Abstract
Multiple successive geometrical inhomogeneities, such as extensive arborization and terminal varicosities, are usual characteristics of axons. Near such regions the velocity of the action potential (AP) changes. This study uses AXONTREE, a modeling tool developed in the companion paper for two purposes: (a) to gain insights into the consequence of these irregularities for the propagation delay along axons, and (b) to simulate the propagation of APs along a reconstructed axon from a cortical cell, taking into account information concerning the distribution of boutons (release sites) along such axons to estimate the distribution of arrival times of APs to the axons release sites. We used Hodgkin and Huxley (1952) like membrane properties at 20 degrees C. Focusing on the propagation delay which results from geometrical changes along the axon (and not from the actual diameters or length of the axon), the main results are: (a) the propagation delay at a region of a single geometrical change (a step change in axon diameter or a branch point) is in the order of a few tenths of a millisecond. This delay critically depends on the kinetics and the density of the excitable channels; (b) as a general rule, the lag imposed on the AP propagation at a region with a geometrical ratio GR greater than 1 is larger than the lead obtained at a region with a reciprocal of that GR value; (c) when the electronic distance between two successive geometrical changes (Xdis) is small, the delay is not the sum of the individual delays at each geometrical change, when isolated. When both geometrical changes are with GR greater than 1 or both with GR less than 1, this delay is supralinear (larger than the sum of individual delays). The two other combinations yield a sublinear delay; and (d) in a varicose axon, where the diameter changes frequently from thin to thick and back to thin, the propagation velocity may be slower than the velocity along a uniform axon with the thin diameter. Finally, we computed propagation delays along a morphologically characterized axon from layer V of the somatosensory cortex of the cat. This axon projects mainly to area 4 but also sends collaterals to areas 3b and 3a. The model predicts that, for this axon, areas 3a, 3b, and the proximal part of area 4 are activated approximately 2 ms before the activation of the distal part of area 4.
Full text
PDF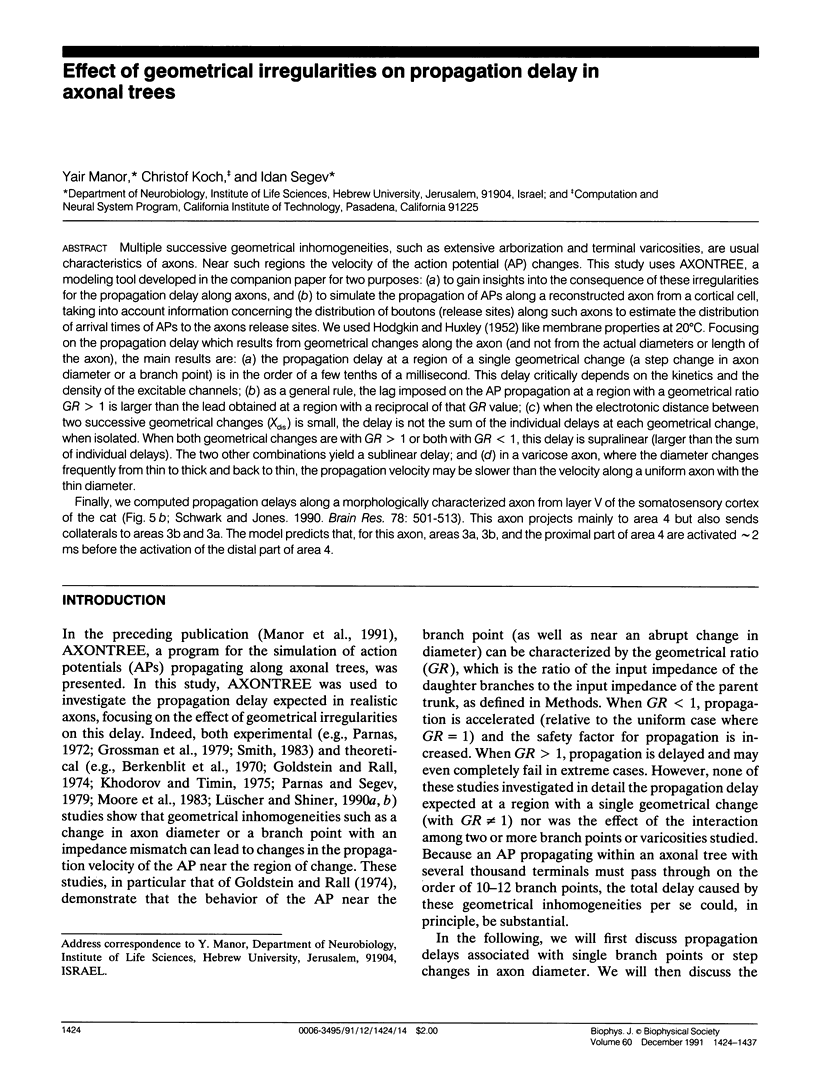
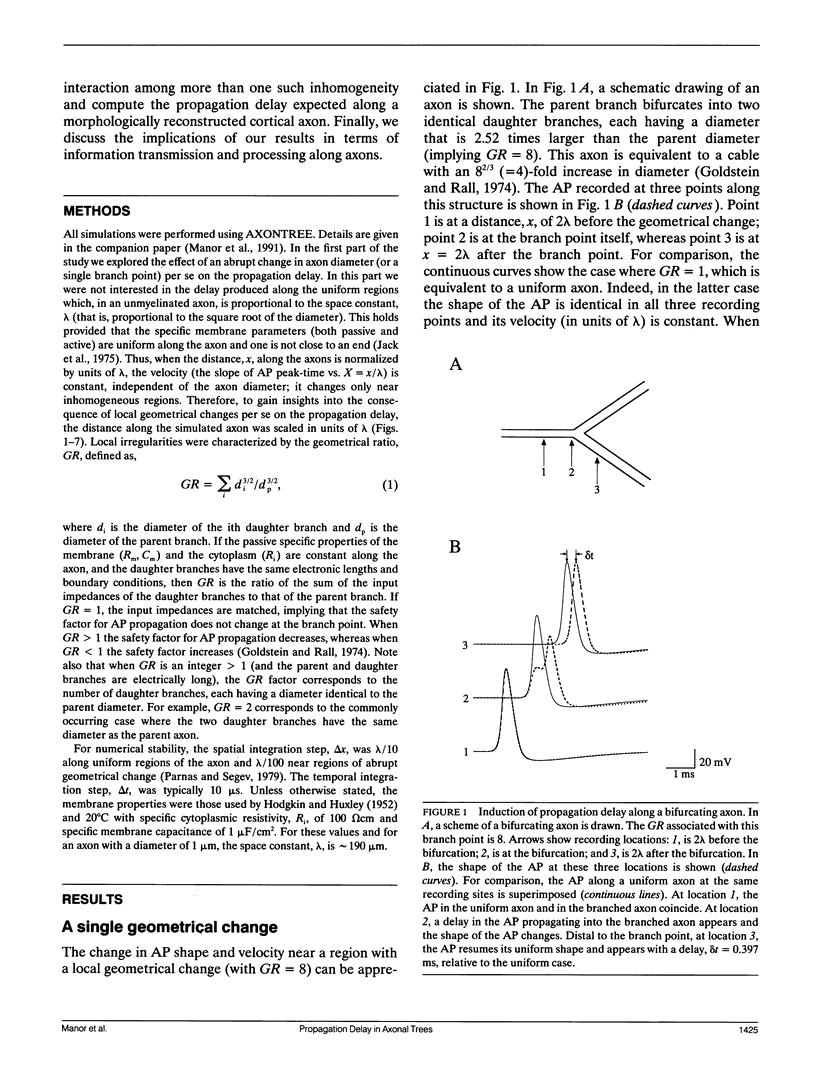
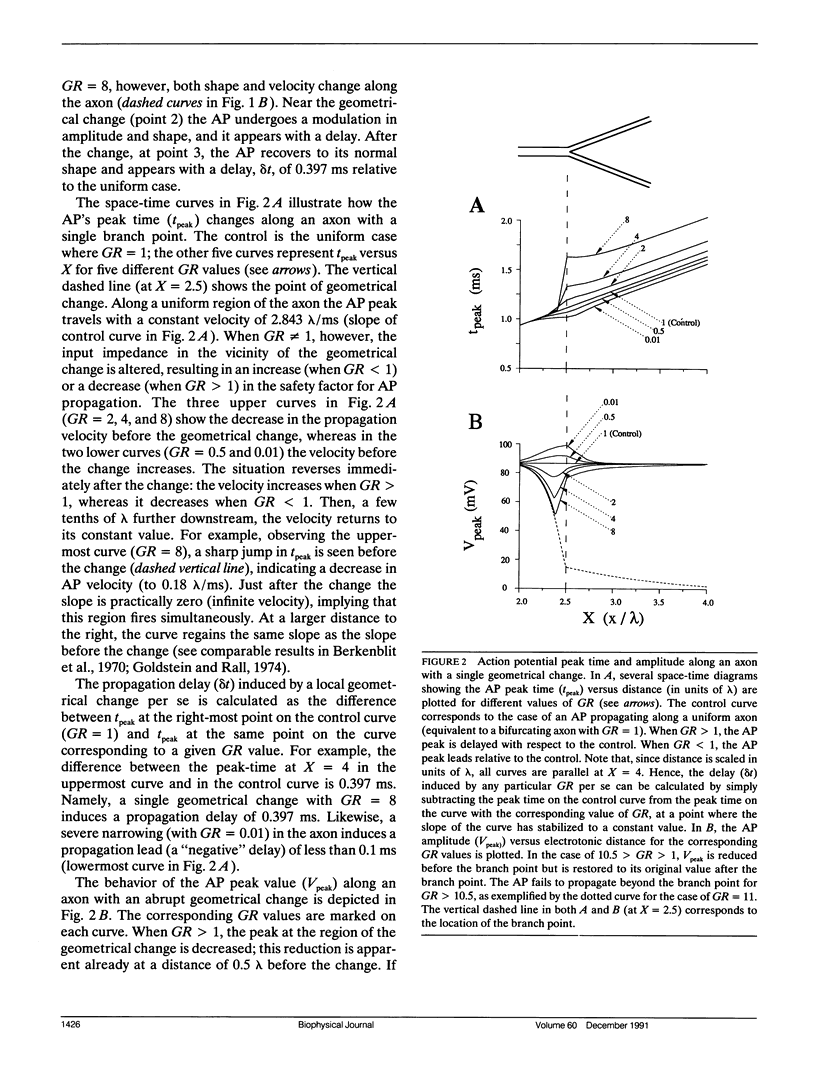
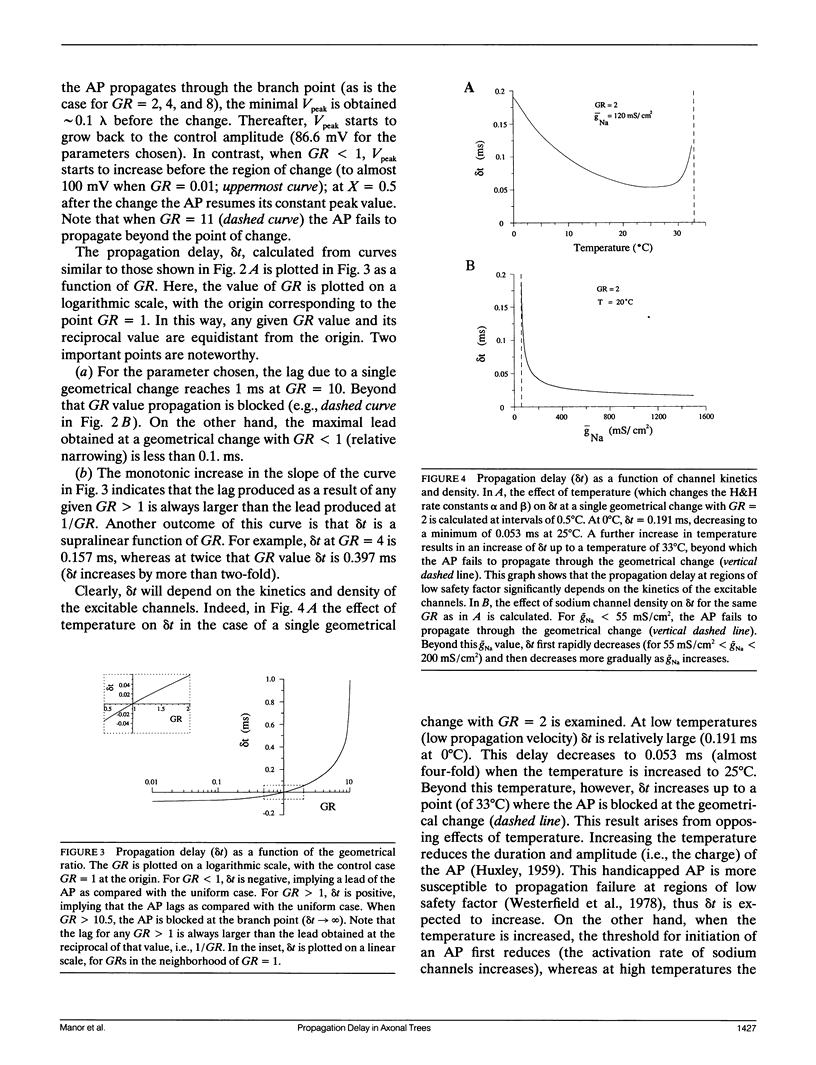
