Abstract
Two experimental techniques have been utilized to explore the barrier properties of lecithin/decane bilayer membranes with the aim of determining the contributions of various domains within the bilayer to the overall barrier. The thickness of lecithin/decane bilayers was systematically varied by modulating the chemical potential of decane in the annulus surrounding the bilayer using different mole fractions of squalene in decane. The dependence of permeability of a model permeant (acetamide) on the thickness of the solvent-filled region of the bilayer was assessed in these bilayers to determine the contribution of this region to the overall barrier. The flux of acetamide was found to vary linearly with bilayer area with Pm = (2.9 +/- 0.3) x 10(-4) cm s-1, after correcting for diffusion through unstirred water layers. The ratio between the overall membrane permeability coefficient and that calculated for diffusion through the hydrocarbon core in membranes having maximum thickness was 0.24, suggesting that the solvent domain contributes only slightly to the overall barrier properties. Consistent with these results, the permeability of acetamide was found to be independent of bilayer thickness. The relative contributions of the bilayer interface and ordered hydrocarbon regions to the transport barrier may be evaluated qualitatively by exploring the effective chemical nature of the barrier microenvironment. This may be probed by comparing functional group contributions to transport with those obtained for partitioning between water and various model bulk solvents ranging in polarity or hydrogen-bonding potential. A novel approach is described for obtaining group contributions to transport using ionizable permeants and pH adjustment. Using this approach, bilayer permeability coefficients of p-toluic acid and p-hydroxymethyl benzoic acid were determined to be 1.1 +/- 0.2 cm s-1 and (1.6 +/- 0.4) x 10(-3) cm s-1, respectively. From these values, the -OH group contribution to bilayer transport [delta(delta G0-OH)] was found to be 3.9 kcal/mol. This result