Abstract
To investigate heme-protein coupling via the Fe(2+)-N epsilon (His F8) linkage we have measured the profile of the Raman band due to the Fe(2+)-N epsilon (His F8) stretching mode (nu Fe-His) of deoxyHb-trout IV and deoxyHbA at various pH between 6.0 and 9.0. Our data establish that the band of this mode is composed of five different sublines. In deoxyHb-trout IV, three of these sublines were assigned to distinct conformations of the alpha-subunit (omega alpha 1 = 202 cm-1, omega alpha 2 = 211 cm-1, omega alpha 3 = 217 cm-1) and the other two to distinct conformations of the beta-subunit (omega beta 1 = 223 cm-1 and omega beta 2 = 228 cm-1). Human deoxyHbA exhibits two alpha-chain sublines at omega alpha 1 = 203 cm-1, omega alpha 2 = 212 cm-1 and two beta-chain sublines at omega beta 1 = 217 cm-1 and omega beta 2 = 225 cm-1. These results reveal that each subunit exists in different conformations. The intensities of the nu Fe-His sublines in deoxyHb-trout IV exhibit a significant pH dependence, whereas the intensities of the corresponding sublines in the deoxyHbA spectrum are independent on pH. This finding suggests that the structural basis of the Bohr effect is different in deoxyHbA and deoxyHb-trout IV. To analyse the pH dependence of the deoxyHb-trout IV sublines we have applied a titration model describing the intensity of each nu Fe-His subline as an incoherent superposition of the intensities from sub-sublines with the same frequency but differing intrinsic intensities due to the different protonation states of the respective subunit. The molar fractions of these protonation states are determined by the corresponding Bohr groups (i.e., pK alpha 1 = pK alpha 2 = 8.5, pK beta 1 = 7.5, pK beta 