Abstract
Molecular dynamics simulations have been done on a system consisting of the polypeptide membrane channel former gramicidin, plus water molecules in the channel and caps of waters at the two ends of the channel. In the absence of explicit simulation of the surrounding membrane, the helical form of the channel was maintained by artificial restraints on the peptide motion. The characteristic time constant of the artificial restraint was varied to assess the effect of the restraints on the channel structure and water motions. Time-correlation analysis was done on the motions of individual channel waters and on the motions of the center of mass of the channel waters. It is found that individual water molecules confined in the channel execute higher frequency motions than bulk water, for all degrees of channel peptide restraint. The center-of-mass motion of the chain of channel waters (which is the motion that is critical for transmembrane transport, due to the mandatory single filing of water in the channel) does not exhibit these higher frequency motions. The mobility of the water chain is dramatically reduced by holding the channel rigid. Thus permeation through the channel is not like flow through a rigid pipe; rather permeation is facilitated by peptide motion. For the looser restraints we used, the mobility of the water chain was not very much affected by the degree of restraint. Depending on which set of experiments is considered, the computed mobility of our water chain in the flexible channel is four to twenty times too high to account for the experimentally measured resistance of the gramicidin channel to water flow. From this result it appears likely that the peptide motions of an actual gramicidin channel embedded in a lipid membrane may be more restrained than in our flexible channel model, and that these restraints may be a significant modulator of channel permeability. For the completely rigid channel model the "trapping" of the water molecules in