suggests that the barrier region of the bilayer does not resemble the hydrogen-bonding environment found in octanol, but is somewhat less selective (more polar) than a hydrocarbon solvent.
Full text
PDF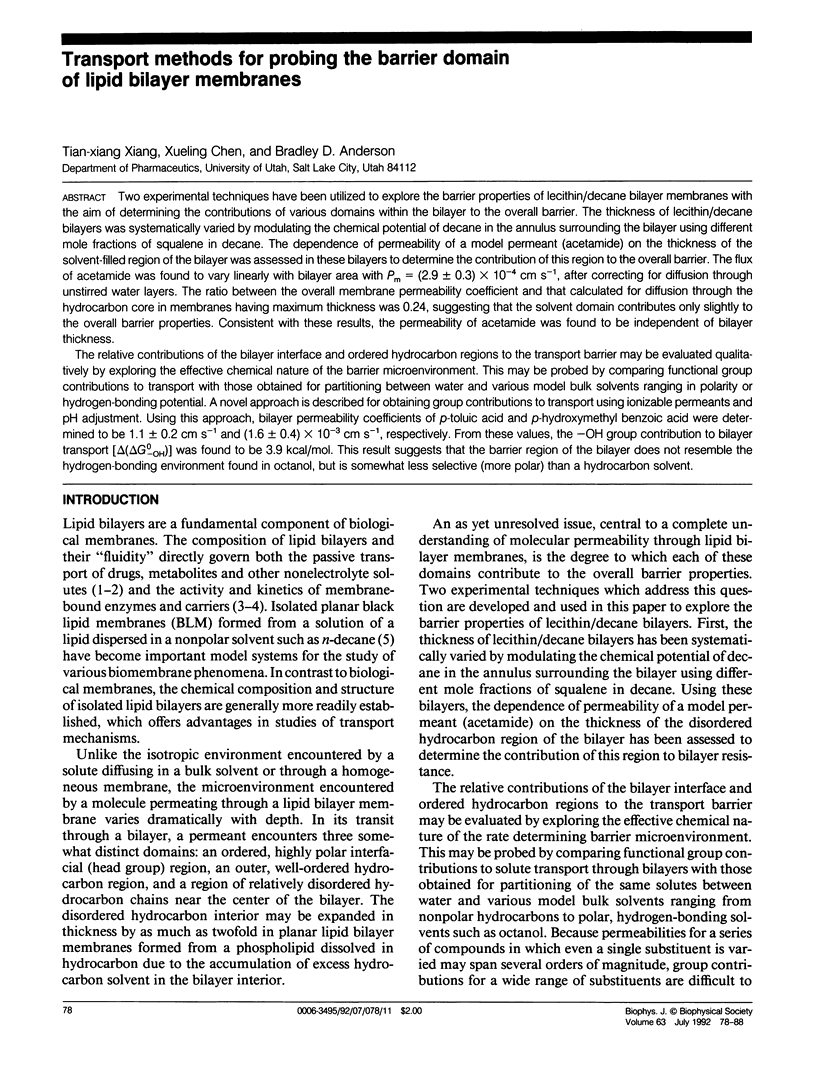
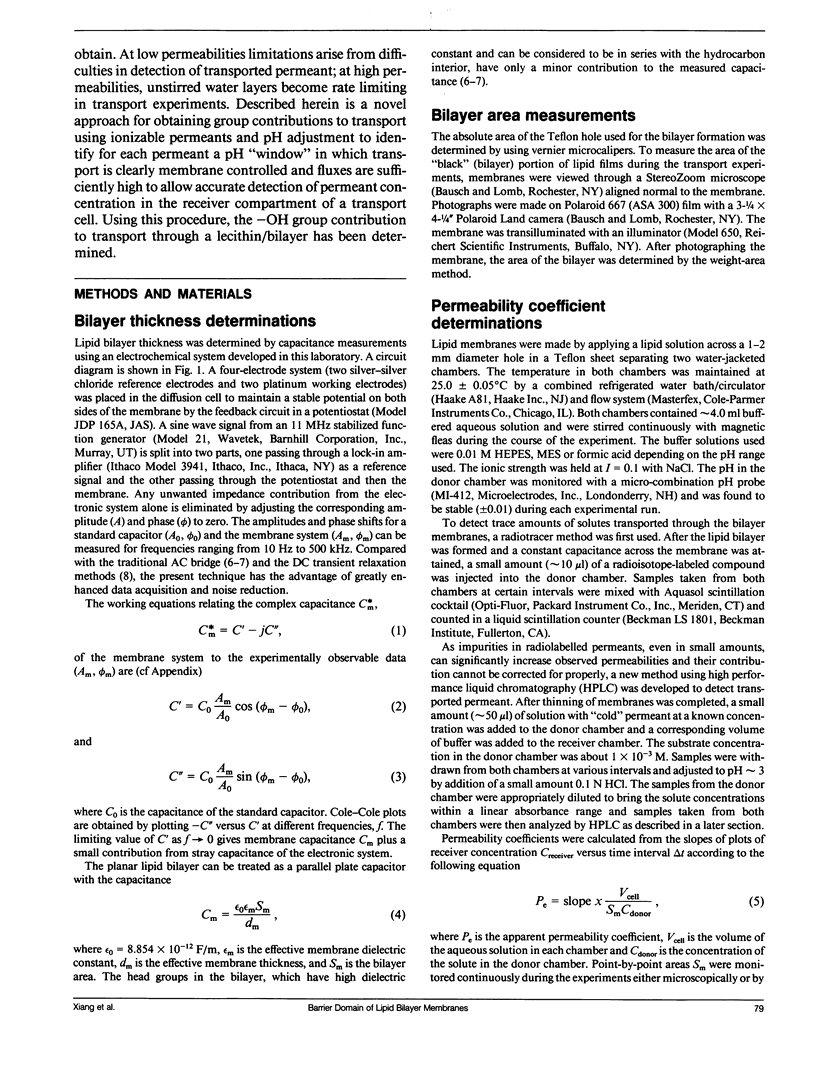
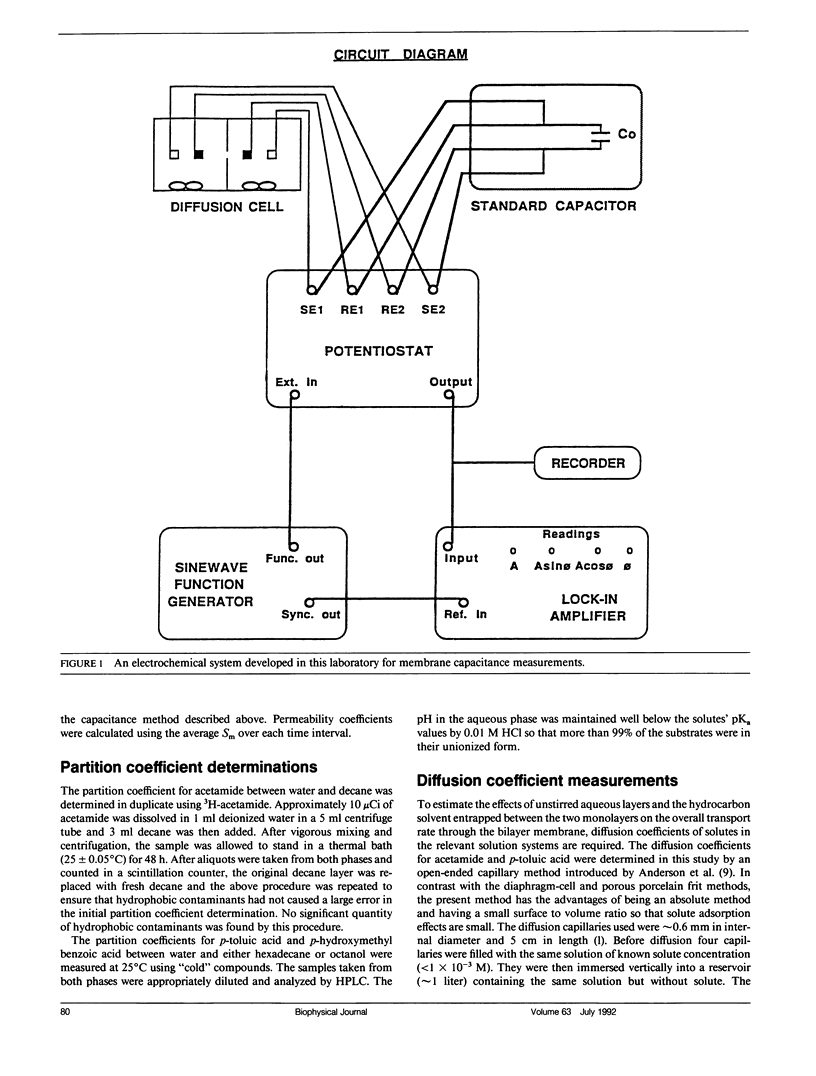
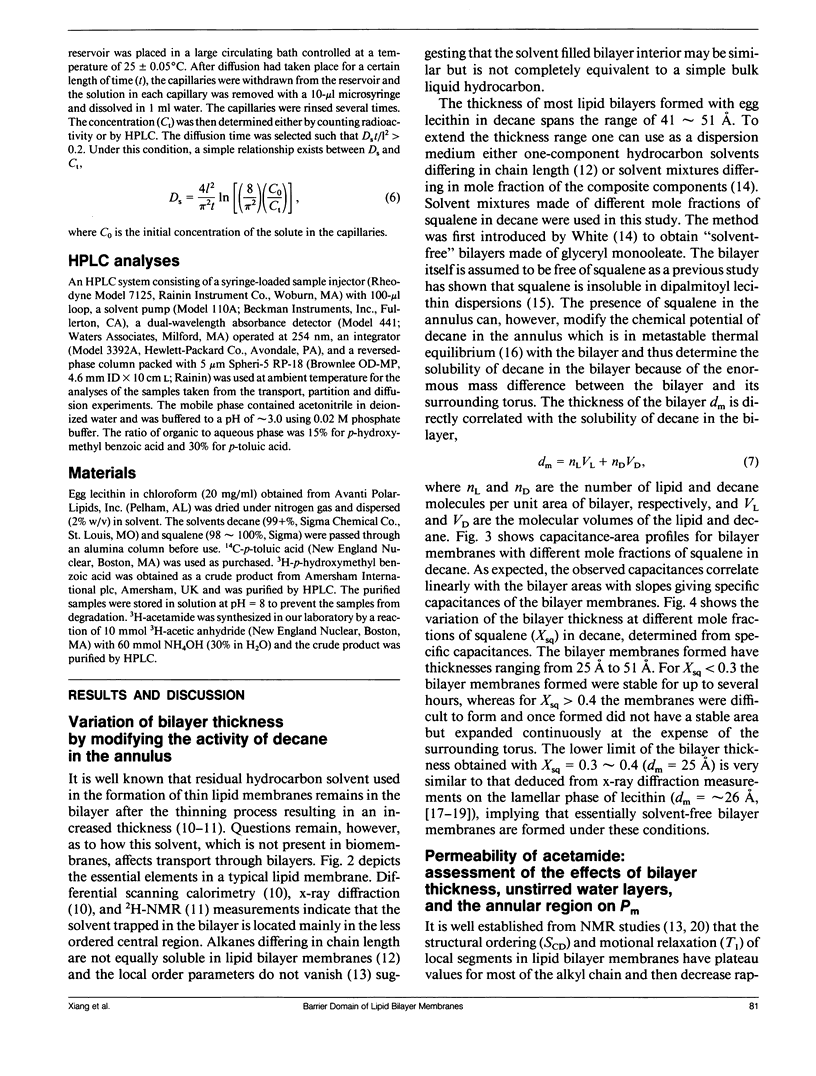
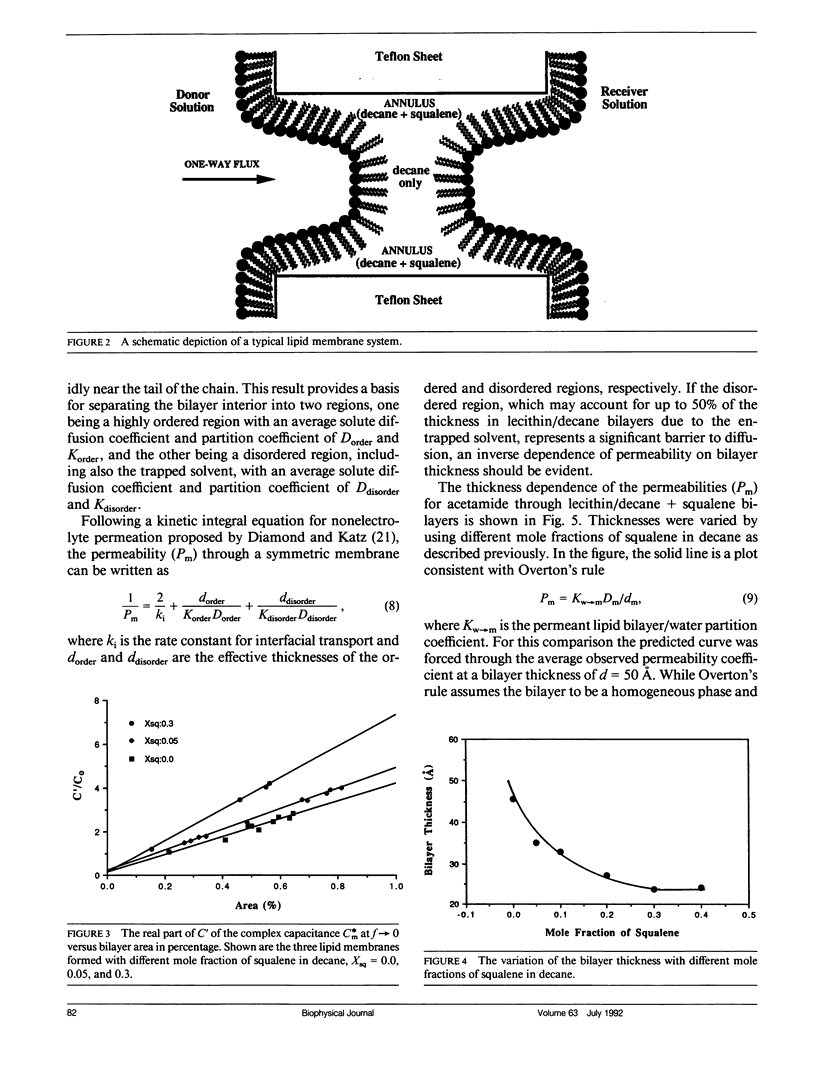
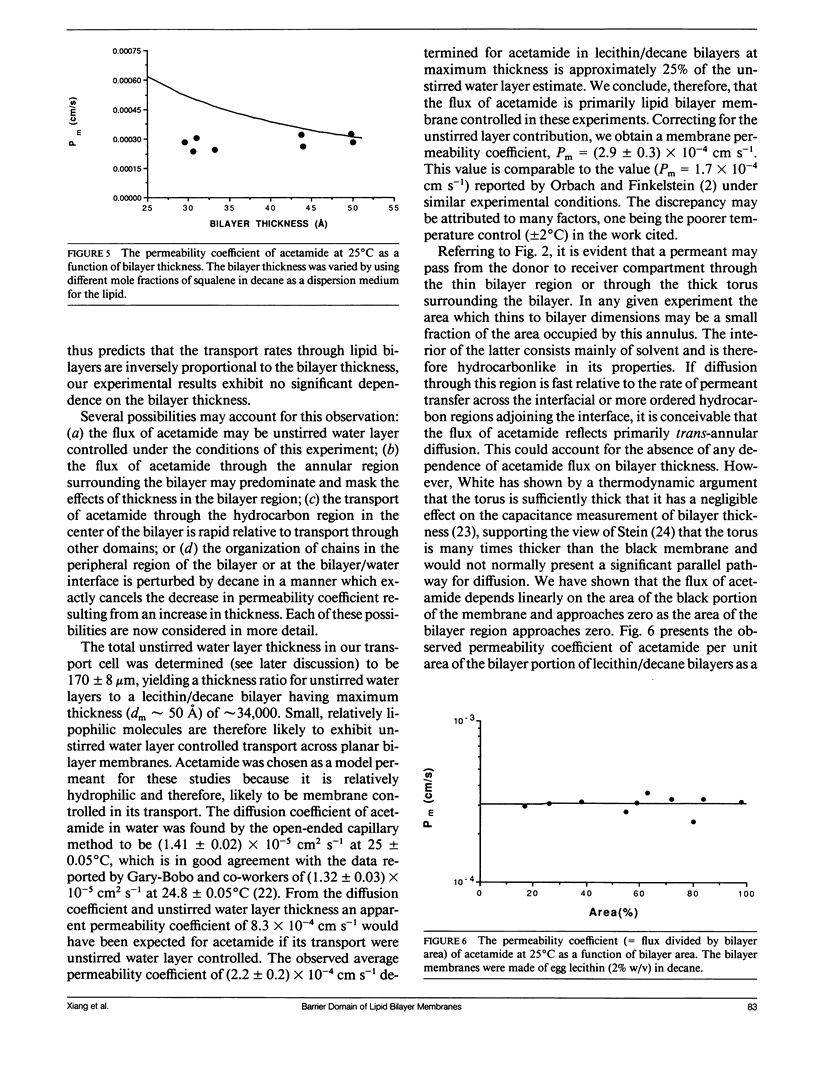
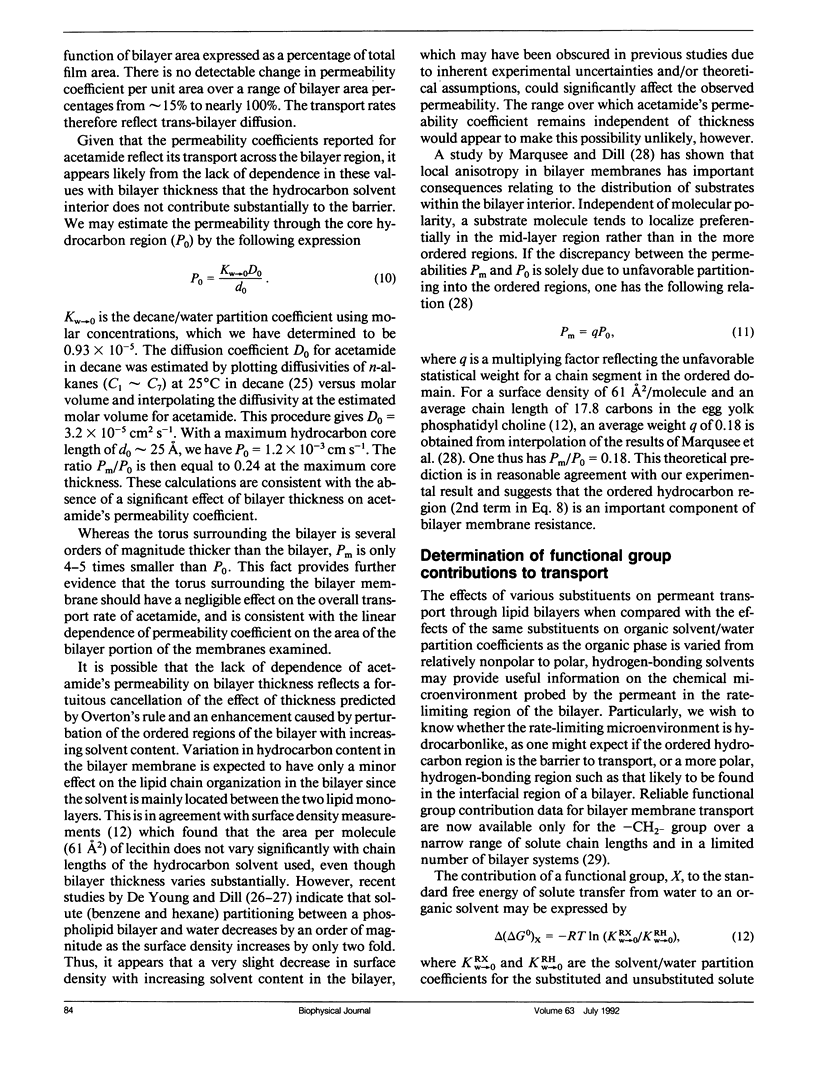
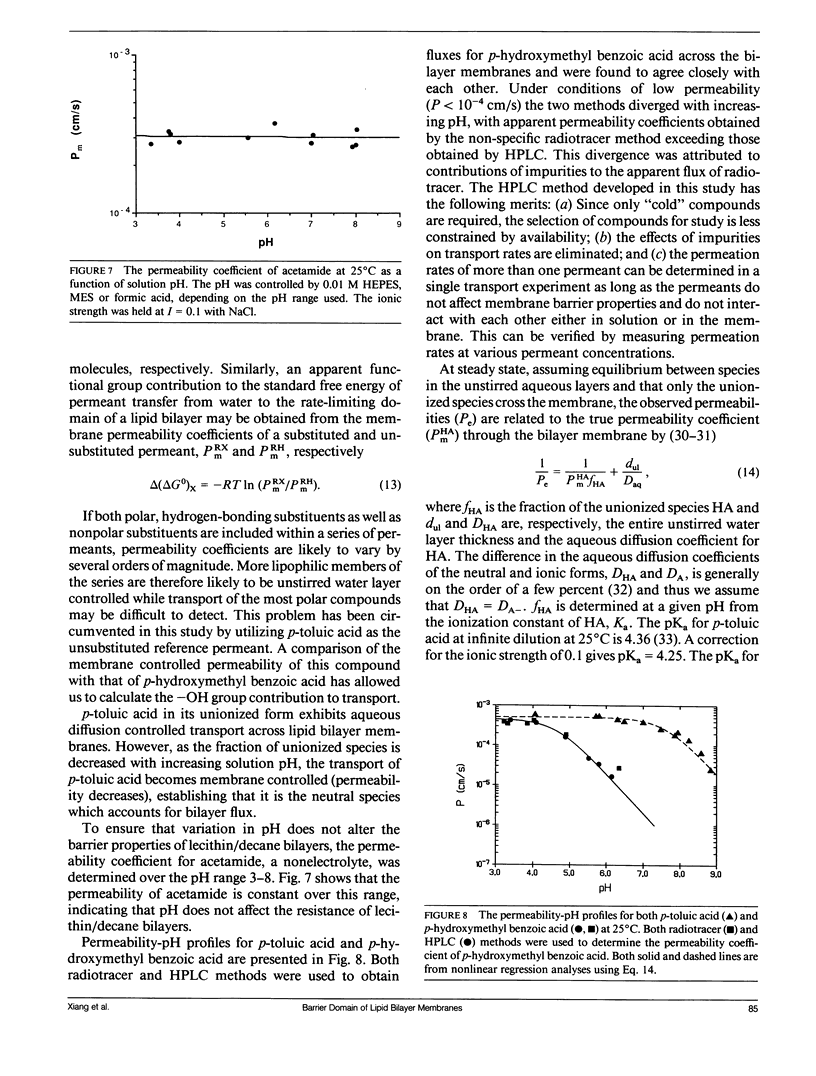
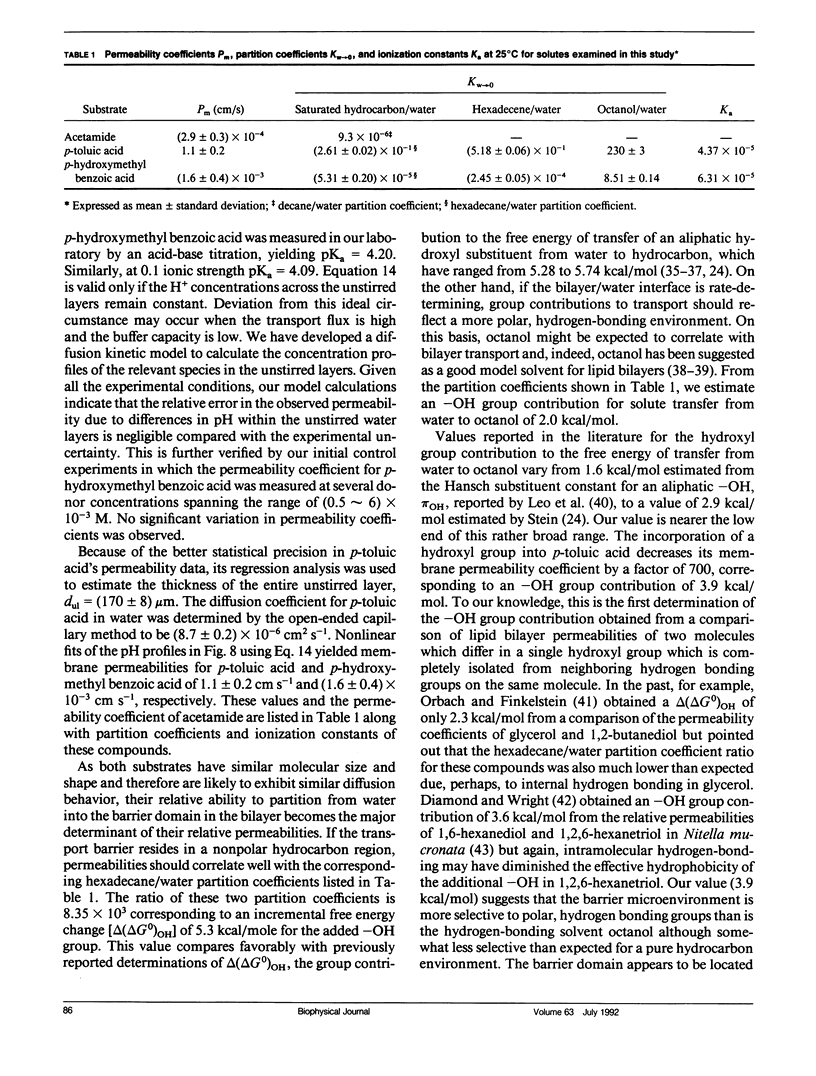
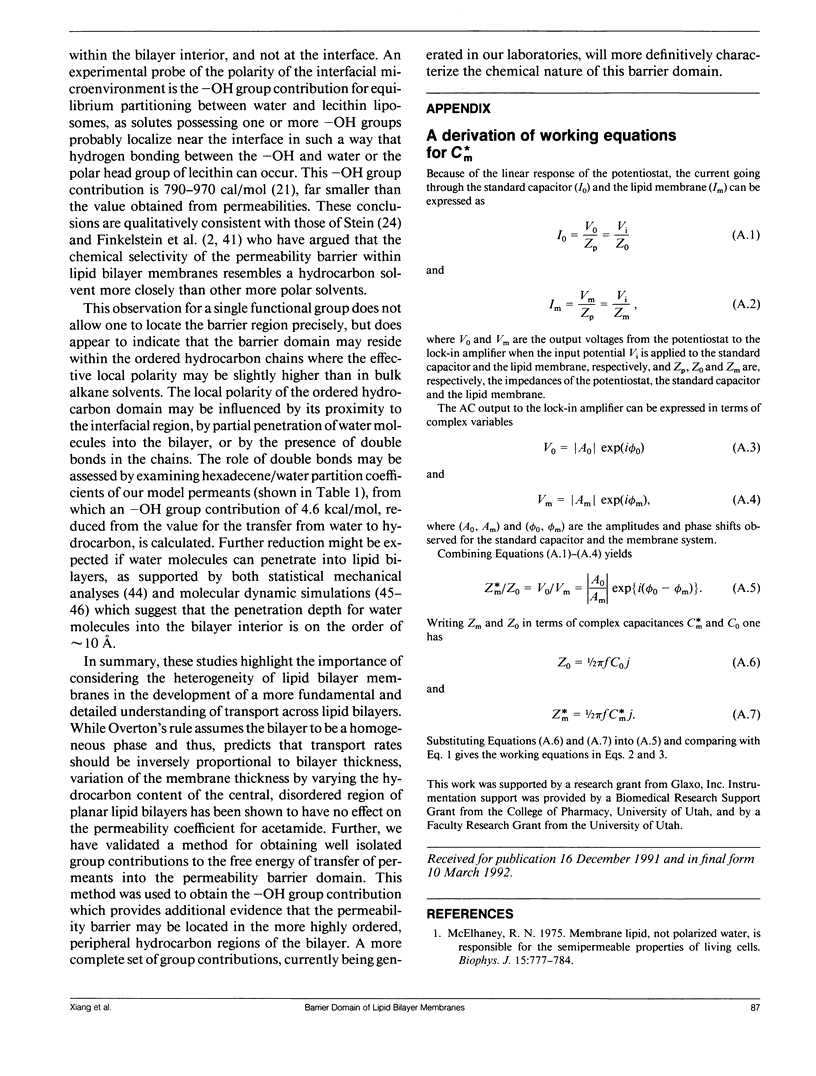
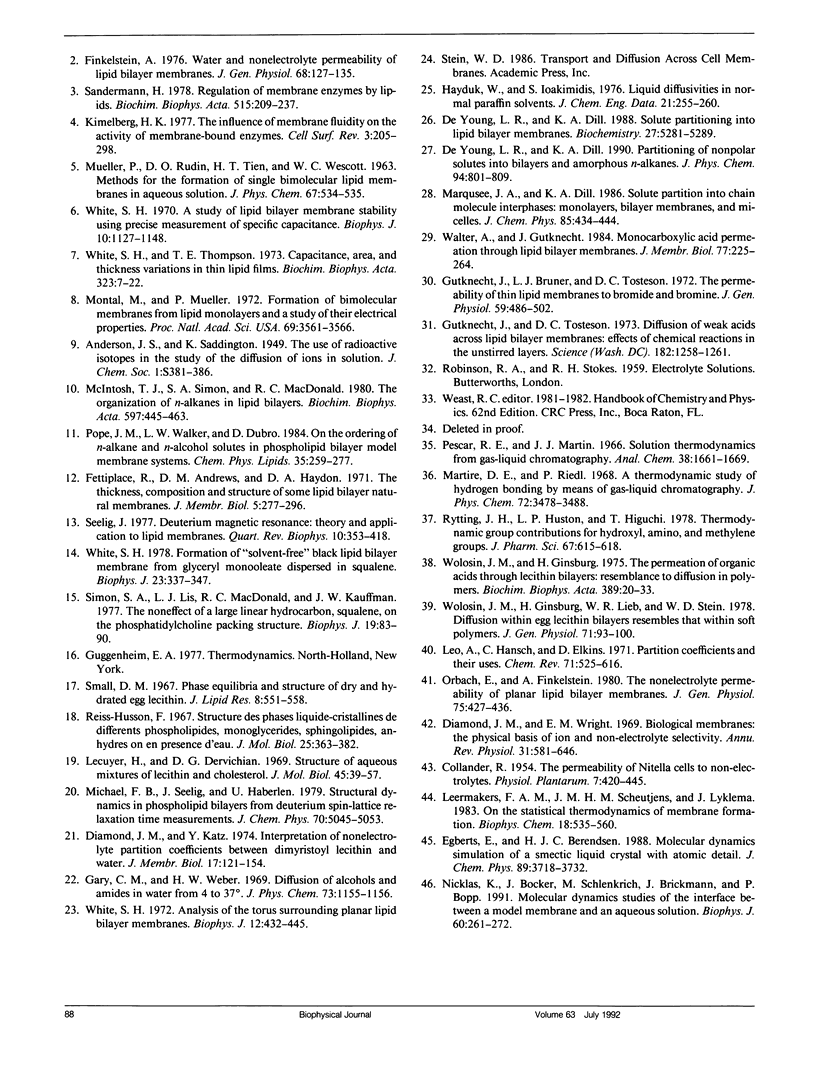
Selected References
These references are in PubMed. This may not be the complete list of references from this article.
- De Young L. R., Dill K. A. Solute partitioning into lipid bilayer membranes. Biochemistry. 1988 Jul 12;27(14):5281–5289. doi: 10.1021/bi00414a050. [DOI] [PubMed] [Google Scholar]