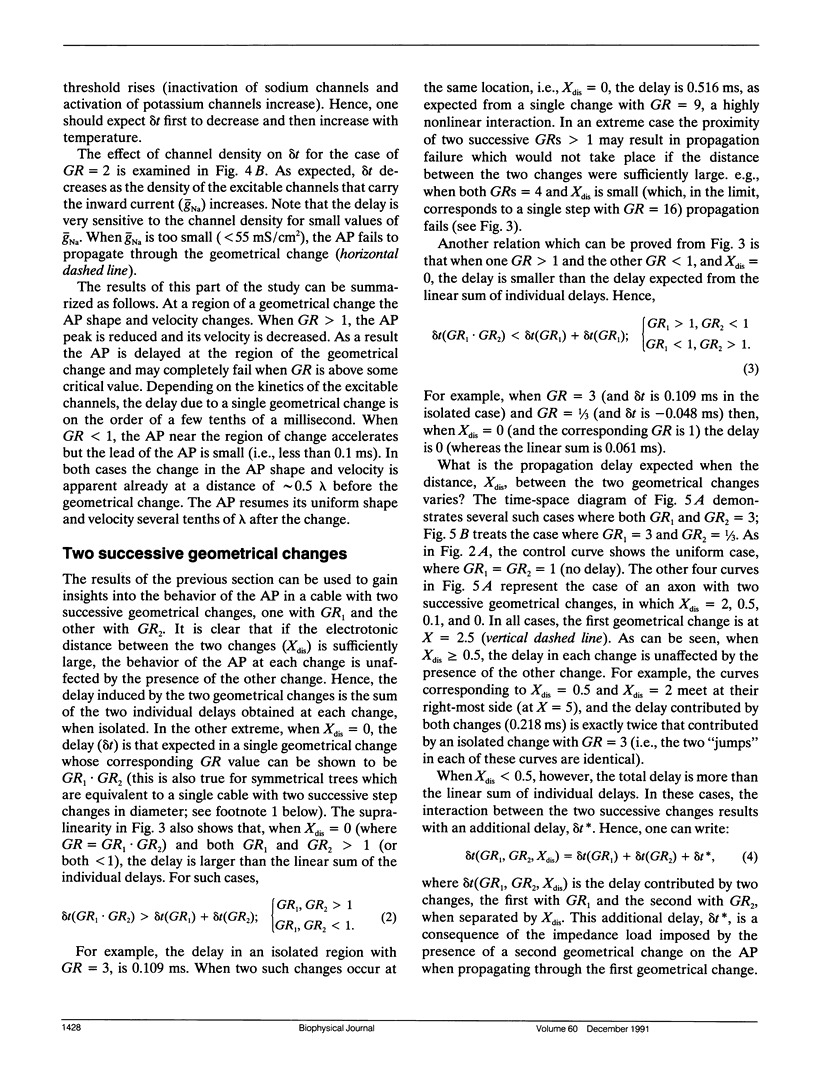
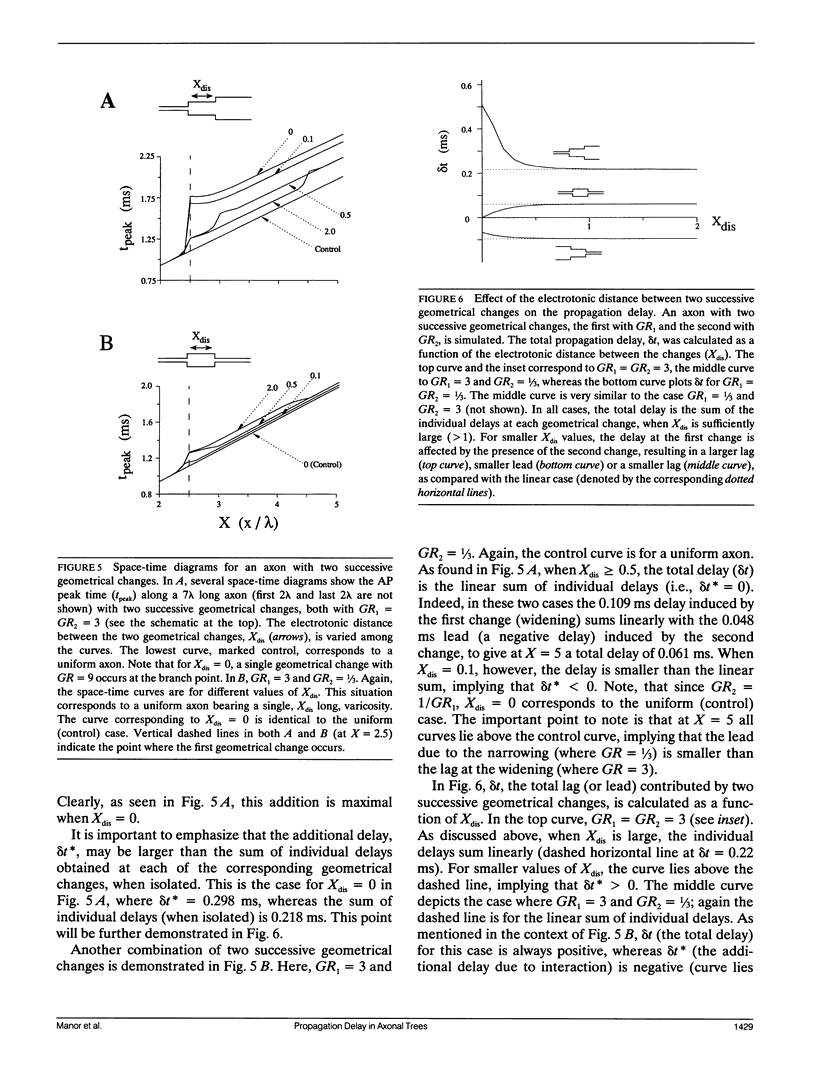
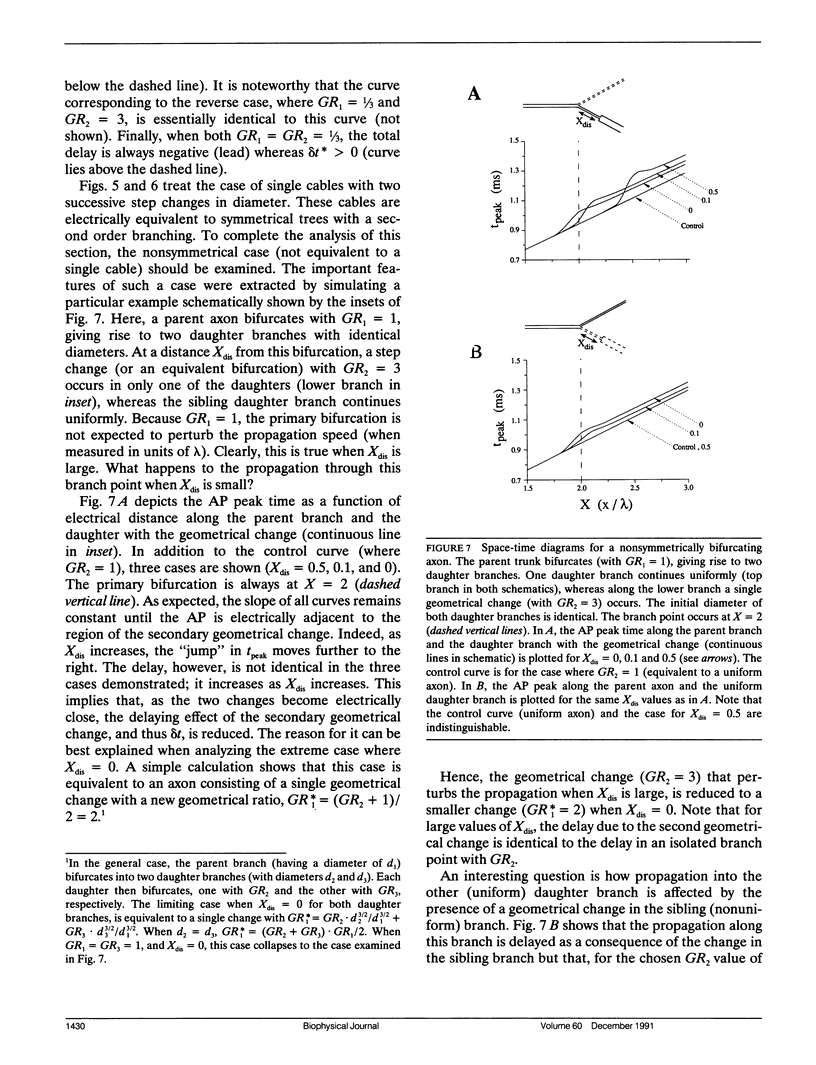
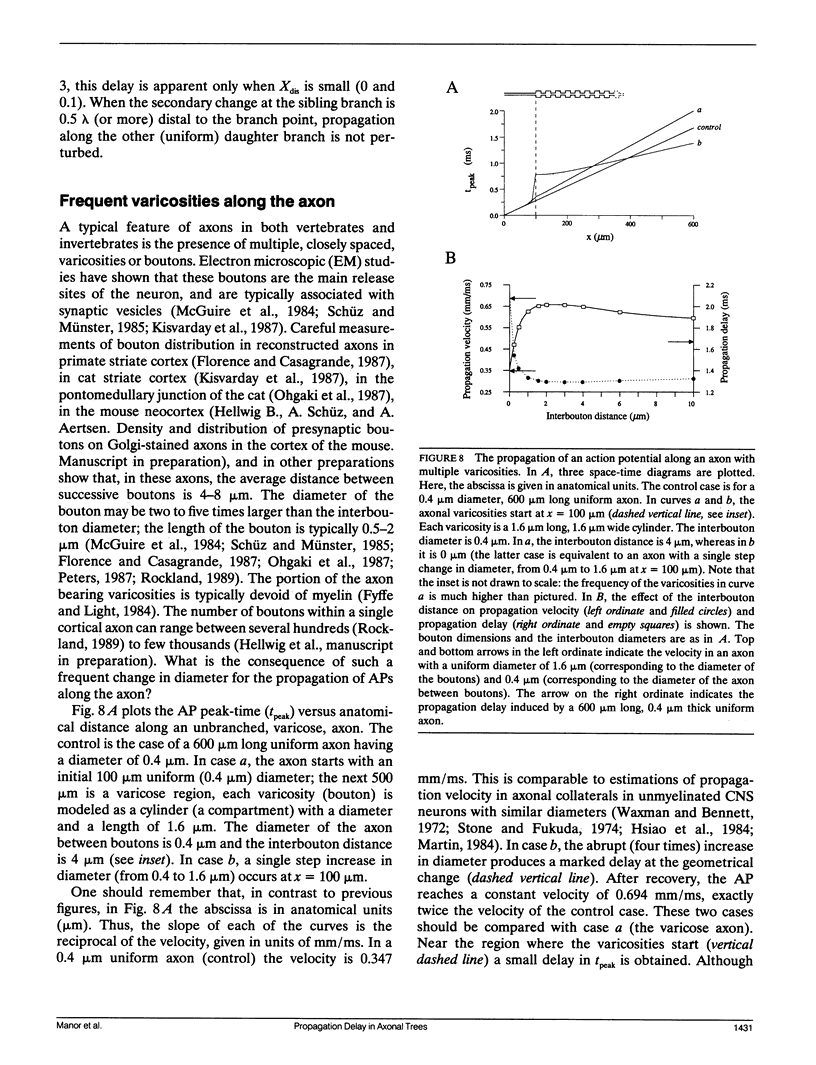
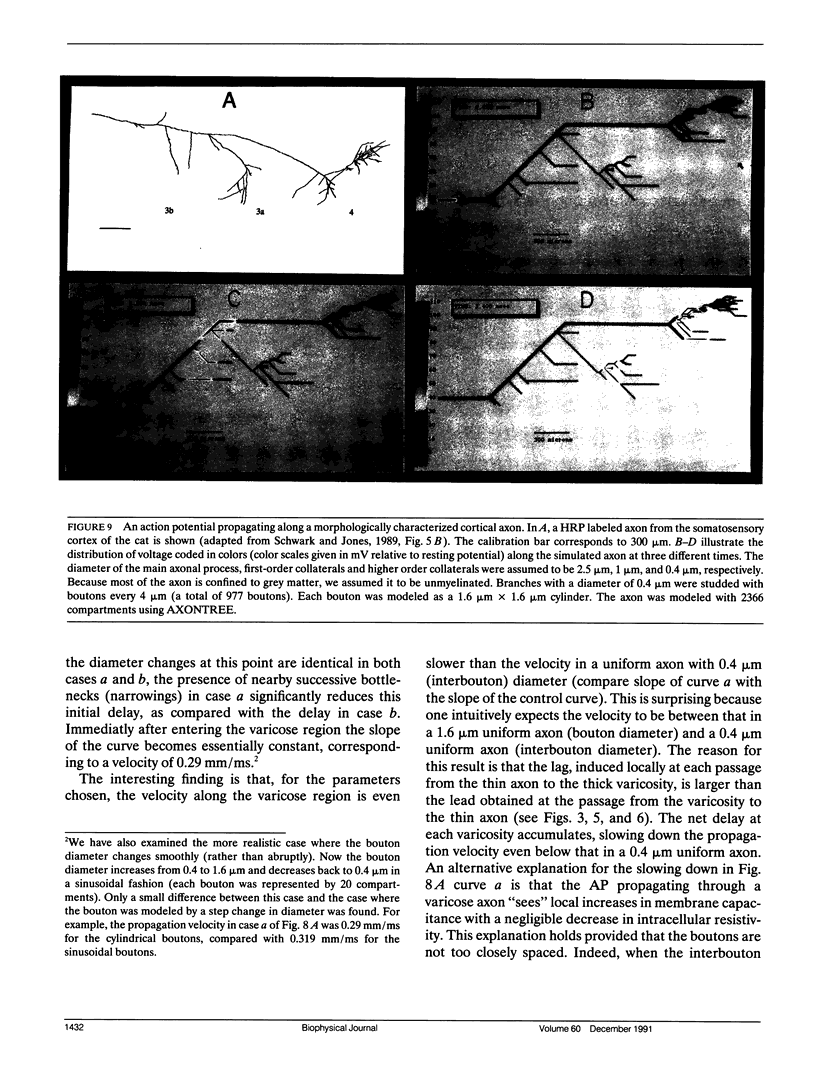
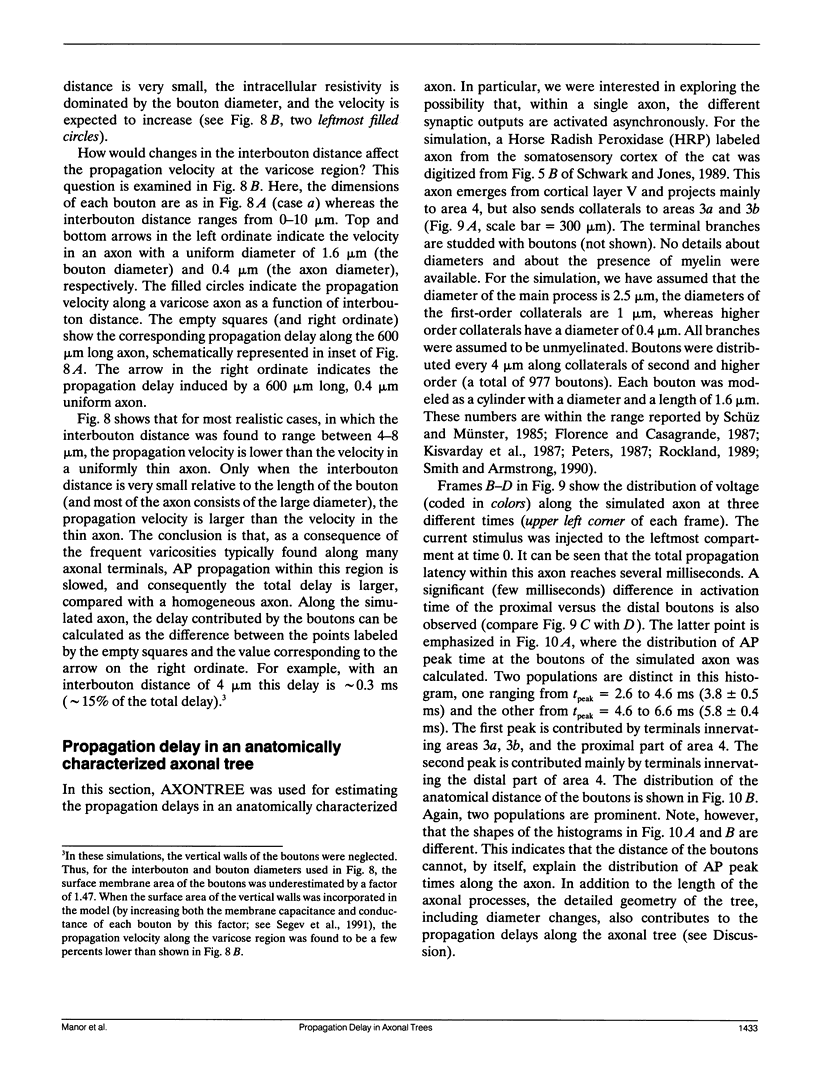
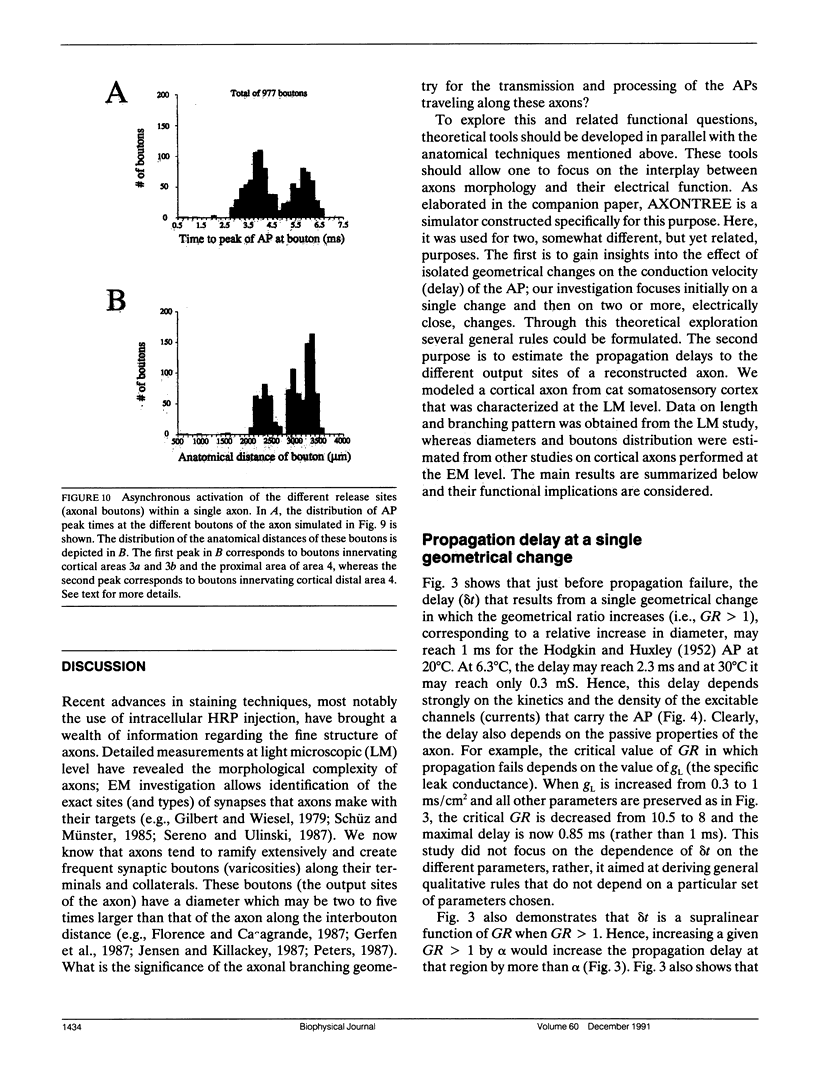
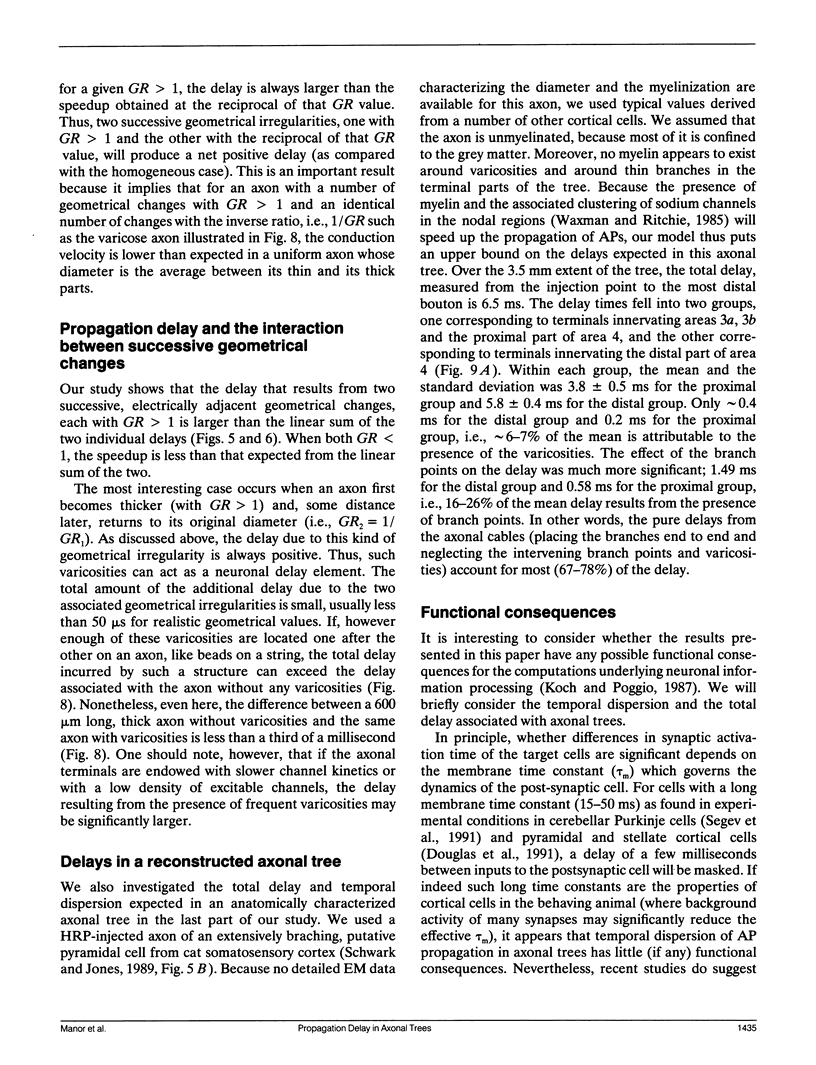
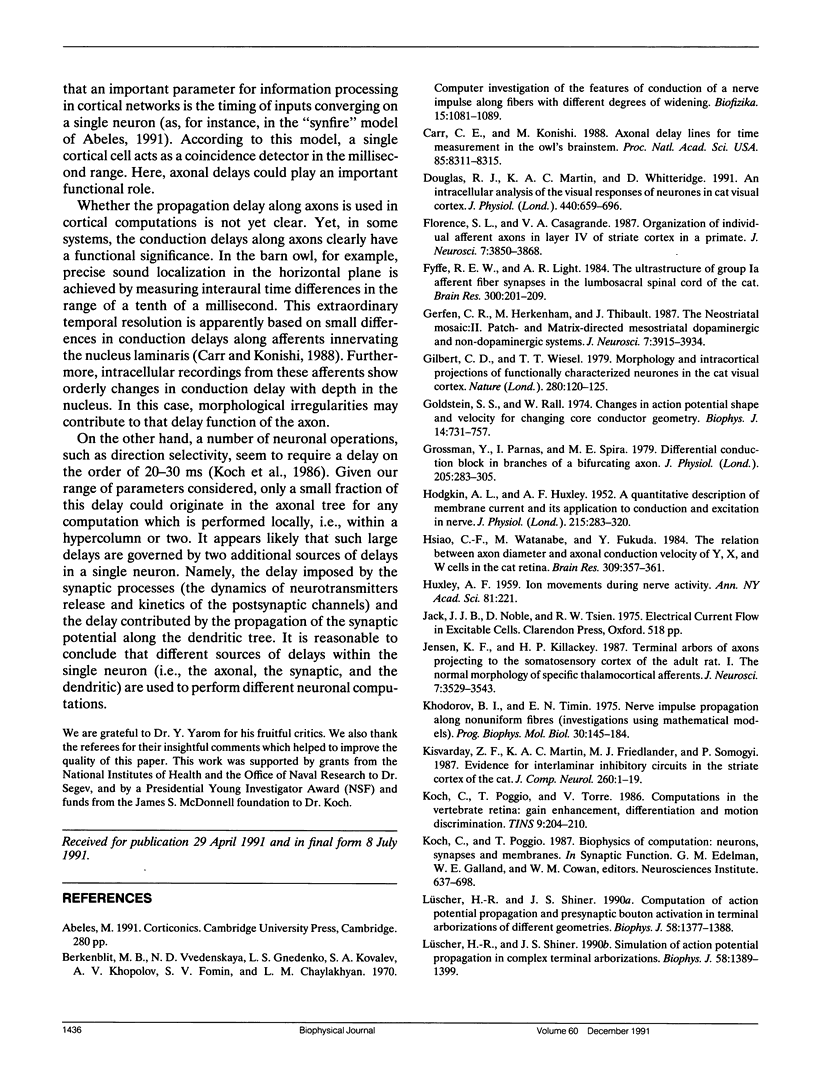
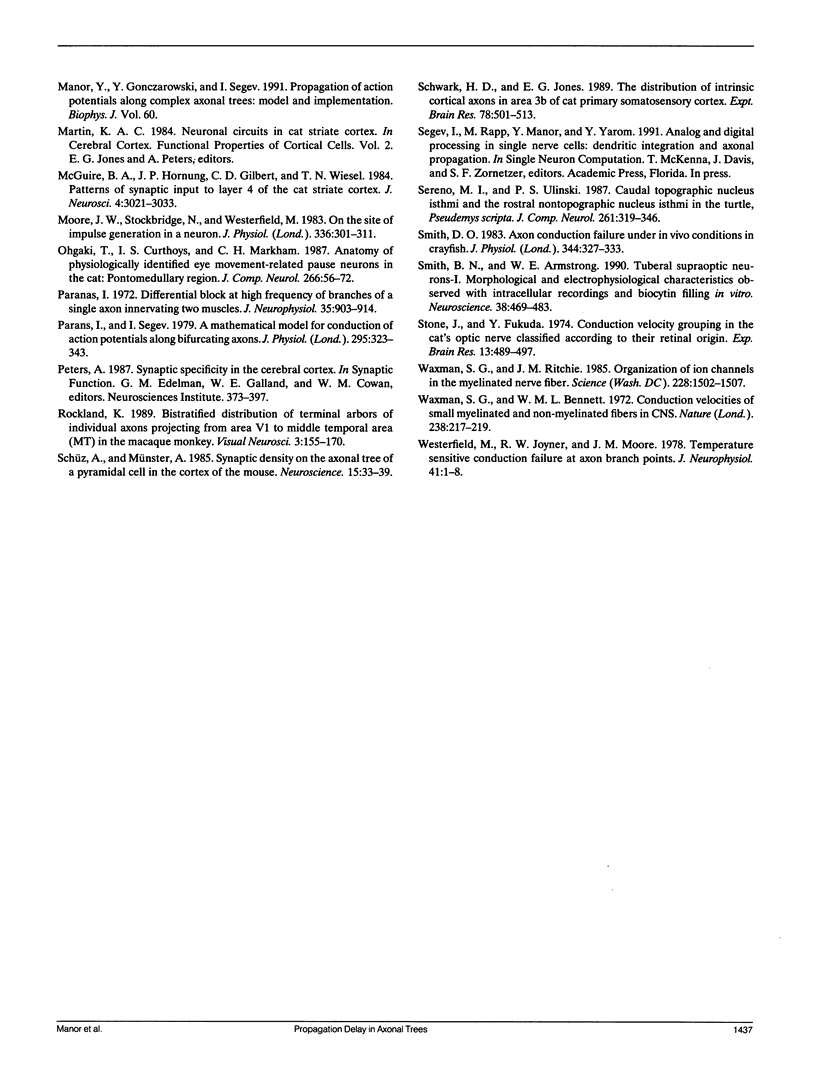
Images in this article
Selected References
These references are in PubMed. This may not be the complete list of references from this article.
- Berkinblit M. B., Vvedenskaia N. D., Gnedenko L. S., Kovalev S. A., Kholopov A. V. Issledovanie na EVM osobennostei provedeniia nervnogo impul'sa po voloknam s raznoi krutiznoi rasshireniia. Biofizika. 1970 Nov-Dec;15(6):1081–1089. [PubMed] [Google Scholar]
- Carr C. E., Konishi M. Axonal delay lines for time measurement in the owl's brainstem. Proc Natl Acad Sci U S A. 1988 Nov;85(21):8311–8315. doi: 10.1073/pnas.85.21.8311. [DOI] [PMC free article] [PubMed] [Google Scholar]
- Douglas R. J., Martin K. A., Whitteridge D. An intracellular analysis of the visual responses of neurones in cat visual cortex. J Physiol. 1991;440:659–696. doi: 10.1113/jphysiol.1991.sp018730. [DOI] [PMC free article] [PubMed] [Google Scholar]