2 = 7.4) and pH. Hence, the intensities of these sublines reflect the pH dependence of the molar fractions of the involved protonation states. Fitting this model to the pH-dependent line intensities yields a good reproduction of the experimental data. To elucidate the structural basis of the observed results we have employed models proposed by Bangchoroenpaurpong, O., K. T. Schomaker, and P. M. Champion. (1984. J. Am. Chem. Soc. 106:5688-5698) and Friedman, J. M., B. F. Campbell, and R. W. Noble. (1990. Biophys. Chem. 37:43-59) which describe the coupling between the sigma *orbitals of the Fe2+-NJ(His F8) bond and the phi * orbitals of the pyrrole nitrogens in terms of the tilt angle theta between its Fe2+-N,(HisF8)-bond and the heme normal and the azimuthal angle phi between the Fe2+-N.(His F8) projection on the heme and the N1-N3 axis.Our results indicate that each subconformation reflected by different frequencies of the VFe His-subline is related to different tilt angles theta, whereas the pH-induced intensity variations of each VFe His subline of the deoxy Hb trout IV spectrum are caused by changes of the azimuthal angle phi.
Full text
PDF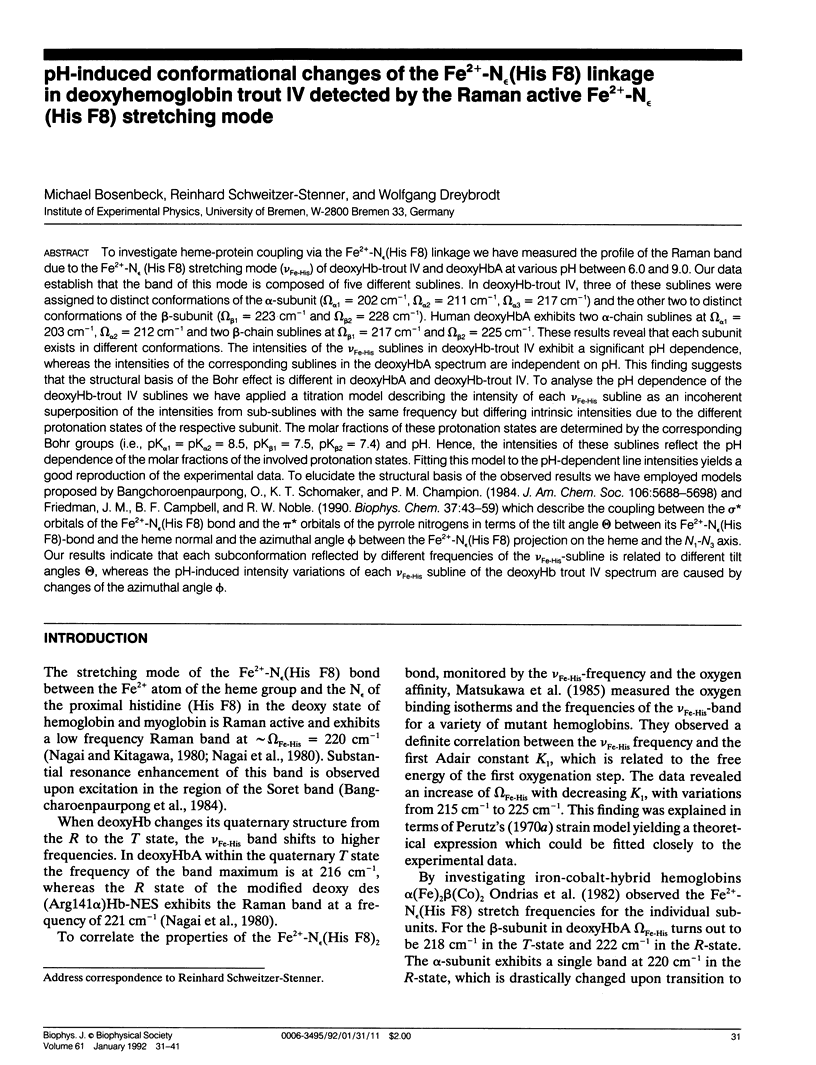
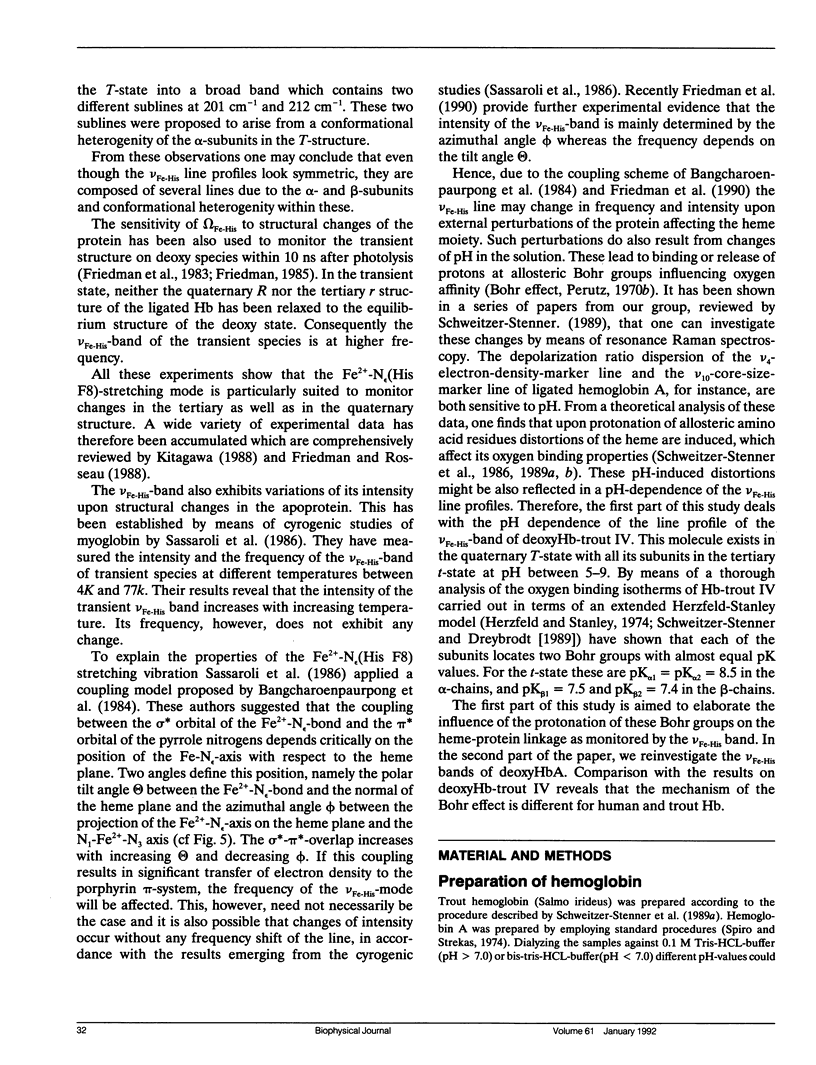
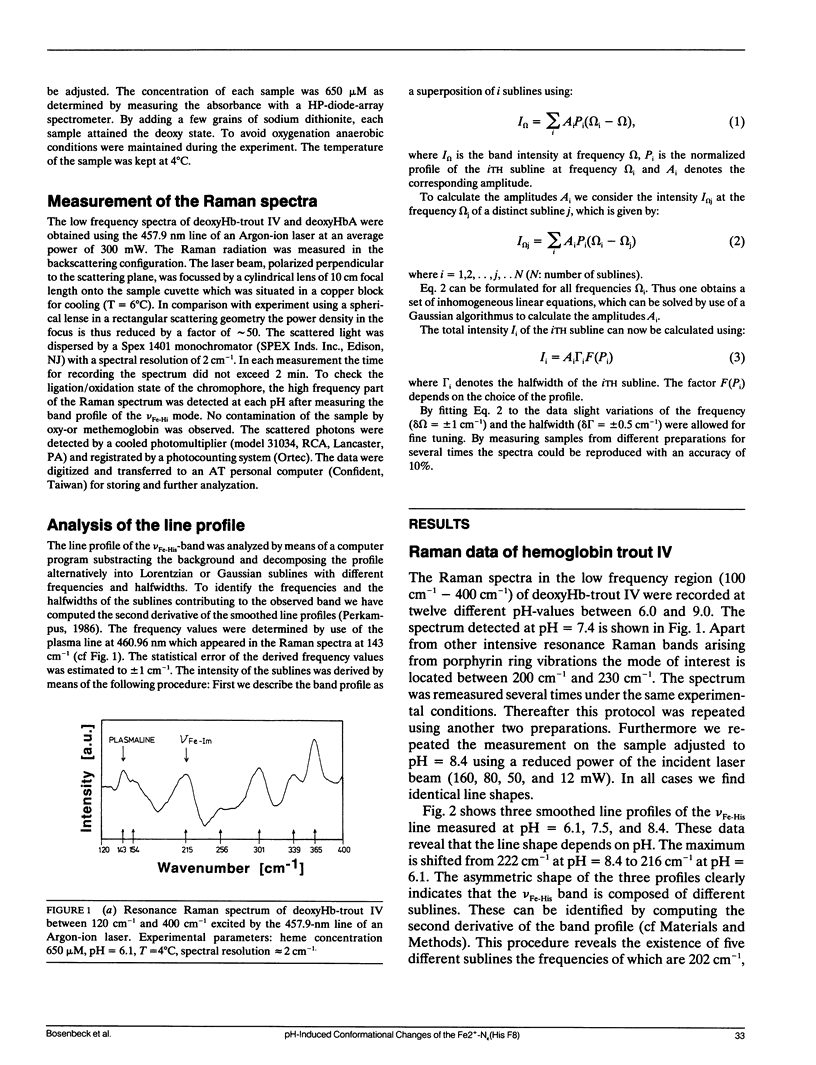
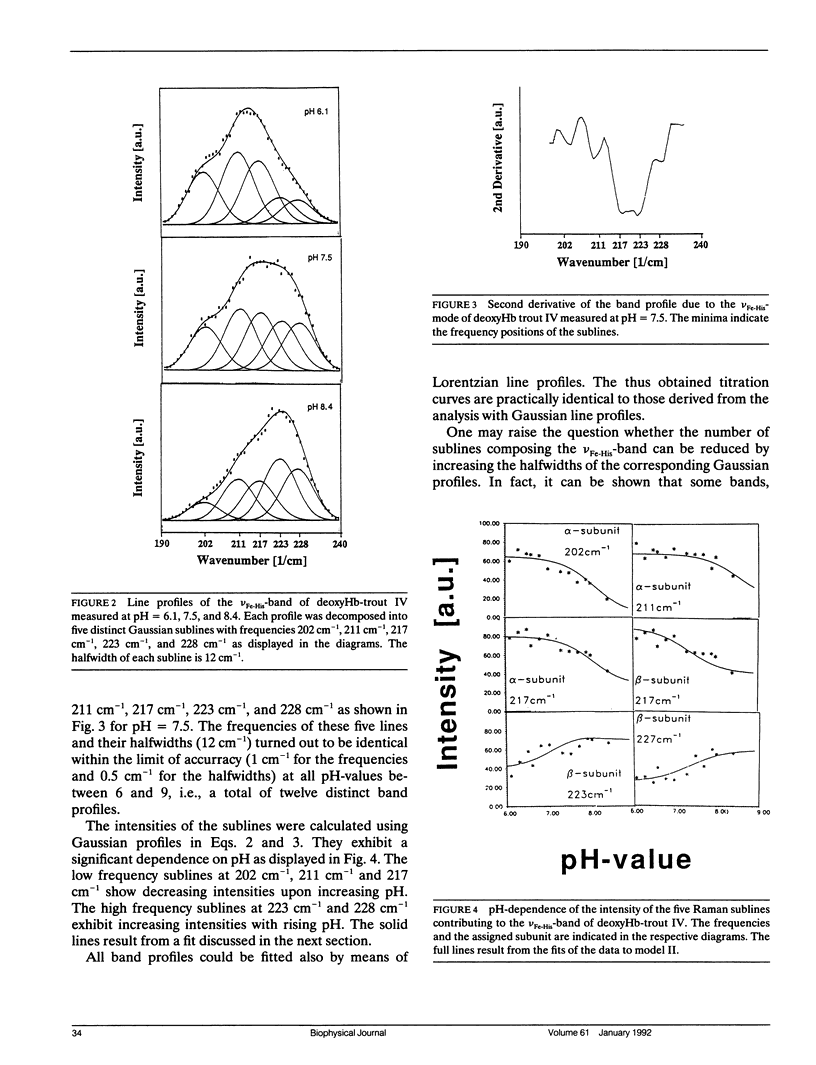
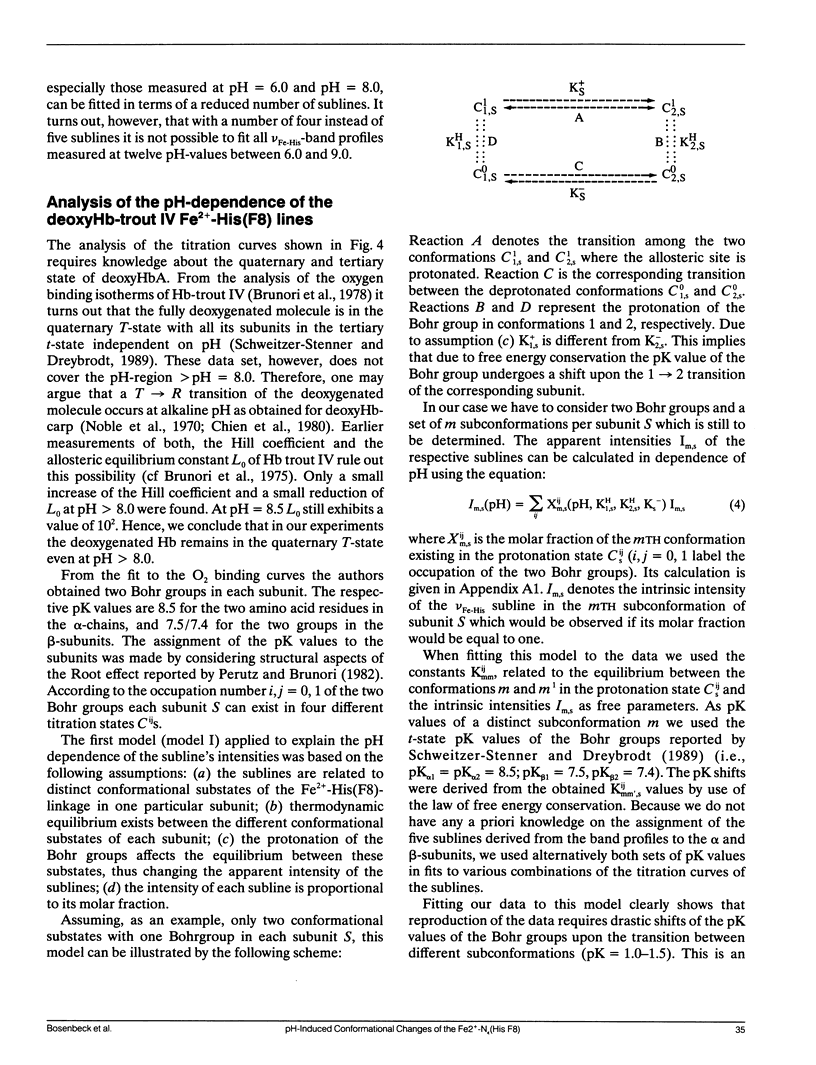
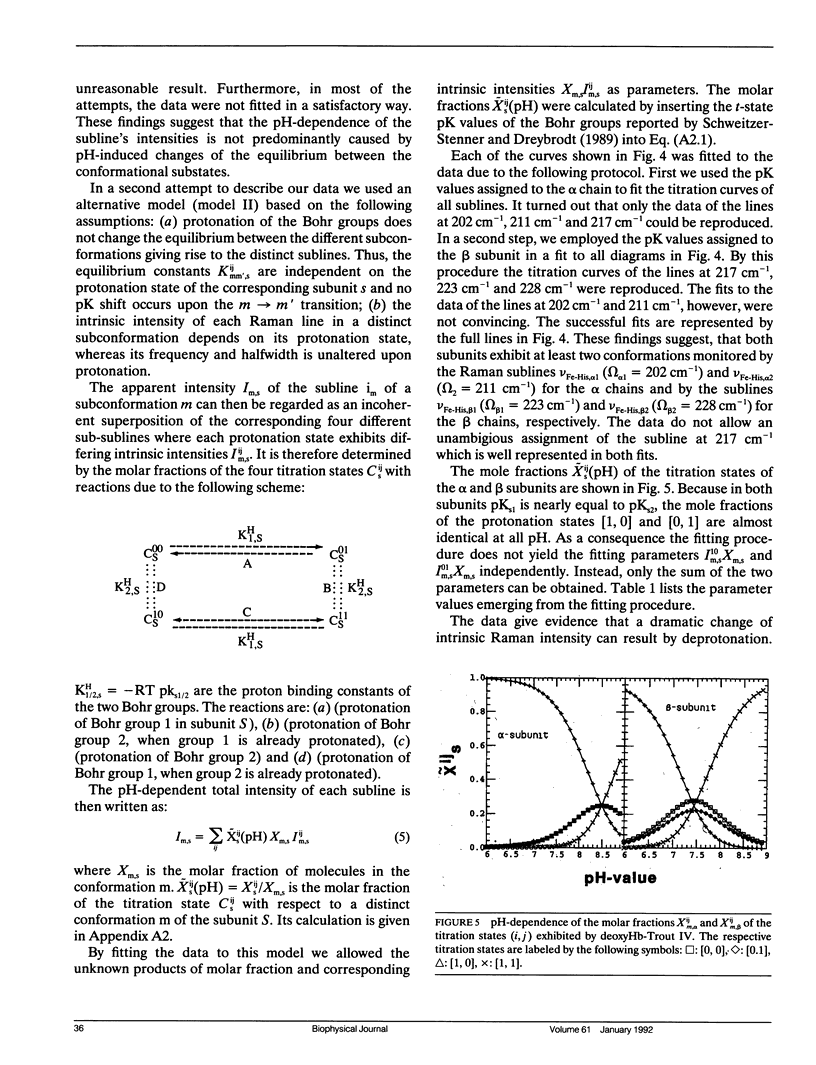
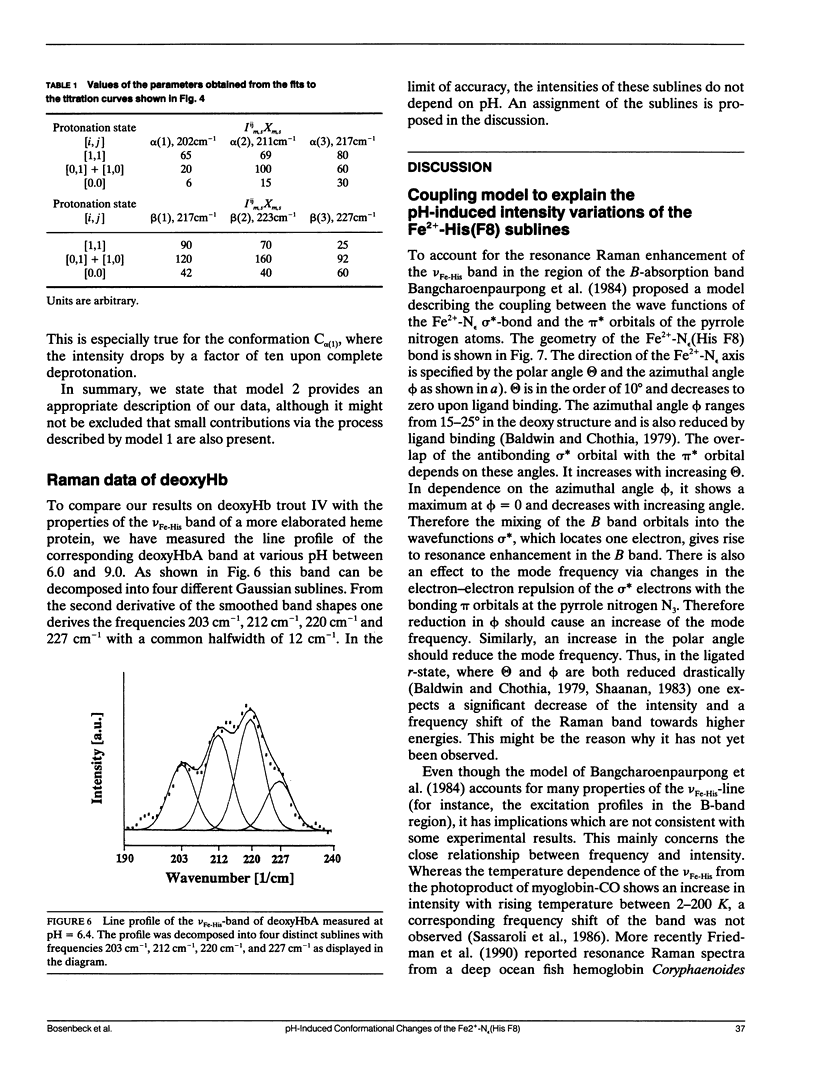
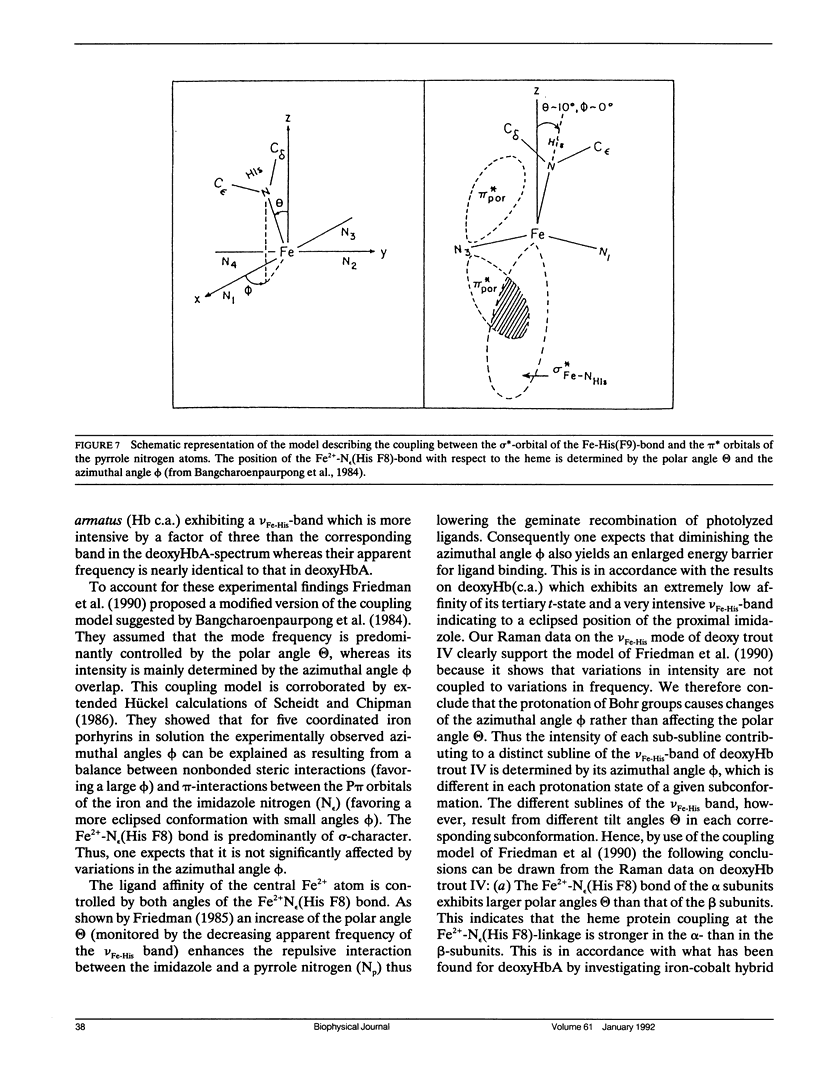
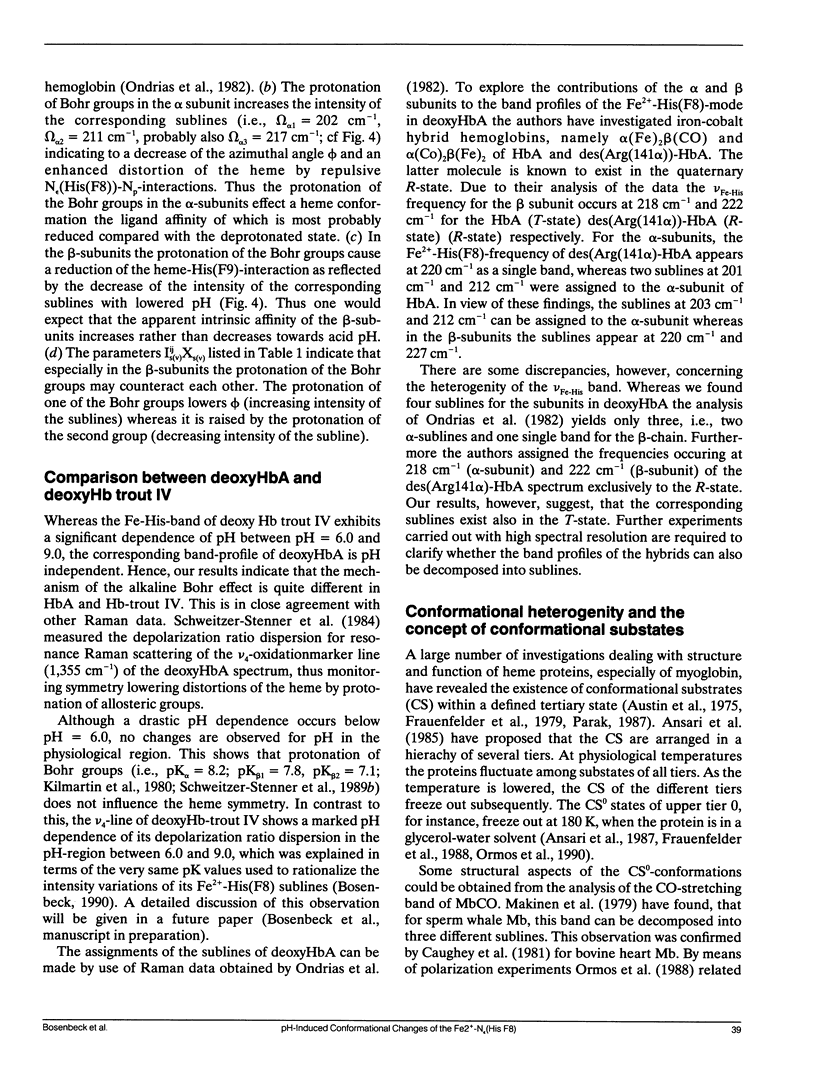
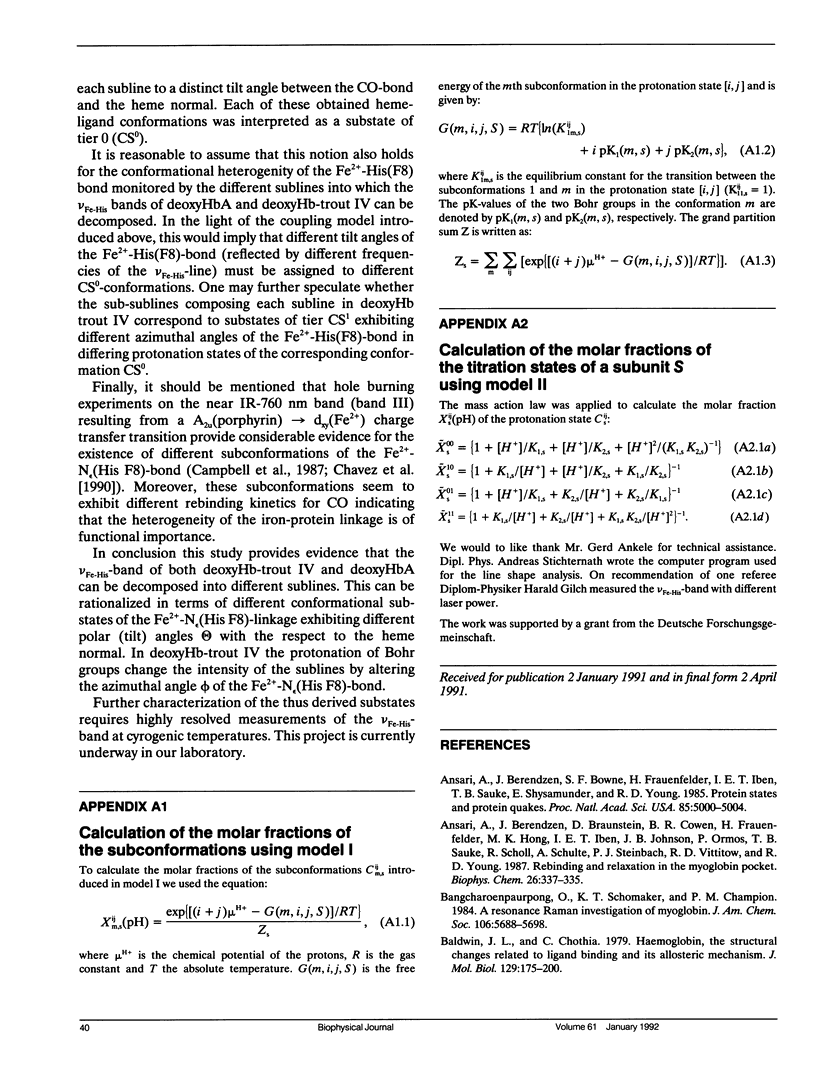
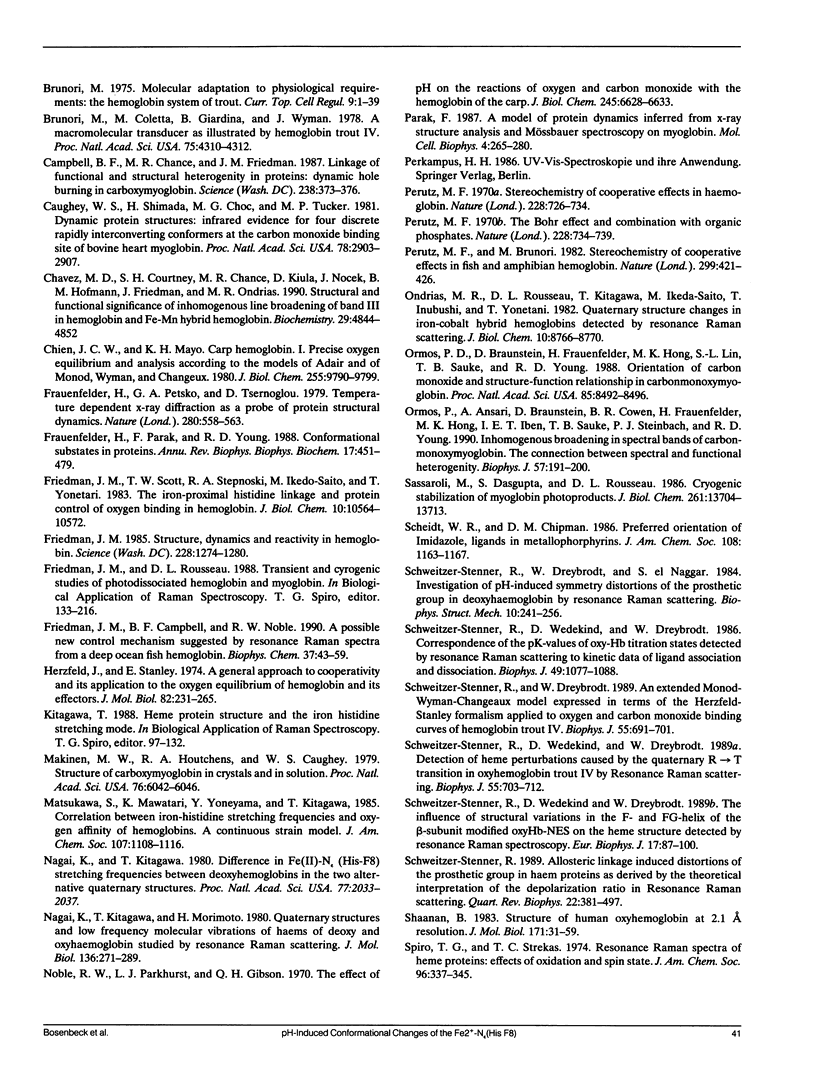
Selected References
These references are in PubMed. This may not be the complete list of references from this article.
