Abstract
The influence of a phospholipid transmembrane redistribution on the shape of nonspherical flaccid vesicles was investigated at a fixed temperature by optical microscopy. In a first series of experiments, a transmembrane pH gradient was imposed on egg phosphatidylcholine (EPC)-egg phosphatidylglycerol (EPG) (100:1) giant vesicles. The delta pH induced an asymmetric distribution of EPG. Simultaneously, discoid vesicles were transformed into tubular or a series of connected small vesicles. The fraction of phospholipid transfer necessary for a shape change from discoid to two connected vesicles was of the order of 0.1% of the total phospholipids. Additional lipid redistribution was accompanied by a sequence of shape changes. In a second series of experiments, lyso phosphatidylcholine (L-PC) was added to, or subtracted from, the external leaflet of giant EPC vesicles. The addition of L-PC induced a change from discoid to a two-vesicle state without further evolution, suggesting that lipid transfer and lipid addition are not equivalent. L-PC depletion from the outer leaflet generated stomatocyte-like vesicles. Whenever possible, we have determined whether the giant vesicles undergoing shape changes were unilamellar or multilamellar by measuring the elastic area compressibility modulus, K, by the micropipette assay (Kwok and Evans, 1981). Shape transformations triggered by phospholipid modification of the most external bilayer were indeed influenced by the presence of other underlying membranes that played a role comparable to that of a passive cytoskeleton layer. It appears that in real cells, invaginations of the plasma membrane or budding of organelles could be triggered by a phospholipid transfer from one leaflet to the other