- Florence S. L., Casagrande V. A. Organization of individual afferent axons in layer IV of striate cortex in a primate. J Neurosci. 1987 Dec;7(12):3850–3868. doi: 10.1523/JNEUROSCI.07-12-03850.1987. [DOI] [PMC free article] [PubMed] [Google Scholar]
- Fyffe R. E., Light A. R. The ultrastructure of group Ia afferent fiber synapses in the lumbosacral spinal cord of the cat. Brain Res. 1984 May 23;300(2):201–209. doi: 10.1016/0006-8993(84)90831-x. [DOI] [PubMed] [Google Scholar]
- Gerfen C. R., Herkenham M., Thibault J. The neostriatal mosaic: II. Patch- and matrix-directed mesostriatal dopaminergic and non-dopaminergic systems. J Neurosci. 1987 Dec;7(12):3915–3934. doi: 10.1523/JNEUROSCI.07-12-03915.1987. [DOI] [PMC free article] [PubMed] [Google Scholar]
- Gilbert C. D., Wiesel T. N. Morphology and intracortical projections of functionally characterised neurones in the cat visual cortex. Nature. 1979 Jul 12;280(5718):120–125. doi: 10.1038/280120a0. [DOI] [PubMed] [Google Scholar]
- Goldstein S. S., Rall W. Changes of action potential shape and velocity for changing core conductor geometry. Biophys J. 1974 Oct;14(10):731–757. doi: 10.1016/S0006-3495(74)85947-3. [DOI] [PMC free article] [PubMed] [Google Scholar]
