Abstract
Ionic permeation in the selectivity filter of ion channels is analyzed by a microscopic model based on molecular kinetic theory. The energy and flux equations are derived by assuming that: (a) the selectivity filter is formed by a symmetrical array of carbonyl groups; (b) ion movement is near the axis of the channel; (c) a fraction of water molecules is separated from the ion while it moves across the selectivity filter; (d) the applied voltage drops linearly across the selectivity filter; (e) ions move independently. Energy profiles, single channel conductances, and the degree of hydration of K+ in a hypothetical K+ channel are examined by varying the following microscopic parameters: ion radius and mass, channel radius, number of effective water dipoles, and number of carbonyl groups. The i-V curve is linear up to +/- 170 mV. If the positions of energy maxima and minima are fixed, this linear range is reduced to +/- 50 mV. Channel radius and ion-water interactions are found to be two major channel structural determinants for selectivity sequences. Both radius and mass of an ion are important in selectivity mediated by these interactions. The theory predicts a total of 15 possible kinetic selectivity sequences for alkali cations in ion channels with a single selectivity filter.
Full text
PDF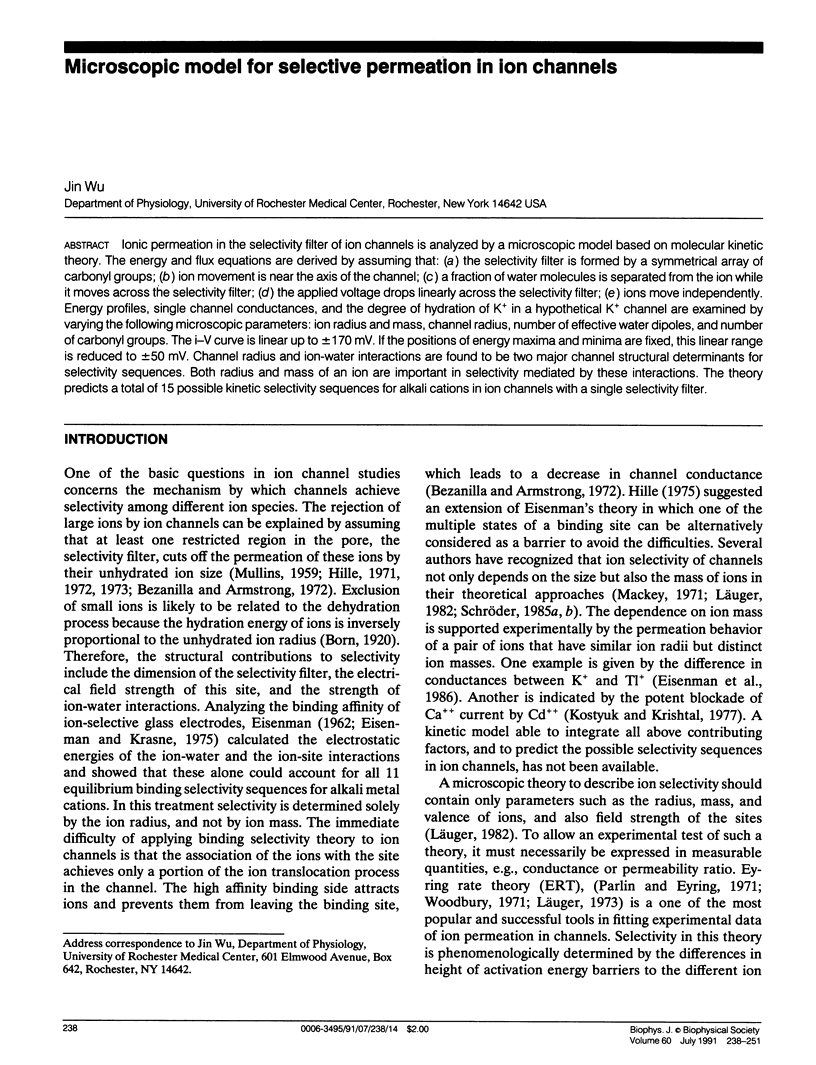
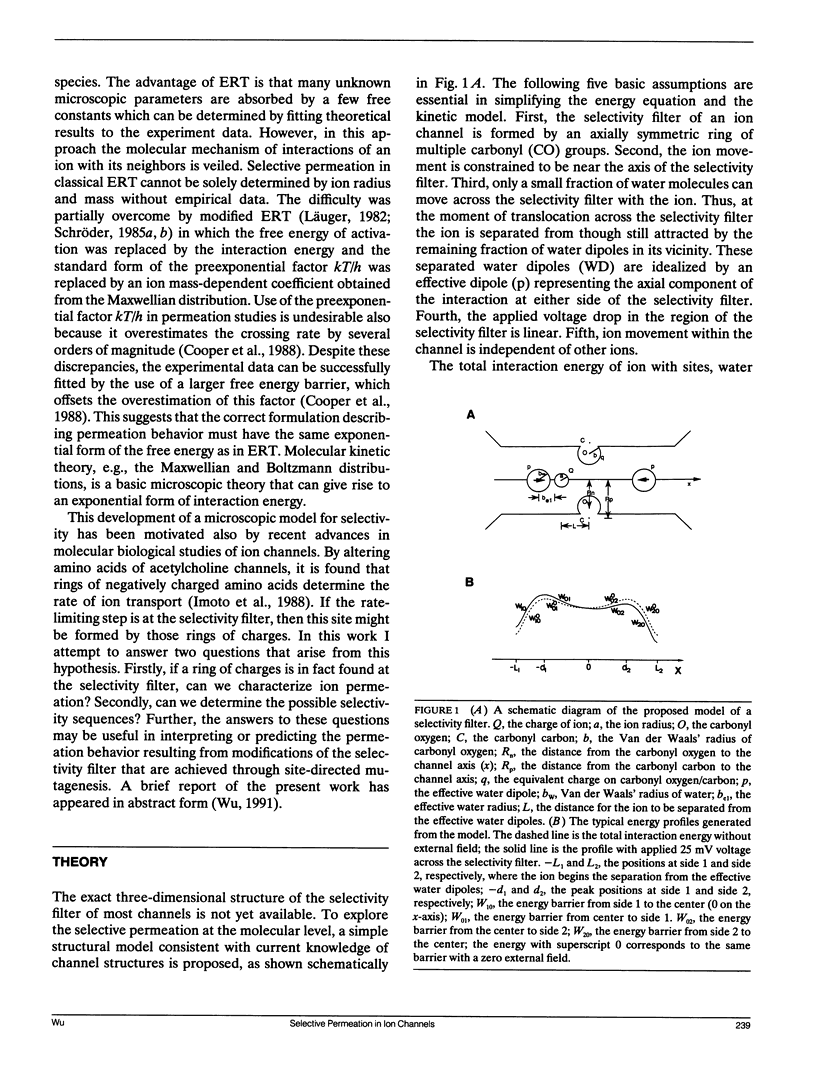
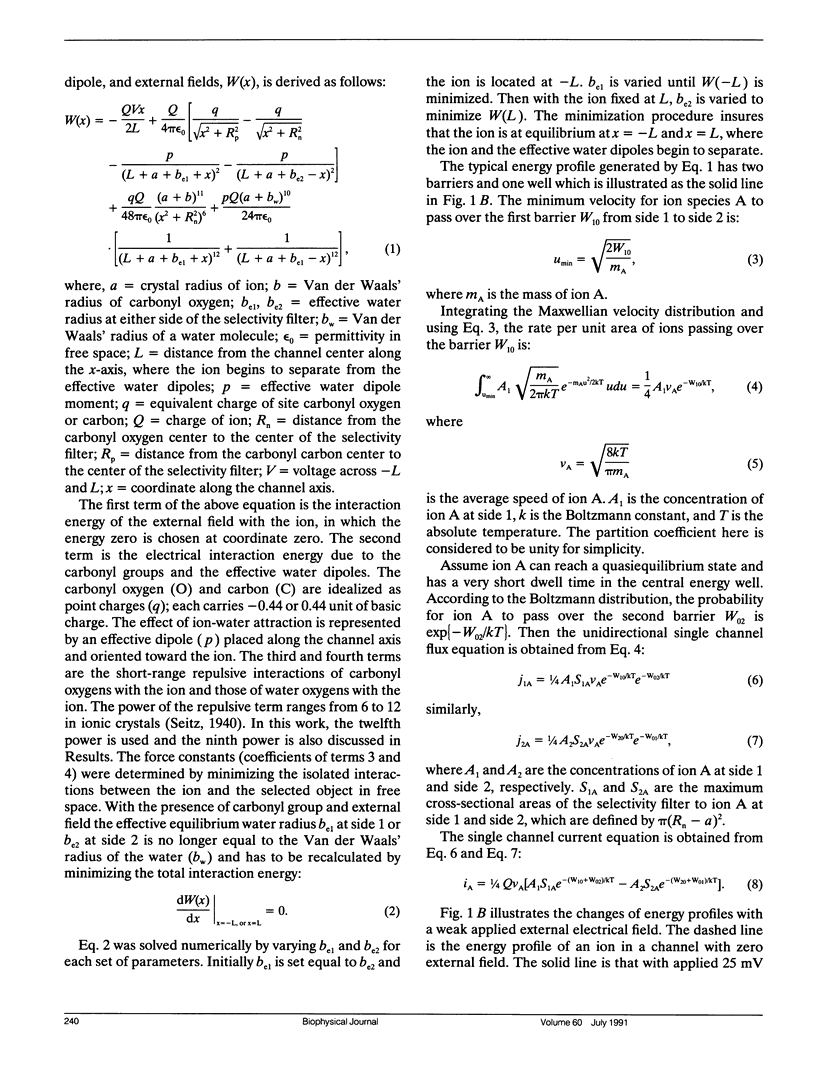
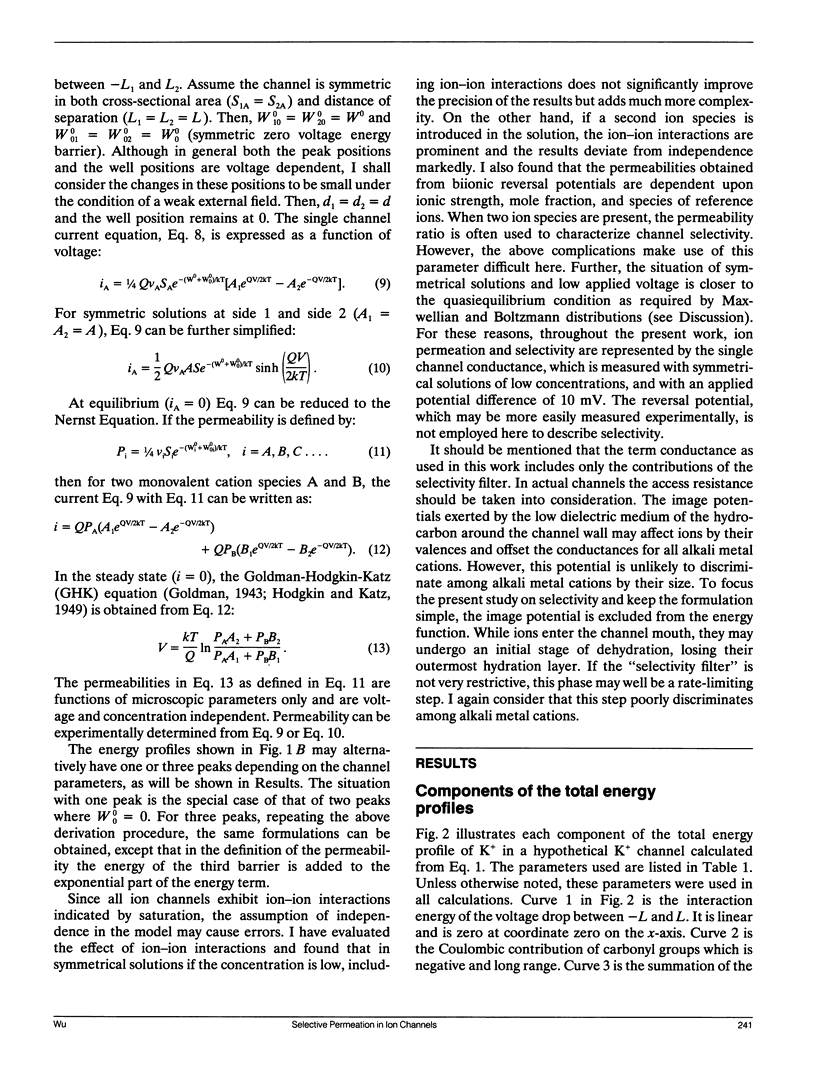
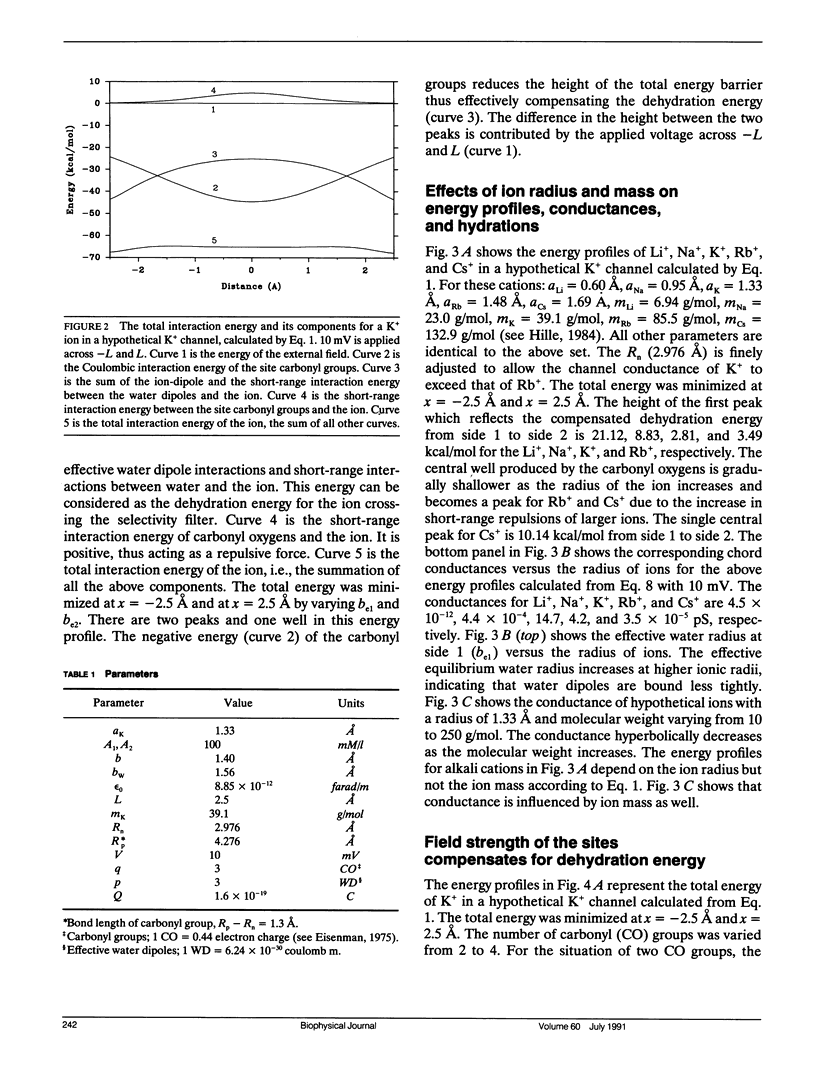
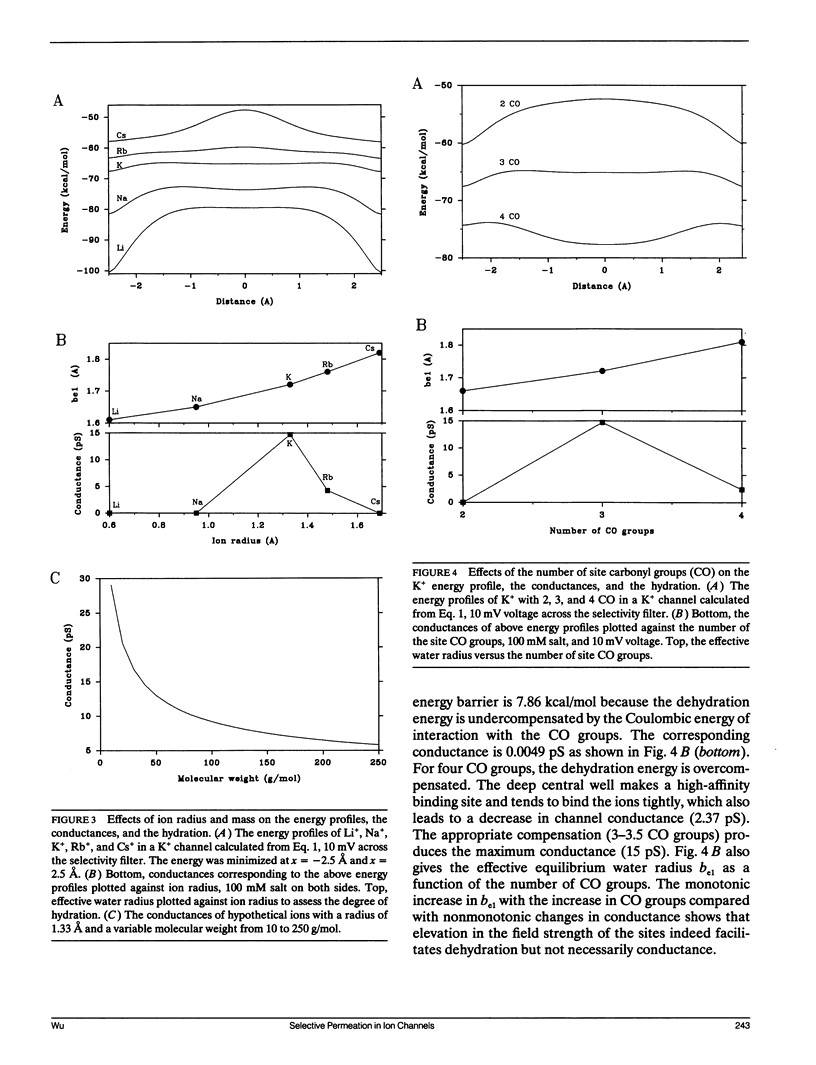
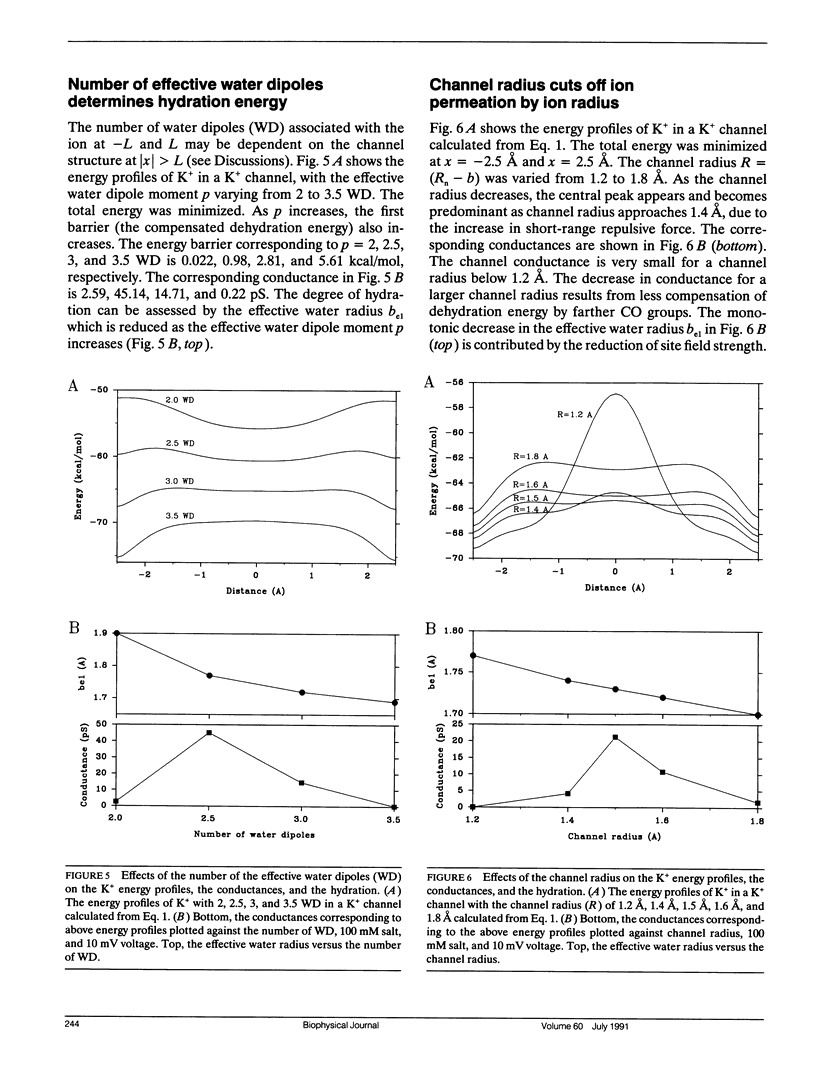
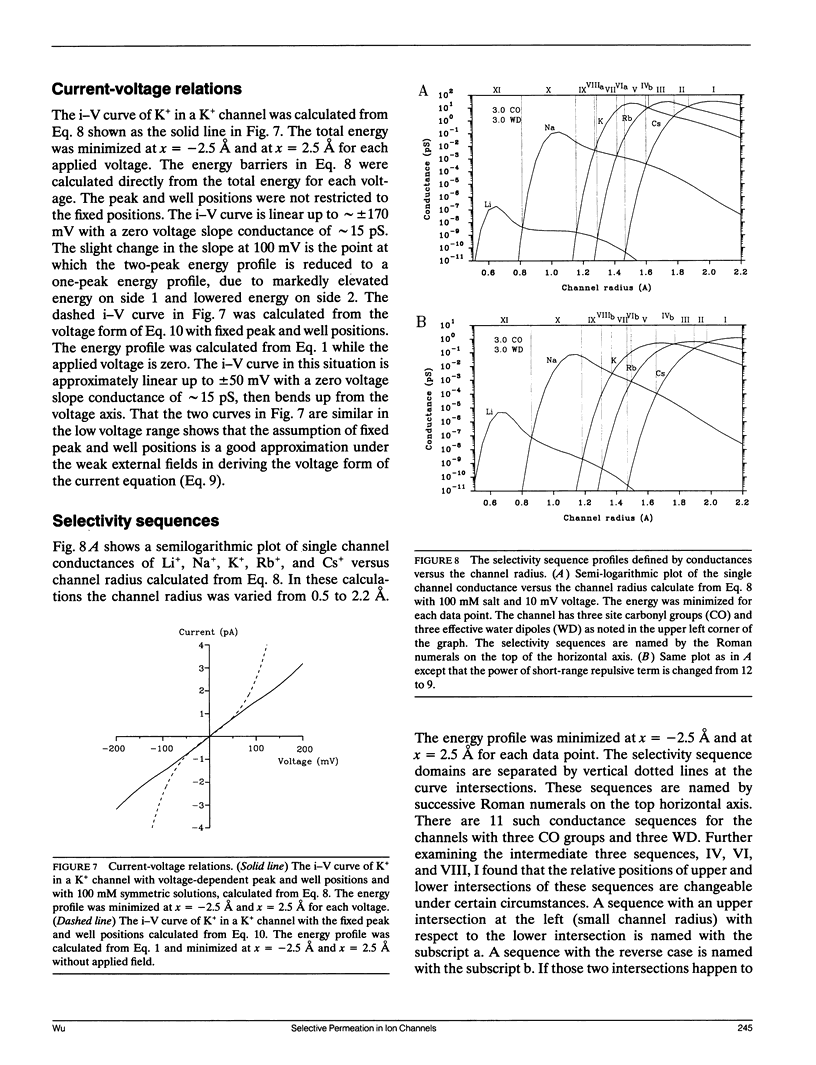
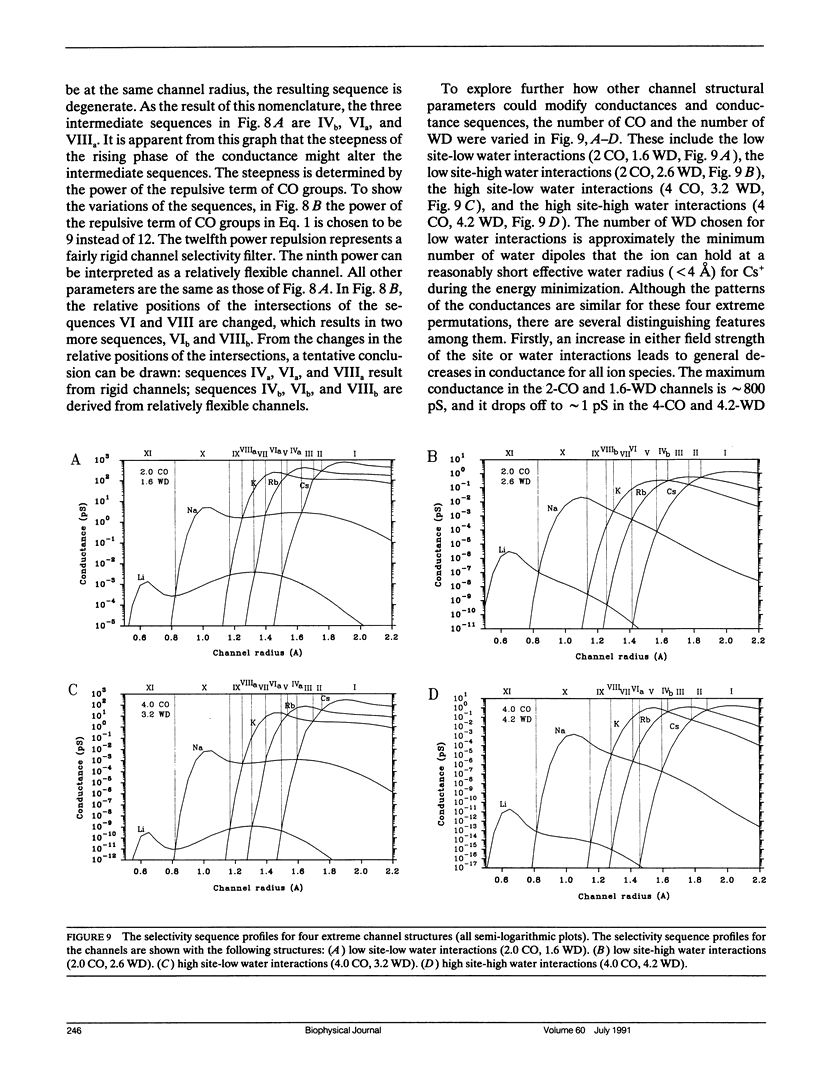
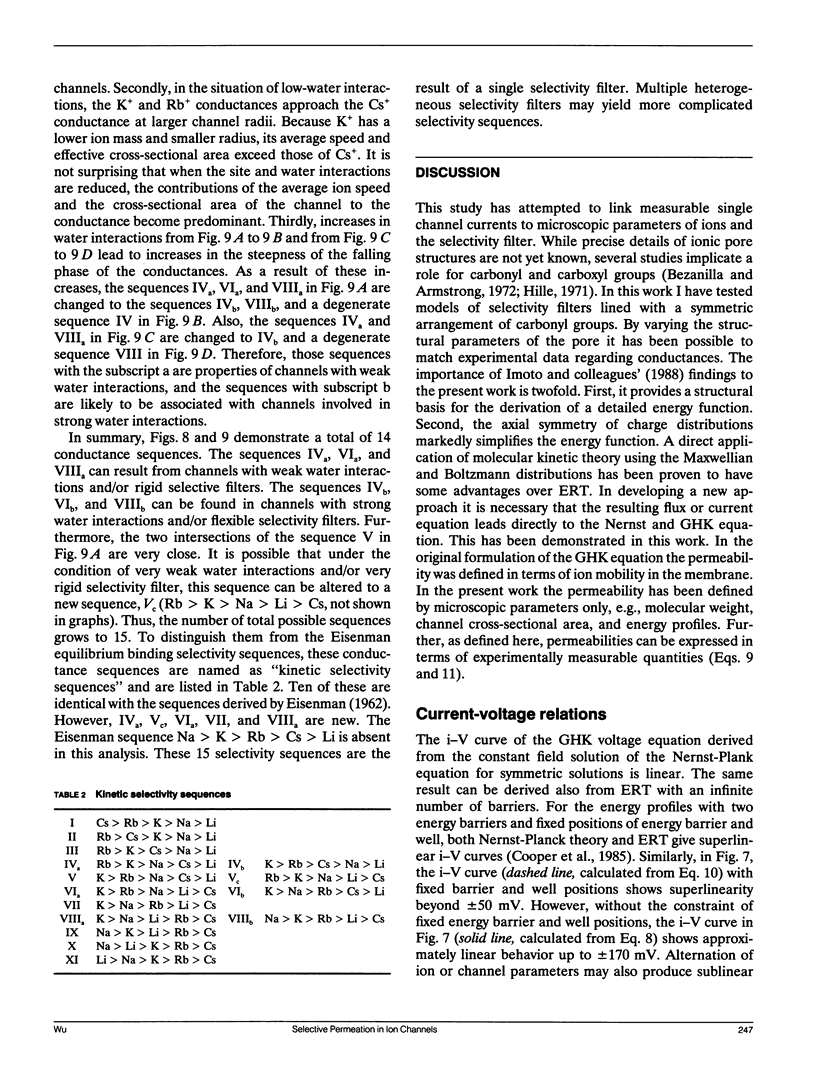
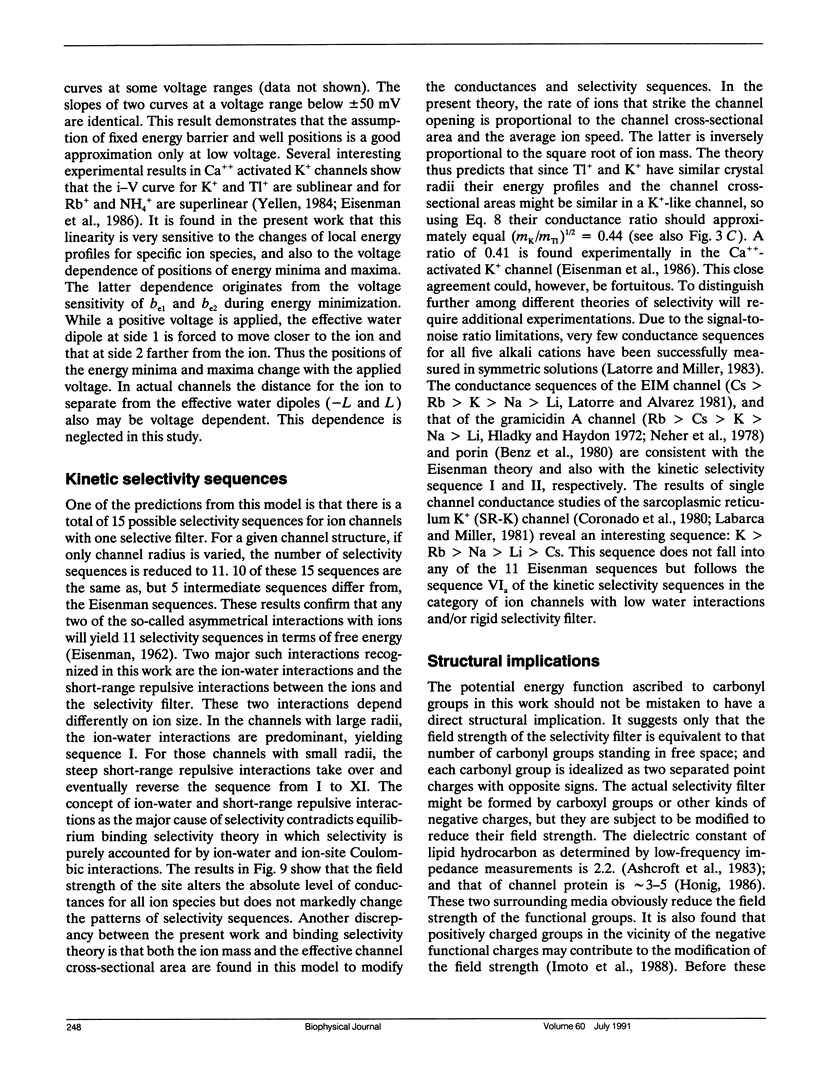
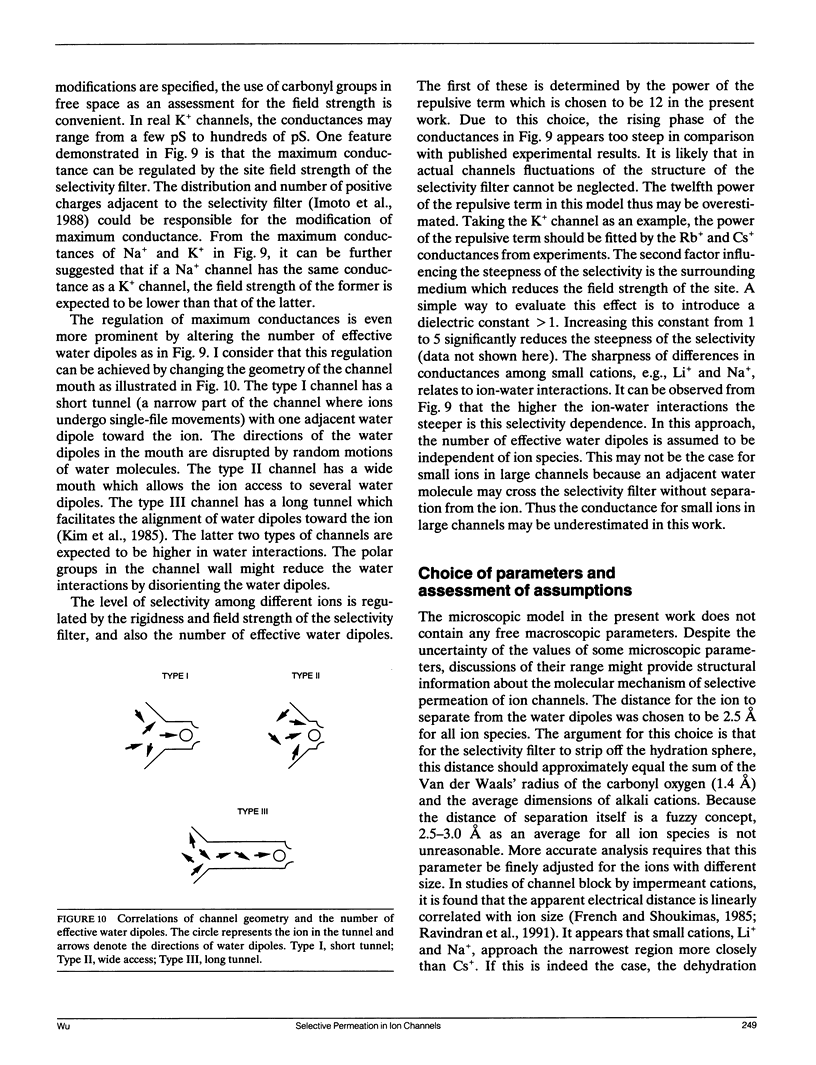
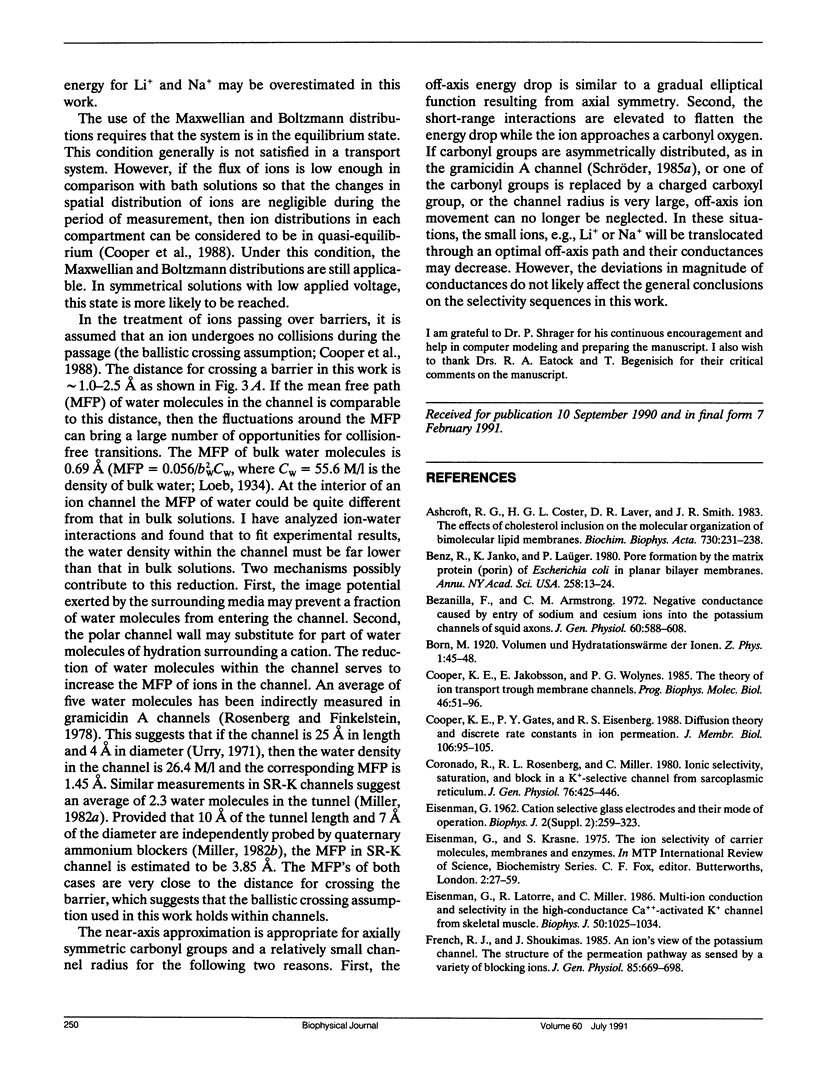
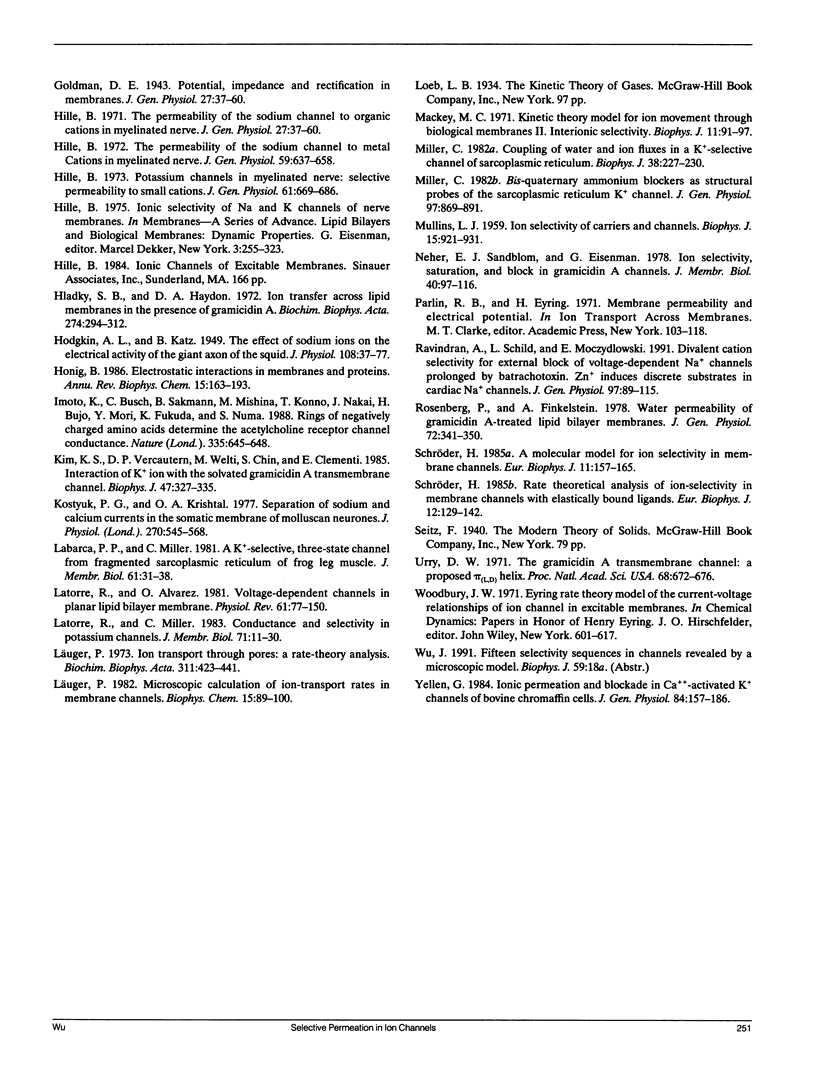
Selected References
These references are in PubMed. This may not be the complete list of references from this article.
- Benz R., Janko K., Läuger P. Pore formation by the matrix protein (porin) of Escherichia coli in planar bilayer membranes. Ann N Y Acad Sci. 1980;358:13–24. doi: 10.1111/j.1749-6632.1980.tb15382.x. [DOI] [PubMed] [Google Scholar]
