Abstract
In this study, we have used the method of target analysis to analyze the ps fluorescence kinetics of pea chloroplasts with open (F0) and closed (Fmax) photosystem II (PS II) centers. Extending the exciton/radical pair equilibrium model (Schatz, G. H., H. Brock, and A. R. Holzwarth. 1988. Biophys. J. 54:397-405) to allow for PS II heterogeneity, we show that two types of PS II (labeled α and β) must be accounted for, each pool being characterized by its own set of molecular rate constants within the model. Simultaneous global target analysis of the data at F0 and Fmax results in a detailed description of the molecular kinetics and energetics of the primary processes in both types of PS II units. This characterization revealed that the PS IIα pool accounts for twice as many Chl molecules as PS IIβ, which suggests a PSIIα/PSIIβ reaction center stoichiometry of close to unity. By extrapolation it is shown that the primary charge separation in hypothetical “isolated” β reaction centers is slower than in isolated α reaction centers: in open centers by a factor of 4 (1/k1int = 11 vs 2.9 ps), in closed centers by a factor of 2 (1/k1int = 34 vs 19 ps). Despite this slower charge separation process in PS IIβ, the quantum efficiency of the charge separation process is hardly affected: a charge stabilization yield at F0, (i.e., P+IQA-) of 86% (as compared to 90% in PS IIα). Reduction of QA (closing PS II) has distinctly different effects on the primary kinetics of PS IIβ, as compared to PS IIα. In PS IIα the charge separation rate drops by a factor of 6, whereas the charge recombination process is hardly affected. In PS IIβ the charge separation is slowed down by a factor of 3, whereas the charge recombination rate increases by a factor of 5. In terms of changes in standard free energy, the reduction to QA- lifts the free energy of the radical pair