- Grossman Y., Parnas I., Spira M. E. Differential conduction block in branches of a bifurcating axon. J Physiol. 1979 Oct;295:283–305. doi: 10.1113/jphysiol.1979.sp012969. [DOI] [PMC free article] [PubMed] [Google Scholar]
- HUXLEY A. F. Ion movements during nerve activity. Ann N Y Acad Sci. 1959 Aug 28;81:221–246. doi: 10.1111/j.1749-6632.1959.tb49311.x. [DOI] [PubMed] [Google Scholar]
- Hsiao C. F., Watanabe M., Fukuda Y. The relation between axon diameter and axonal conduction velocity of Y, X and W cells in the cat retina. Brain Res. 1984 Sep 10;309(2):357–361. doi: 10.1016/0006-8993(84)90604-8. [DOI] [PubMed] [Google Scholar]
- Jensen K. F., Killackey H. P. Terminal arbors of axons projecting to the somatosensory cortex of the adult rat. I. The normal morphology of specific thalamocortical afferents. J Neurosci. 1987 Nov;7(11):3529–3543. doi: 10.1523/JNEUROSCI.07-11-03529.1987. [DOI] [PMC free article] [PubMed] [Google Scholar]
- Khodorov B. I., Timin E. N. Nerve impulse propagation along nonuniform fibres. Prog Biophys Mol Biol. 1975;30(2-3):145–184. doi: 10.1016/0079-6107(76)90008-0. [DOI] [PubMed] [Google Scholar]
- Kisvarday Z. F., Martin K. A., Friedlander M. J., Somogyi P. Evidence for interlaminar inhibitory circuits in the striate cortex of the cat. J Comp Neurol. 1987 Jun 1;260(1):1–19. doi: 10.1002/cne.902600102. [DOI] [PubMed] [Google Scholar]
