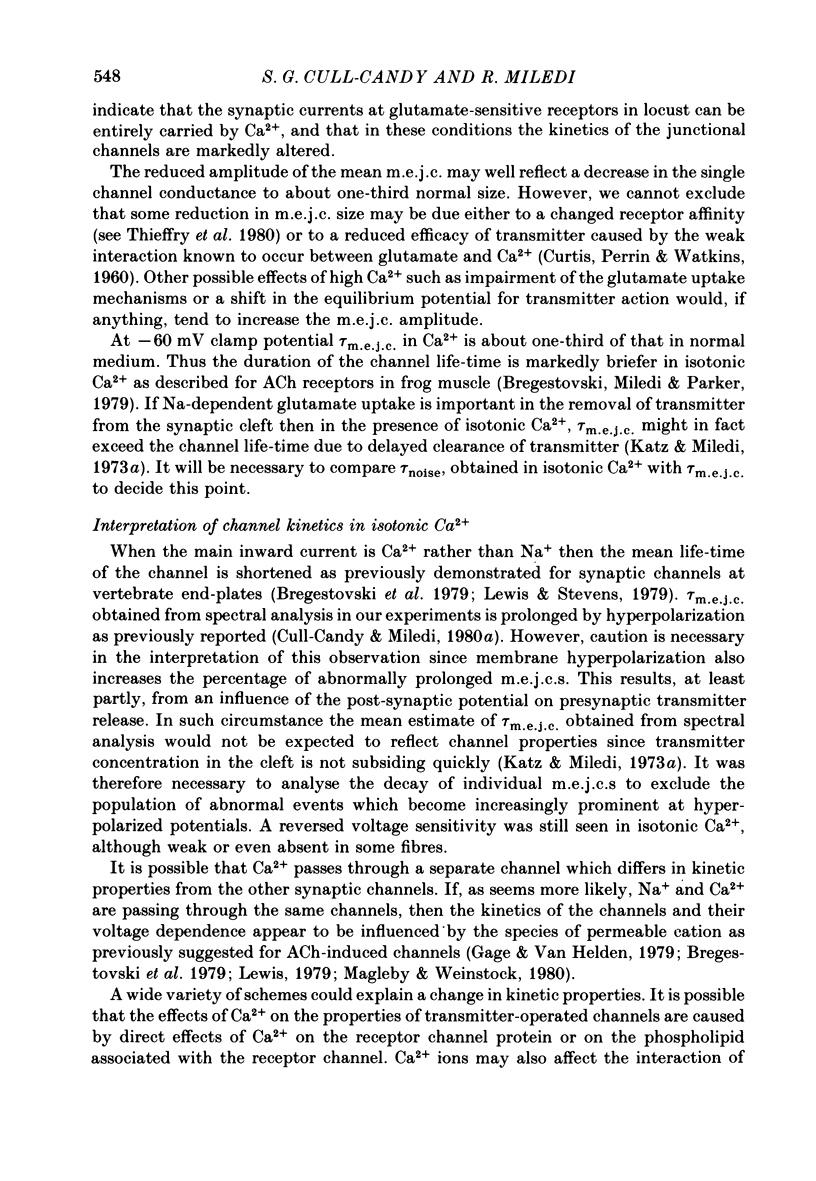
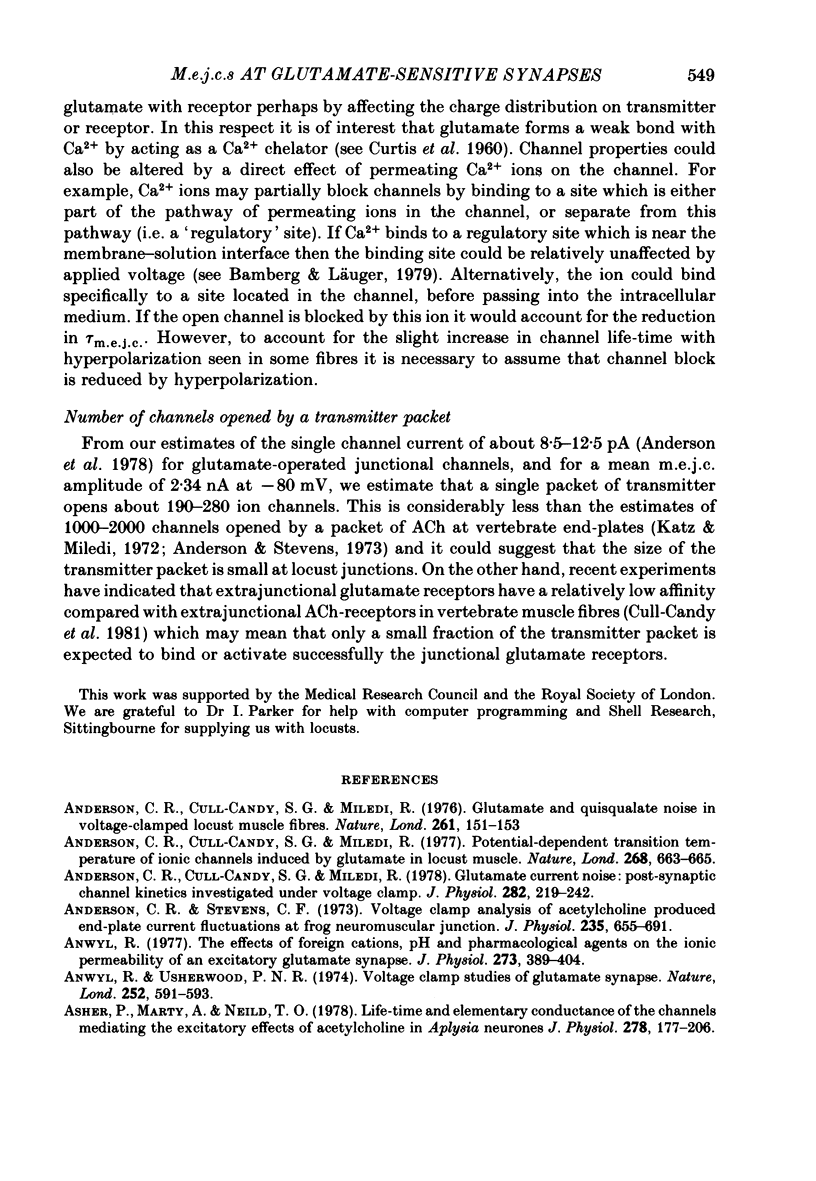
