Abstract
The relaxation kinetics of the gel-liquid crystalline transition of phosphatidylcholine (DC14PC, DC16PC, and DC18PC) multilamellar vesicles have been examined using volume-perturbation calorimetry. The time-dependent temperature and pressure changes associated with a periodic volume perturbation are monitored in real time. Data collected in the time domain are transformed to the frequency domain using Fourier series representations of the perturbation and response functions. Because a very small perturbation is imposed during the experiment, linear response theory is suitable for analysis of the relaxation process. The Laplace transform of the classical Kolmogorov-Avrami relation of transition kinetics is used to describe the dynamic response in the frequency domain. For DC14PC and DC16PC, the relaxation process is better fit with an effective dimensionality of n = 2 rather than n = 1. For DC18PC, we estimate that an effective dimensionality of approximately 1.5 will best fit the data. These results indicate that the gel-liquid crystalline transition of these lipid bilayers follows the classical Kolmogorov-Avrami kinetic model with an effective dimensionality greater than 1 and the assumption of simple exponential decay (n = 1) commonly used in data analysis may not always be valid for lipid transitions. Insofar as the dimensionality of the relaxation reflects the geometry of fluctuating lipid clusters, this parameter may be useful in connecting experimental thermodynamic and kinetic results with those obtained from Monte Carlo simulations.