- Lüscher H. R., Shiner J. S. Computation of action potential propagation and presynaptic bouton activation in terminal arborizations of different geometries. Biophys J. 1990 Dec;58(6):1377–1388. doi: 10.1016/S0006-3495(90)82484-X. [DOI] [PMC free article] [PubMed] [Google Scholar]
- Lüscher H. R., Shiner J. S. Simulation of action potential propagation in complex terminal arborizations. Biophys J. 1990 Dec;58(6):1389–1399. doi: 10.1016/S0006-3495(90)82485-1. [DOI] [PMC free article] [PubMed] [Google Scholar]
- McGuire B. A., Hornung J. P., Gilbert C. D., Wiesel T. N. Patterns of synaptic input to layer 4 of cat striate cortex. J Neurosci. 1984 Dec;4(12):3021–3033. doi: 10.1523/JNEUROSCI.04-12-03021.1984. [DOI] [PMC free article] [PubMed] [Google Scholar]
- Moore J. W., Stockbridge N., Westerfield M. On the site of impulse initiation in a neurone. J Physiol. 1983 Mar;336:301–311. doi: 10.1113/jphysiol.1983.sp014582. [DOI] [PMC free article] [PubMed] [Google Scholar]
- Ohgaki T., Curthoys I. S., Markham C. H. Anatomy of physiologically identified eye-movement-related pause neurons in the cat: pontomedullary region. J Comp Neurol. 1987 Dec 1;266(1):56–72. doi: 10.1002/cne.902660106. [DOI] [PubMed] [Google Scholar]