- Bezanilla F., Armstrong C. M. Negative conductance caused by entry of sodium and cesium ions into the potassium channels of squid axons. J Gen Physiol. 1972 Nov;60(5):588–608. doi: 10.1085/jgp.60.5.588. [DOI] [PMC free article] [PubMed] [Google Scholar]
- Cooper K. E., Gates P. Y., Eisenberg R. S. Diffusion theory and discrete rate constants in ion permeation. J Membr Biol. 1988 Dec;106(2):95–105. doi: 10.1007/BF01871391. [DOI] [PubMed] [Google Scholar]
- Cooper K., Jakobsson E., Wolynes P. The theory of ion transport through membrane channels. Prog Biophys Mol Biol. 1985;46(1):51–96. doi: 10.1016/0079-6107(85)90012-4. [DOI] [PubMed] [Google Scholar]
- Coronado R., Rosenberg R. L., Miller C. Ionic selectivity, saturation, and block in a K+-selective channel from sarcoplasmic reticulum. J Gen Physiol. 1980 Oct;76(4):425–446. doi: 10.1085/jgp.76.4.425. [DOI] [PMC free article] [PubMed] [Google Scholar]
- EISENMAN G. Cation selective glass electrodes and their mode of operation. Biophys J. 1962 Mar;2(2 Pt 2):259–323. doi: 10.1016/s0006-3495(62)86959-8. [DOI] [PMC free article] [PubMed] [Google Scholar]
- Eisenman G., Latorre R., Miller C. Multi-ion conduction and selectivity in the high-conductance Ca++-activated K+ channel from skeletal muscle. Biophys J. 1986 Dec;50(6):1025–1034. doi: 10.1016/S0006-3495(86)83546-9. [DOI] [PMC free article] [PubMed] [Google Scholar]