preferred positions throughout the molecular dynamics run precludes a reasonable assessment of mobility, but it seems to be quite low.
Full text
PDF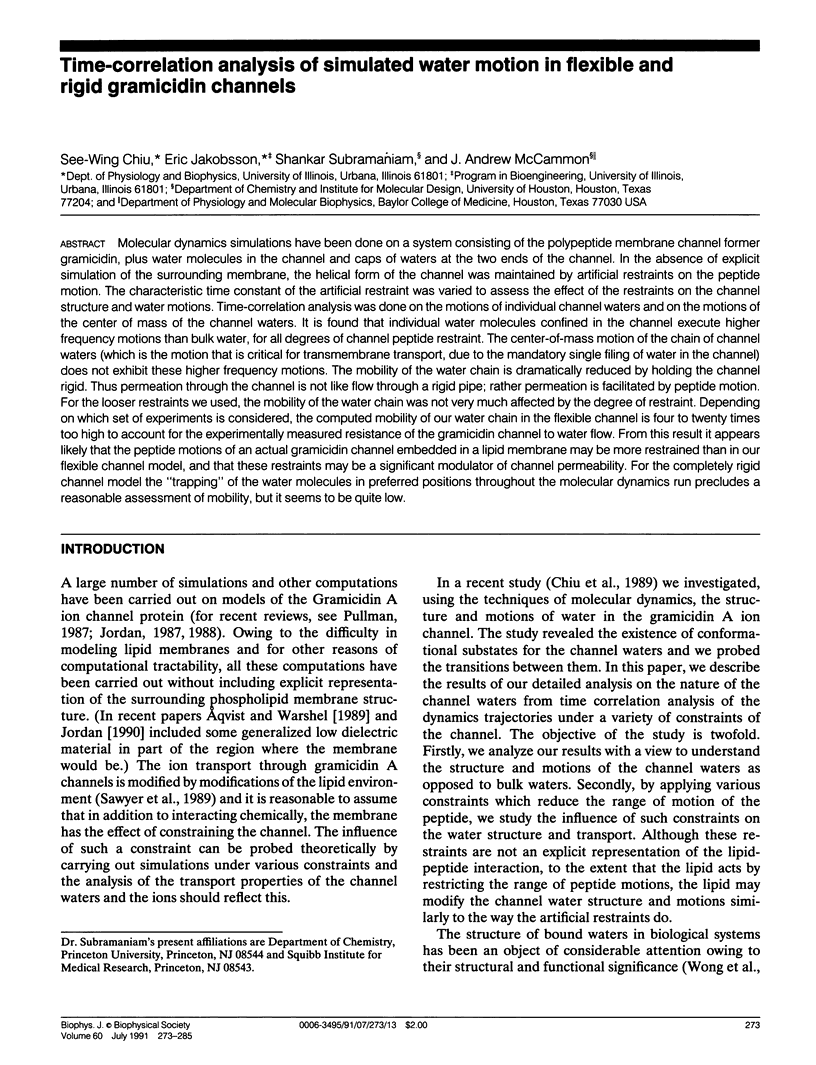
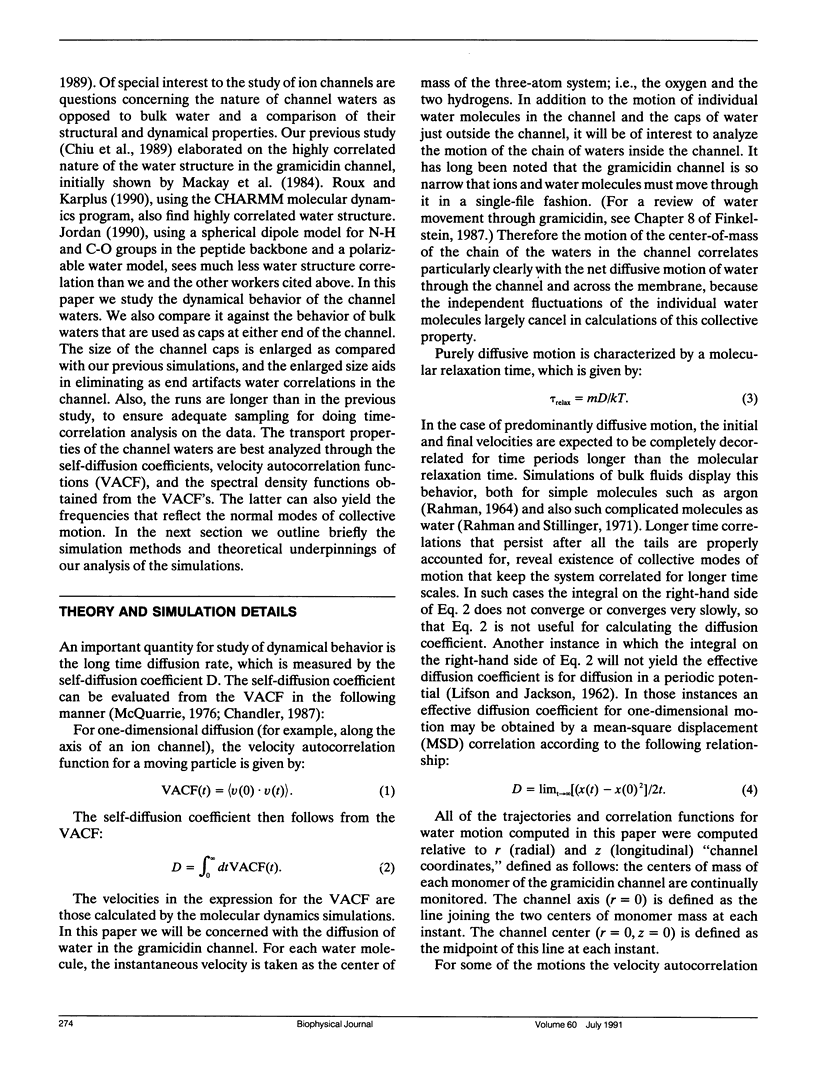
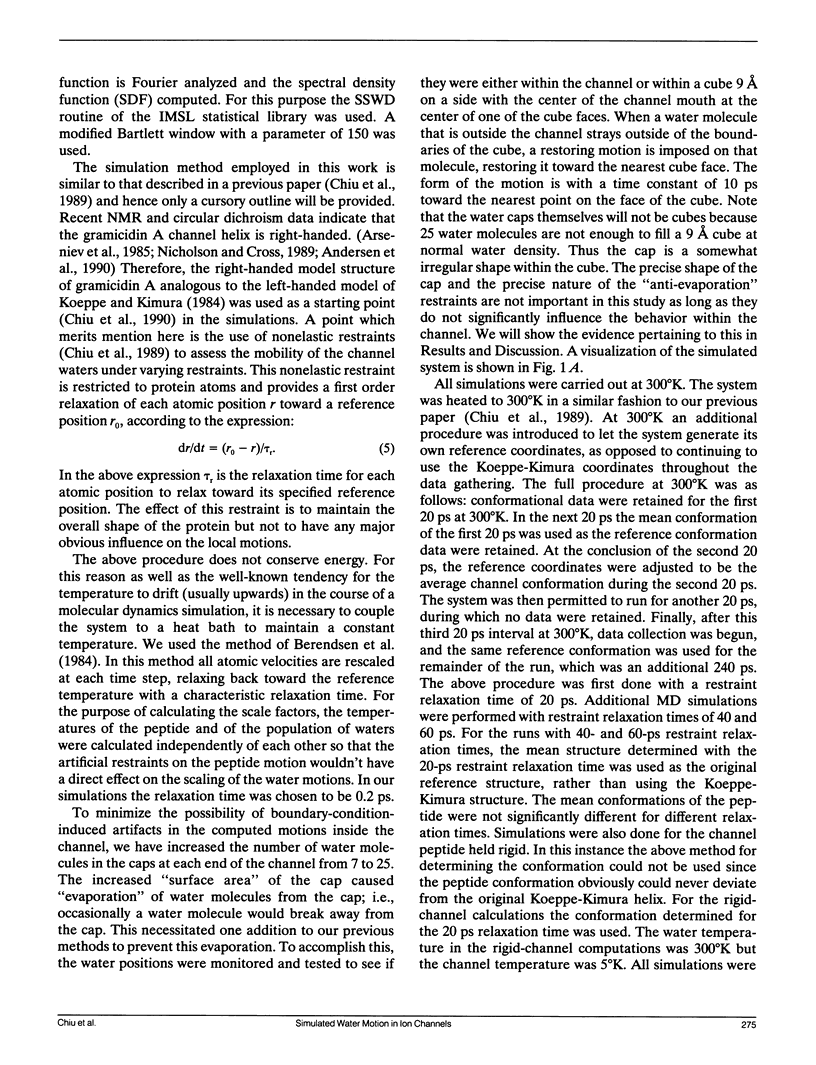
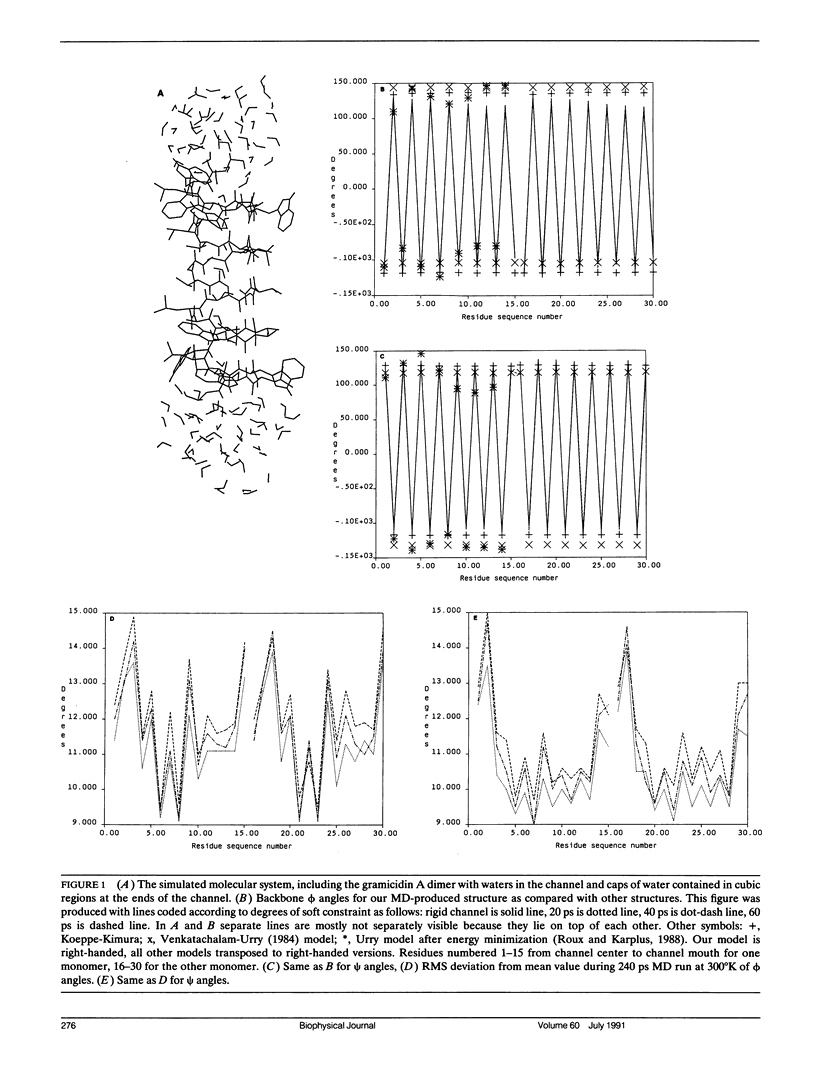
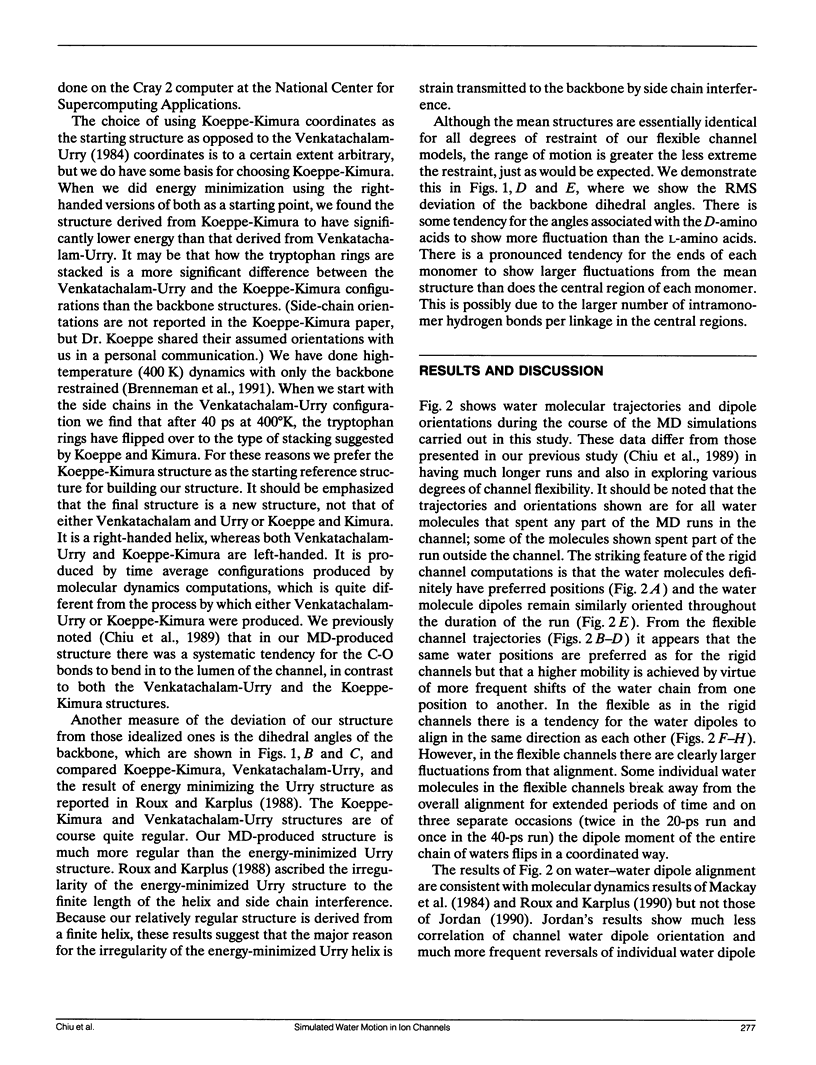
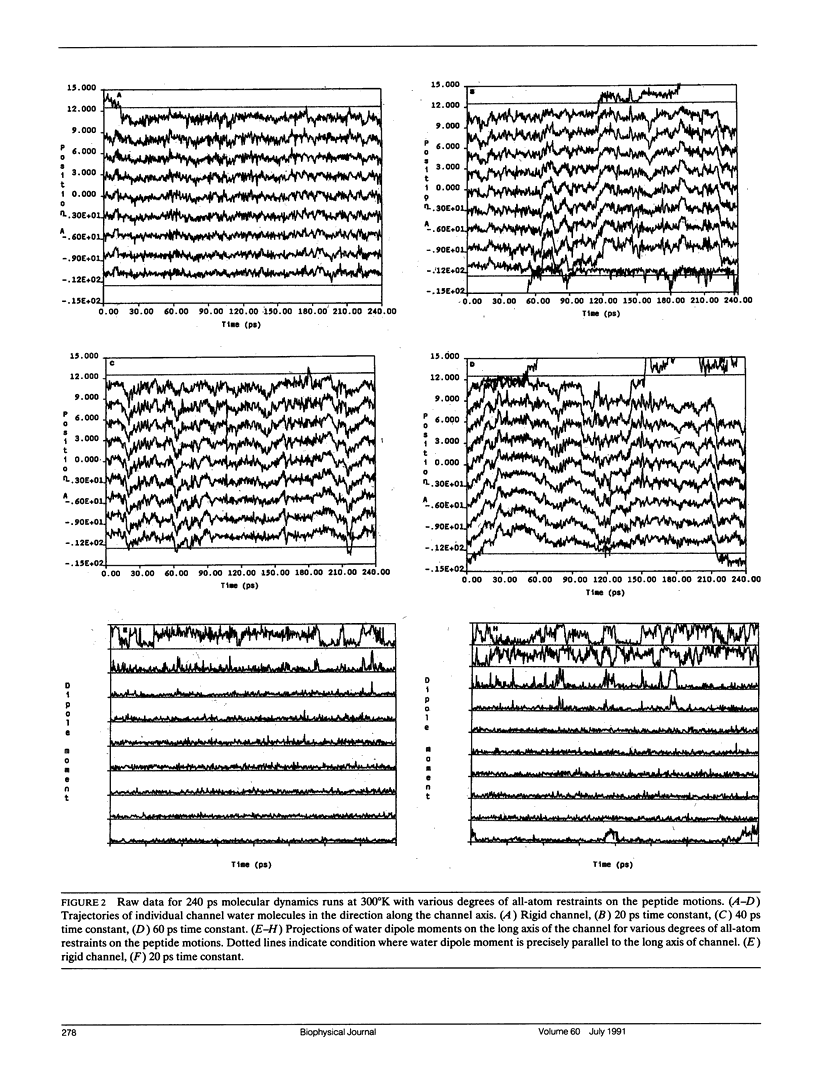