- Diamond J. M., Katz Y. Interpretation of nonelectrolyte partition coefficients between dimyristoyl lecithin and water. J Membr Biol. 1974;17(2):121–154. doi: 10.1007/BF01870176. [DOI] [PubMed] [Google Scholar]
- Diamond J. M., Wright E. M. Biological membranes: the physical basis of ion and nonelectrolyte selectivity. Annu Rev Physiol. 1969;31:581–646. doi: 10.1146/annurev.ph.31.030169.003053. [DOI] [PubMed] [Google Scholar]
- Finkelstein A. Water and nonelectrolyte permeability of lipid bilayer membranes. J Gen Physiol. 1976 Aug;68(2):127–135. doi: 10.1085/jgp.68.2.127. [DOI] [PMC free article] [PubMed] [Google Scholar]
- Gutknecht J., Bruner L. J., Tosteson D. C. The permeability of thin lipid membranes to bromide and bromine. J Gen Physiol. 1972 Apr;59(4):486–592. doi: 10.1085/jgp.59.4.486. [DOI] [PMC free article] [PubMed] [Google Scholar]
- Gutknecht J., Tosteson D. C. Diffusion of weak acids across lipid bilayer membranes: effects of chemical reactions in the unstirred layers. Science. 1973 Dec 21;182(4118):1258–1261. doi: 10.1126/science.182.4118.1258. [DOI] [PubMed] [Google Scholar]