- French R. J., Shoukimas J. J. An ion's view of the potassium channel. The structure of the permeation pathway as sensed by a variety of blocking ions. J Gen Physiol. 1985 May;85(5):669–698. doi: 10.1085/jgp.85.5.669. [DOI] [PMC free article] [PubMed] [Google Scholar]
- Goldman D. E. POTENTIAL, IMPEDANCE, AND RECTIFICATION IN MEMBRANES. J Gen Physiol. 1943 Sep 20;27(1):37–60. doi: 10.1085/jgp.27.1.37. [DOI] [PMC free article] [PubMed] [Google Scholar]
- HODGKIN A. L., KATZ B. The effect of sodium ions on the electrical activity of giant axon of the squid. J Physiol. 1949 Mar 1;108(1):37–77. doi: 10.1113/jphysiol.1949.sp004310. [DOI] [PMC free article] [PubMed] [Google Scholar]
- Hille B. Potassium channels in myelinated nerve. Selective permeability to small cations. J Gen Physiol. 1973 Jun;61(6):669–686. doi: 10.1085/jgp.61.6.669. [DOI] [PMC free article] [PubMed] [Google Scholar]
- Hille B. The permeability of the sodium channel to metal cations in myelinated nerve. J Gen Physiol. 1972 Jun;59(6):637–658. doi: 10.1085/jgp.59.6.637. [DOI] [PMC free article] [PubMed] [Google Scholar]