Full text
PDF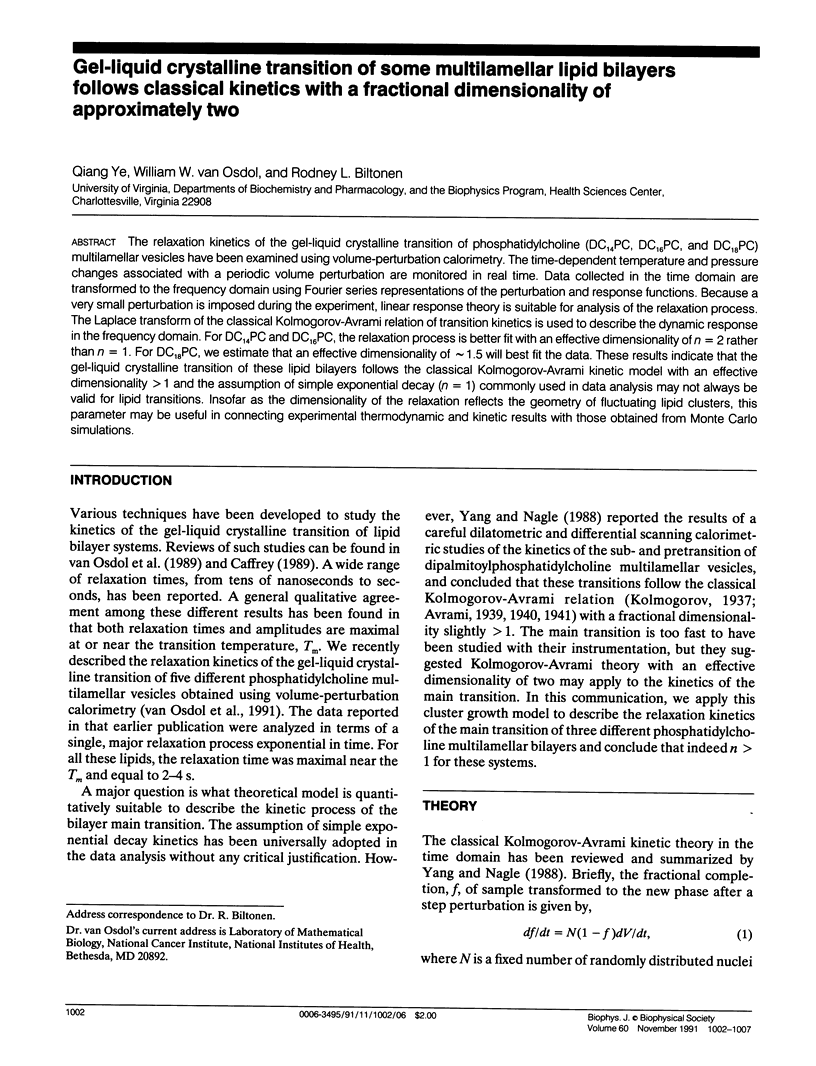
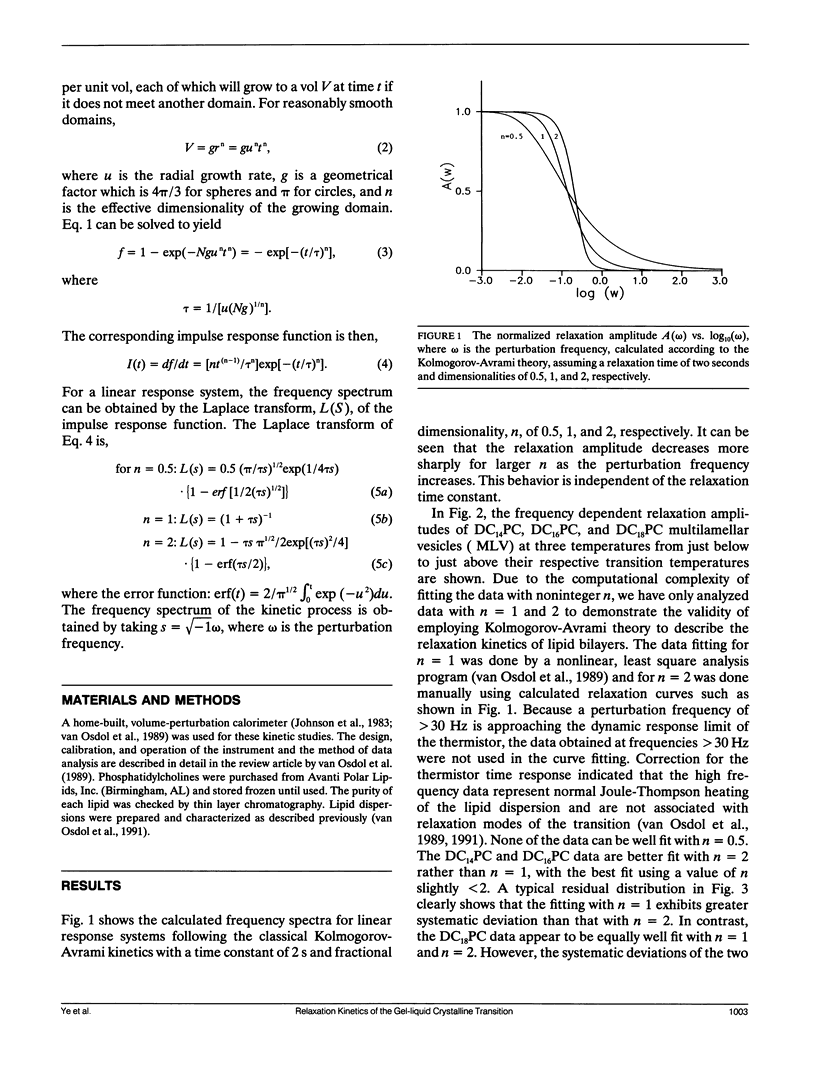
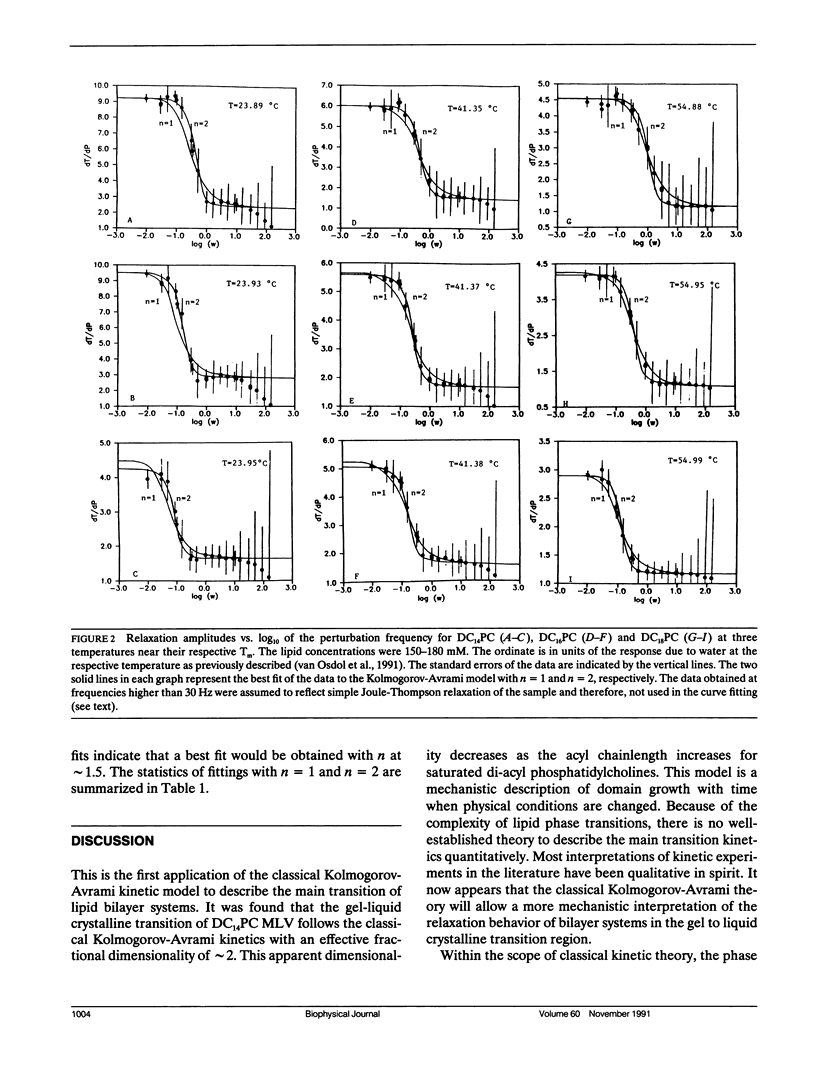
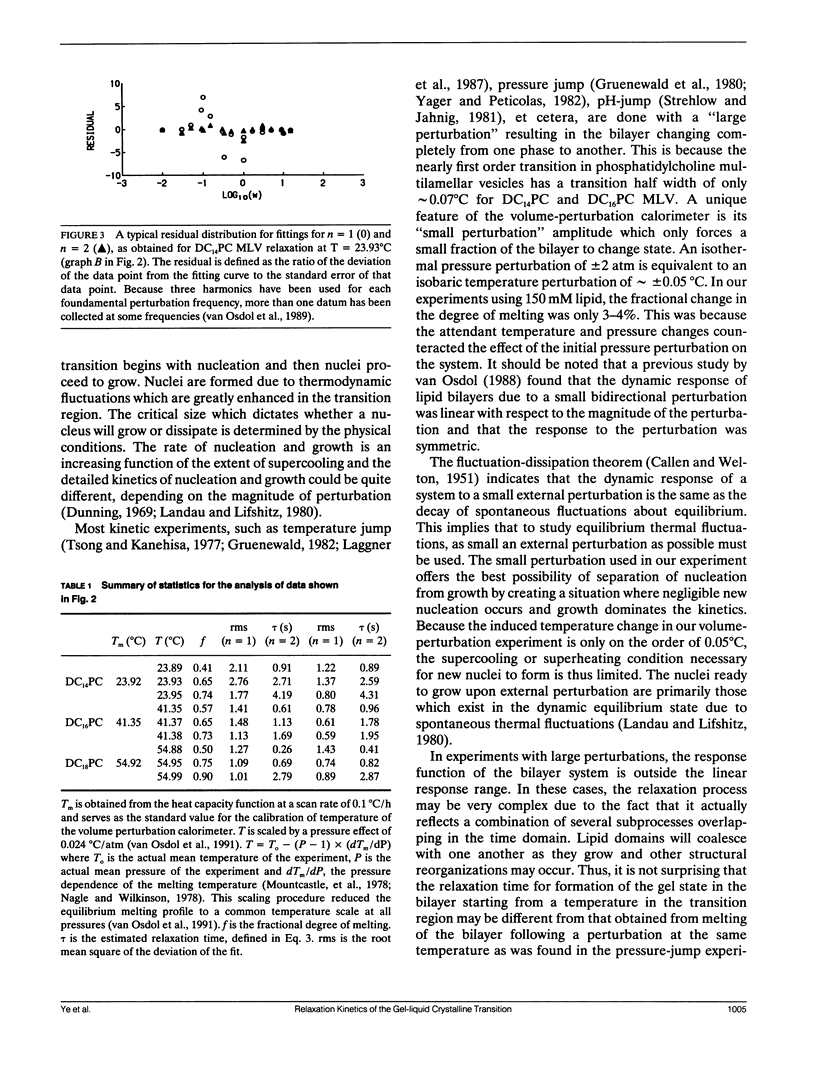
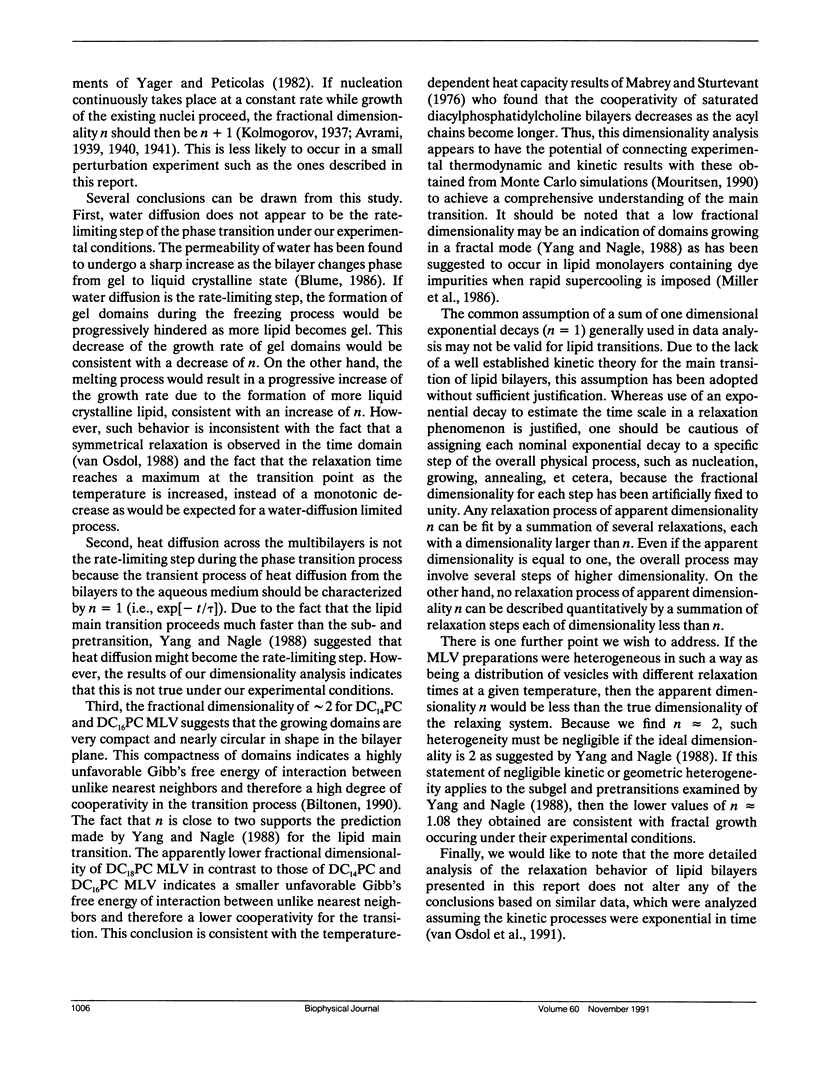
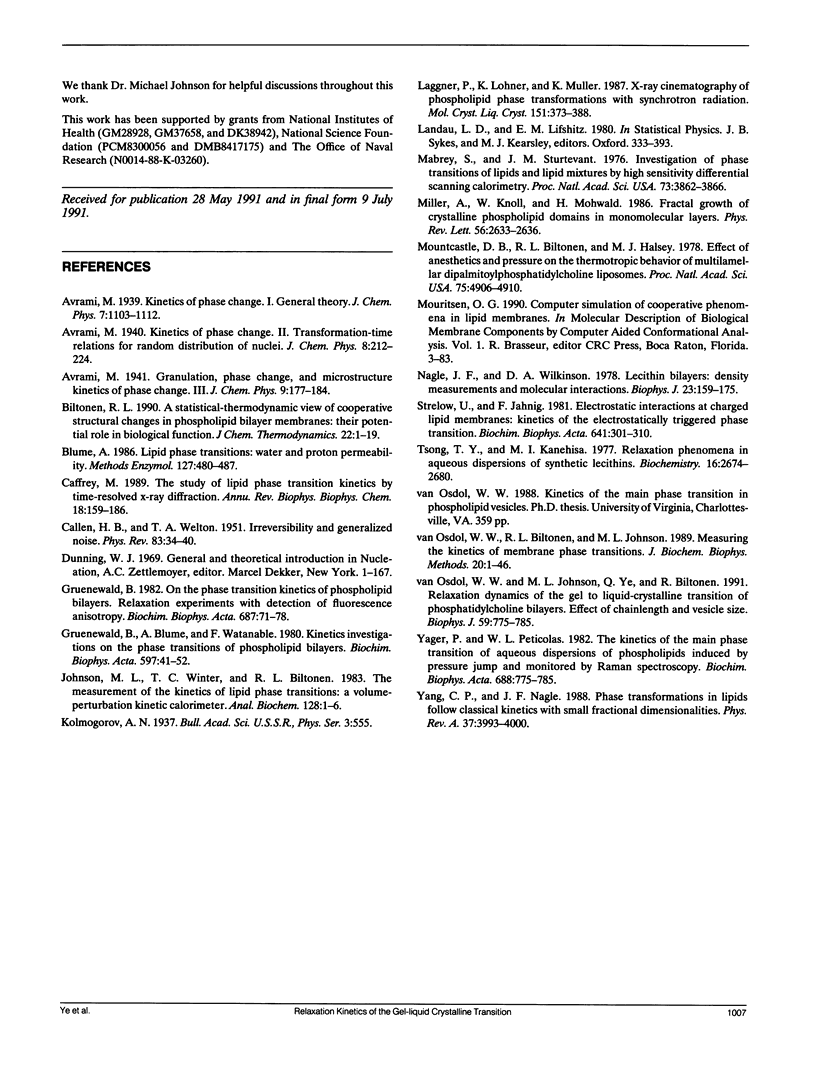
Selected References
These references are in PubMed. This may not be the complete list of references from this article.
