Abstract
Recently, it has become clear that the influenza virus fusion protein, hemagglutinin (HA), produces membrane destabilization and fusion by a multistep process, which involves the aggregation of the HAs to form a fusion site. While the details of this process are under debate, it is important to recognize that proposing any sequence of "microscopic" fusion intermediates encumbers general "macroscopic" kinetic consequences, i.e., with respect to membrane mixing rates. Using a kinetic scheme which incorporates the essential elements of several recently proposed models, some of these measurable properties have been elucidated. First, a rigorous mathematical relationship between fusion intermediates and the fusion event itself is defined. Second, it is shown that what is measured as the macroscopic "fusion rate constant" is a simple function of all of the rate constants governing the transitions between intermediates, whether or not one of the microscopic steps is rate limiting. Third, while this kinetic scheme predicts a delay (or lag) time for fusion, as has been observed, it will be very difficult to extract reliable microscopic information from these data. Furthermore, it is predicted that the delay time can depend upon HA surface density even when the HA aggregation step is very rapid compared with fusion, i.e., the delay time need not be due to HA aggregation. Fourth, the inactivation process observed for influenza virions at low pH can be described within this kinetic scheme simply, yet rigorously, via the loss of the fusion intermediates. Fifth, predicted Arrhenius plots of fusion rates can be linear for this multistep scheme, even though there is no single rate-determining step and even when a branched step is introduced, i.e., where one pathway predominates at low temperature and the other pathway predominates at high temperature. Furthermore, the apparent activation energies obtained from these plots bear little or no quantitative resemblance to the