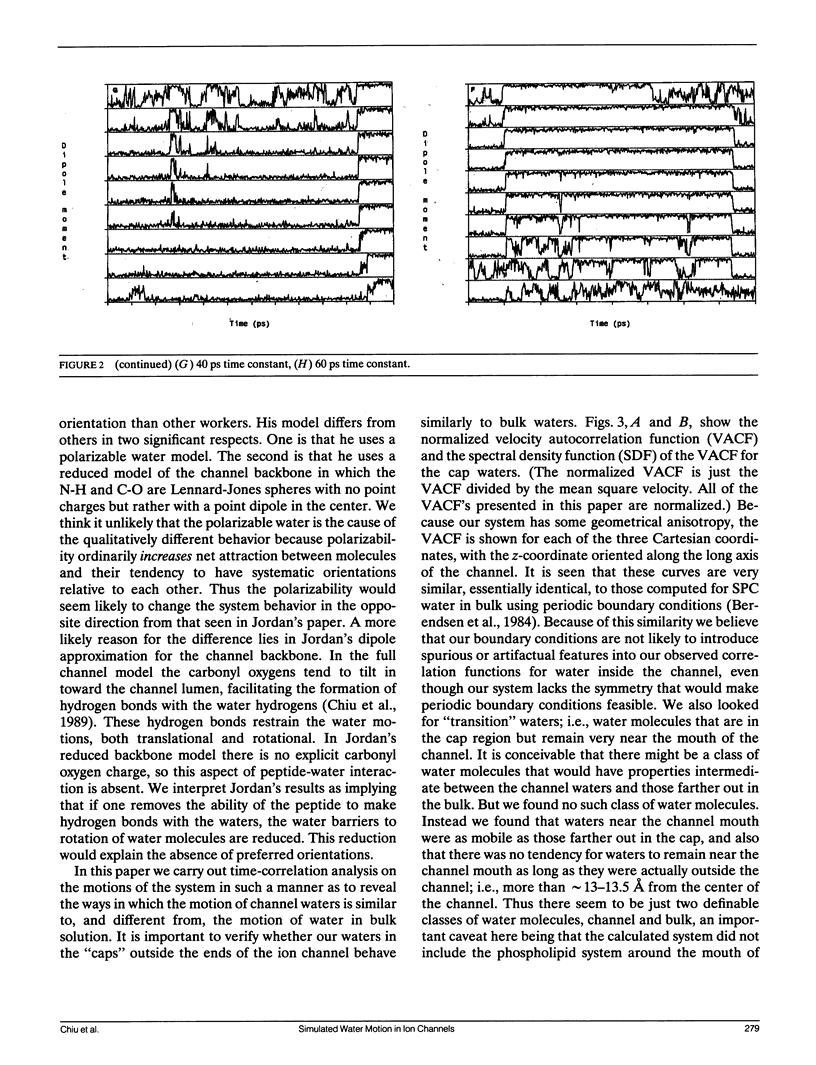
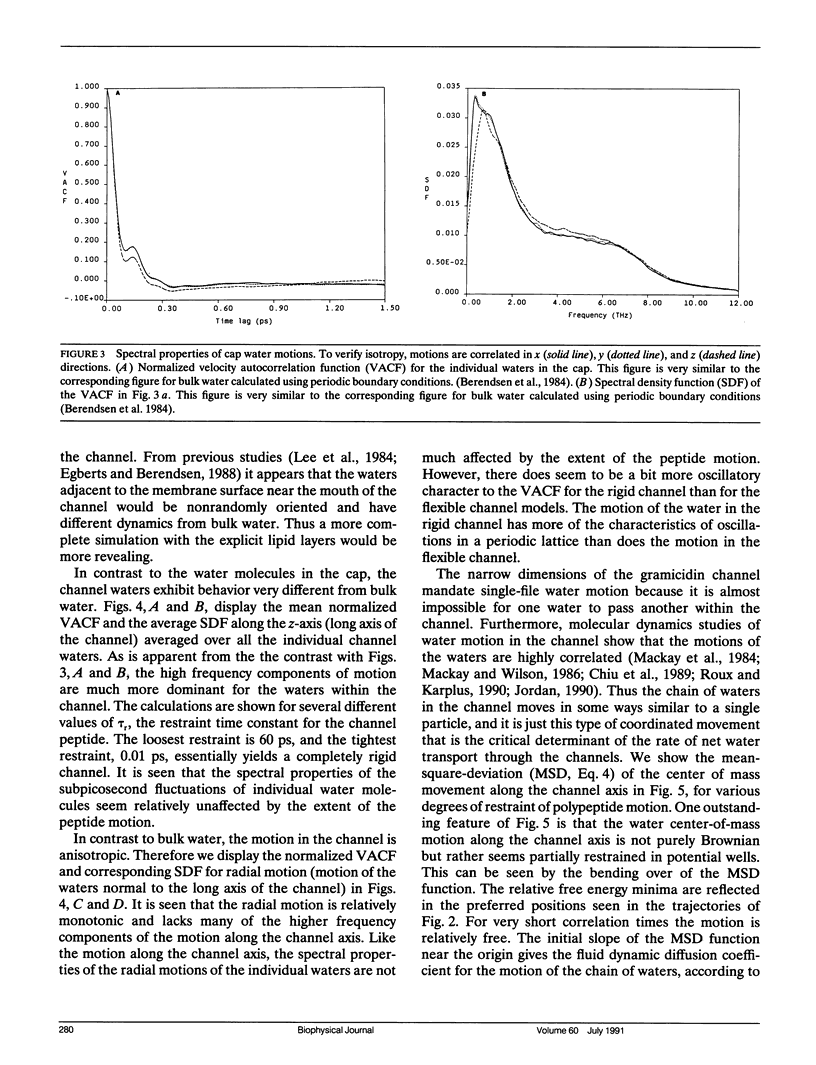
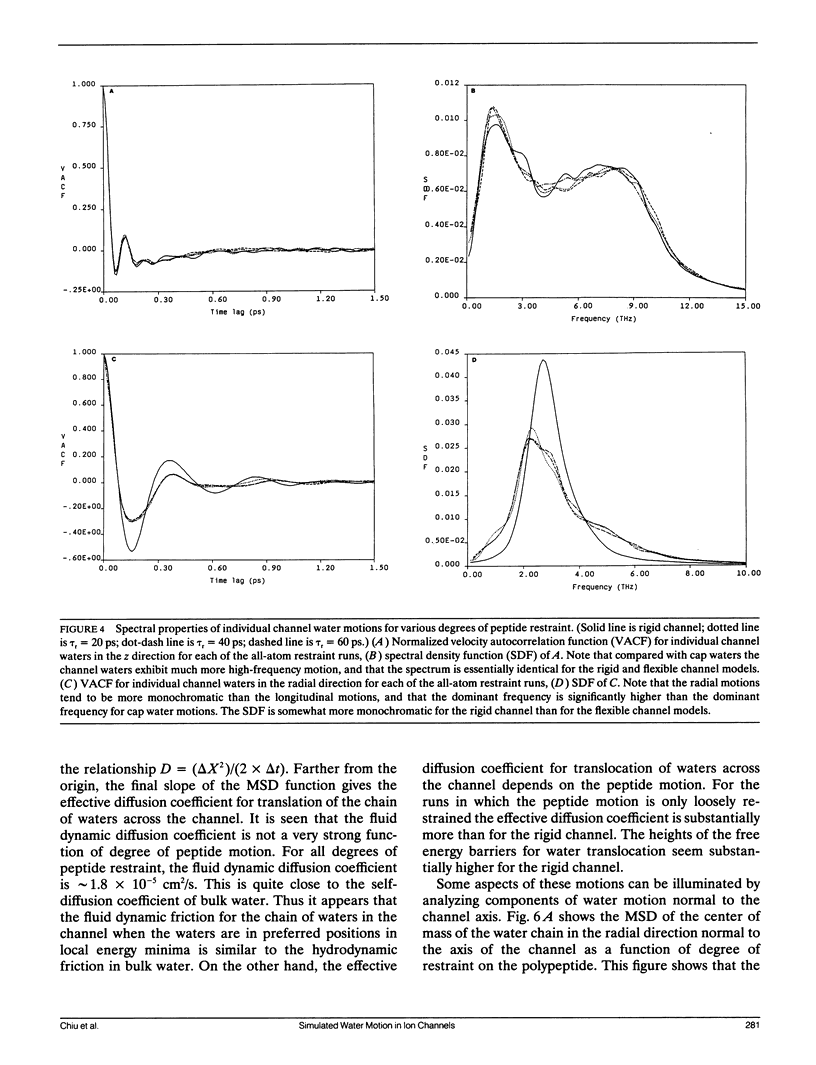
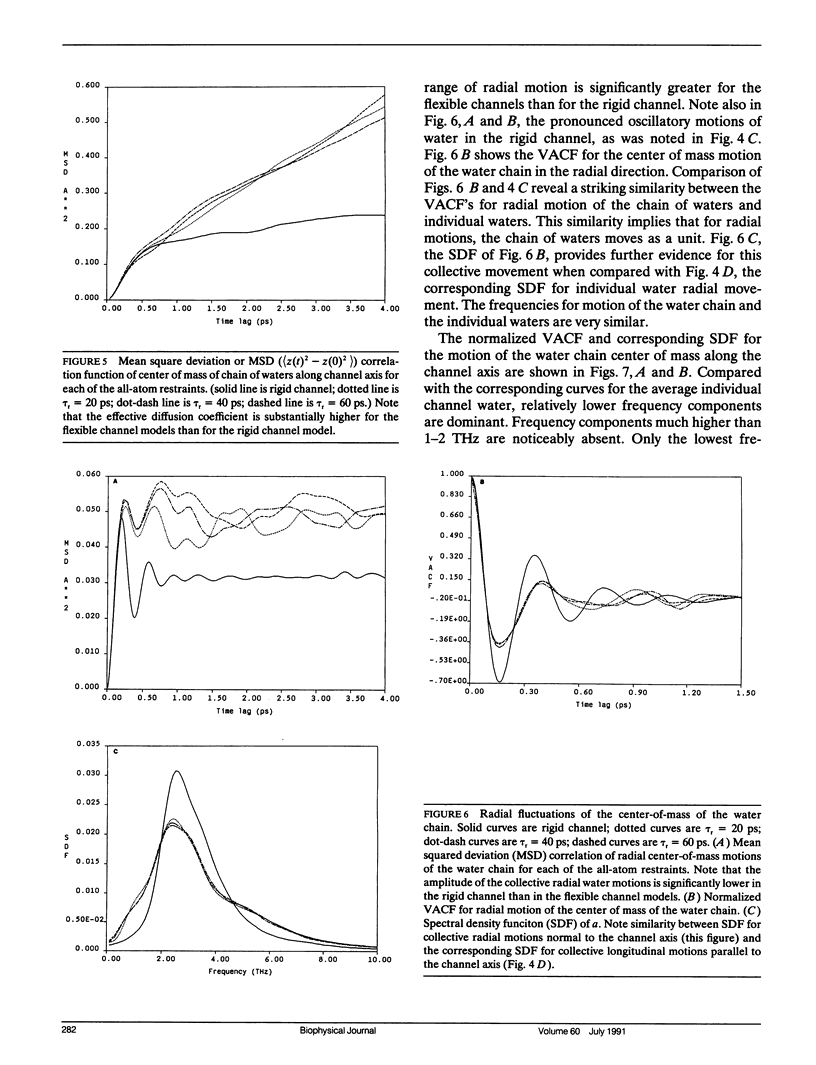
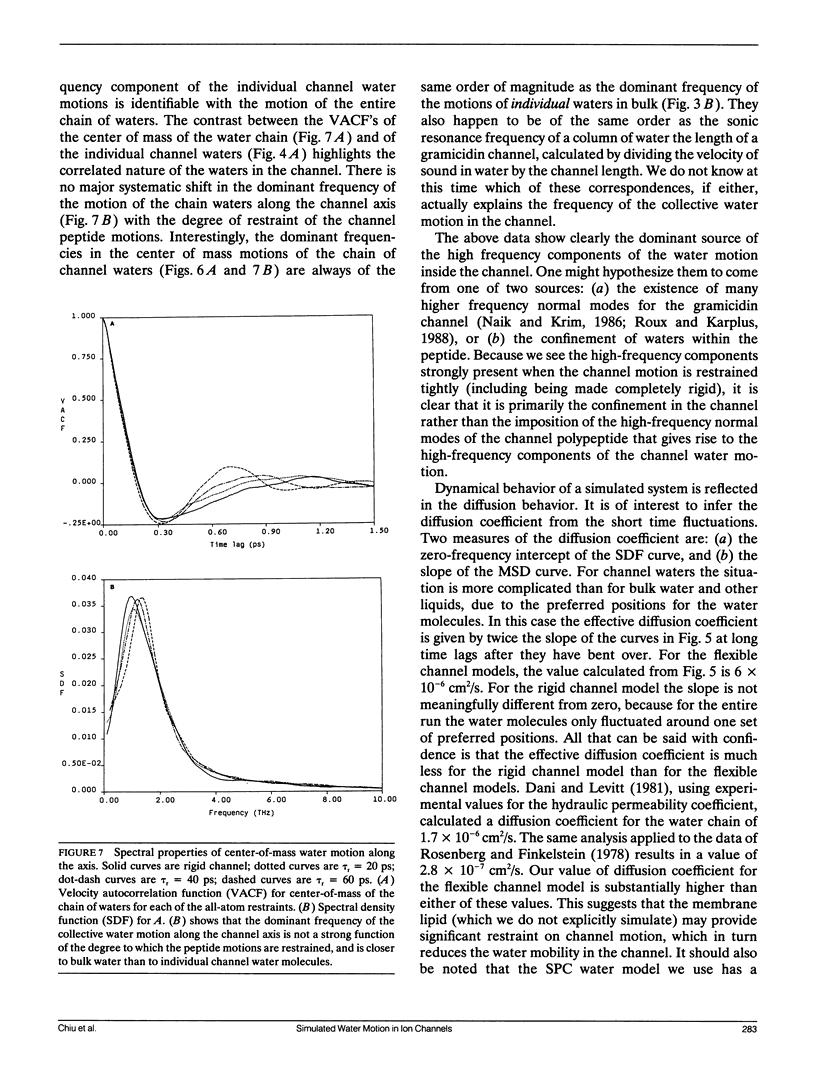
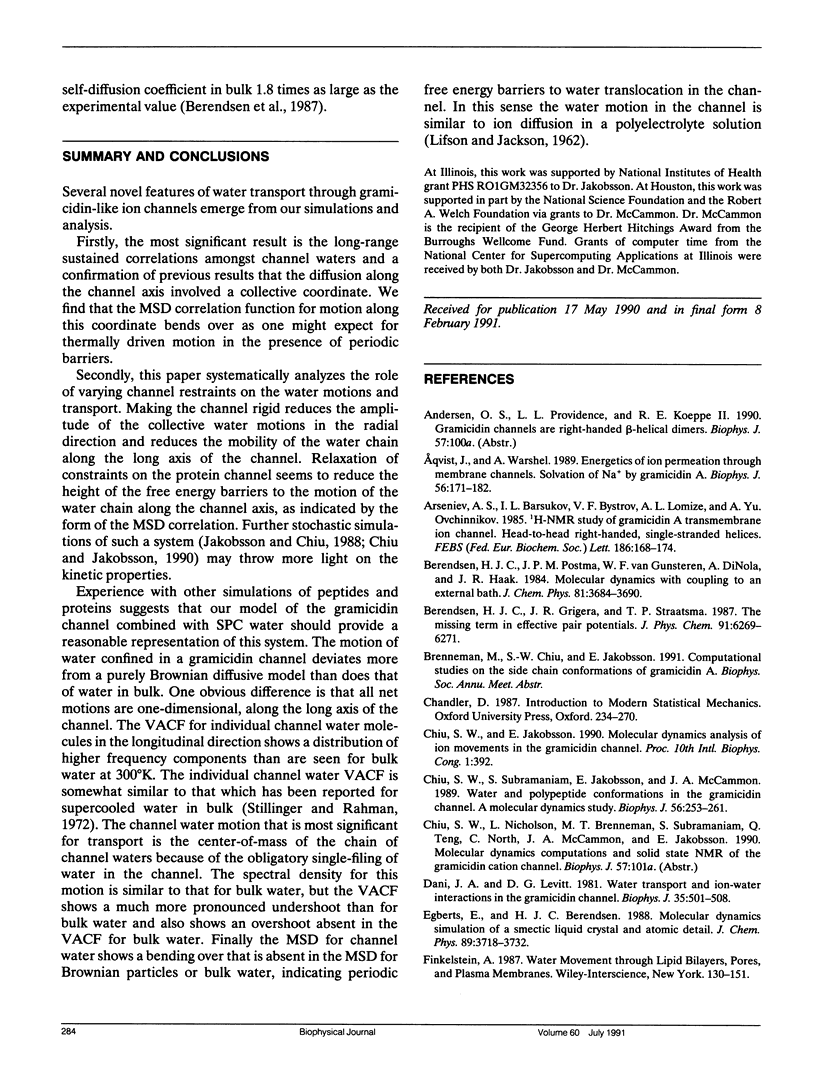
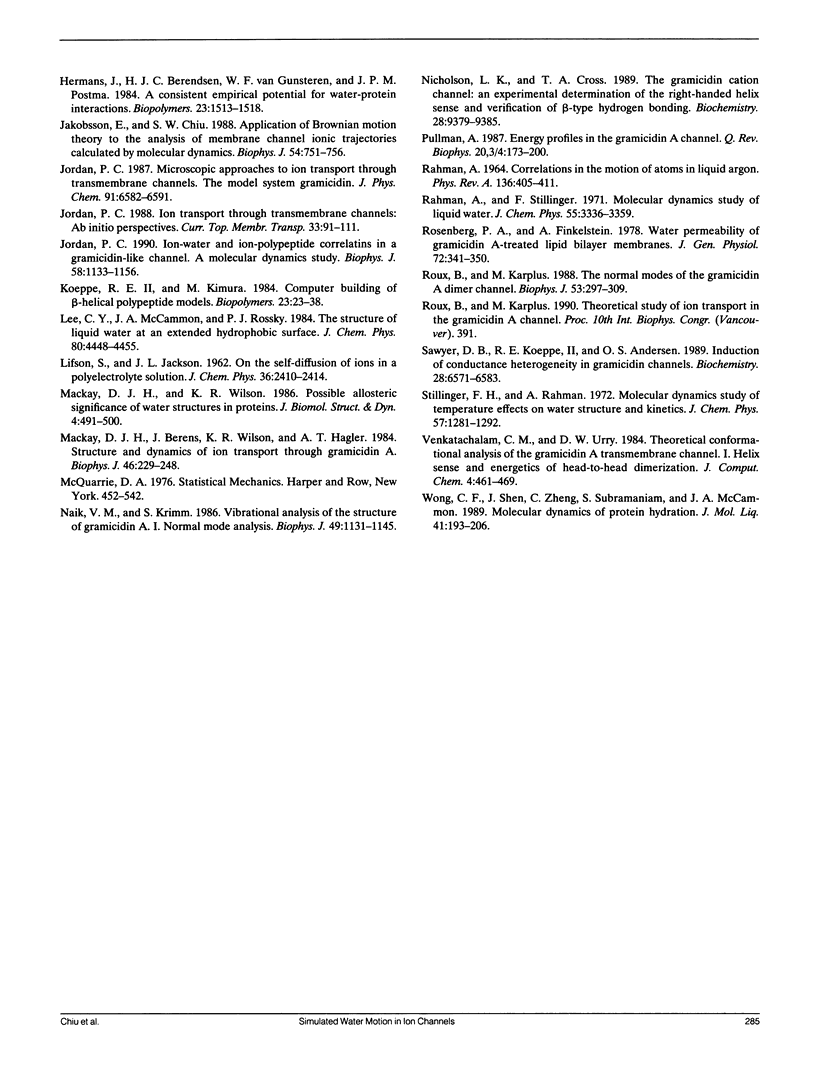
Selected References
These references are in PubMed. This may not be the complete list of references from this article.