- Hladky S. B., Haydon D. A. Ion transfer across lipid membranes in the presence of gramicidin A. I. Studies of the unit conductance channel. Biochim Biophys Acta. 1972 Aug 9;274(2):294–312. doi: 10.1016/0005-2736(72)90178-2. [DOI] [PubMed] [Google Scholar]
- Honig B. H., Hubbell W. L., Flewelling R. F. Electrostatic interactions in membranes and proteins. Annu Rev Biophys Biophys Chem. 1986;15:163–193. doi: 10.1146/annurev.bb.15.060186.001115. [DOI] [PubMed] [Google Scholar]
- Imoto K., Busch C., Sakmann B., Mishina M., Konno T., Nakai J., Bujo H., Mori Y., Fukuda K., Numa S. Rings of negatively charged amino acids determine the acetylcholine receptor channel conductance. Nature. 1988 Oct 13;335(6191):645–648. doi: 10.1038/335645a0. [DOI] [PubMed] [Google Scholar]
- Kim K. S., Vercauteren D. P., Welti M., Chin S., Clementi E. Interaction of K+ ion with the solvated gramicidin A transmembrane channel. Biophys J. 1985 Mar;47(3):327–335. doi: 10.1016/S0006-3495(85)83923-0. [DOI] [PMC free article] [PubMed] [Google Scholar]
- Kostyuk P. G., Krishtal O. A., Shakhovalov Y. A. Separation of sodium and calcium currents in the somatic membrane of mollusc neurones. J Physiol. 1977 Sep;270(3):545–568. doi: 10.1113/jphysiol.1977.sp011968. [DOI] [PMC free article] [PubMed] [Google Scholar]