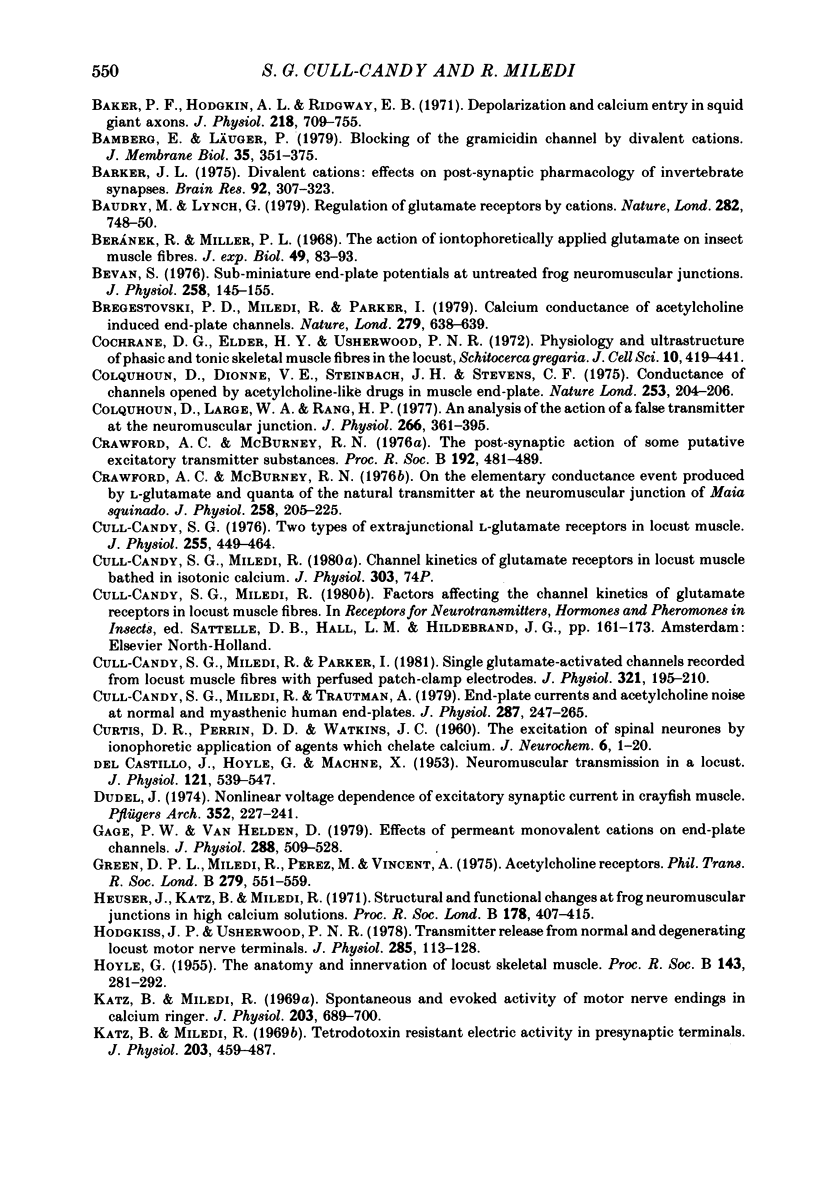
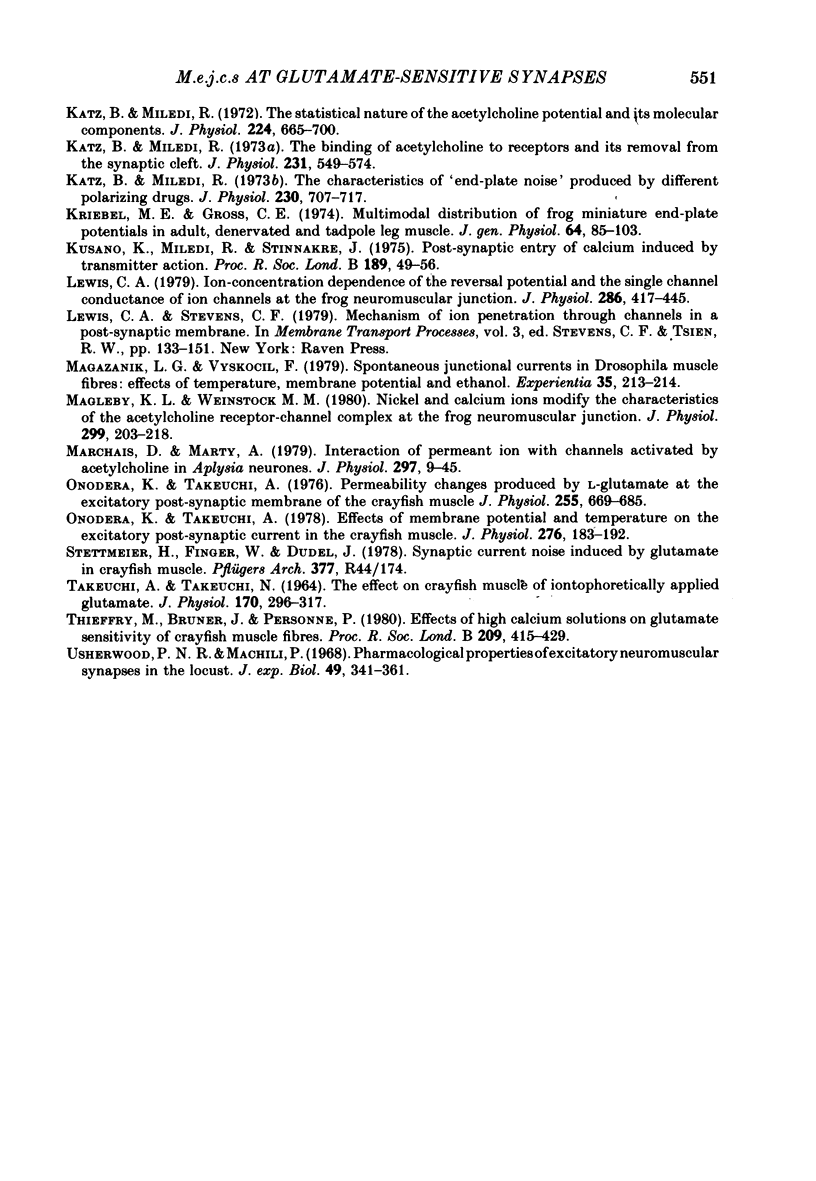
Selected References
These references are in PubMed. This may not be the complete list of references from this article.