P+I-, relative to the excited state (Chln/P)*, by 47 meV in PS IIα and by 67 meV in PS IIβ. The concomitant increase in fluorescence quantum yield is the same for both types of PS II. These results show that PS IIα and PS IIβ exhibit a different molecular functioning with respect to the primary processes, which might have its origin in a different molecular structure of the reaction centers and/or a different local environment of these centers. Location in different parts of the thylakoid membrane might be involved. We also applied different error analysis procedures to determine the error ranges of the values found for the molecular rate constants. It is shown that the commonly used standard error has very little meaning, as it assumes independence of the fit parameters. Instead, an exhaustive search procedure, accounting for all possible correlations between the fit parameters, gives a more realistic view on the accuracy of the fit parameters.
Full text
PDF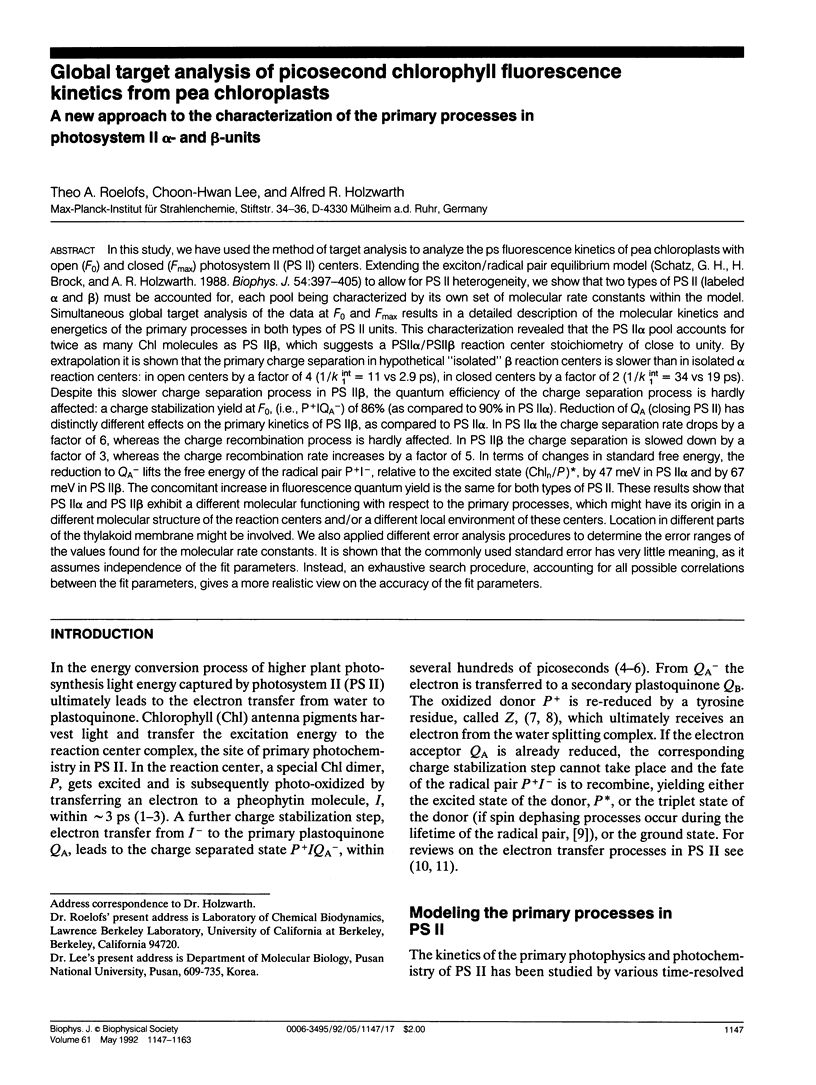
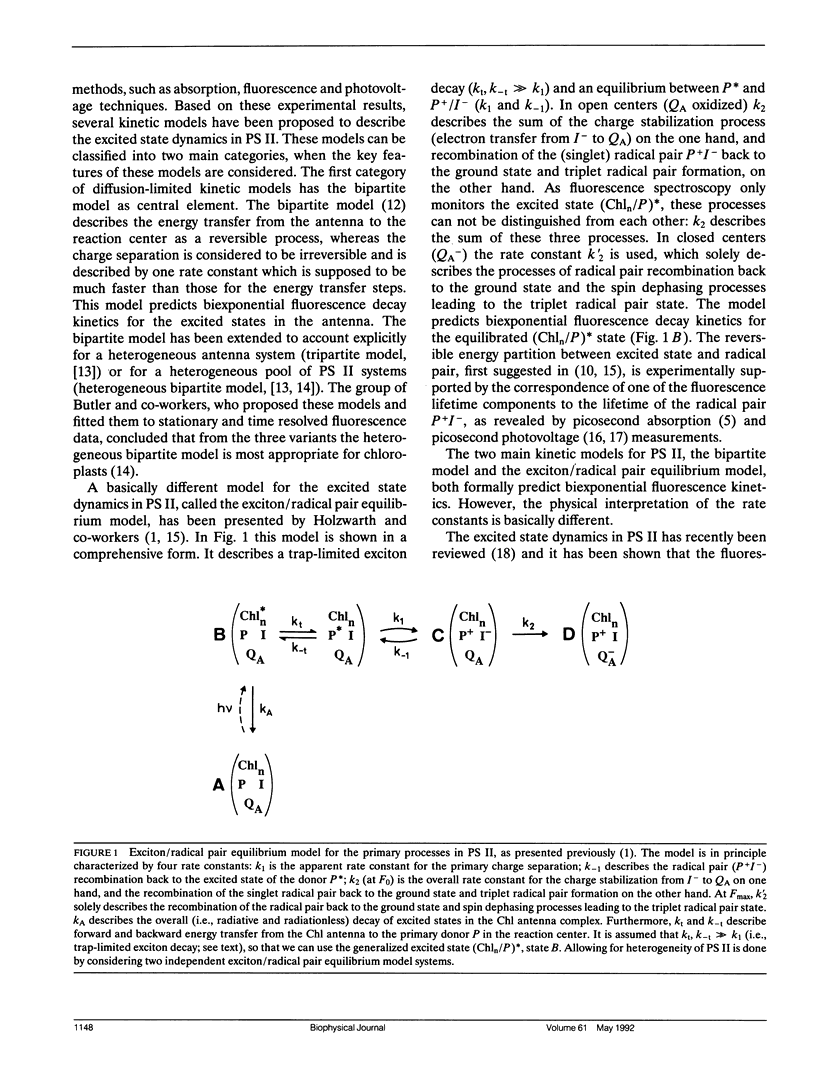
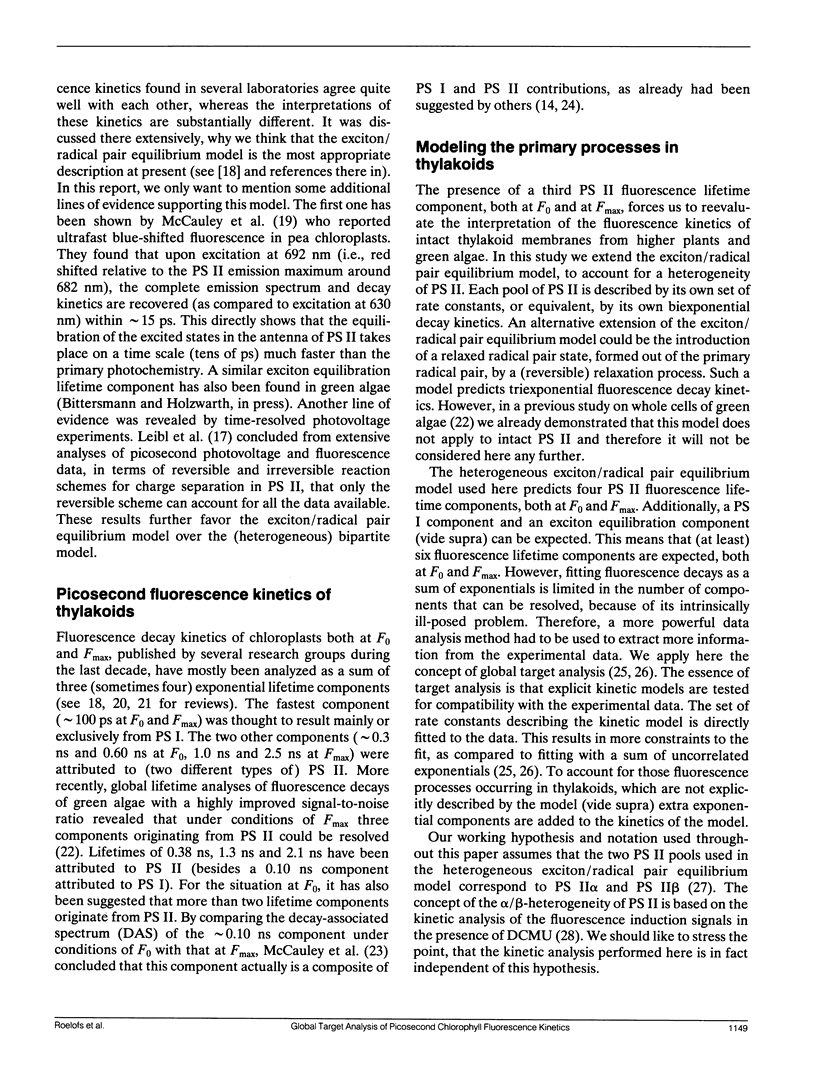
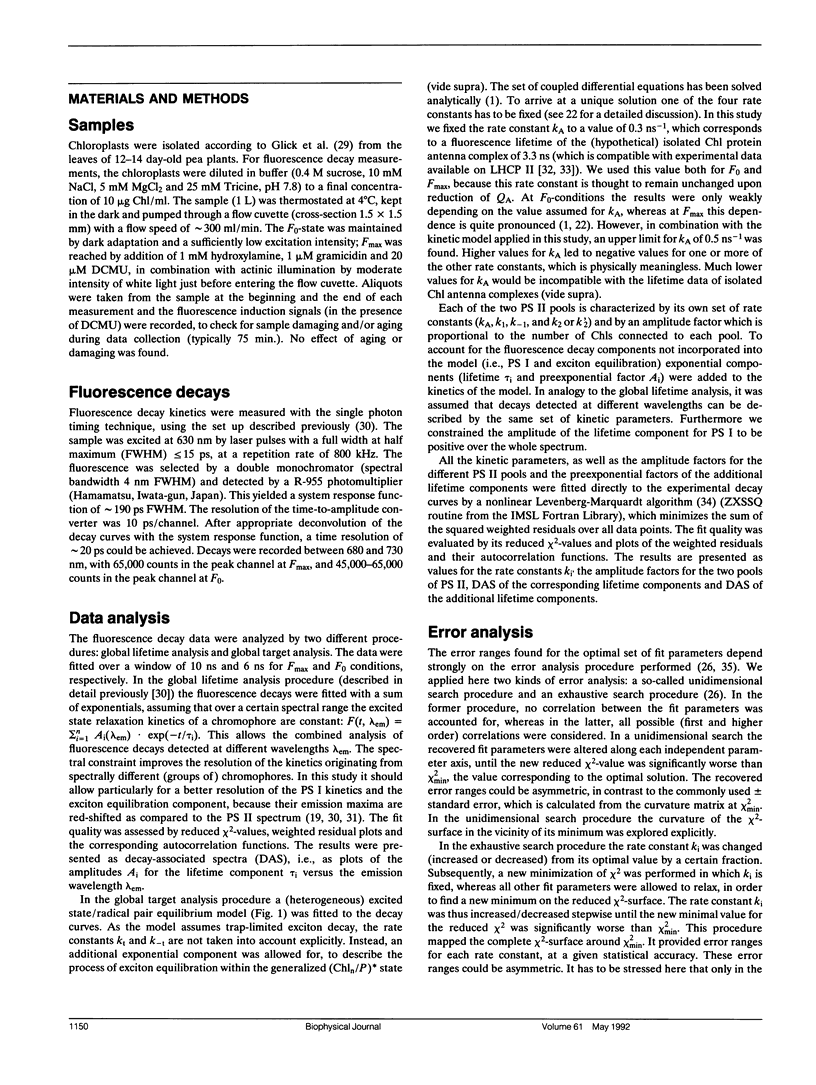
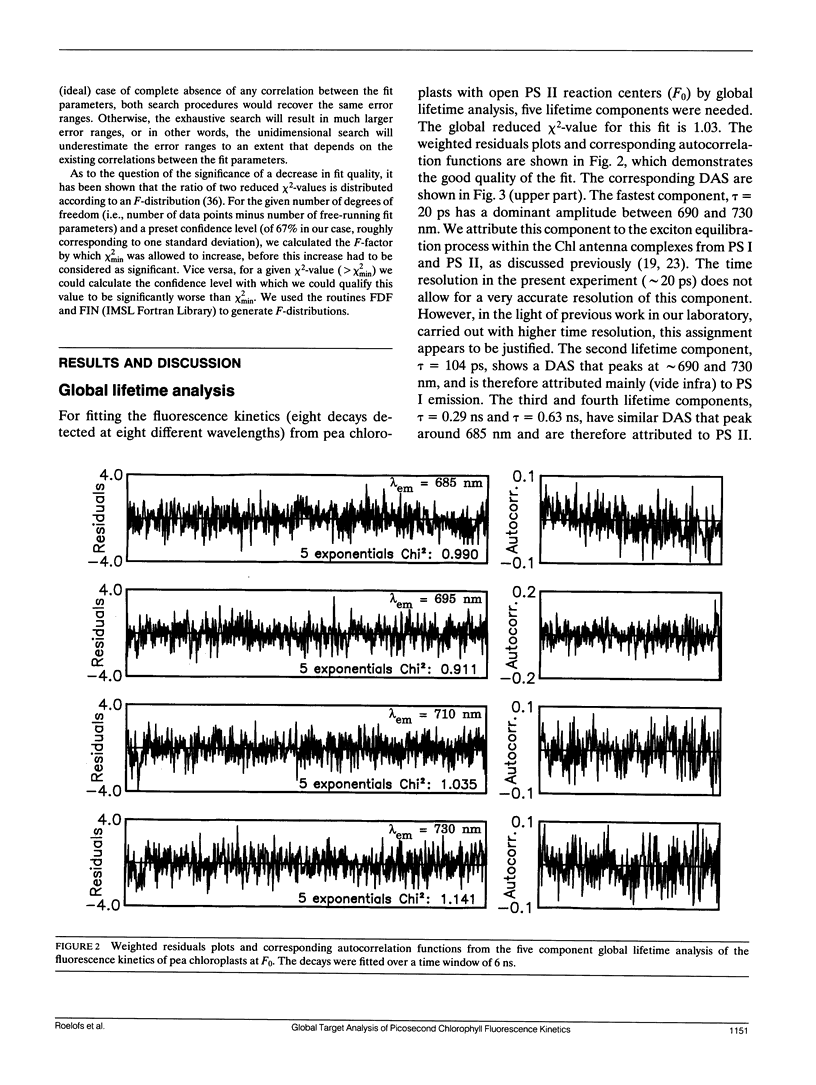
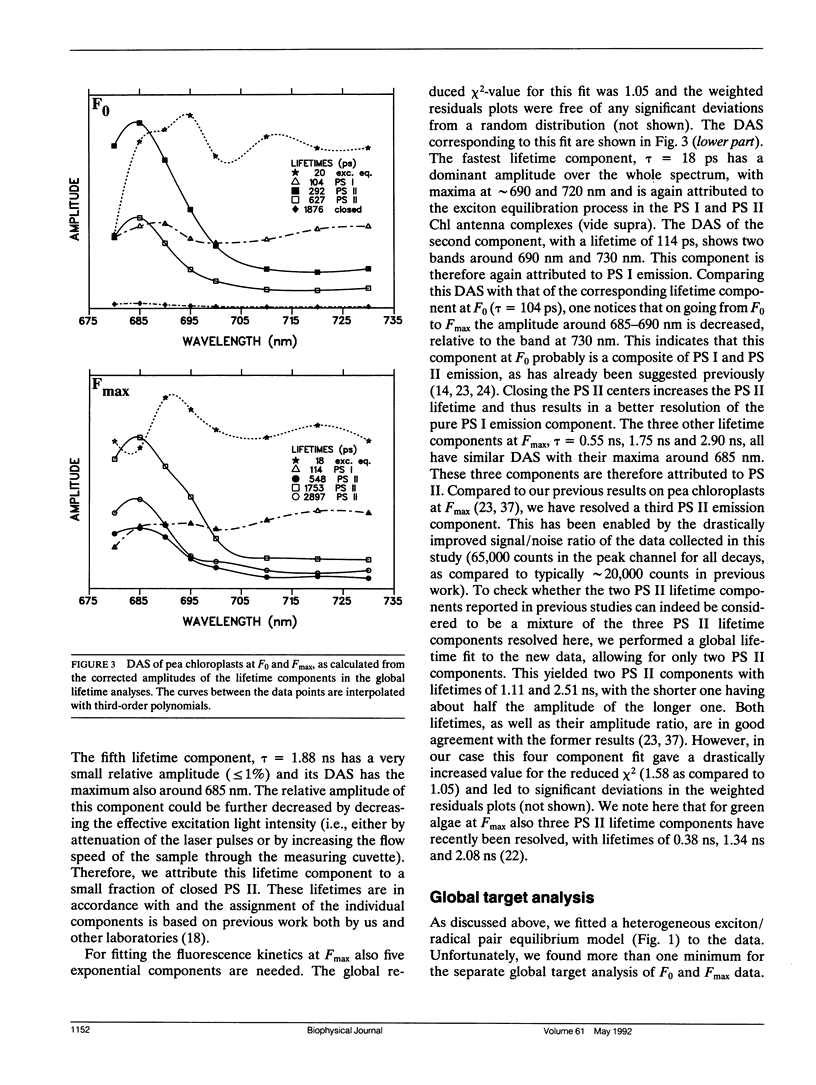
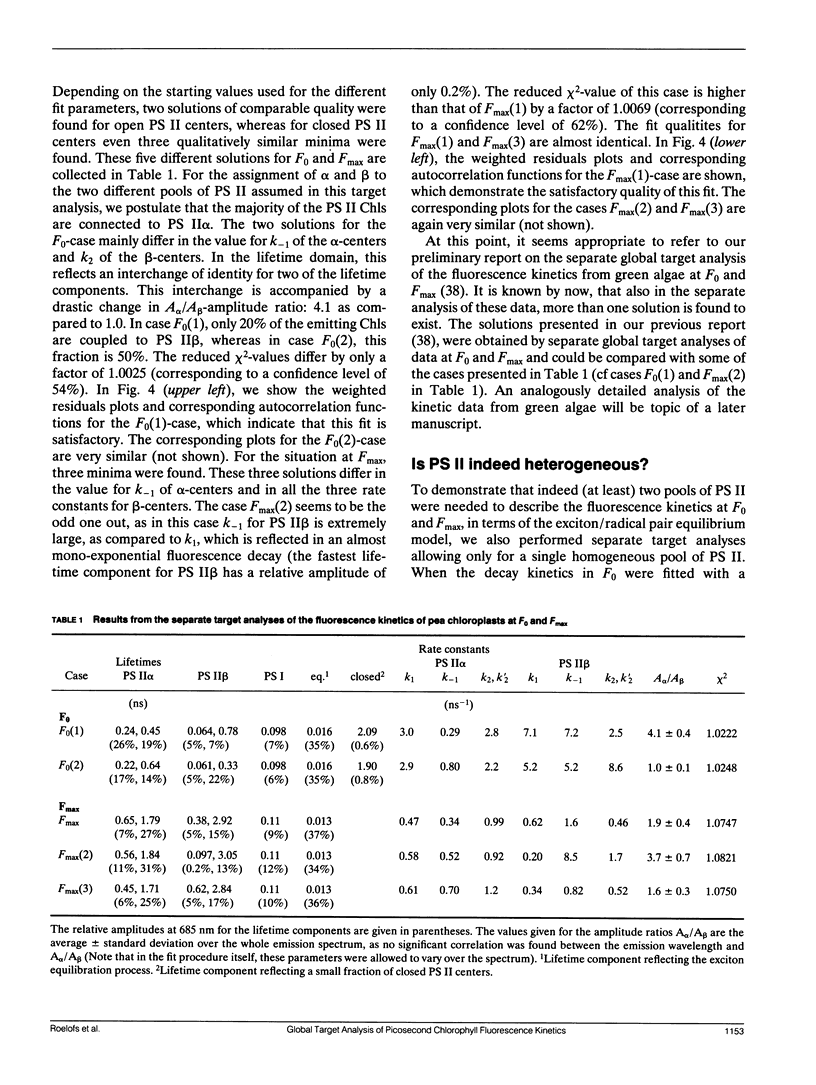
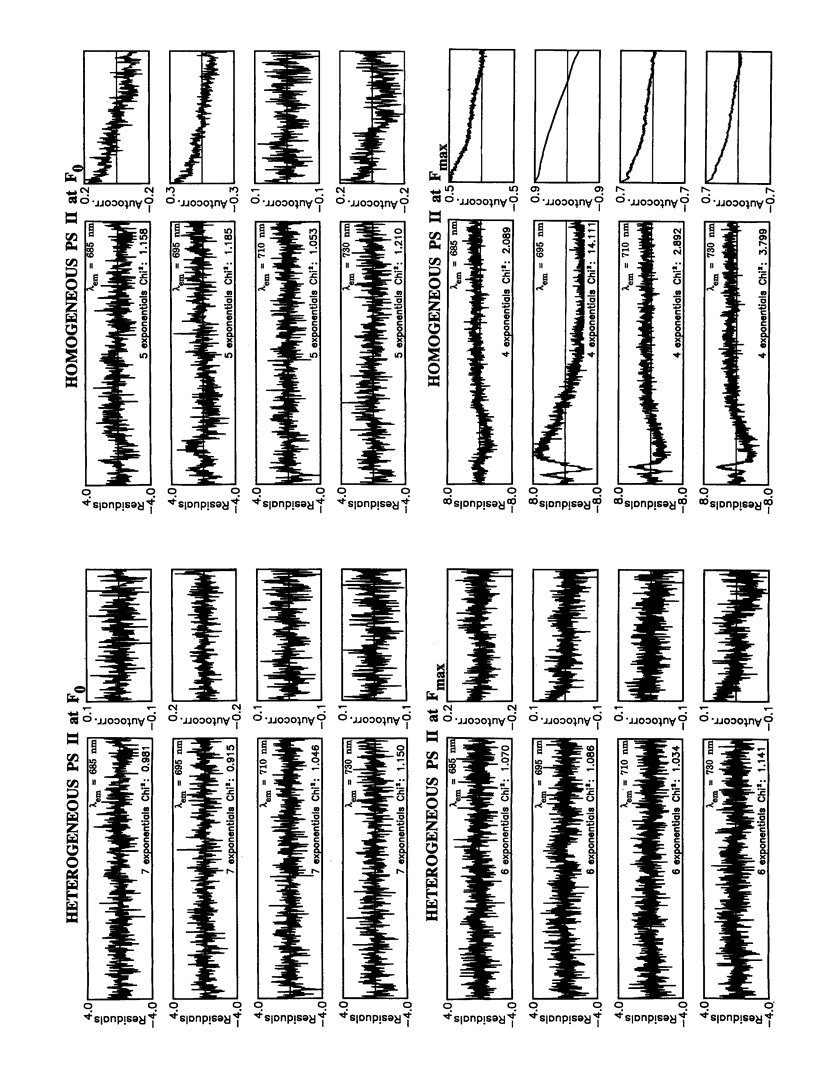
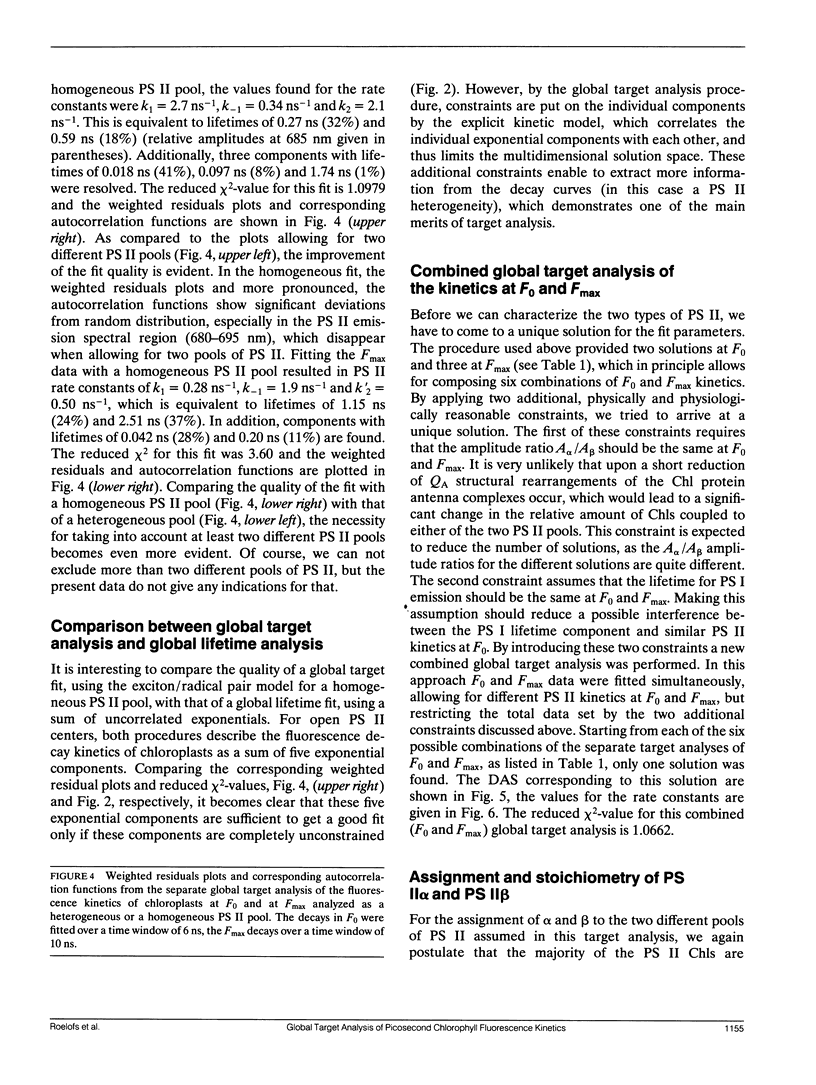
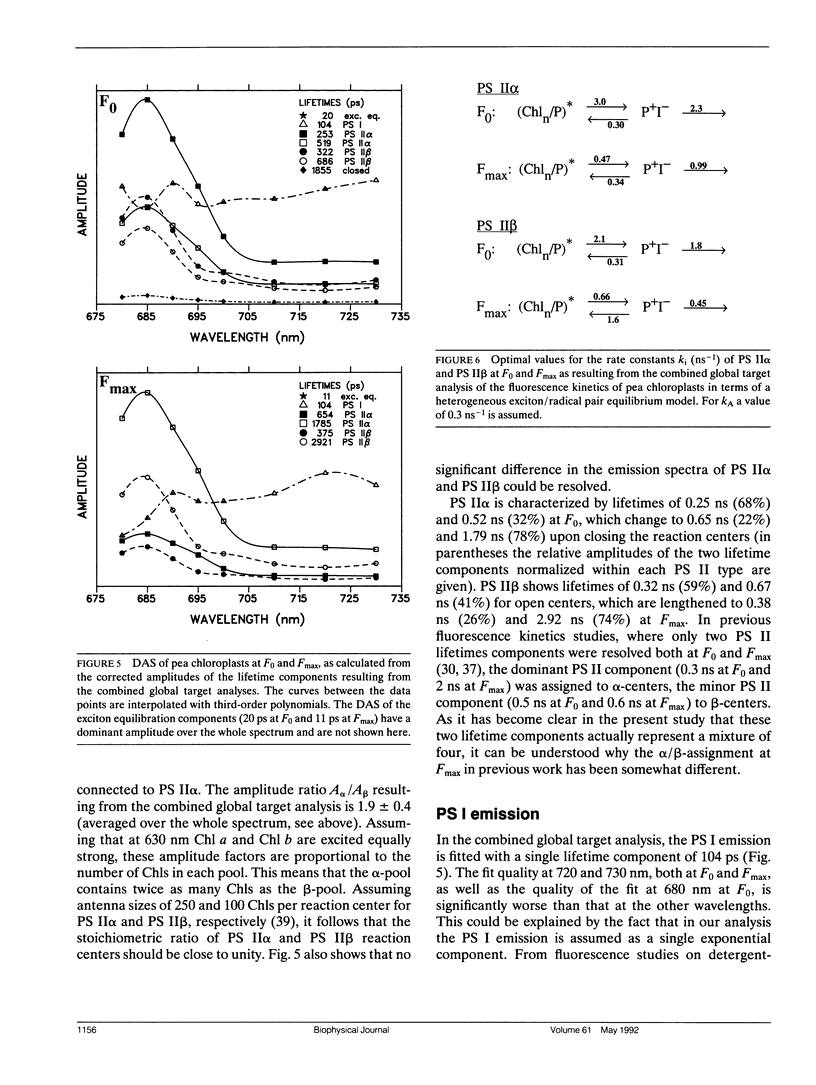
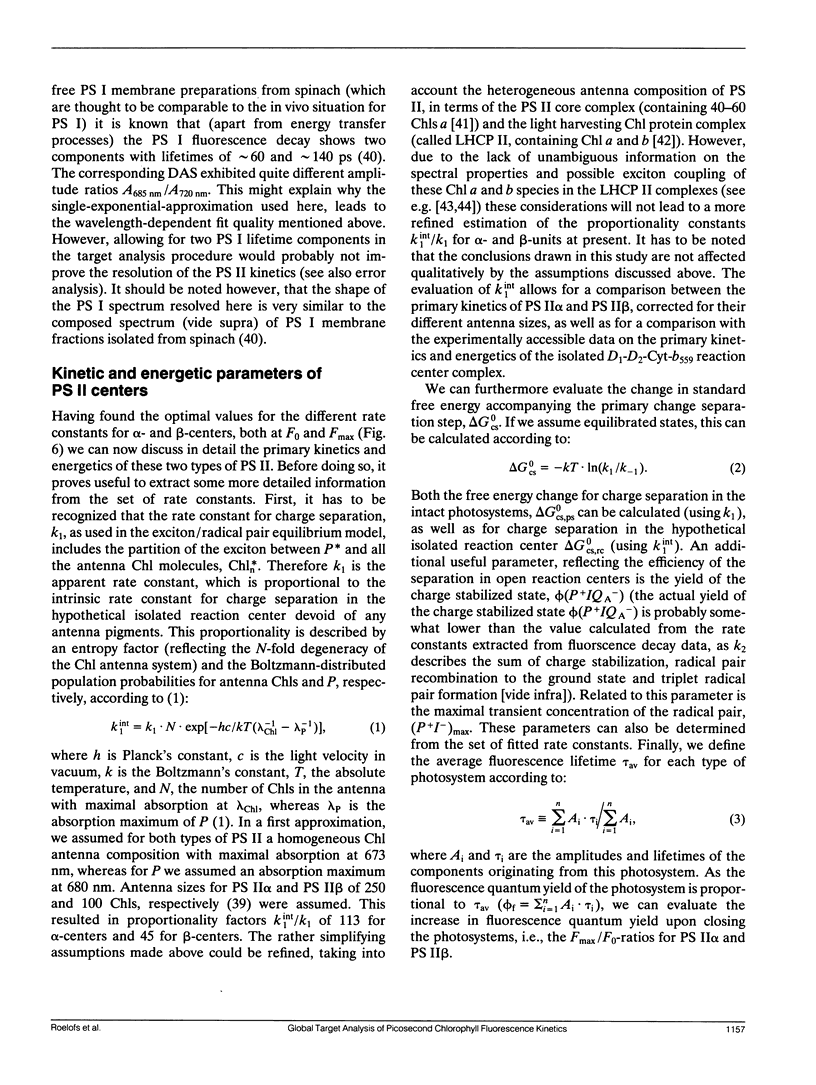
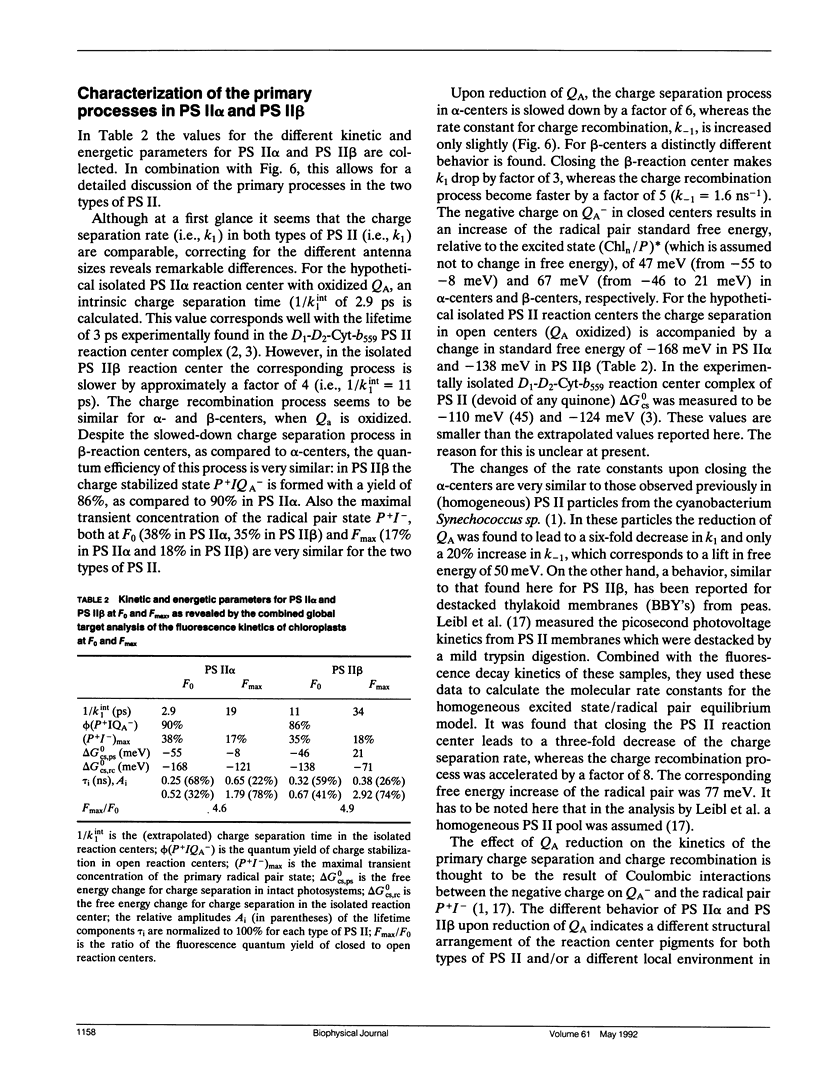
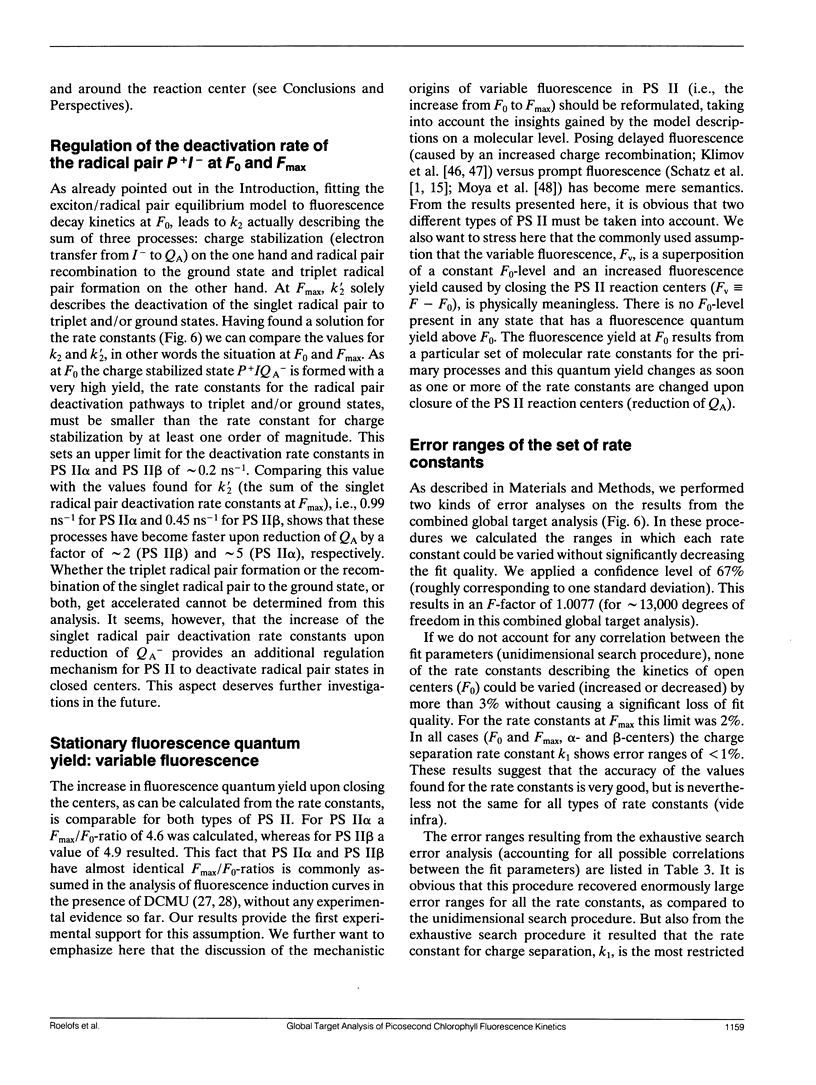
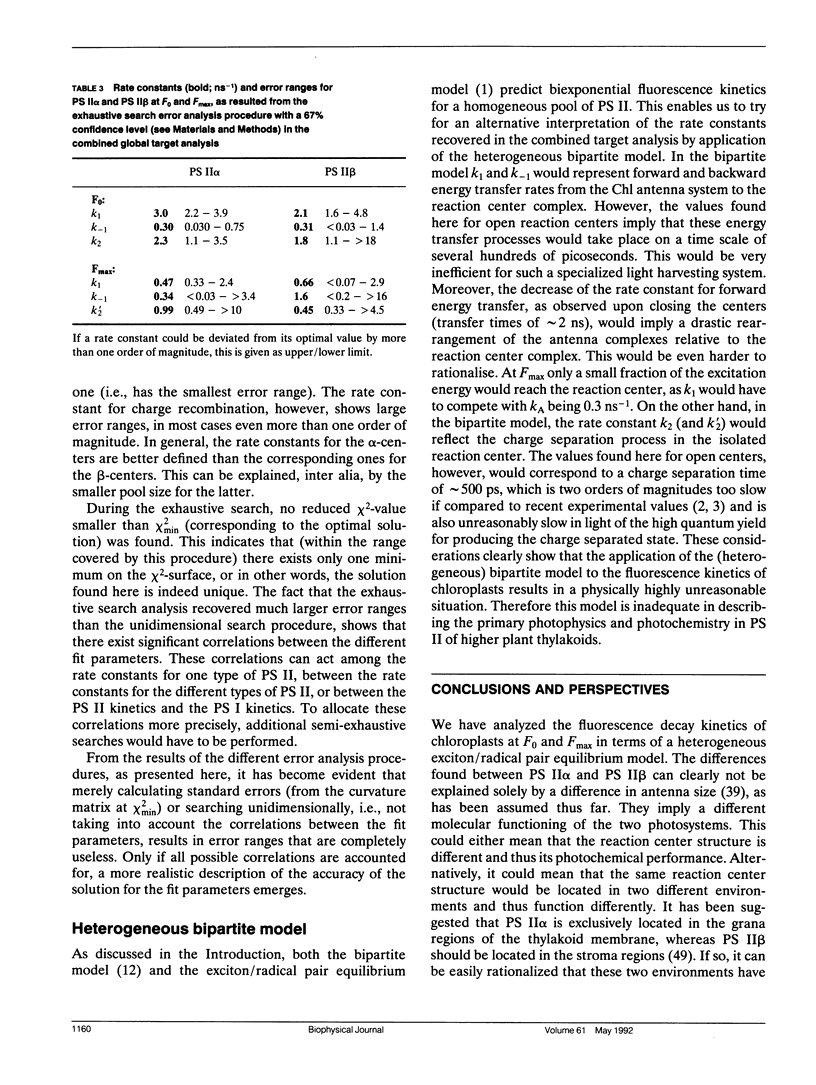
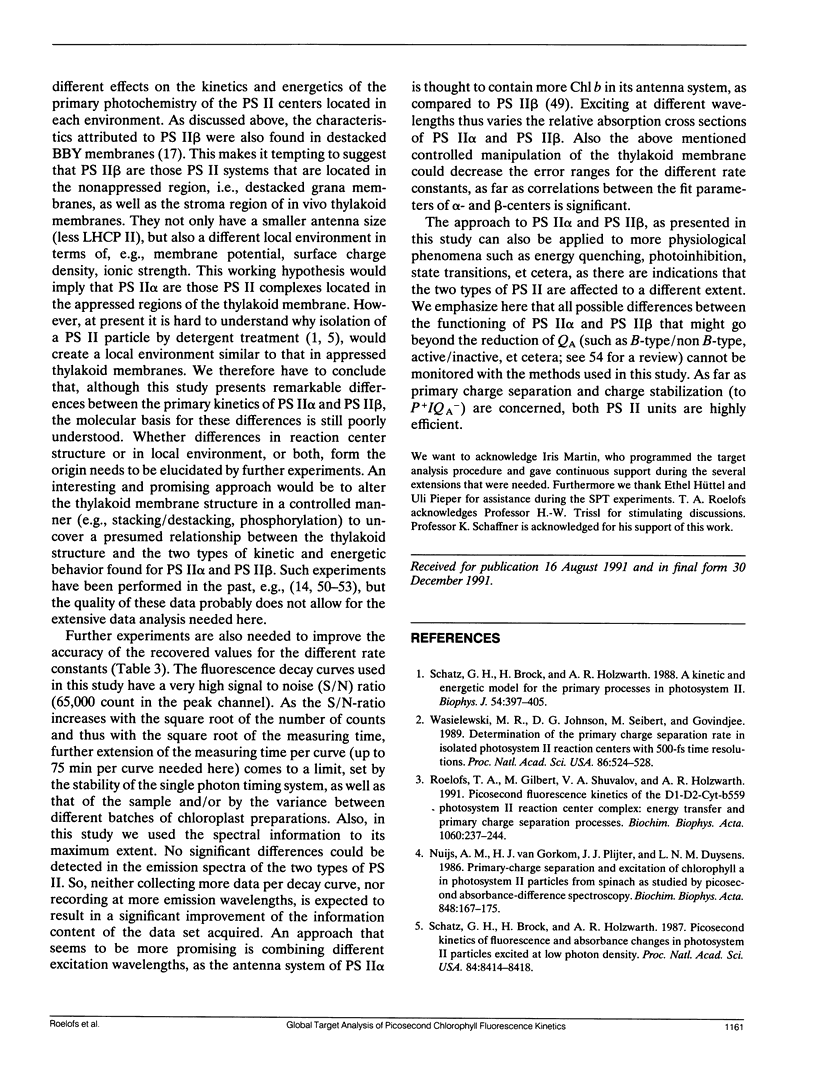
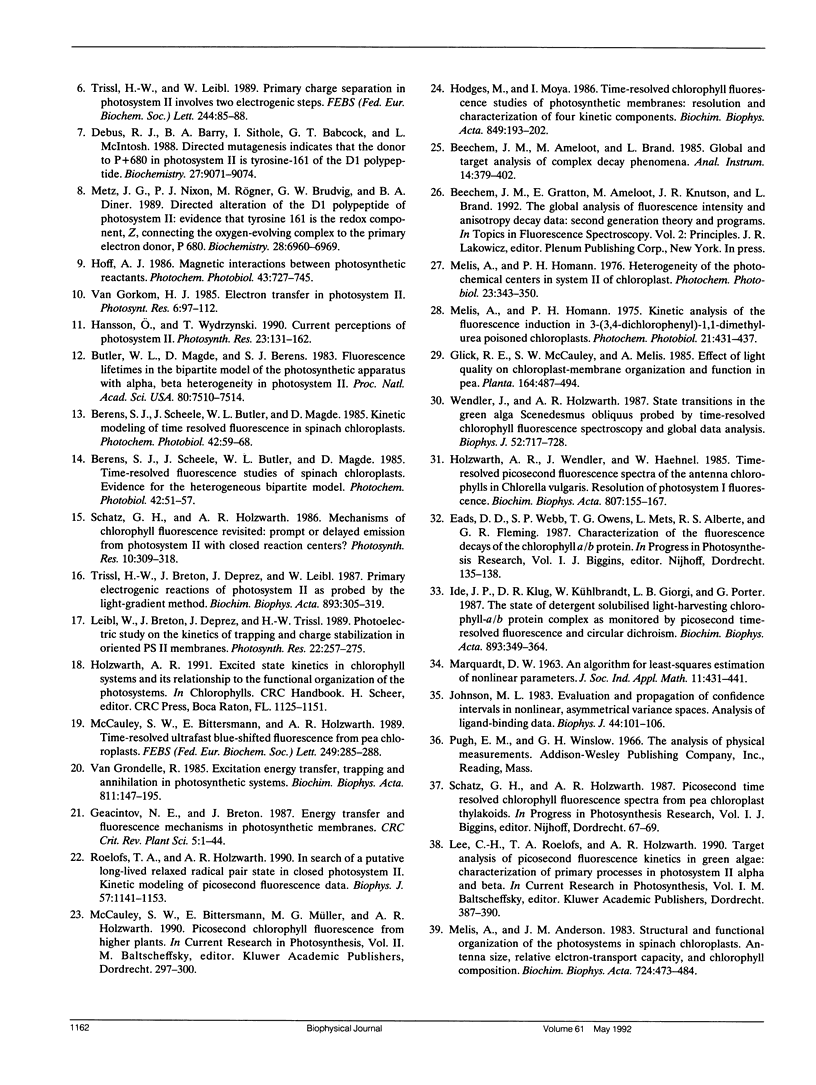
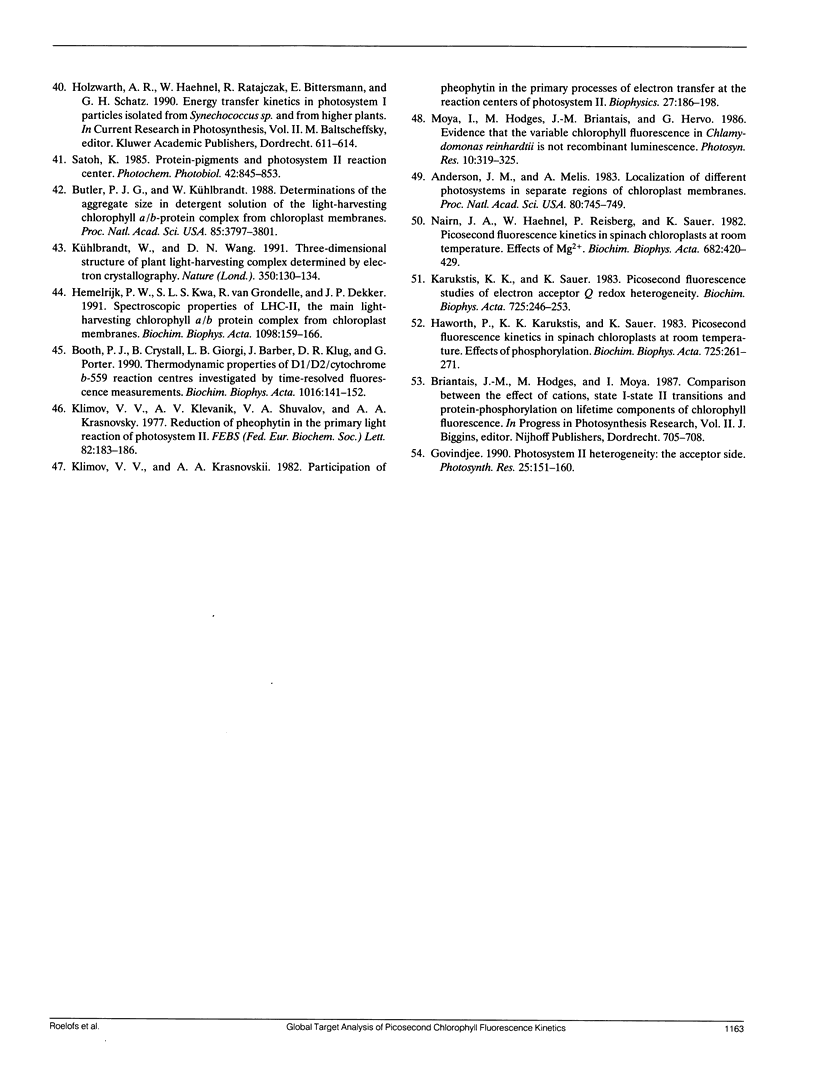
Selected References
These references are in PubMed. This may not be the complete list of references from this article.