- Aqvist J., Warshel A. Energetics of ion permeation through membrane channels. Solvation of Na+ by gramicidin A. Biophys J. 1989 Jul;56(1):171–182. doi: 10.1016/S0006-3495(89)82662-1. [DOI] [PMC free article] [PubMed] [Google Scholar]
- Arseniev A. S., Barsukov I. L., Bystrov V. F., Lomize A. L., Ovchinnikov YuA 1H-NMR study of gramicidin A transmembrane ion channel. Head-to-head right-handed, single-stranded helices. FEBS Lett. 1985 Jul 8;186(2):168–174. doi: 10.1016/0014-5793(85)80702-x. [DOI] [PubMed] [Google Scholar]
- Chiu S. W., Subramaniam S., Jakobsson E., McCammon J. A. Water and polypeptide conformations in the gramicidin channel. A molecular dynamics study. Biophys J. 1989 Aug;56(2):253–261. doi: 10.1016/S0006-3495(89)82671-2. [DOI] [PMC free article] [PubMed] [Google Scholar]
- Dani J. A., Levitt D. G. Water transport and ion-water interaction in the gramicidin channel. Biophys J. 1981 Aug;35(2):501–508. doi: 10.1016/S0006-3495(81)84805-9. [DOI] [PMC free article] [PubMed] [Google Scholar]
- Jakobsson E., Chiu S. W. Application of Brownian motion theory to the analysis of membrane channel ionic trajectories calculated by molecular dynamics. Biophys J. 1988 Oct;54(4):751–756. doi: 10.1016/S0006-3495(88)83012-1. [DOI] [PMC free article] [PubMed] [Google Scholar]
- Jordan P. C. Ion-water and ion-polypeptide correlations in a gramicidin-like channel. A molecular dynamics study. Biophys J. 1990 Nov;58(5):1133–1156. doi: 10.1016/S0006-3495(90)82456-5. [DOI] [PMC free article] [PubMed] [Google Scholar]
- Mackay D. H., Berens P. H., Wilson K. R., Hagler A. T. Structure and dynamics of ion transport through gramicidin A. Biophys J. 1984 Aug;46(2):229–248. doi: 10.1016/S0006-3495(84)84016-3. [DOI] [PMC free article] [PubMed] [Google Scholar]
- Mackay D. H., Wilson K. R. Possible allosteric significance of water structures in proteins. J Biomol Struct Dyn. 1986 Dec;4(3):491–500. doi: 10.1080/07391102.1986.10506364. [DOI] [PubMed] [Google Scholar]
- Naik V. M., Krimm S. Vibrational analysis of the structure of gramicidin A. I. Normal mode analysis. Biophys J. 1986 Jun;49(6):1131–1145. doi: 10.1016/S0006-3495(86)83742-0. [DOI] [PMC free article] [PubMed] [Google Scholar]
- Nicholson L. K., Cross T. A. Gramicidin cation channel: an experimental determination of the right-handed helix sense and verification of beta-type hydrogen bonding. Biochemistry. 1989 Nov 28;28(24):9379–9385. doi: 10.1021/bi00450a019. [DOI] [PubMed] [Google Scholar]
- Pullman A. Energy profiles in the gramicidin A channel. Q Rev Biophys. 1987 Nov;20(3-4):173–200. doi: 10.1017/s0033583500004170. [DOI] [PubMed] [Google Scholar]
- Rosenberg P. A., Finkelstein A. Water permeability of gramicidin A-treated lipid bilayer membranes. J Gen Physiol. 1978 Sep;72(3):341–350. doi: 10.1085/jgp.72.3.341. [DOI] [PMC free article] [PubMed] [Google Scholar]
- Roux B., Karplus M. The normal modes of the gramicidin-A dimer channel. Biophys J. 1988 Mar;53(3):297–309. doi: 10.1016/S0006-3495(88)83107-2. [DOI] [PMC free article] [PubMed] [Google Scholar]
- Sawyer D. B., Koeppe R. E., 2nd, Andersen O. S. Induction of conductance heterogeneity in gramicidin channels. Biochemistry. 1989 Aug 8;28(16):6571–6583. doi: 10.1021/bi00442a007. [DOI] [PubMed] [Google Scholar]