- Labarca P. P., Miller C. A K+-selective, three-state channel from fragmented sarcoplasmic reticulum of frog leg muscle. J Membr Biol. 1981;61(1):31–38. doi: 10.1007/BF01870750. [DOI] [PubMed] [Google Scholar]
- Latorre R., Alvarez O. Voltage-dependent channels in planar lipid bilayer membranes. Physiol Rev. 1981 Jan;61(1):77–150. doi: 10.1152/physrev.1981.61.1.77. [DOI] [PubMed] [Google Scholar]
- Latorre R., Miller C. Conduction and selectivity in potassium channels. J Membr Biol. 1983;71(1-2):11–30. doi: 10.1007/BF01870671. [DOI] [PubMed] [Google Scholar]
- Läuger P. Ion transport through pores: a rate-theory analysis. Biochim Biophys Acta. 1973 Jul 6;311(3):423–441. doi: 10.1016/0005-2736(73)90323-4. [DOI] [PubMed] [Google Scholar]
- Läuger P. Microscopic calculation of ion-transport rates in membrane channels. Biophys Chem. 1982 May;15(2):89–100. doi: 10.1016/0301-4622(82)80021-5. [DOI] [PubMed] [Google Scholar]
- Mackey M. C. Kinetic theory model for ion movement through biological membranes. II. Interionic selectivity. Biophys J. 1971 Jan;11(1):91–97. doi: 10.1016/S0006-3495(71)86197-0. [DOI] [PMC free article] [PubMed] [Google Scholar]
