Abstract
Dielectrophoresis and electrorotation are commonly used to measure dielectric properties and membrane electrical parameters of biological cells. We have derived quantitative relationships for several critical points, defined in Fig. A 1, which characterize the dielectrophoretic spectrum and the electrorotational spectrum of a cell, based on the single-shell model (Pauly, H., and H.P. Schwan, 1959. Z. Naturforsch. 14b:125-131; Sauer, F.A. 1985. Interactions between Electromagnetic Field and Cells. A. Chiabrera, C. Nicolini, and H.P. Schwan, editors. Plenum Publishing Corp., New York. 181-202). To test these equations and to obtain membrane electrical parameters, a technique which allowed simultaneous measurements of the dielectrophoresis and the electrorotation of single cells in the same chamber, was developed and applied to the study of Neurospora slime and the Myeloma Tib9 cell line. Membrane electrical parameters were determined by the dependence of the first critical frequency of dielectrophoresis (fct1) and the first characteristic frequency of electrorotation (fc1) on the conductivity of the suspending medium. Membrane conductances of Neurospora slime and Myeloma also were found to be 500 and 380 S m-2, respectively. Several observations indicate that these cells are more complex than that described by the single-shell model. First, the membrane capacities from fct1 (0.81 x 10(-2) and 1.55 x 10(-2) F m-2 for neurospora slime and Myeloma, respectively) were at least twice those derived from fc1. Second, the electrorotation spectrum of Myeloma cells deviated from the single-shell like behavior. These discrepancies could be eliminated by adapting a three-shell model (Furhr, G., J. Gimsa, and R. Glaser. 1985. Stud. Biophys. 108:149-164). Apparently, there was more than one membrane relaxation process which could influence the lower frequency region of the beta-dispersion. fct1 of Myeloma in a medium of given external conductivity were found to be similar