- Ansari A., Berendzen J., Bowne S. F., Frauenfelder H., Iben I. E., Sauke T. B., Shyamsunder E., Young R. D. Protein states and proteinquakes. Proc Natl Acad Sci U S A. 1985 Aug;82(15):5000–5004. doi: 10.1073/pnas.82.15.5000. [DOI] [PMC free article] [PubMed] [Google Scholar]
- Ansari A., Berendzen J., Braunstein D., Cowen B. R., Frauenfelder H., Hong M. K., Iben I. E., Johnson J. B., Ormos P., Sauke T. B. Rebinding and relaxation in the myoglobin pocket. Biophys Chem. 1987 May 9;26(2-3):337–355. doi: 10.1016/0301-4622(87)80034-0. [DOI] [PubMed] [Google Scholar]
- Baldwin J., Chothia C. Haemoglobin: the structural changes related to ligand binding and its allosteric mechanism. J Mol Biol. 1979 Apr 5;129(2):175–220. doi: 10.1016/0022-2836(79)90277-8. [DOI] [PubMed] [Google Scholar]
- Brunori M., Coletta M., Giardina B., Wyman J. A macromolecular transducer as illustrated by trout hemoglobin IV. Proc Natl Acad Sci U S A. 1978 Sep;75(9):4310–4312. doi: 10.1073/pnas.75.9.4310. [DOI] [PMC free article] [PubMed] [Google Scholar]
- Brunori M. Molecular adaptation to physiological requirements: the hemoglobin system of trout. Curr Top Cell Regul. 1975;9:1–39. doi: 10.1016/b978-0-12-152809-6.50008-1. [DOI] [PubMed] [Google Scholar]
- Campbell B. F., Chance M. R., Friedman J. M. Linkage of functional and structural heterogeneity in proteins: dynamic hole burning in carboxymyoglobin. Science. 1987 Oct 16;238(4825):373–376. doi: 10.1126/science.3659921. [DOI] [PubMed] [Google Scholar]
- Caughey W. S., Shimada H., Choc M. G., Tucker M. P. Dynamic protein structures: infrared evidence for four discrete rapidly interconverting conformers at the carbon monoxide binding site of bovine heart myoglobin. Proc Natl Acad Sci U S A. 1981 May;78(5):2903–2907. doi: 10.1073/pnas.78.5.2903. [DOI] [PMC free article] [PubMed] [Google Scholar]
- Chavez M. D., Courtney S. H., Chance M. R., Kiula D., Nocek J., Hoffman B. M., Friedman J. M., Ondrias M. R. Structural and functional significance of inhomogeneous line broadening of band III in hemoglobin and Fe-Mn hybrid hemoglobins. Biochemistry. 1990 May 22;29(20):4844–4852. doi: 10.1021/bi00472a014. [DOI] [PubMed] [Google Scholar]
- Chien J. C., Mayo K. H. Carp hemoglobin. I. Precise oxygen equilibrium and analysis according to the models of Adair and of Monod, Wyman, and Changeux. J Biol Chem. 1980 Oct 25;255(20):9790–9799. [PubMed] [Google Scholar]
- Frauenfelder H., Parak F., Young R. D. Conformational substates in proteins. Annu Rev Biophys Biophys Chem. 1988;17:451–479. doi: 10.1146/annurev.bb.17.060188.002315. [DOI] [PubMed] [Google Scholar]
- Frauenfelder H., Petsko G. A., Tsernoglou D. Temperature-dependent X-ray diffraction as a probe of protein structural dynamics. Nature. 1979 Aug 16;280(5723):558–563. doi: 10.1038/280558a0. [DOI] [PubMed] [Google Scholar]
- Friedman J. M., Campbell B. F., Noble R. W. A possible new control mechanism suggested by resonance Raman spectra from a deep ocean fish hemoglobin. Biophys Chem. 1990 Aug 31;37(1-3):43–59. doi: 10.1016/0301-4622(90)88006-e. [DOI] [PubMed] [Google Scholar]
- Friedman J. M., Scott T. W., Stepnoski R. A., Ikeda-Saito M., Yonetani T. The iron-proximal histidine linkage and protein control of oxygen binding in hemoglobin. A transient Raman study. J Biol Chem. 1983 Sep 10;258(17):10564–10572. [PubMed] [Google Scholar]
- Friedman J. M. Structure, dynamics, and reactivity in hemoglobin. Science. 1985 Jun 14;228(4705):1273–1280. doi: 10.1126/science.4001941. [DOI] [PubMed] [Google Scholar]
- Herzfeld J., Stanley H. E. A general approach to co-operativity and its application to the oxygen equilibrium of hemoglobin and its effectors. J Mol Biol. 1974 Jan 15;82(2):231–265. doi: 10.1016/0022-2836(74)90343-x. [DOI] [PubMed] [Google Scholar]
- Makinen M. W., Houtchens R. A., Caughey W. S. Structure of carboxymyoglobin in crystals and in solution. Proc Natl Acad Sci U S A. 1979 Dec;76(12):6042–6046. doi: 10.1073/pnas.76.12.6042. [DOI] [PMC free article] [PubMed] [Google Scholar]