- Lecuyer H., Dervichian D. G. Structure of aqueous mixtures of lecithin and cholesterol. J Mol Biol. 1969 Oct 14;45(1):39–57. doi: 10.1016/0022-2836(69)90208-3. [DOI] [PubMed] [Google Scholar]
- McElhaney R. N. Membrane lipid, not polarized water, is responsible for the semipermeable properties of living cells. Biophys J. 1975 Aug;15(8):777–784. doi: 10.1016/S0006-3495(75)85854-1. [DOI] [PMC free article] [PubMed] [Google Scholar]
- McIntosh T. J., Simon S. A., MacDonald R. C. The organization of n-alkanes in lipid bilayers. Biochim Biophys Acta. 1980 Apr 24;597(3):445–463. doi: 10.1016/0005-2736(80)90219-9. [DOI] [PubMed] [Google Scholar]
- Montal M., Mueller P. Formation of bimolecular membranes from lipid monolayers and a study of their electrical properties. Proc Natl Acad Sci U S A. 1972 Dec;69(12):3561–3566. doi: 10.1073/pnas.69.12.3561. [DOI] [PMC free article] [PubMed] [Google Scholar]
- Nicklas K., Böcker J., Schlenkrich M., Brickmann J., Bopp P. Molecular dynamics studies of the interface between a model membrane and an aqueous solution. Biophys J. 1991 Jul;60(1):261–272. doi: 10.1016/S0006-3495(91)82048-3. [DOI] [PMC free article] [PubMed] [Google Scholar]