for most cells, but for some a dramatically increased fct1 was recorded. Model analysis suggested that a decrease in the cytoplasmatic conductivity due to a drastic ion loss in a cell could cause this increase in fct1. Model analysis also suggested that the electrorotation spectrum in the counter-field rotation range and fc1 would be more sensitive to conductivity changes of the cytoplasmic fluid and to the influence of internal membranes than would fct1, although the latter would be sensitive to changes in capacitance of the cytoplasmic membranes.
Full text
PDF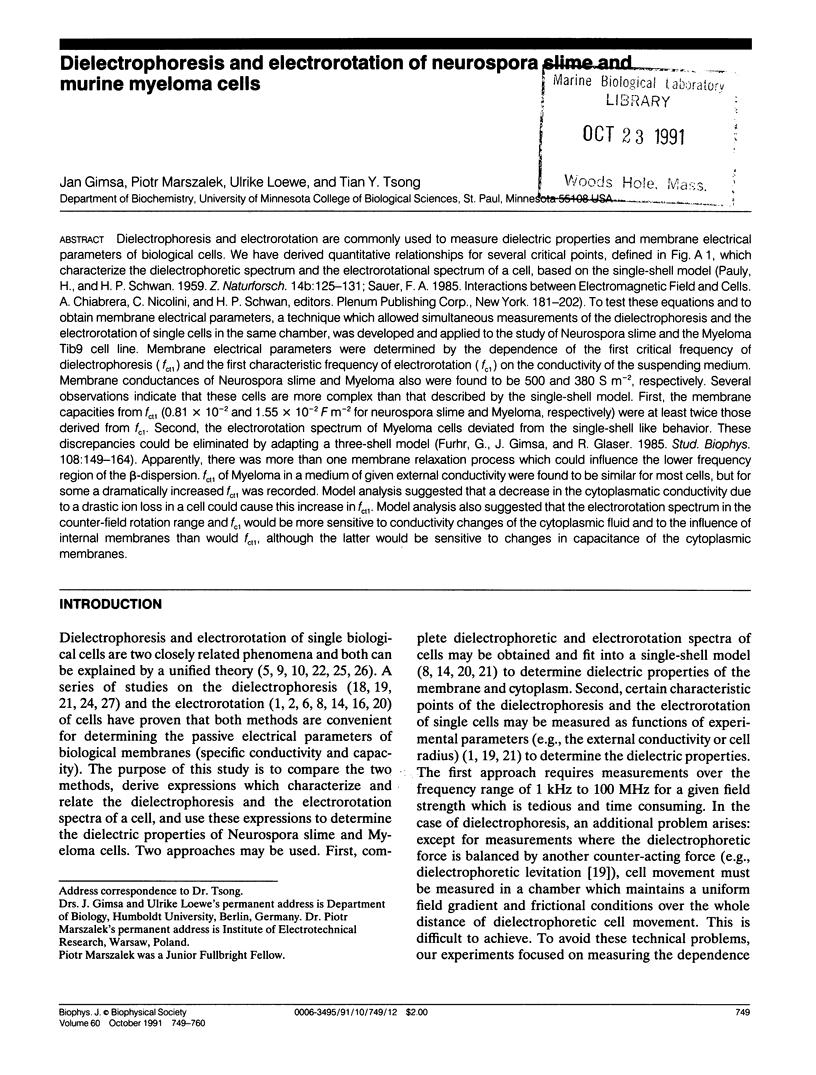
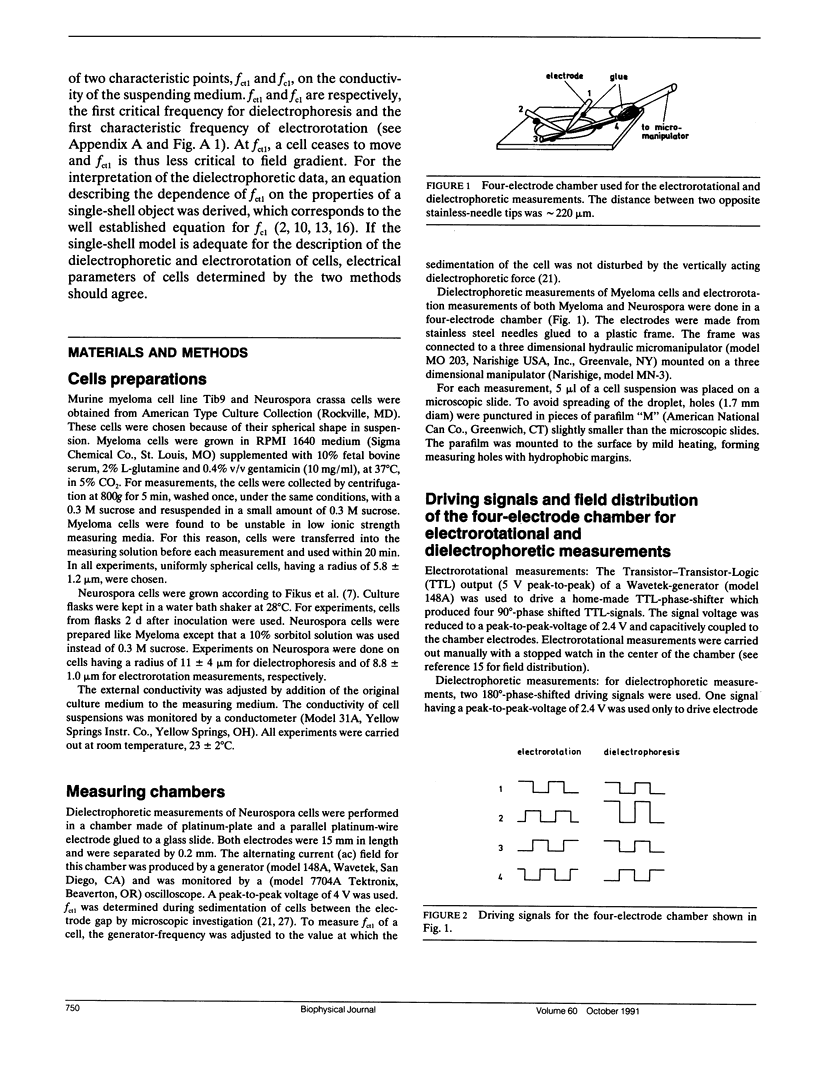