- Miller C. Bis-quaternary ammonium blockers as structural probes of the sarcoplasmic reticulum K+ channel. J Gen Physiol. 1982 May;79(5):869–891. doi: 10.1085/jgp.79.5.869. [DOI] [PMC free article] [PubMed] [Google Scholar]
- Miller C. Coupling of water and ion fluxes in a K+-selective channel of sarcoplasmic reticulum. Biophys J. 1982 Jun;38(3):227–230. doi: 10.1016/S0006-3495(82)84552-9. [DOI] [PMC free article] [PubMed] [Google Scholar]
- Mullins L. J. Ion selectivity of carriers and channels. Biophys J. 1975 Sep;15(9):921–931. doi: 10.1016/S0006-3495(75)85867-X. [DOI] [PMC free article] [PubMed] [Google Scholar]
- Neher E., Sandblom J., Eisenman G. Ionic selectivity, saturation, and block in gramicidin A channels. II. Saturation behavior of single channel conductances and evidence for the existence of multiple binding sites in the channel. J Membr Biol. 1978 Apr 26;40(2):97–116. doi: 10.1007/BF01871143. [DOI] [PubMed] [Google Scholar]
- Ravindran A., Schild L., Moczydlowski E. Divalent cation selectivity for external block of voltage-dependent Na+ channels prolonged by batrachotoxin. Zn2+ induces discrete substates in cardiac Na+ channels. J Gen Physiol. 1991 Jan;97(1):89–115. doi: 10.1085/jgp.97.1.89. [DOI] [PMC free article] [PubMed] [Google Scholar]
- Rosenberg P. A., Finkelstein A. Water permeability of gramicidin A-treated lipid bilayer membranes. J Gen Physiol. 1978 Sep;72(3):341–350. doi: 10.1085/jgp.72.3.341. [DOI] [PMC free article] [PubMed] [Google Scholar]
- Schröder H. A molecular model for ion selectivity in membrane channels. Eur Biophys J. 1985;11(3):157–165. doi: 10.1007/BF00257394. [DOI] [PubMed] [Google Scholar]
- Schröder H. Rate theoretical analysis of ion-selectivity in membrane channels with elastically bound ligands. Eur Biophys J. 1985;12(3):129–142. doi: 10.1007/BF00254071. [DOI] [PubMed] [Google Scholar]
- Urry D. W. The gramicidin A transmembrane channel: a proposed pi(L,D) helix. Proc Natl Acad Sci U S A. 1971 Mar;68(3):672–676. doi: 10.1073/pnas.68.3.672. [DOI] [PMC free article] [PubMed] [Google Scholar]
- Yellen G. Ionic permeation and blockade in Ca2+-activated K+ channels of bovine chromaffin cells. J Gen Physiol. 1984 Aug;84(2):157–186. doi: 10.1085/jgp.84.2.157. [DOI] [PMC free article] [PubMed] [Google Scholar]