- Orbach E., Finkelstein A. The nonelectrolyte permeability of planar lipid bilayer membranes. J Gen Physiol. 1980 Apr;75(4):427–436. doi: 10.1085/jgp.75.4.427. [DOI] [PMC free article] [PubMed] [Google Scholar]
- Reiss-Husson F. Structure des phases liquide-cristallines de différents phospholipides, monoglycérides, sphingolipides, anhydres ou en présence d'eau. J Mol Biol. 1967 May 14;25(3):363–382. doi: 10.1016/0022-2836(67)90192-1. [DOI] [PubMed] [Google Scholar]
- Rytting J. H., Huston L. P., Higuchi T. Thermodynamic group contributions for hydroxyl, amino, and methylene groups. J Pharm Sci. 1978 May;67(5):615–618. doi: 10.1002/jps.2600670510. [DOI] [PubMed] [Google Scholar]
- Sandermann H., Jr Regulation of membrane enzymes by lipids. Biochim Biophys Acta. 1978 Sep 29;515(3):209–237. doi: 10.1016/0304-4157(78)90015-1. [DOI] [PubMed] [Google Scholar]
- Seelig J. Deuterium magnetic resonance: theory and application to lipid membranes. Q Rev Biophys. 1977 Aug;10(3):353–418. doi: 10.1017/s0033583500002948. [DOI] [PubMed] [Google Scholar]
- Simon S. A., Lis L. J., MacDonald R. C., Kauffman J. W. The noneffect of a large linear hydrocarbon, squalene, on the phosphatidylcholine packing structure. Biophys J. 1977 Jul;19(1):83–90. doi: 10.1016/S0006-3495(77)85570-7. [DOI] [PMC free article] [PubMed] [Google Scholar]
- Small D. M. Phase equilibria and structure of dry and hydrated egg lecithin. J Lipid Res. 1967 Nov;8(6):551–557. [PubMed] [Google Scholar]
- Walter A., Gutknecht J. Monocarboxylic acid permeation through lipid bilayer membranes. J Membr Biol. 1984;77(3):255–264. doi: 10.1007/BF01870573. [DOI] [PubMed] [Google Scholar]
- White S. H. A study of lipid bilayer membrane stability using precise measurements of specific capacitance. Biophys J. 1970 Dec;10(12):1127–1148. doi: 10.1016/S0006-3495(70)86360-3. [DOI] [PMC free article] [PubMed] [Google Scholar]