- Parnas I. Differential block at high frequency of branches of a single axon innervating two muscles. J Neurophysiol. 1972 Nov;35(6):903–914. doi: 10.1152/jn.1972.35.6.903. [DOI] [PubMed] [Google Scholar]
- Parnas I., Segev I. A mathematical model for conduction of action potentials along bifurcating axons. J Physiol. 1979 Oct;295:323–343. doi: 10.1113/jphysiol.1979.sp012971. [DOI] [PMC free article] [PubMed] [Google Scholar]
- Rockland K. S. Bistratified distribution of terminal arbors of individual axons projecting from area V1 to middle temporal area (MT) in the macaque monkey. Vis Neurosci. 1989 Aug;3(2):155–170. doi: 10.1017/s0952523800004466. [DOI] [PubMed] [Google Scholar]
- Schwark H. D., Jones E. G. The distribution of intrinsic cortical axons in area 3b of cat primary somatosensory cortex. Exp Brain Res. 1989;78(3):501–513. doi: 10.1007/BF00230238. [DOI] [PubMed] [Google Scholar]
- Schüz A., Münster A. Synaptic density on the axonal tree of a pyramidal cell in the cortex of the mouse. Neuroscience. 1985 May;15(1):33–39. doi: 10.1016/0306-4522(85)90120-4. [DOI] [PubMed] [Google Scholar]
- Sereno M. I., Ulinski P. S. Caudal topographic nucleus isthmi and the rostral nontopographic nucleus isthmi in the turtle, Pseudemys scripta. J Comp Neurol. 1987 Jul 15;261(3):319–346. doi: 10.1002/cne.902610302. [DOI] [PubMed] [Google Scholar]