- Nagai K., Kitagawa T. Differences in Fe(II)-N epsilon(His-F8) stretching frequencies between deoxyhemoglobins in the two alternative quaternary structures. Proc Natl Acad Sci U S A. 1980 Apr;77(4):2033–2037. doi: 10.1073/pnas.77.4.2033. [DOI] [PMC free article] [PubMed] [Google Scholar]
- Nagai K., Kitagawa T., Morimoto H. Quaternary structures and low frequency molecular vibrations of haems of deoxy and oxyhaemoglobin studied by resonance raman scattering. J Mol Biol. 1980 Jan 25;136(3):271–289. doi: 10.1016/0022-2836(80)90374-5. [DOI] [PubMed] [Google Scholar]
- Noble R. W., Parkhurst L. J., Gibson Q. H. The effect of pH on the reactions of oxygen and carbon monoxide with the hemoglobin of the carp, Cyprinus carpio. J Biol Chem. 1970 Dec 25;245(24):6628–6633. [PubMed] [Google Scholar]
- Ondrias M. R., Rousseau D. L., Kitagawa T., Ikeda-Saito M., Inubushi T., Yonetani T. Quaternary structure changes in iron-cobalt hybrid hemoglobins detected by resonance Raman scattering. J Biol Chem. 1982 Aug 10;257(15):8766–8770. [PubMed] [Google Scholar]
- Ormos P., Ansari A., Braunstein D., Cowen B. R., Frauenfelder H., Hong M. K., Iben I. E., Sauke T. B., Steinbach P. J., Young R. D. Inhomogeneous broadening in spectral bands of carbonmonoxymyoglobin. The connection between spectral and functional heterogeneity. Biophys J. 1990 Feb;57(2):191–199. doi: 10.1016/S0006-3495(90)82522-4. [DOI] [PMC free article] [PubMed] [Google Scholar]
- Ormos P., Braunstein D., Frauenfelder H., Hong M. K., Lin S. L., Sauke T. B., Young R. D. Orientation of carbon monoxide and structure-function relationship in carbonmonoxymyoglobin. Proc Natl Acad Sci U S A. 1988 Nov;85(22):8492–8496. doi: 10.1073/pnas.85.22.8492. [DOI] [PMC free article] [PubMed] [Google Scholar]
- Perutz M. F., Brunori M. Stereochemistry of cooperative effects in fish an amphibian haemoglobins. Nature. 1982 Sep 30;299(5882):421–426. doi: 10.1038/299421a0. [DOI] [PubMed] [Google Scholar]
- Perutz M. F. Stereochemistry of cooperative effects in haemoglobin. Nature. 1970 Nov 21;228(5273):726–739. doi: 10.1038/228726a0. [DOI] [PubMed] [Google Scholar]
- Sassaroli M., Dasgupta S., Rousseau D. L. Cryogenic stabilization of myoglobin photoproducts. J Biol Chem. 1986 Oct 15;261(29):13704–13713. [PubMed] [Google Scholar]
- Schweitzer-Stenner R. Allosteric linkage-induced distortions of the prosthetic group in haem proteins as derived by the theoretical interpretation of the depolarization ratio in resonance Raman scattering. Q Rev Biophys. 1989 Nov;22(4):381–479. doi: 10.1017/s0033583500003164. [DOI] [PubMed] [Google Scholar]
- Schweitzer-Stenner R., Dreybrodt W. An extended Monod-Wyman-Changeaux-model expressed in terms of the Herzfeld-Stanley formalism applied to oxygen and carbonmonoxide binding curves of hemoglobin trout IV. Biophys J. 1989 Apr;55(4):691–701. doi: 10.1016/S0006-3495(89)82868-1. [DOI] [PMC free article] [PubMed] [Google Scholar]
- Schweitzer-Stenner R., Wedekind D., Dreybrodt W. Correspondence of the pK values of oxyHb-titration states detected by resonance Raman scattering to kinetic data of ligand dissociation and association. Biophys J. 1986 May;49(5):1077–1088. doi: 10.1016/S0006-3495(86)83736-5. [DOI] [PMC free article] [PubMed] [Google Scholar]
- Schweitzer-Stenner R., Wedekind D., Dreybrodt W. Detection of the heme perturbations caused by the quaternary R----T transition in oxyhemoglobin trout IV by resonance Raman scattering. Biophys J. 1989 Apr;55(4):703–712. doi: 10.1016/S0006-3495(89)82869-3. [DOI] [PMC free article] [PubMed] [Google Scholar]
- Schweitzer-Stenner R., Wedekind D., Dreybrodt W. The influence of structural variations in the F- and FG-helix of the beta-subunit modified oxyHb-NES on the heme structure detected by resonance Raman spectroscopy. Eur Biophys J. 1989;17(2):87–100. doi: 10.1007/BF00257106. [DOI] [PubMed] [Google Scholar]
- Shaanan B. Structure of human oxyhaemoglobin at 2.1 A resolution. J Mol Biol. 1983 Nov 25;171(1):31–59. doi: 10.1016/s0022-2836(83)80313-1. [DOI] [PubMed] [Google Scholar]
- Spiro T. G., Strekas T. C. Resonance Raman spectra of heme proteins. Effects of oxidation and spin state. J Am Chem Soc. 1974 Jan 23;96(2):338–345. doi: 10.1021/ja00809a004. [DOI] [PubMed] [Google Scholar]