- White S. H. Analysis of the torus surrounding planar lipid bilayer membranes. Biophys J. 1972 Apr;12(4):432–445. doi: 10.1016/S0006-3495(72)86095-8. [DOI] [PMC free article] [PubMed] [Google Scholar]
- White S. H. Formation of "solvent-free" black lipid bilayer membranes from glyceryl monooleate dispersed in squalene. Biophys J. 1978 Sep;23(3):337–347. doi: 10.1016/S0006-3495(78)85453-8. [DOI] [PMC free article] [PubMed] [Google Scholar]
- White S. H., Thompson T. E. Capacitance, area, and thickness variations in thin lipid films. Biochim Biophys Acta. 1973 Sep 27;323(1):7–22. doi: 10.1016/0005-2736(73)90428-8. [DOI] [PubMed] [Google Scholar]
- Wolosin J. M., Ginsburg H., Lieb W. R., Stein W. D. Diffusion within egg lecithin bilayers resembles that within soft polymers. J Gen Physiol. 1978 Jan;71(1):93–100. doi: 10.1085/jgp.71.1.93. [DOI] [PMC free article] [PubMed] [Google Scholar]
- Wolosin J. M., Ginsburg H. The permeation of organic acids through lecithin bilayers. Resemblance to diffusion in polymers. Biochim Biophys Acta. 1975 Apr 21;389(1):20–33. doi: 10.1016/0005-2736(75)90382-x. [DOI] [PubMed] [Google Scholar]