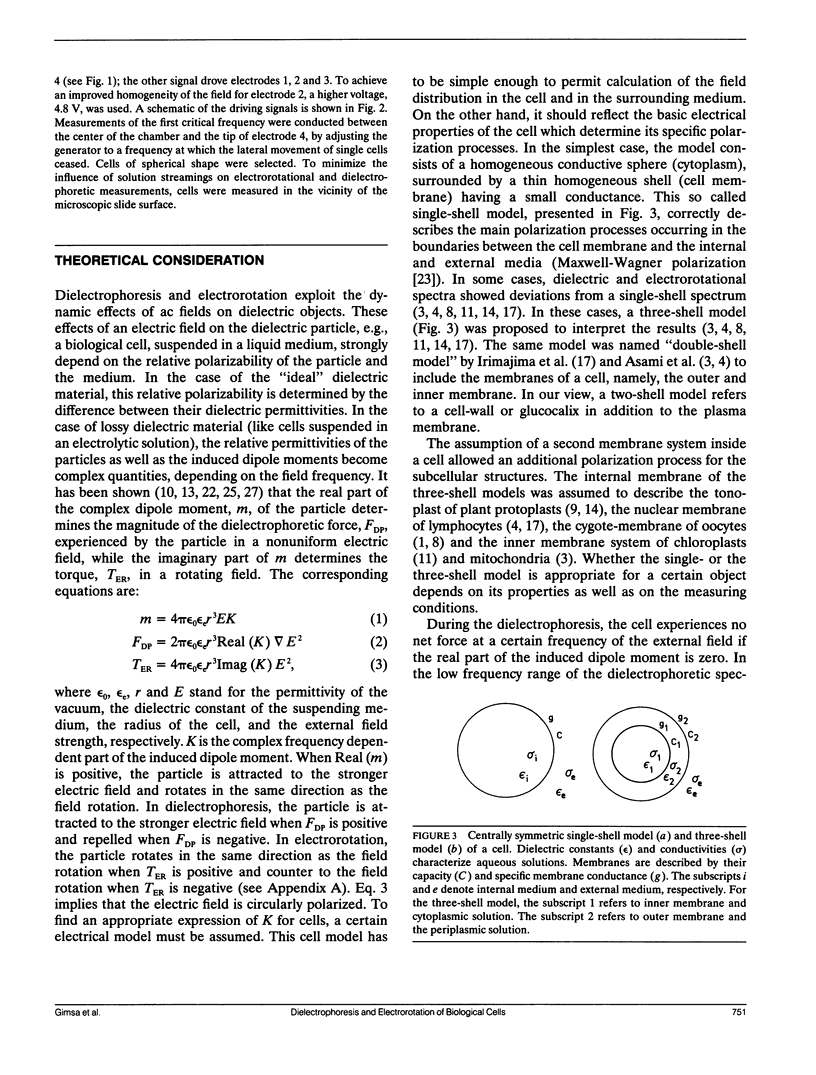
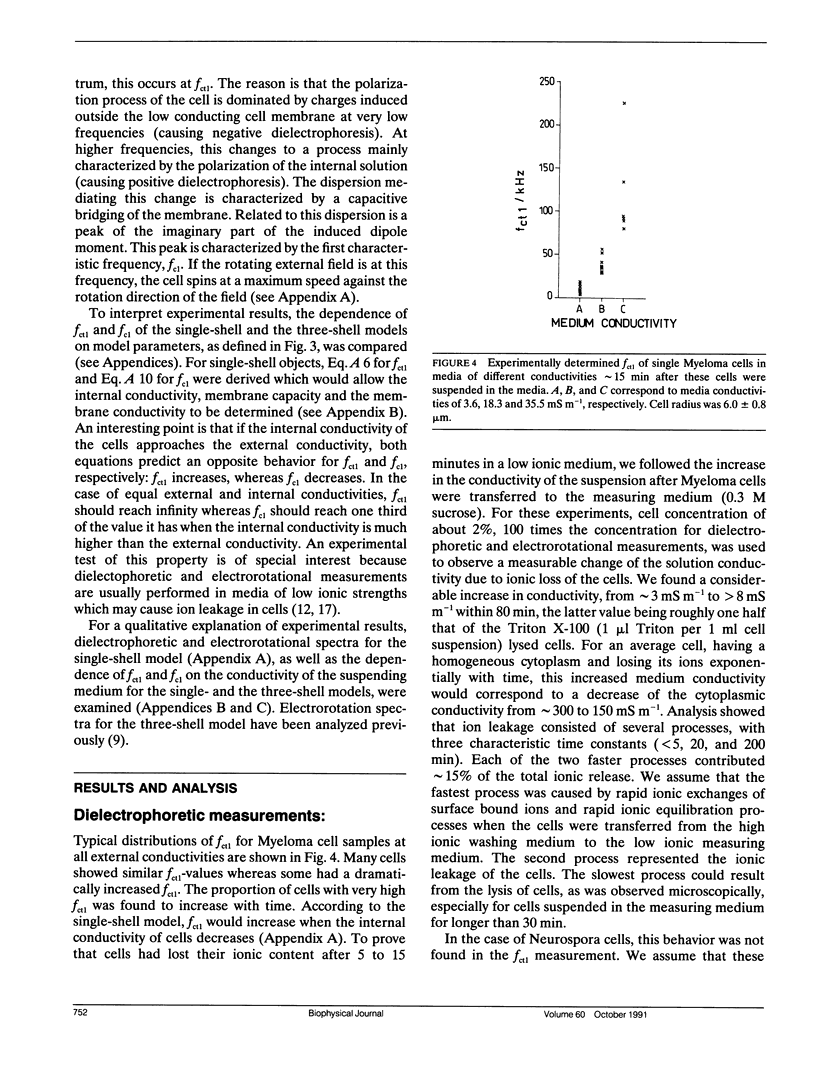
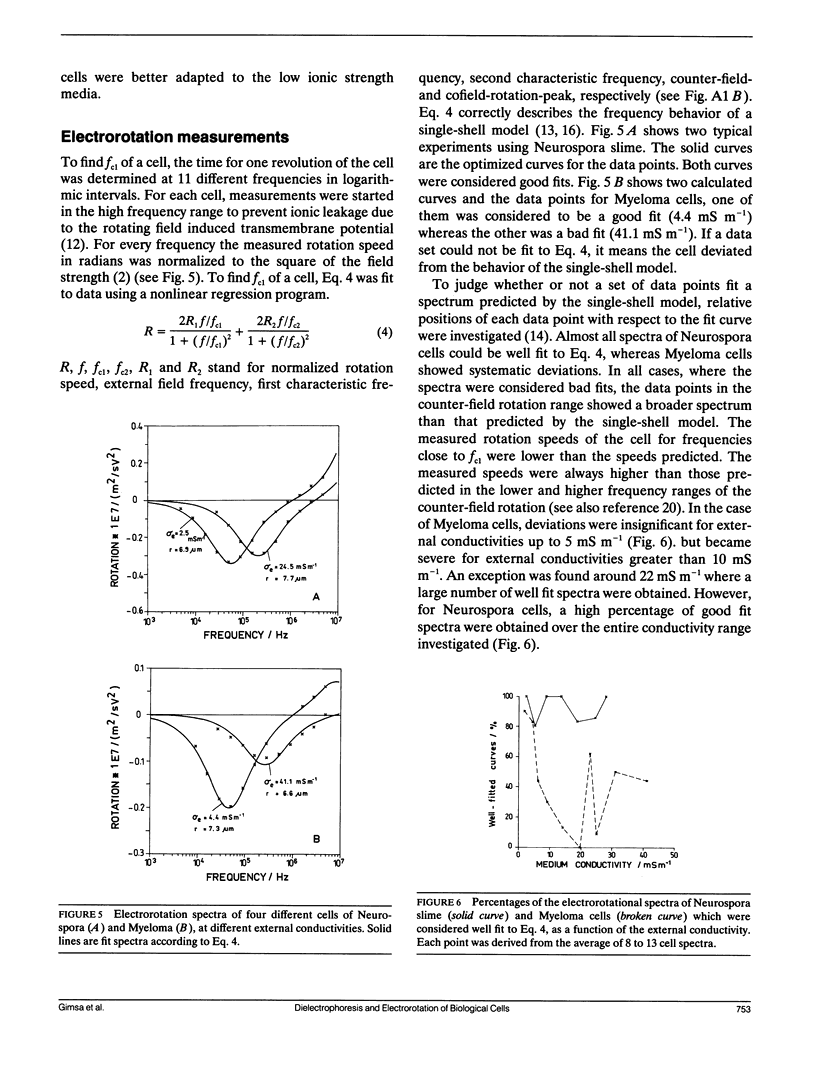
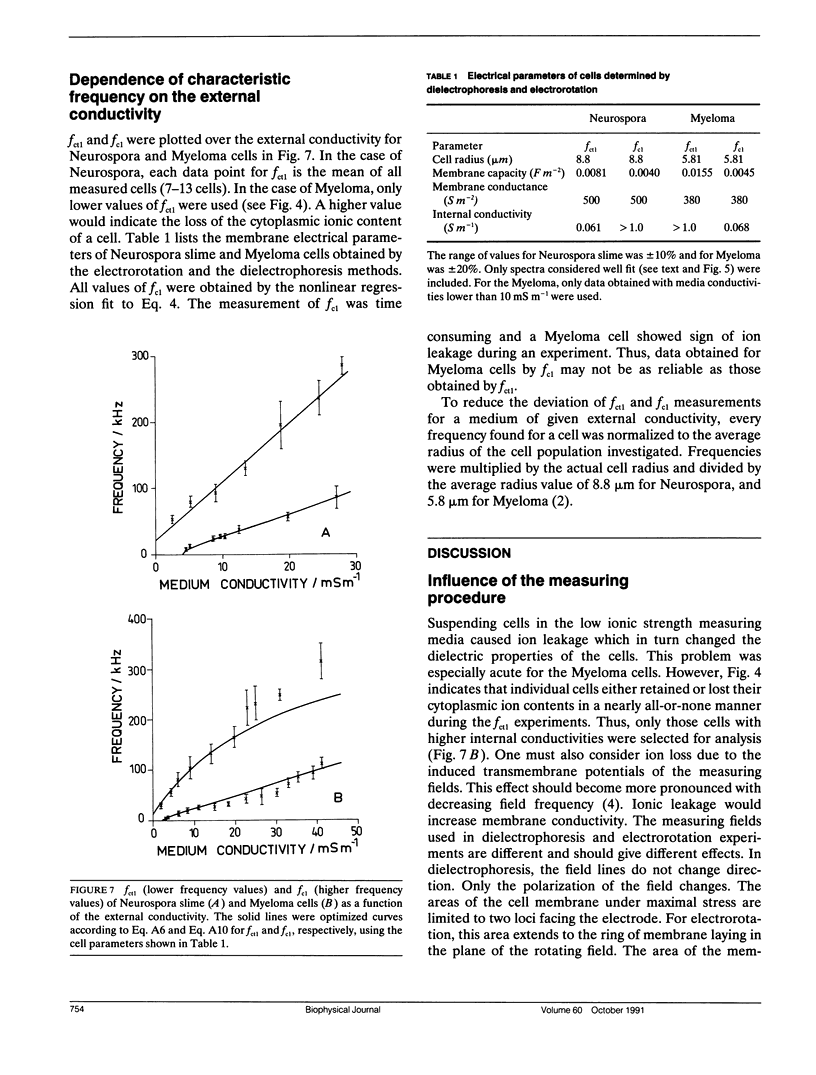
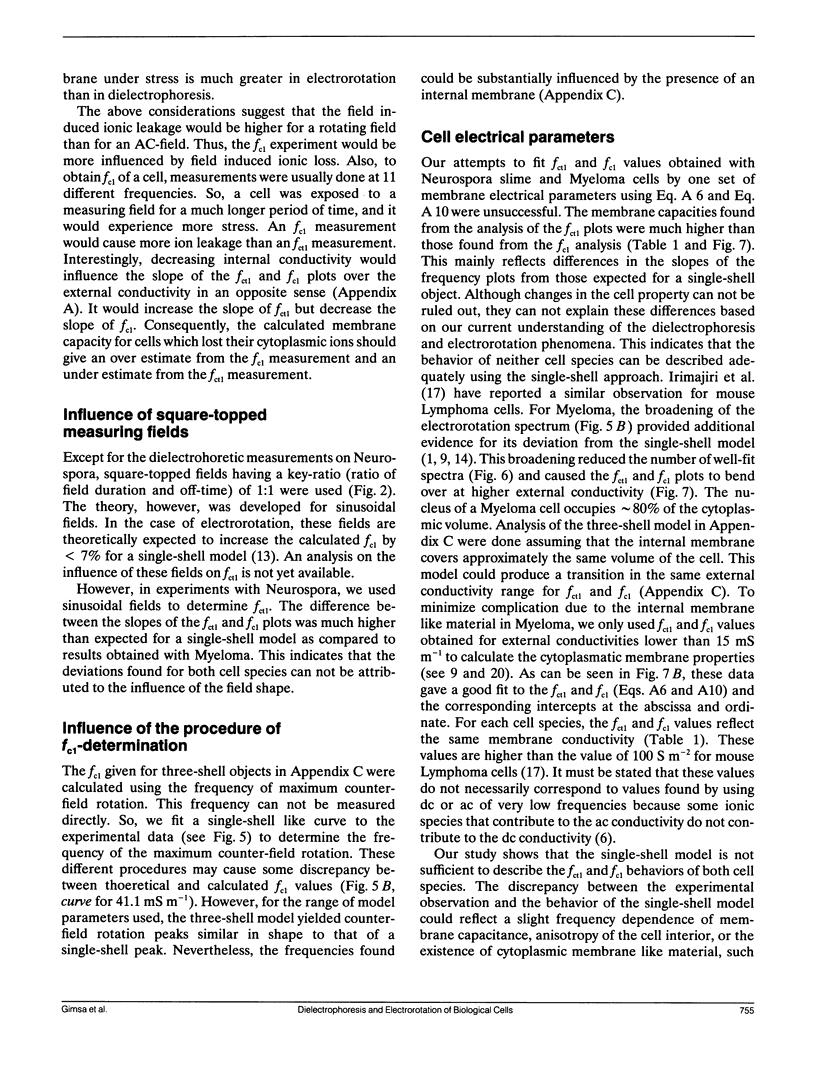
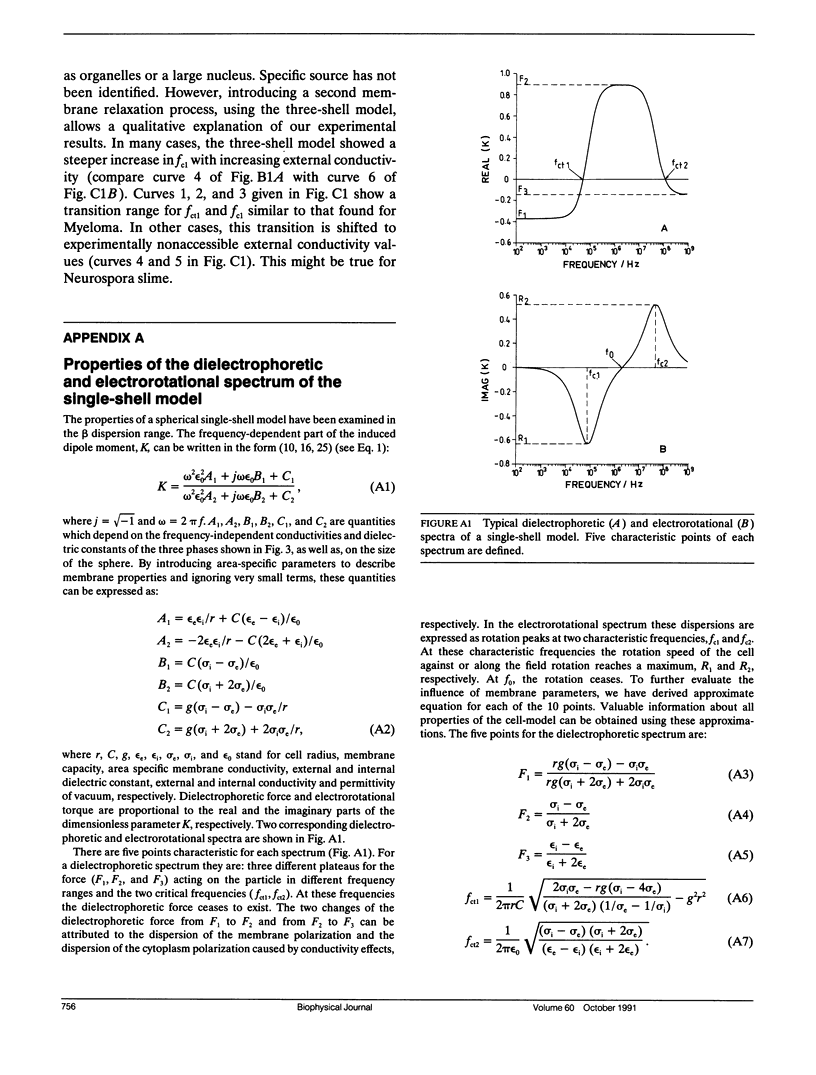
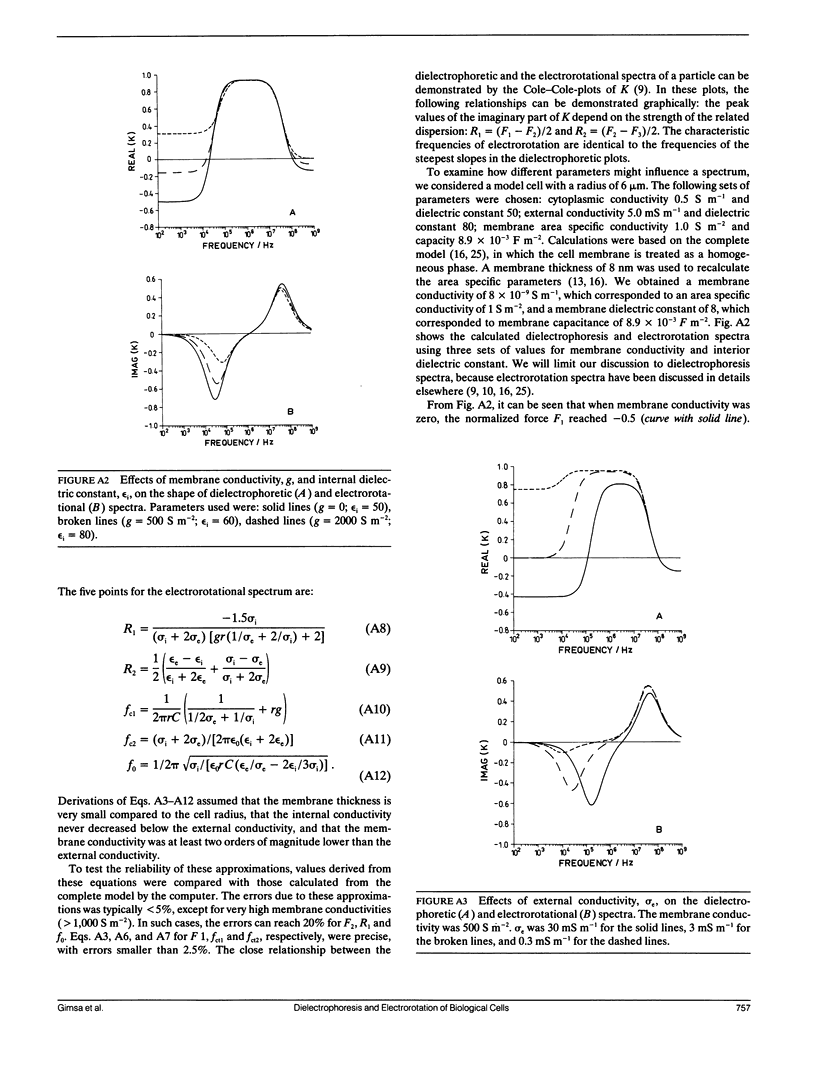
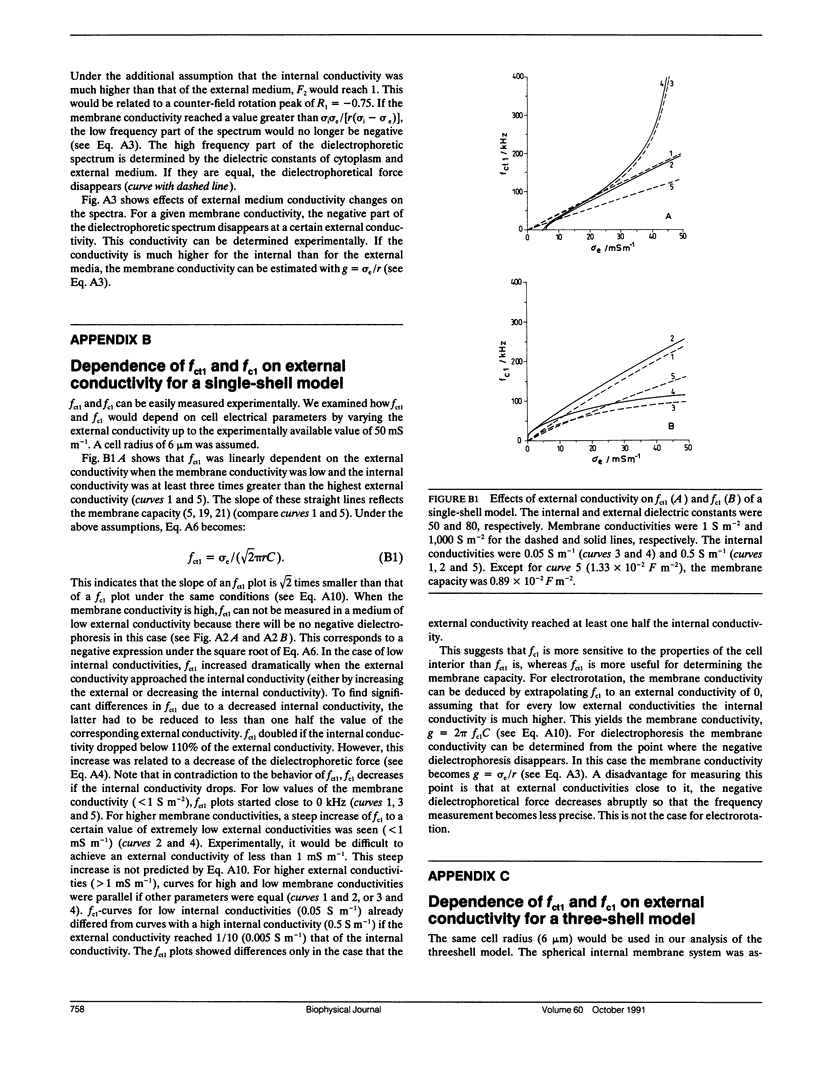
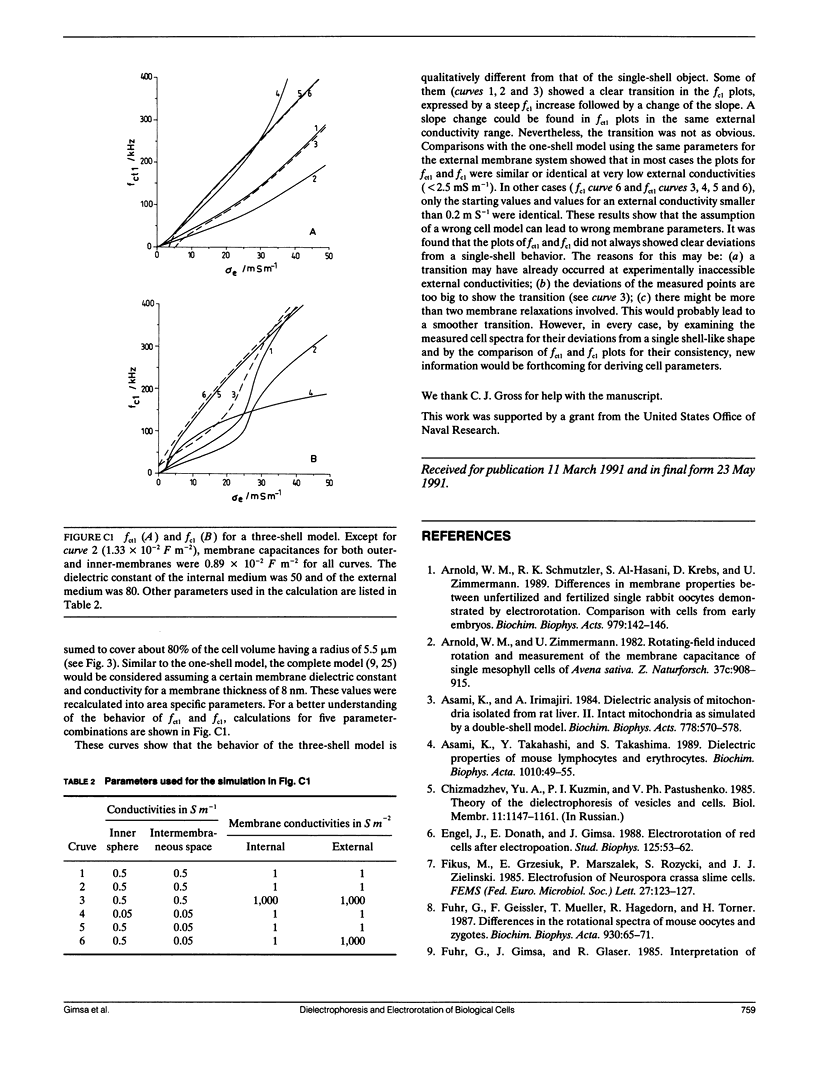
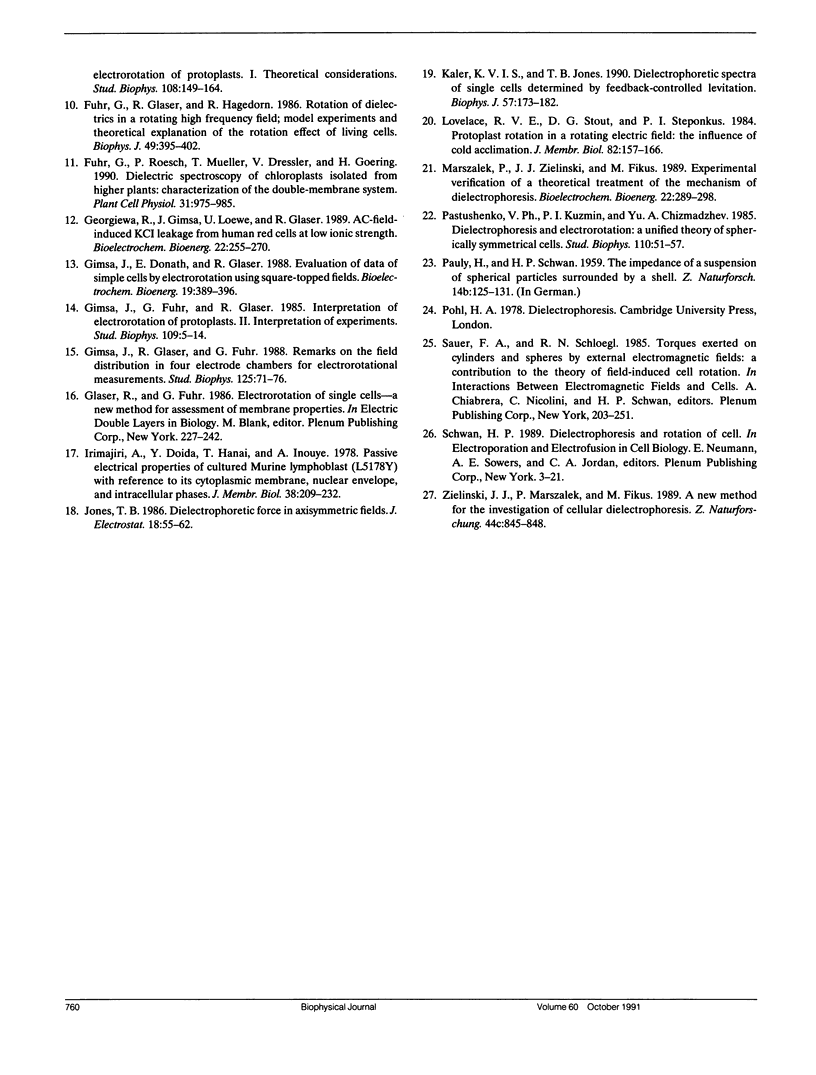
Selected References
These references are in PubMed. This may not be the complete list of references from this article.
- Arnold W. M., Schmutzler R. K., Al-Hasani S., Krebs D., Zimmermann U. Differences in membrane properties between unfertilised and fertilised single