caused, for instance, by the aminophospholipid translocase which is present in eukaryotic membranes.
Full text
PDF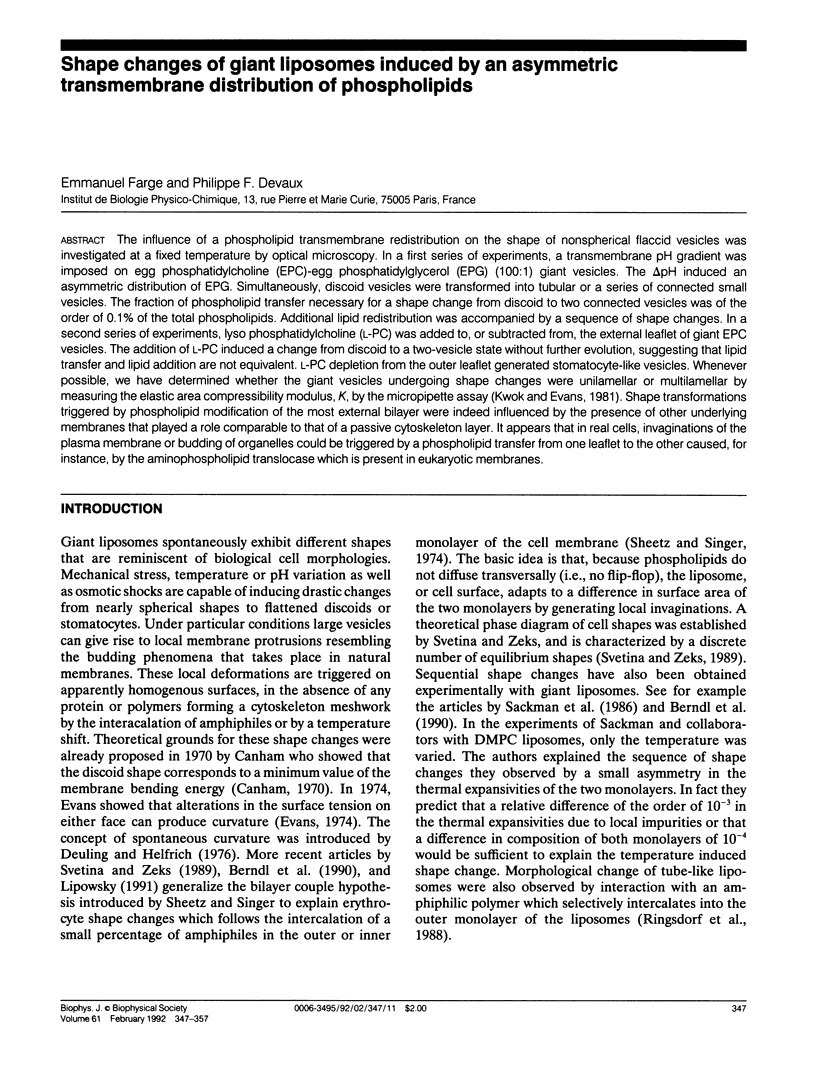
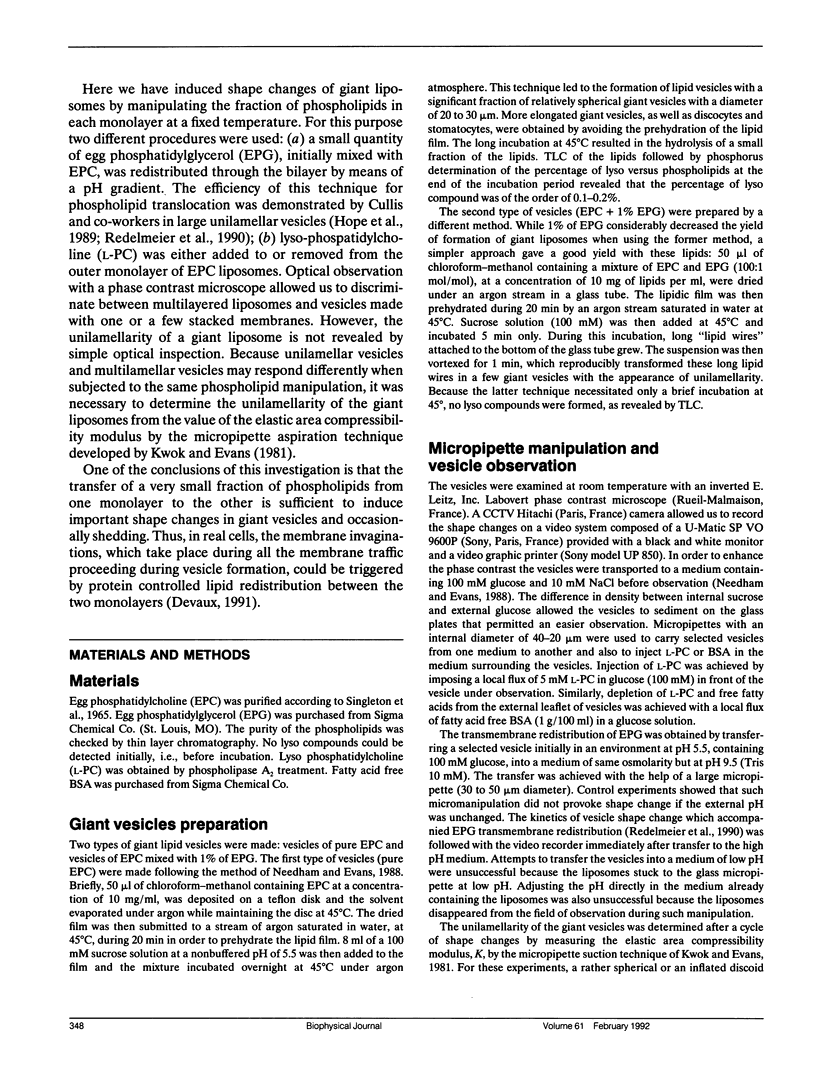
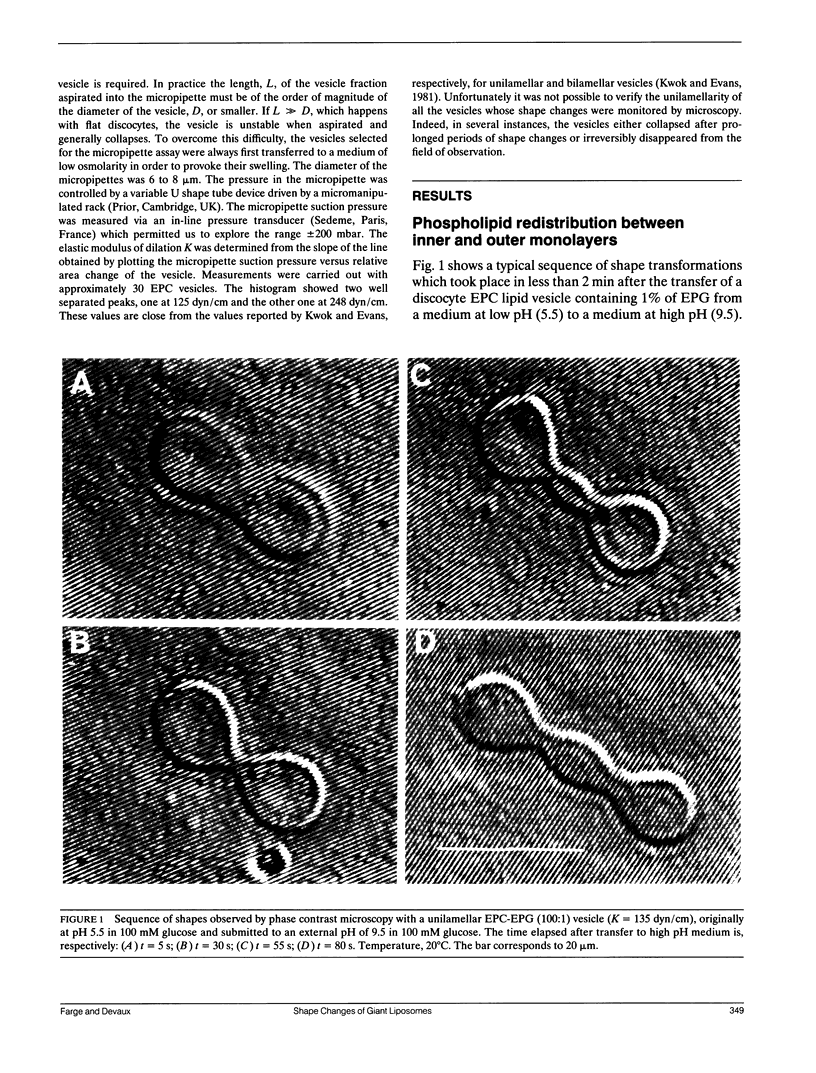
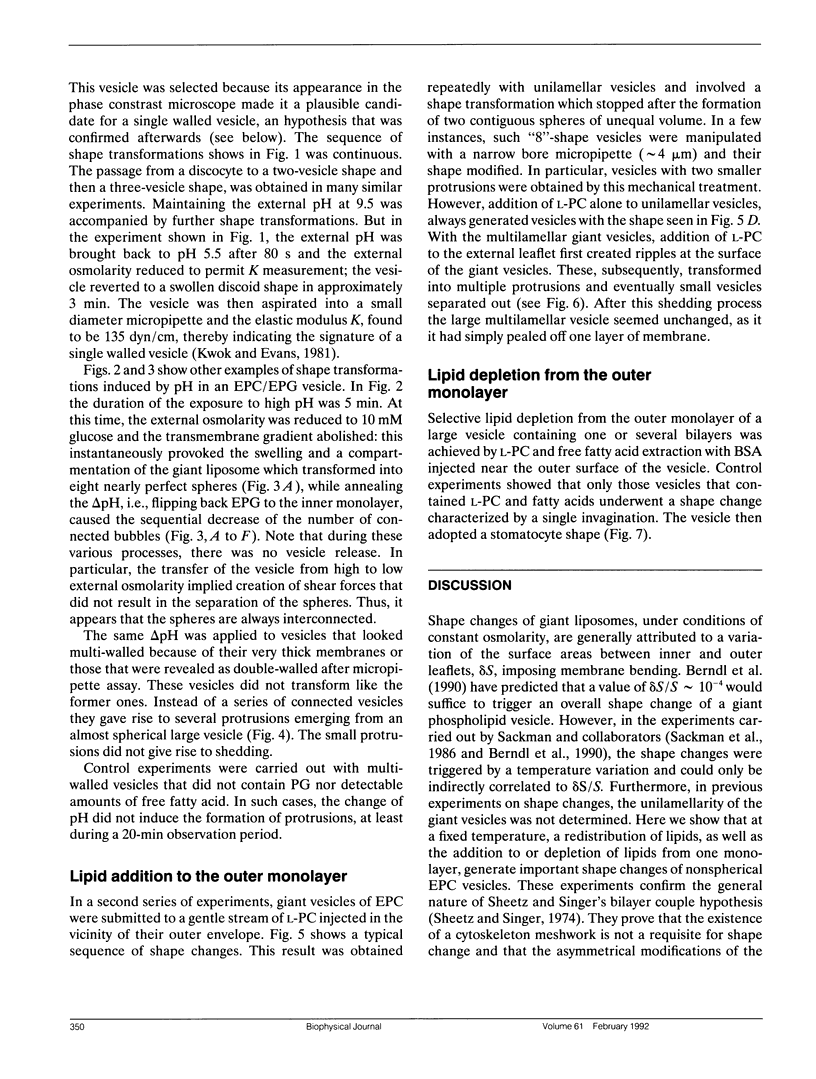
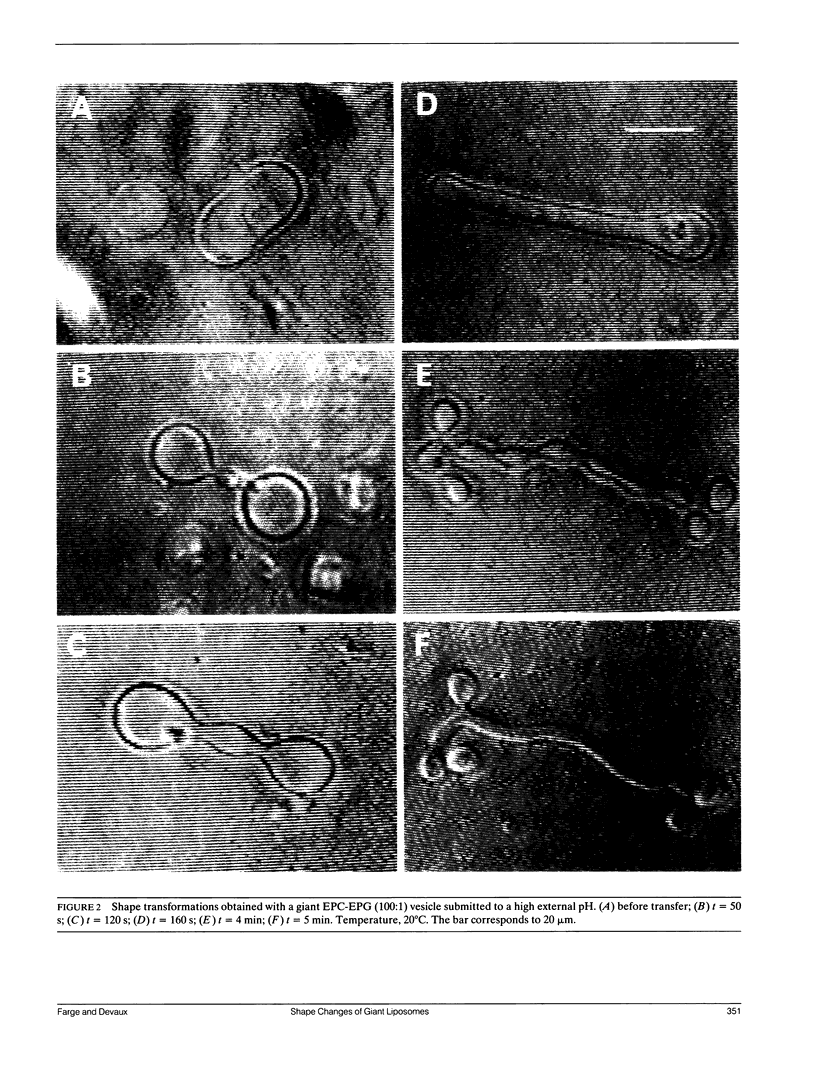
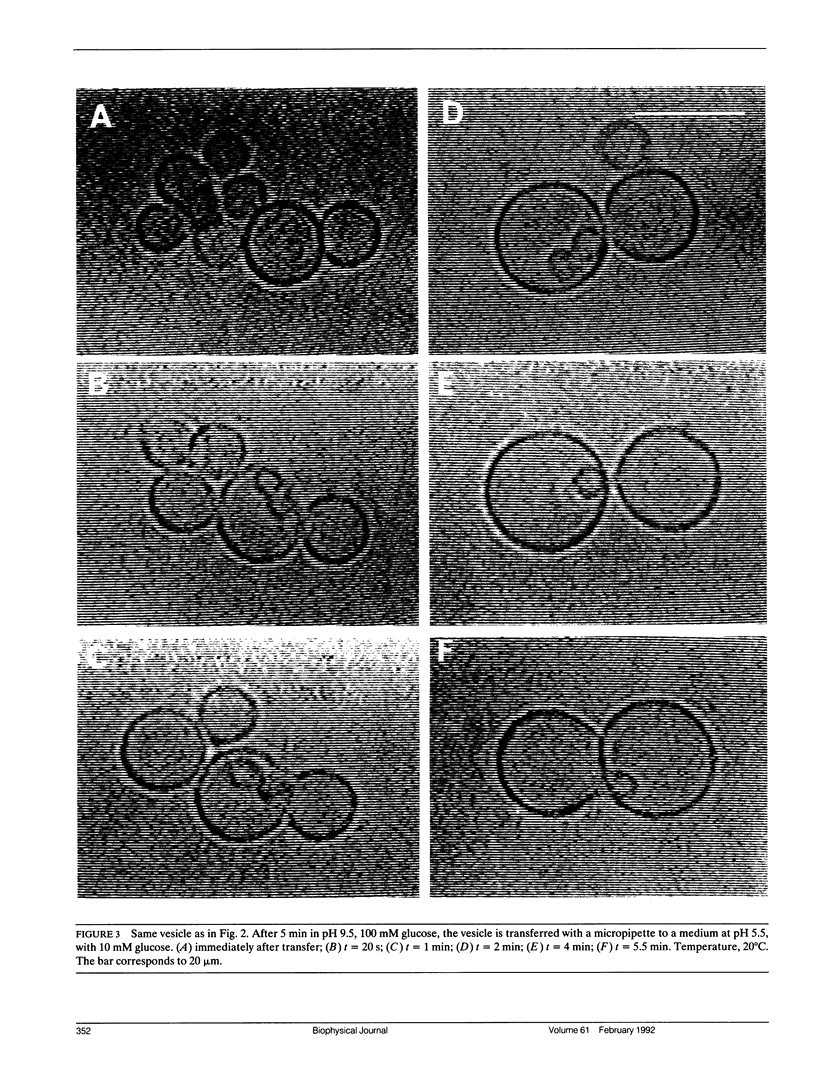