- Anderson J. M., Melis A. Localization of different photosystems in separate regions of chloroplast membranes. Proc Natl Acad Sci U S A. 1983 Feb;80(3):745–749. doi: 10.1073/pnas.80.3.745. [DOI] [PMC free article] [PubMed] [Google Scholar]
- Butler P. J., Kühlbrandt W. Determination of the aggregate size in detergent solution of the light-harvesting chlorophyll a/b-protein complex from chloroplast membranes. Proc Natl Acad Sci U S A. 1988 Jun;85(11):3797–3801. doi: 10.1073/pnas.85.11.3797. [DOI] [PMC free article] [PubMed] [Google Scholar]
- Butler W. L., Magde D., Berens S. J. Fluorescence lifetimes in the bipartite model of the photosynthetic apparatus with alpha, beta heterogeneity in photosystem II. Proc Natl Acad Sci U S A. 1983 Dec;80(24):7510–7514. doi: 10.1073/pnas.80.24.7510. [DOI] [PMC free article] [PubMed] [Google Scholar]
- Debus R. J., Barry B. A., Sithole I., Babcock G. T., McIntosh L. Directed mutagenesis indicates that the donor to P+680 in photosystem II is tyrosine-161 of the D1 polypeptide. Biochemistry. 1988 Dec 27;27(26):9071–9074. doi: 10.1021/bi00426a001. [DOI] [PubMed] [Google Scholar]