rabbit oocytes demonstrated by electro-rotation. Comparison with cells from early embryos. Biochim Biophys Acta. 1989 Feb 13;979(1):142–146. doi: 10.1016/0005-2736(89)90535-x. [DOI] [PubMed] [Google Scholar]
- Asami K., Irimajiri A. Dielectric analysis of mitochondria isolated from rat liver. II. Intact mitochondria as simulated by a double-shell model. Biochim Biophys Acta. 1984 Dec 19;778(3):570–578. doi: 10.1016/0005-2736(84)90408-5. [DOI] [PubMed] [Google Scholar]
- Asami K., Takahashi Y., Takashima S. Dielectric properties of mouse lymphocytes and erythrocytes. Biochim Biophys Acta. 1989 Jan 17;1010(1):49–55. doi: 10.1016/0167-4889(89)90183-3. [DOI] [PubMed] [Google Scholar]
- Fuhr G., Geissler F., Müller T., Hagedorn R., Torner H. Differences in the rotation spectra of mouse oocytes and zygotes. Biochim Biophys Acta. 1987 Aug 19;930(1):65–71. doi: 10.1016/0167-4889(87)90156-x. [DOI] [PubMed] [Google Scholar]
- Fuhr G., Glaser R., Hagedorn R. Rotation of dielectrics in a rotating electric high-frequency field. Model experiments and theoretical explanation of the rotation effect of living cells. Biophys J. 1986 Feb;49(2):395–402. doi: 10.1016/S0006-3495(86)83649-9. [DOI] [PMC free article] [PubMed] [Google Scholar]
- Irimajiri A., Doida Y., Hanai T., Inouye A. Passive electrical properties of cultured murine lymphoblast (L5178Y) with reference to its cytoplasmic membrane, nuclear envelope, and intracellular phases. J Membr Biol. 1978 Jan 18;38(3):209–232. doi: 10.1007/BF01871923. [DOI] [PubMed] [Google Scholar]
- Kaler K. V., Jones T. B. Dielectrophoretic spectra of single cells determined by feedback-controlled levitation. Biophys J. 1990 Feb;57(2):173–182. doi: 10.1016/S0006-3495(90)82520-0. [DOI] [PMC free article] [PubMed] [Google Scholar]
- Zieliński J. J., Marszałek P., Fikus M. A new method for the investigation of cellular dielectrophoresis. Z Naturforsch C. 1989 Sep-Oct;44(9-10):845–848. doi: 10.1515/znc-1989-9-1025. [DOI] [PubMed] [Google Scholar]