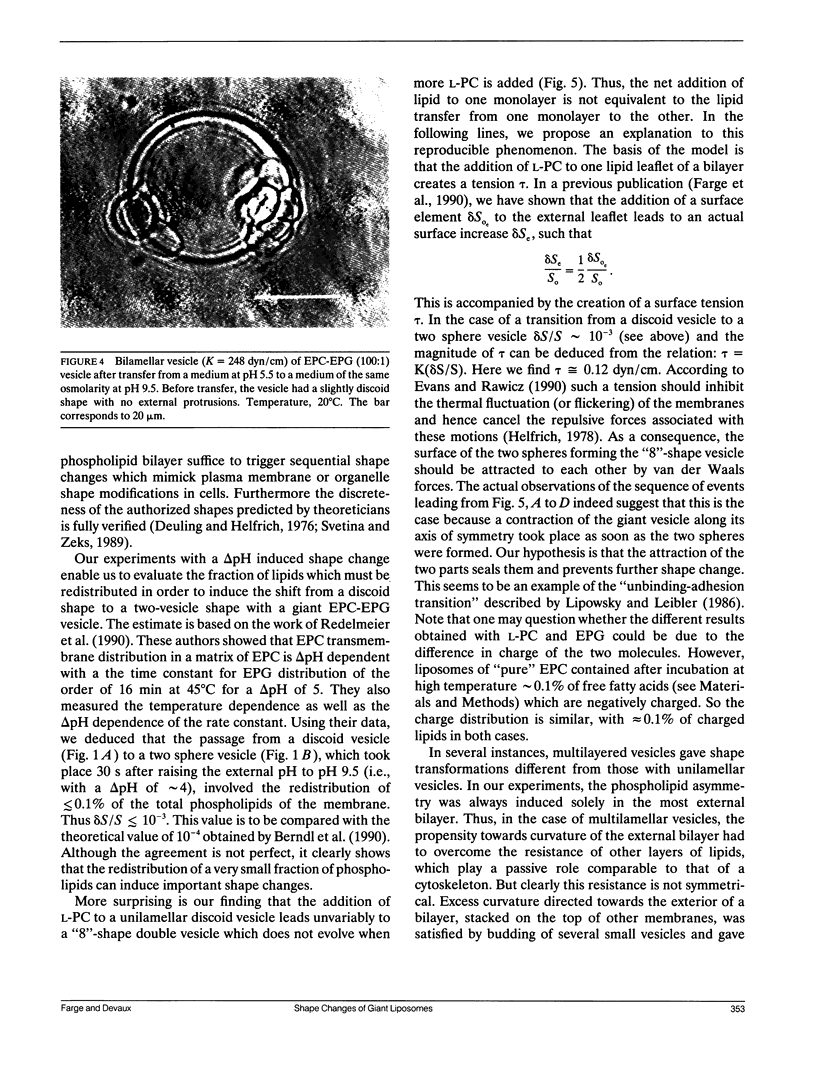
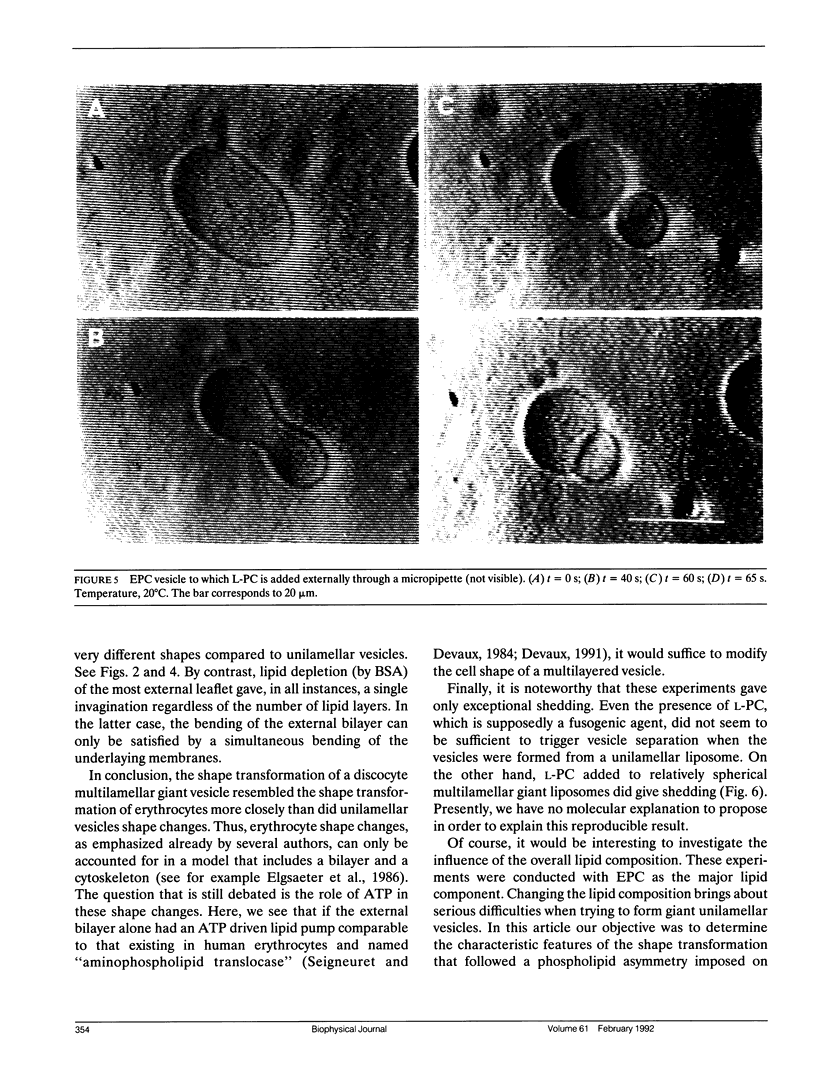
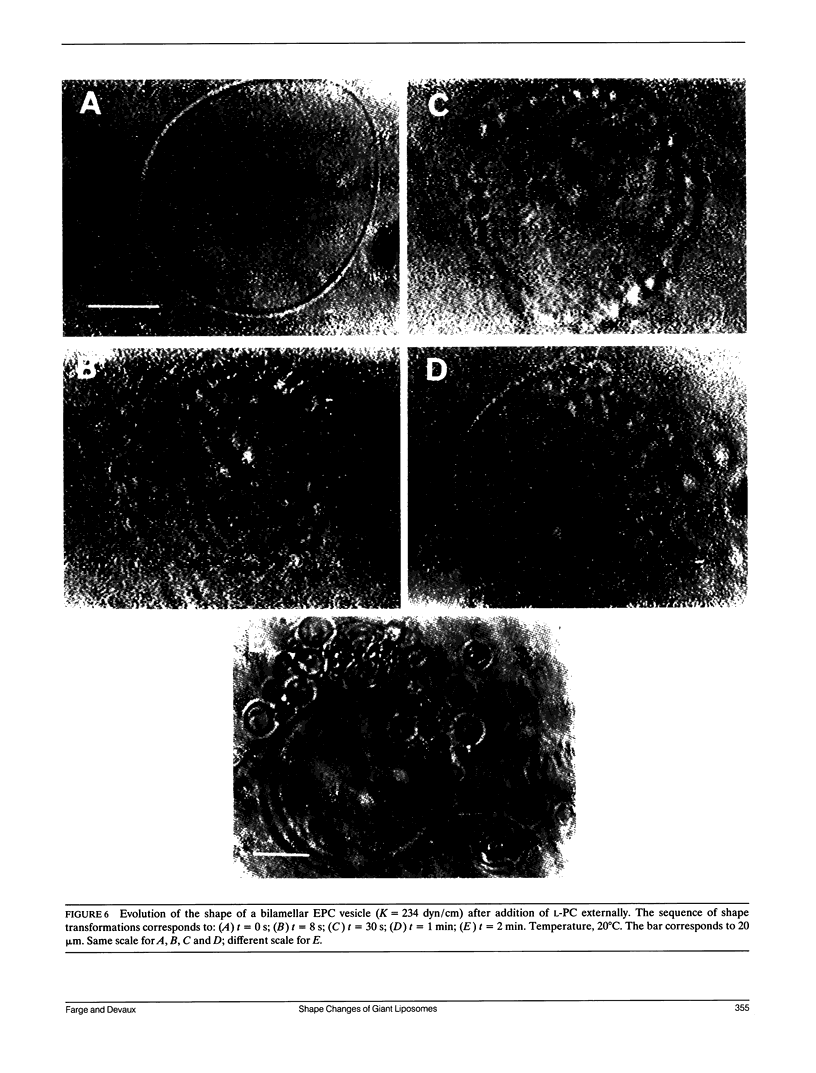
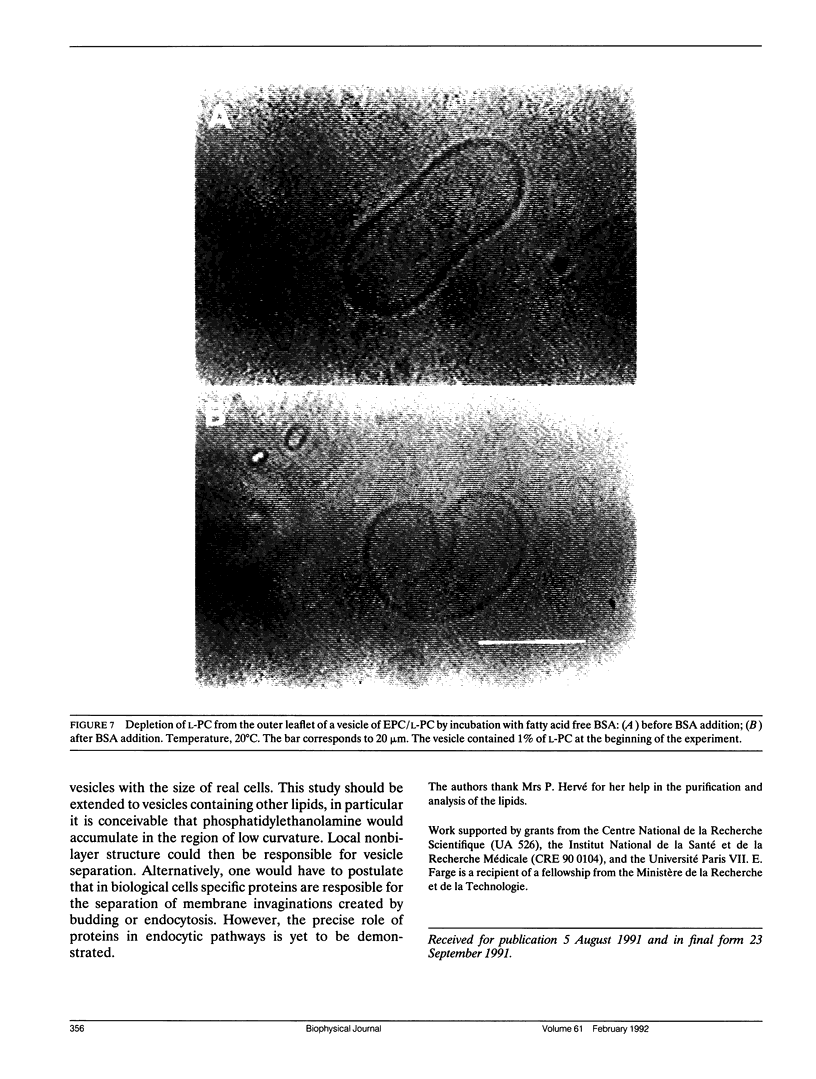
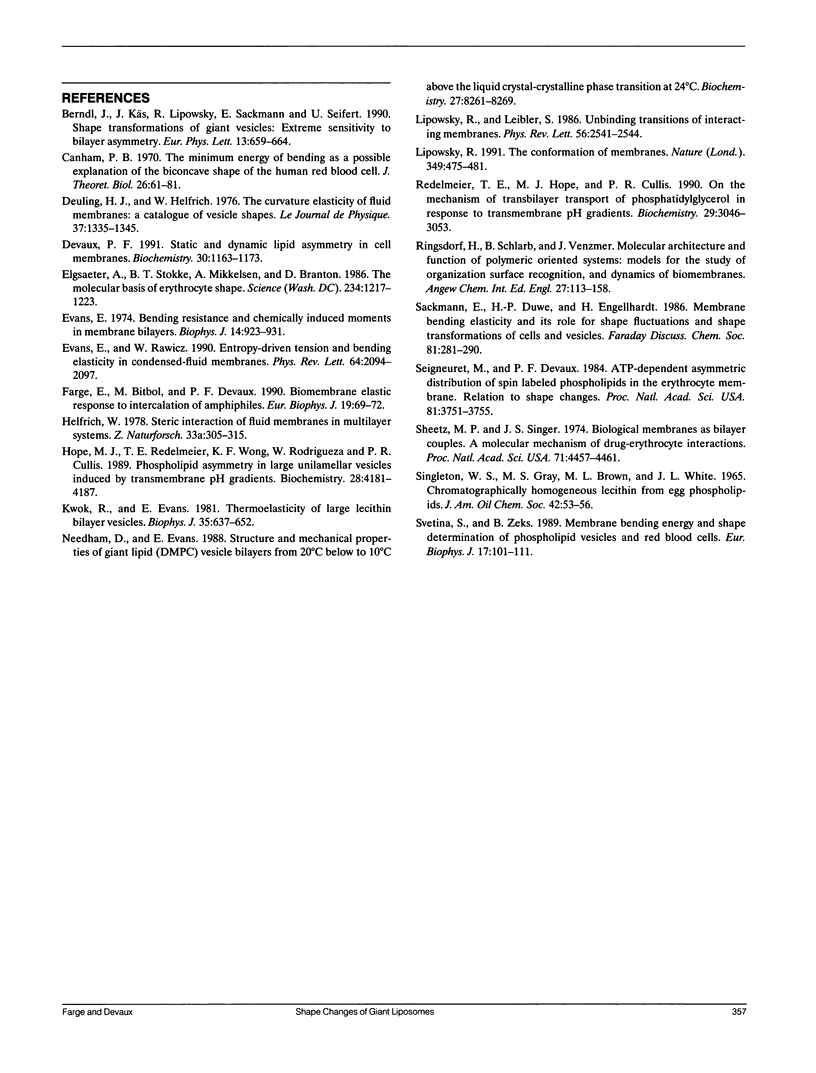
Images in this article
Selected References
These references are in PubMed. This may not be the complete list of references from this article.