- Blume A. Lipid phase transitions: water and proton permeability. Methods Enzymol. 1986;127:480–486. doi: 10.1016/0076-6879(86)27038-x. [DOI] [PubMed] [Google Scholar]
- Caffrey M. The study of lipid phase transition kinetics by time-resolved X-ray diffraction. Annu Rev Biophys Biophys Chem. 1989;18:159–186. doi: 10.1146/annurev.bb.18.060189.001111. [DOI] [PubMed] [Google Scholar]
- Gruenewald B., Blume A., Watanabe F. Kinetic investigations on the phase transition of phospholipid bilayers. Biochim Biophys Acta. 1980 Mar 27;597(1):41–52. doi: 10.1016/0005-2736(80)90148-0. [DOI] [PubMed] [Google Scholar]
- Gruenewald B. On the phase transition kinetics of phospholipid bilayers. Relaxation experiments with detection of fluorescent anisotropy. Biochim Biophys Acta. 1982 Apr 23;687(1):71–78. doi: 10.1016/0005-2736(82)90171-7. [DOI] [PubMed] [Google Scholar]
- Johnson M. L., Winter T. C., Biltonen R. L. The measurement of the kinetics of lipid phase transitions: a volume-perturbation kinetic calorimeter. Anal Biochem. 1983 Jan;128(1):1–6. doi: 10.1016/0003-2697(83)90335-4. [DOI] [PubMed] [Google Scholar]
- Mabrey S., Sturtevant J. M. Investigation of phase transitions of lipids and lipid mixtures by sensitivity differential scanning calorimetry. Proc Natl Acad Sci U S A. 1976 Nov;73(11):3862–3866. doi: 10.1073/pnas.73.11.3862. [DOI] [PMC free article] [PubMed] [Google Scholar]
- Miller A, Knoll W, Möhwald H. Fractal growth of crystalline phospholipid domains in monomolecular layers. Phys Rev Lett. 1986 Jun 16;56(24):2633–2636. doi: 10.1103/PhysRevLett.56.2633. [DOI] [PubMed] [Google Scholar]
- Mountcastle D. B., Biltonen R. L., Halsey M. J. Effect of anesthetics and pressure on the thermotropic behavior of multilamellar dipalmitoylphosphatidylcholine liposomes. Proc Natl Acad Sci U S A. 1978 Oct;75(10):4906–4910. doi: 10.1073/pnas.75.10.4906. [DOI] [PMC free article] [PubMed] [Google Scholar]
- Nagle J. F., Wilkinson D. A. Lecithin bilayers. Density measurement and molecular interactions. Biophys J. 1978 Aug;23(2):159–175. doi: 10.1016/S0006-3495(78)85441-1. [DOI] [PMC free article] [PubMed] [Google Scholar]
- Strehlow U., Jähnig F. Electrostatic interactions at charged lipid membranes. Kinetics of the electrostatically triggered phase transition. Biochim Biophys Acta. 1981 Mar 6;641(2):301–310. doi: 10.1016/0005-2736(81)90487-9. [DOI] [PubMed] [Google Scholar]
- Tsong T. Y., Kanehisa M. I. Relaxation phenomena in aqueous dispersions of synthetic lecithins. Biochemistry. 1977 Jun 14;16(12):2674–2680. doi: 10.1021/bi00631a014. [DOI] [PubMed] [Google Scholar]
- Van Osdol W. W., Biltonen R. L., Johnson M. L. Measuring the kinetics of membrane phase transitions. J Biochem Biophys Methods. 1989;20(1):1–46. doi: 10.1016/0165-022x(89)90079-1. [DOI] [PubMed] [Google Scholar]
- Yager P., Peticolas W. L. The kinetics of the main phase transition of aqueous dispersions of phospholipids induced by pressure jump and monitored by Raman spectroscopy. Biochim Biophys Acta. 1982 Jun 28;688(3):775–785. doi: 10.1016/0005-2736(82)90291-7. [DOI] [PubMed] [Google Scholar]
- Yang CP, Nagle JF. Phase transformations in lipids follow classical kinetics with small fractional dimensionalities. Phys Rev A Gen Phys. 1988 May 15;37(10):3993–4000. doi: 10.1103/physreva.37.3993. [DOI] [PubMed] [Google Scholar]
- van Osdol W. W., Johnson M. L., Ye Q., Biltonen R. L. Relaxation dynamics of the gel to liquid-crystalline transition of phosphatidylcholine bilayers. Effects of chainlength and vesicle size. Biophys J. 1991 Apr;59(4):775–785. doi: 10.1016/S0006-3495(91)82290-1. [DOI] [PMC free article] [PubMed] [Google Scholar]