- Anderson C. R., Cull-Candy S. G., Miledi R. Glutamate and quisqualate noise in voltage-clamped locust muscle fibres. Nature. 1976 May 13;261(5556):151–153. doi: 10.1038/261151a0. [DOI] [PubMed] [Google Scholar]
- Anderson C. R., Cull-Candy S. G., Miledi R. Glutamate current noise: post-synaptic channel kinetics investigated under voltage clamp. J Physiol. 1978 Sep;282:219–242. doi: 10.1113/jphysiol.1978.sp012459. [DOI] [PMC free article] [PubMed] [Google Scholar]
- Anderson C. R., Cull-Candy S. G., Miledi R. Potential-dependent transition temperature of ionic channels induced by glutamate in locust muscle. Nature. 1977 Aug 18;268(5621):663–665. doi: 10.1038/268663a0. [DOI] [PubMed] [Google Scholar]
- Anderson C. R., Stevens C. F. Voltage clamp analysis of acetylcholine produced end-plate current fluctuations at frog neuromuscular junction. J Physiol. 1973 Dec;235(3):655–691. doi: 10.1113/jphysiol.1973.sp010410. [DOI] [PMC free article] [PubMed] [Google Scholar]
- Anwyl R. The effect of foreign cations, pH and pharmacological agents on the ionic permeability of an excitatory glutamate synapse. J Physiol. 1977 Dec;273(2):389–404. doi: 10.1113/jphysiol.1977.sp012100. [DOI] [PMC free article] [PubMed] [Google Scholar]
- Anwyl R., Usherwood P. N. Voltage clamp studies of glutamate synapse. Nature. 1974 Dec 13;252(5484):591–593. doi: 10.1038/252591a0. [DOI] [PubMed] [Google Scholar]
- Ascher P., Marty A., Neild T. O. Life time and elementary conductance of the channels mediating the excitatory effects of acetylcholine in Aplysia neurones. J Physiol. 1978 May;278:177–206. doi: 10.1113/jphysiol.1978.sp012299. [DOI] [PMC free article] [PubMed] [Google Scholar]
- Baker P. F., Hodgkin A. L., Ridgway E. B. Depolarization and calcium entry in squid giant axons. J Physiol. 1971 Nov;218(3):709–755. doi: 10.1113/jphysiol.1971.sp009641. [DOI] [PMC free article] [PubMed] [Google Scholar]
- Barker J. L. Divalent cations: effects on post-synaptic pharmacology of invertebrate synapses. Brain Res. 1975 Jul 11;92(2):307–323. doi: 10.1016/0006-8993(75)90277-2. [DOI] [PubMed] [Google Scholar]
- Baudry M., Lynch G. Regulation of glutamate receptors by cations. Nature. 1979 Dec 13;282(5740):748–750. doi: 10.1038/282748a0. [DOI] [PubMed] [Google Scholar]
- Bevan S. Sub-miniature end-plate potentials at untreated frog neuromuscular junctions. J Physiol. 1976 Jun;258(1):145–155. doi: 10.1113/jphysiol.1976.sp011411. [DOI] [PMC free article] [PubMed] [Google Scholar]
- Bregestovski P. D., Miledi R., Parker I. Calcium conductance of acetylcholine-induced endplate channels. Nature. 1979 Jun 14;279(5714):638–639. doi: 10.1038/279638a0. [DOI] [PubMed] [Google Scholar]
- CURTIS D. R., PERRIN D. D., WATKINS J. C. The excitation of spinal neurones by the ionophoretic application of agents which chelate calcium. J Neurochem. 1960 Aug;6:1–20. doi: 10.1111/j.1471-4159.1960.tb13443.x. [DOI] [PubMed] [Google Scholar]
- Cochrane D. G., Elder H. Y., Usherwood P. N. Physiology and ultrastructure of phasic and tonic skeletal muscle fibres in the locust, Schistocerca gregaria. J Cell Sci. 1972 Mar;10(2):419–441. doi: 10.1242/jcs.10.2.419. [DOI] [PubMed] [Google Scholar]