- Canham P. B. The minimum energy of bending as a possible explanation of the biconcave shape of the human red blood cell. J Theor Biol. 1970 Jan;26(1):61–81. doi: 10.1016/s0022-5193(70)80032-7. [DOI] [PubMed] [Google Scholar]
- Devaux P. F. Static and dynamic lipid asymmetry in cell membranes. Biochemistry. 1991 Feb 5;30(5):1163–1173. doi: 10.1021/bi00219a001. [DOI] [PubMed] [Google Scholar]
- Elgsaeter A., Stokke B. T., Mikkelsen A., Branton D. The molecular basis of erythrocyte shape. Science. 1986 Dec 5;234(4781):1217–1223. doi: 10.1126/science.3775380. [DOI] [PubMed] [Google Scholar]
- Evans E. A. Bending resistance and chemically induced moments in membrane bilayers. Biophys J. 1974 Dec;14(12):923–931. doi: 10.1016/S0006-3495(74)85959-X. [DOI] [PMC free article] [PubMed] [Google Scholar]
- Evans E, Rawicz W. Entropy-driven tension and bending elasticity in condensed-fluid membranes. Phys Rev Lett. 1990 Apr 23;64(17):2094–2097. doi: 10.1103/PhysRevLett.64.2094. [DOI] [PubMed] [Google Scholar]
- Farge E., Bitbol M., Devaux P. F. Biomembrane elastic response to intercalation of amphiphiles. Eur Biophys J. 1990;19(2):69–72. doi: 10.1007/BF00185088. [DOI] [PubMed] [Google Scholar]
- Hope M. J., Redelmeier T. E., Wong K. F., Rodrigueza W., Cullis P. R. Phospholipid asymmetry in large unilamellar vesicles induced by transmembrane pH gradients. Biochemistry. 1989 May 16;28(10):4181–4187. doi: 10.1021/bi00436a009. [DOI] [PubMed] [Google Scholar]
- Kwok R., Evans E. Thermoelasticity of large lecithin bilayer vesicles. Biophys J. 1981 Sep;35(3):637–652. doi: 10.1016/S0006-3495(81)84817-5. [DOI] [PMC free article] [PubMed] [Google Scholar]
- Lipowsky R. The conformation of membranes. Nature. 1991 Feb 7;349(6309):475–481. doi: 10.1038/349475a0. [DOI] [PubMed] [Google Scholar]
- Lipowsky R, Leibler S. Unbinding transitions of interacting membranes. Phys Rev Lett. 1986 Jun 9;56(23):2541–2544. doi: 10.1103/PhysRevLett.56.2541. [DOI] [PubMed] [Google Scholar]
- Needham D., Evans E. Structure and mechanical properties of giant lipid (DMPC) vesicle bilayers from 20 degrees C below to 10 degrees C above the liquid crystal-crystalline phase transition at 24 degrees C. Biochemistry. 1988 Oct 18;27(21):8261–8269. doi: 10.1021/bi00421a041. [DOI] [PubMed] [Google Scholar]
- Redelmeier T. E., Hope M. J., Cullis P. R. On the mechanism of transbilayer transport of phosphatidylglycerol in response to transmembrane pH gradients. Biochemistry. 1990 Mar 27;29(12):3046–3053. doi: 10.1021/bi00464a022. [DOI] [PubMed] [Google Scholar]
- SINGLETON W. S., GRAY M. S., BROWN M. L., WHITE J. L. CHROMATOGRAPHICALLY HOMOGENEOUS LECITHIN FROM EGG PHOSPHOLIPIDS. J Am Oil Chem Soc. 1965 Jan;42:53–56. doi: 10.1007/BF02558256. [DOI] [PubMed] [Google Scholar]
- Sackmann E., Duwe H. P., Engelhardt H. Membrane bending elasticity and its role for shape fluctuations and shape transformations of cells and vesicles. Faraday Discuss Chem Soc. 1986;(81):281–290. doi: 10.1039/dc9868100281. [DOI] [PubMed] [Google Scholar]
- Seigneuret M., Devaux P. F. ATP-dependent asymmetric distribution of spin-labeled phospholipids in the erythrocyte membrane: relation to shape changes. Proc Natl Acad Sci U S A. 1984 Jun;81(12):3751–3755. doi: 10.1073/pnas.81.12.3751. [DOI] [PMC free article] [PubMed] [Google Scholar]
- Sheetz M. P., Singer S. J. Biological membranes as bilayer couples. A molecular mechanism of drug-erythrocyte interactions. Proc Natl Acad Sci U S A. 1974 Nov;71(11):4457–4461. doi: 10.1073/pnas.71.11.4457. [DOI] [PMC free article] [PubMed] [Google Scholar]
- Svetina S., Zeks B. Membrane bending energy and shape determination of phospholipid vesicles and red blood cells. Eur Biophys J. 1989;17(2):101–111. doi: 10.1007/BF00257107. [DOI] [PubMed] [Google Scholar]