- Smith B. N., Armstrong W. E. Tuberal supraoptic neurons--I. Morphological and electrophysiological characteristics observed with intracellular recording and biocytin filling in vitro. Neuroscience. 1990;38(2):469–483. doi: 10.1016/0306-4522(90)90043-4. [DOI] [PubMed] [Google Scholar]
- Stone J., Freeman R. B., Jr Conduction velocity groups in the cat's optic nerve classified according to their retinal origin. Exp Brain Res. 1971 Nov 30;13(5):489–497. doi: 10.1007/BF00234279. [DOI] [PubMed] [Google Scholar]
- Waxman S. G., Bennett M. V. Relative conduction velocities of small myelinated and non-myelinated fibres in the central nervous system. Nat New Biol. 1972 Aug 16;238(85):217–219. doi: 10.1038/newbio238217a0. [DOI] [PubMed] [Google Scholar]
- Waxman S. G., Ritchie J. M. Organization of ion channels in the myelinated nerve fiber. Science. 1985 Jun 28;228(4707):1502–1507. doi: 10.1126/science.2409596. [DOI] [PubMed] [Google Scholar]
- Westerfield M., Joyner R. W., Moore J. W. Temperature-sensitive conduction failure at axon branch points. J Neurophysiol. 1978 Jan;41(1):1–8. doi: 10.1152/jn.1978.41.1.1. [DOI] [PubMed] [Google Scholar]