- Colquhoun D., Dionne V. E., Steinbach J. H., Stevens C. F. Conductance of channels opened by acetylcholine-like drugs in muscle end-plate. Nature. 1975 Jan 17;253(5488):204–206. doi: 10.1038/253204a0. [DOI] [PubMed] [Google Scholar]
- Crawford A. C., McBurney R. N. On the elementary conductance event produced by L-glutamate and quanta of the natural transmitter at the neuromuscular junctions of Maia squinado. J Physiol. 1976 Jun;258(1):205–225. doi: 10.1113/jphysiol.1976.sp011415. [DOI] [PMC free article] [PubMed] [Google Scholar]
- Crawford A. C., McBurney R. N. The post-synaptic action of some putative excitatory transmitter substances. Proc R Soc Lond B Biol Sci. 1976 Mar 16;192(1109):481–489. doi: 10.1098/rspb.1976.0026. [DOI] [PubMed] [Google Scholar]
- Cull-Candy S. G., Miledi R., Parker I. Single glutamate-activated channels recorded from locust muscle fibres with perfused patch-clamp electrodes. J Physiol. 1981 Dec;321:195–210. doi: 10.1113/jphysiol.1981.sp013979. [DOI] [PMC free article] [PubMed] [Google Scholar]
- Cull-Candy S. G., Miledi R., Trautmann A. End-plate currents and acetylcholine noise at normal and myasthenic human end-plates. J Physiol. 1979 Feb;287:247–265. doi: 10.1113/jphysiol.1979.sp012657. [DOI] [PMC free article] [PubMed] [Google Scholar]
- Cull-Candy S. G. Two types of extrajunctional L-glutamate receptors in locust muscle fibres. J Physiol. 1976 Feb;255(2):449–464. doi: 10.1113/jphysiol.1976.sp011289. [DOI] [PMC free article] [PubMed] [Google Scholar]
- DEL CASTILLO J., HOYLE G., MACHNE X. Neuromuscular transmission in a locust. J Physiol. 1953 Sep;121(3):539–547. doi: 10.1113/jphysiol.1953.sp004963. [DOI] [PMC free article] [PubMed] [Google Scholar]
- Dudel J. Nonlinear voltage dependence of excitatory synaptic current in crayfish muscle. Pflugers Arch. 1974;352(3):227–241. doi: 10.1007/BF00590488. [DOI] [PubMed] [Google Scholar]
- Gage P. W., Van Helden D. Effects of permeant monovalent cations on end-plate channels. J Physiol. 1979 Mar;288:509–528. [PMC free article] [PubMed] [Google Scholar]
- Green D. P., Miledi R., Perez de la Mora M., Vincent A. Acetylcholine receptors. Philos Trans R Soc Lond B Biol Sci. 1975 Jun 10;270(908):551–559. doi: 10.1098/rstb.1975.0031. [DOI] [PubMed] [Google Scholar]
- HOYLE G. The anatomy and innervation of locust skeletal muscle. Proc R Soc Lond B Biol Sci. 1955 Jan 27;143(911):281–292. doi: 10.1098/rspb.1955.0011. [DOI] [PubMed] [Google Scholar]
- Hodgkiss J. P., Usherwood P. N. Transmitter release from normal and degenerating locust motor nerve terminals. J Physiol. 1978 Dec;285:113–128. doi: 10.1113/jphysiol.1978.sp012561. [DOI] [PMC free article] [PubMed] [Google Scholar]
- Katz B., Miledi R. Spontaneous and evoked activity of motor nerve endings in calcium Ringer. J Physiol. 1969 Aug;203(3):689–706. doi: 10.1113/jphysiol.1969.sp008887. [DOI] [PMC free article] [PubMed] [Google Scholar]
- Katz B., Miledi R. Tetrodotoxin-resistant electric activity in presynaptic terminals. J Physiol. 1969 Aug;203(2):459–487. doi: 10.1113/jphysiol.1969.sp008875. [DOI] [PMC free article] [PubMed] [Google Scholar]