- Johnson M. L. Evaluation and propagation of confidence intervals in nonlinear, asymmetrical variance spaces. Analysis of ligand-binding data. Biophys J. 1983 Oct;44(1):101–106. doi: 10.1016/S0006-3495(83)84281-7. [DOI] [PMC free article] [PubMed] [Google Scholar]
- Klimov V. V., Klevanik A. V., Shuvalov V. A., Kransnovsky A. A. Reduction of pheophytin in the primary light reaction of photosystem II. FEBS Lett. 1977 Oct 15;82(2):183–186. doi: 10.1016/0014-5793(77)80580-2. [DOI] [PubMed] [Google Scholar]
- Kühlbrandt W., Wang D. N. Three-dimensional structure of plant light-harvesting complex determined by electron crystallography. Nature. 1991 Mar 14;350(6314):130–134. doi: 10.1038/350130a0. [DOI] [PubMed] [Google Scholar]
- Melis A., Homann P. H. Heterogeneity of the photochemical centers in system II of chloroplasts. Photochem Photobiol. 1976 May;23(5):343–350. doi: 10.1111/j.1751-1097.1976.tb07259.x. [DOI] [PubMed] [Google Scholar]
- Metz J. G., Nixon P. J., Rögner M., Brudvig G. W., Diner B. A. Directed alteration of the D1 polypeptide of photosystem II: evidence that tyrosine-161 is the redox component, Z, connecting the oxygen-evolving complex to the primary electron donor, P680. Biochemistry. 1989 Aug 22;28(17):6960–6969. doi: 10.1021/bi00443a028. [DOI] [PubMed] [Google Scholar]
- Roelofs T. A., Holzwarth A. R. In search of a putative long-lived relaxed radical pair state in closed photosystem II: Kinetic modeling of picosecond fluorescence data. Biophys J. 1990 Jun;57(6):1141–1153. doi: 10.1016/S0006-3495(90)82634-5. [DOI] [PMC free article] [PubMed] [Google Scholar]
- Schatz G. H., Brock H., Holzwarth A. R. Kinetic and Energetic Model for the Primary Processes in Photosystem II. Biophys J. 1988 Sep;54(3):397–405. doi: 10.1016/S0006-3495(88)82973-4. [DOI] [PMC free article] [PubMed] [Google Scholar]
- Schatz G. H., Brock H., Holzwarth A. R. Picosecond kinetics of fluorescence and absorbance changes in photosystem II particles excited at low photon density. Proc Natl Acad Sci U S A. 1987 Dec;84(23):8414–8418. doi: 10.1073/pnas.84.23.8414. [DOI] [PMC free article] [PubMed] [Google Scholar]
- Wasielewski M. R., Johnson D. G., Seibert M., Govindjee Determination of the primary charge separation rate in isolated photosystem II reaction centers with 500-fs time resolution. Proc Natl Acad Sci U S A. 1989 Jan;86(2):524–528. doi: 10.1073/pnas.86.2.524. [DOI] [PMC free article] [PubMed] [Google Scholar]
- Wendler J., Holzwarth A. R. State transitions in the green alga scenedesmus obliquus probed by time-resolved chlorophyll fluorescence spectroscopy and global data analysis. Biophys J. 1987 Nov;52(5):717–728. doi: 10.1016/S0006-3495(87)83266-6. [DOI] [PMC free article] [PubMed] [Google Scholar]