- Katz B., Miledi R. The binding of acetylcholine to receptors and its removal from the synaptic cleft. J Physiol. 1973 Jun;231(3):549–574. doi: 10.1113/jphysiol.1973.sp010248. [DOI] [PMC free article] [PubMed] [Google Scholar]
- Katz B., Miledi R. The characteristics of 'end-plate noise' produced by different depolarizing drugs. J Physiol. 1973 May;230(3):707–717. doi: 10.1113/jphysiol.1973.sp010213. [DOI] [PMC free article] [PubMed] [Google Scholar]
- Katz B., Miledi R. The statistical nature of the acetycholine potential and its molecular components. J Physiol. 1972 Aug;224(3):665–699. doi: 10.1113/jphysiol.1972.sp009918. [DOI] [PMC free article] [PubMed] [Google Scholar]
- Kriebel M. E., Gross C. E. Multimodal distribution of frog miniature endplate potentials in adult denervated and tadpole leg muscle. J Gen Physiol. 1974 Jul;64(1):85–103. doi: 10.1085/jgp.64.1.85. [DOI] [PMC free article] [PubMed] [Google Scholar]
- Kusano K., Miledi R., Stinnakre J. Postsynaptic entry of calcium induced by transmitter action. Proc R Soc Lond B Biol Sci. 1975 Apr 29;189(1094):49–56. doi: 10.1098/rspb.1975.0040. [DOI] [PubMed] [Google Scholar]
- Lewis C. A. Ion-concentration dependence of the reversal potential and the single channel conductance of ion channels at the frog neuromuscular junction. J Physiol. 1979 Jan;286:417–445. doi: 10.1113/jphysiol.1979.sp012629. [DOI] [PMC free article] [PubMed] [Google Scholar]
- Magazanik L. G., Vyskocil F. Spontaneous junctional currents in Drosophila muscle fibres: effects of temperature, membrane potential and ethanol. Experientia. 1979 Feb 15;35(2):213–214. doi: 10.1007/BF01920623. [DOI] [PubMed] [Google Scholar]
- Magleby K. L., Weinstock M. M. Nickel and calcium ions modify the characteristics of the acetylcholine receptor-channel complex at the frog neuromuscular junction. J Physiol. 1980 Feb;299:203–218. doi: 10.1113/jphysiol.1980.sp013120. [DOI] [PMC free article] [PubMed] [Google Scholar]
- Marchais D., Marty A. Interaction of permeant ions with channels activated by acetylcholine in Aplysia neurones. J Physiol. 1979 Dec;297(0):9–45. doi: 10.1113/jphysiol.1979.sp013025. [DOI] [PMC free article] [PubMed] [Google Scholar]
- Onodera K., Takeuchi A. Effects of membrane potential and temperature on the excitatory post-synaptic current in the crayfish muscle. J Physiol. 1978 Mar;276:183–192. doi: 10.1113/jphysiol.1978.sp012227. [DOI] [PMC free article] [PubMed] [Google Scholar]
- Onodera K., Takeuchi A. Permeability changes produced by L-glutamate at the excitatory post-synaptic membrane of the crayfish muscle. J Physiol. 1976 Mar;255(3):669–685. doi: 10.1113/jphysiol.1976.sp011302. [DOI] [PMC free article] [PubMed] [Google Scholar]
- TAKEUCHI A., TAKEUCHI N. THE EFFECT ON CRAYFISH MUSCLE OF IONTOPHORETICALLY APPLIED GLUTAMATE. J Physiol. 1964 Mar;170:296–317. doi: 10.1113/jphysiol.1964.sp007332. [DOI] [PMC free article] [PubMed] [Google Scholar]
- Thieffry M., Bruner J., Personne P. Effects of high calcium solutions on glutamate sensitivity of crayfish muscle fibres. Proc R Soc Lond B Biol Sci. 1980 Sep 26;209(1176):415–429. doi: 10.1098/rspb.1980.0103. [DOI] [PubMed] [Google Scholar]