microscopic activation energies used to simulate the data. Overall, these results clearly show that the intermediates of protein mediated fusion can be studied only by using assays sensitive to the formation of each proposed intermediate.
Full text
PDF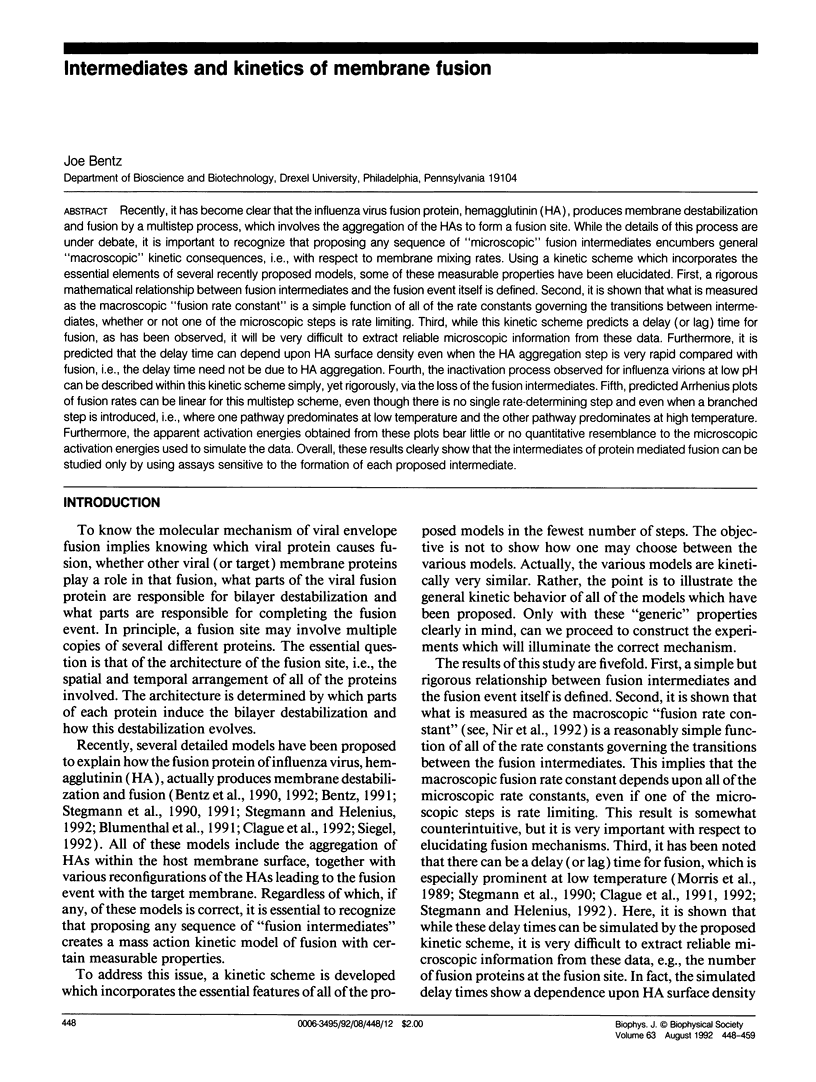
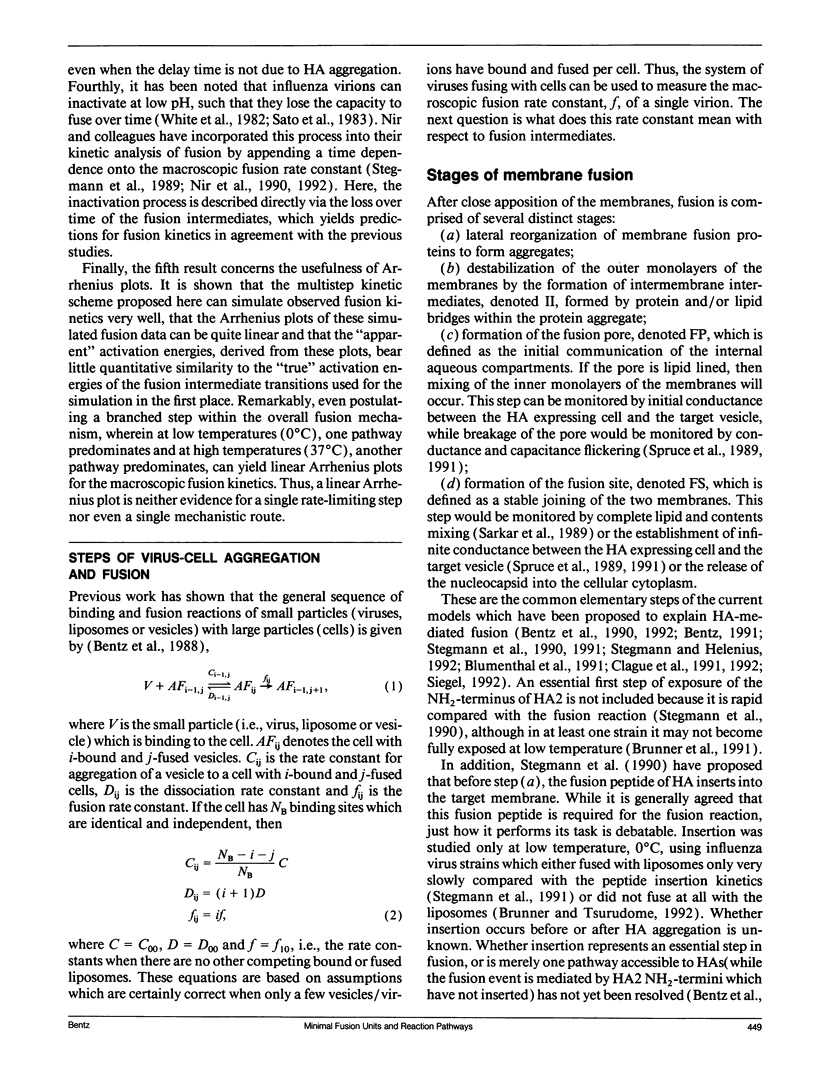
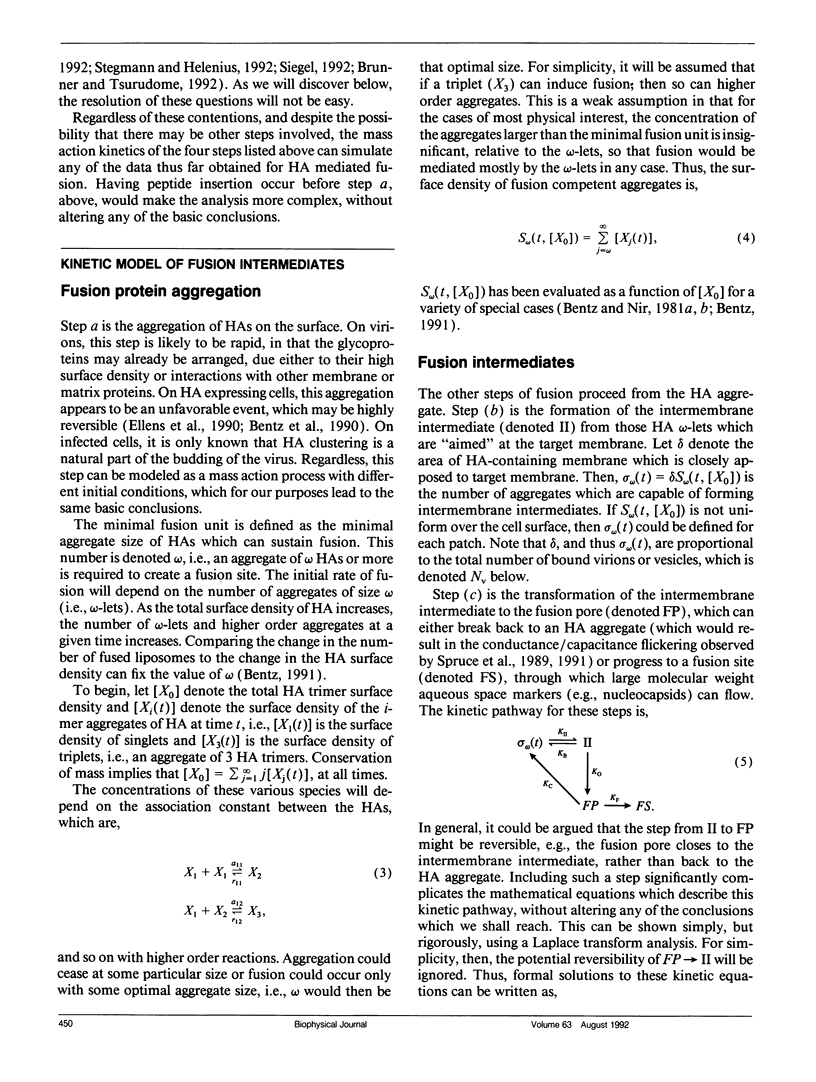
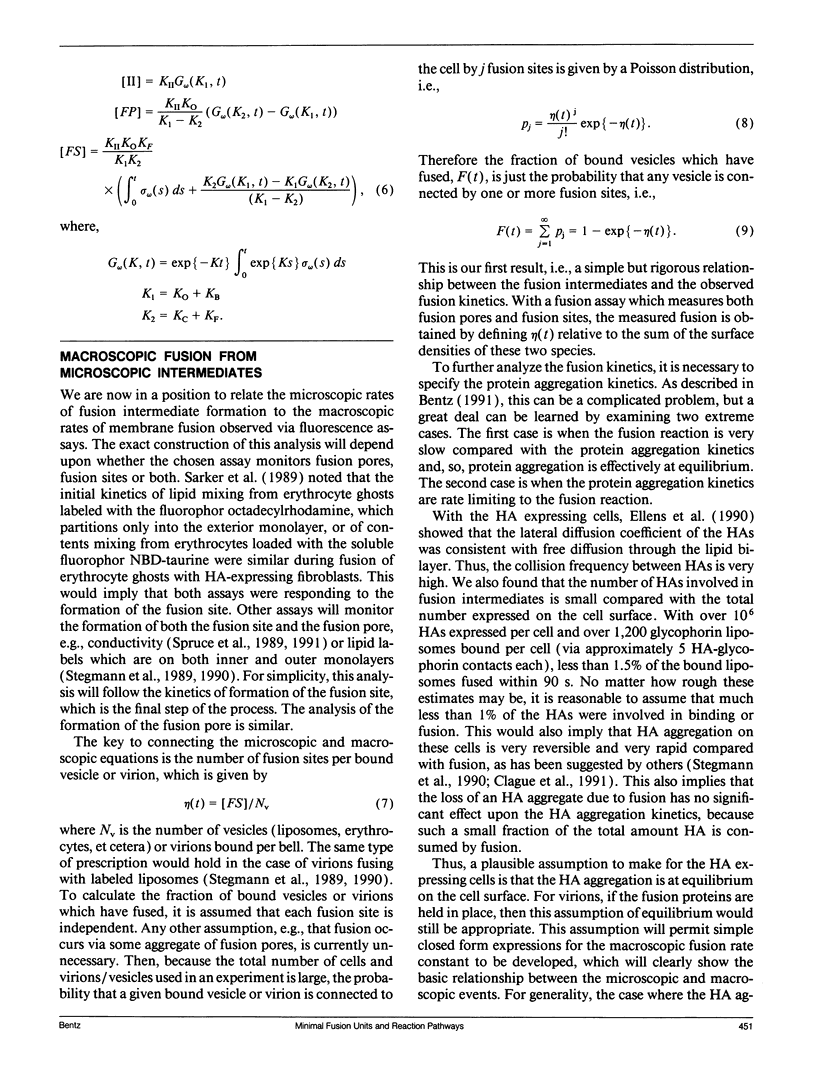
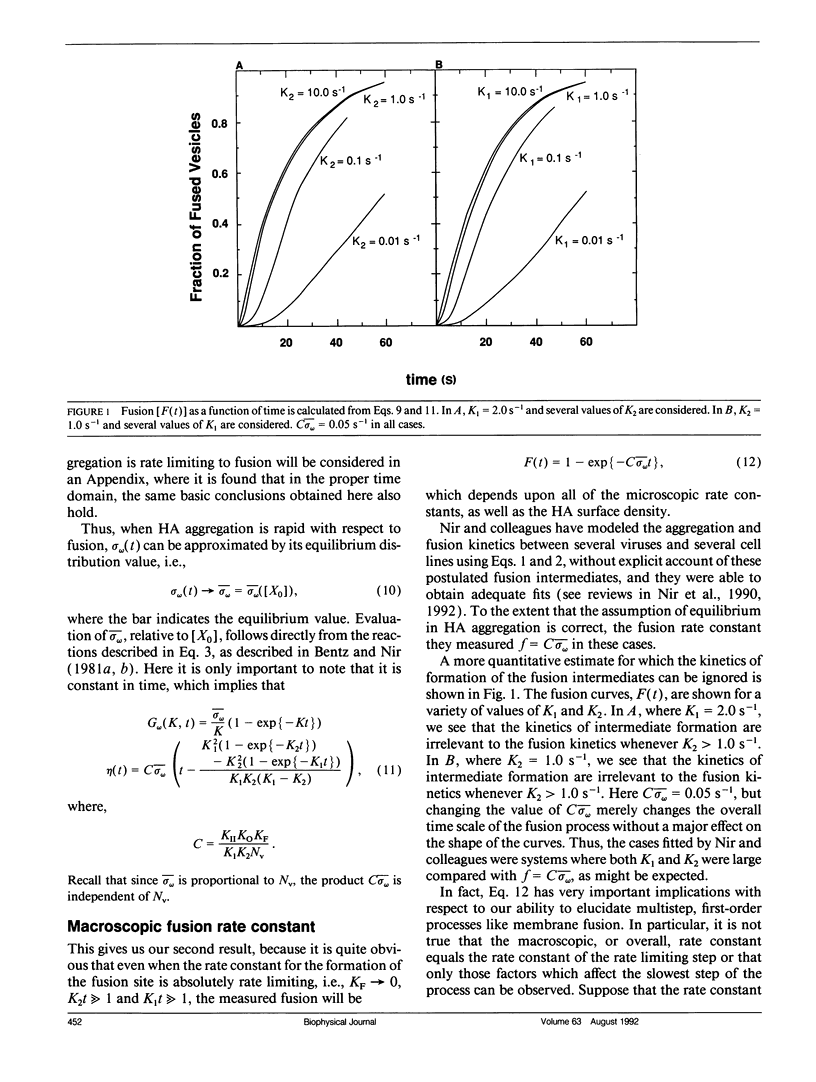
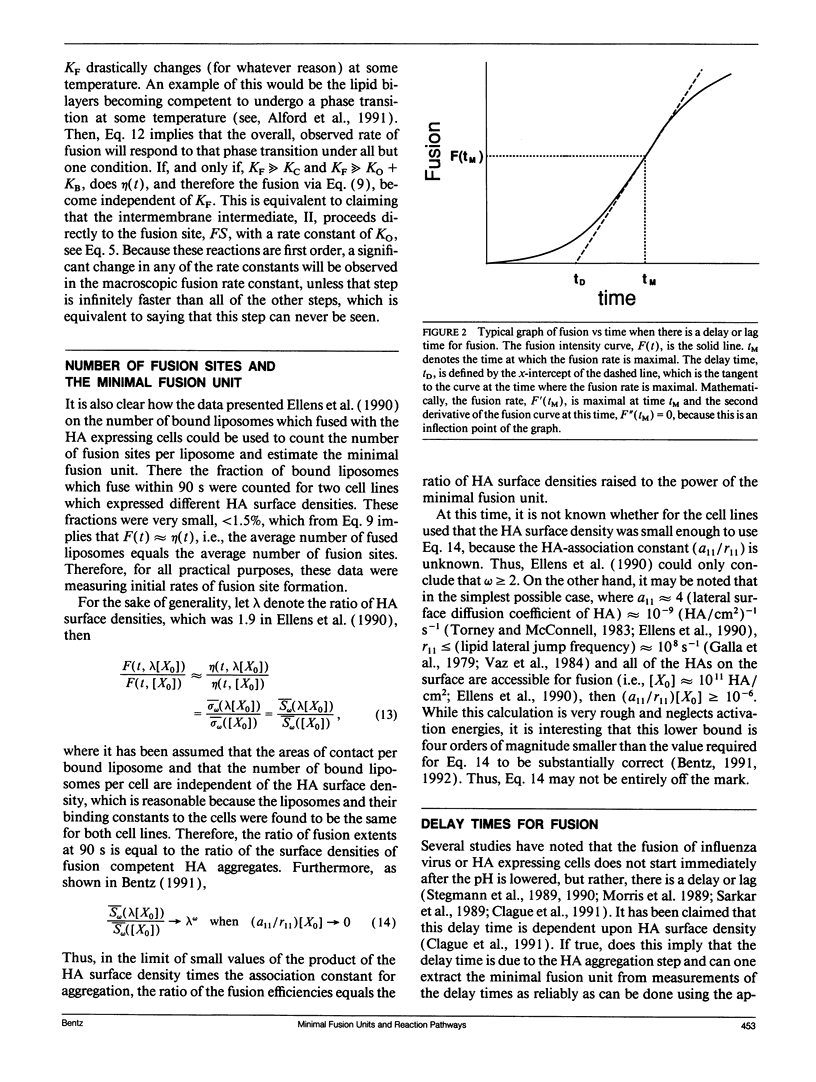
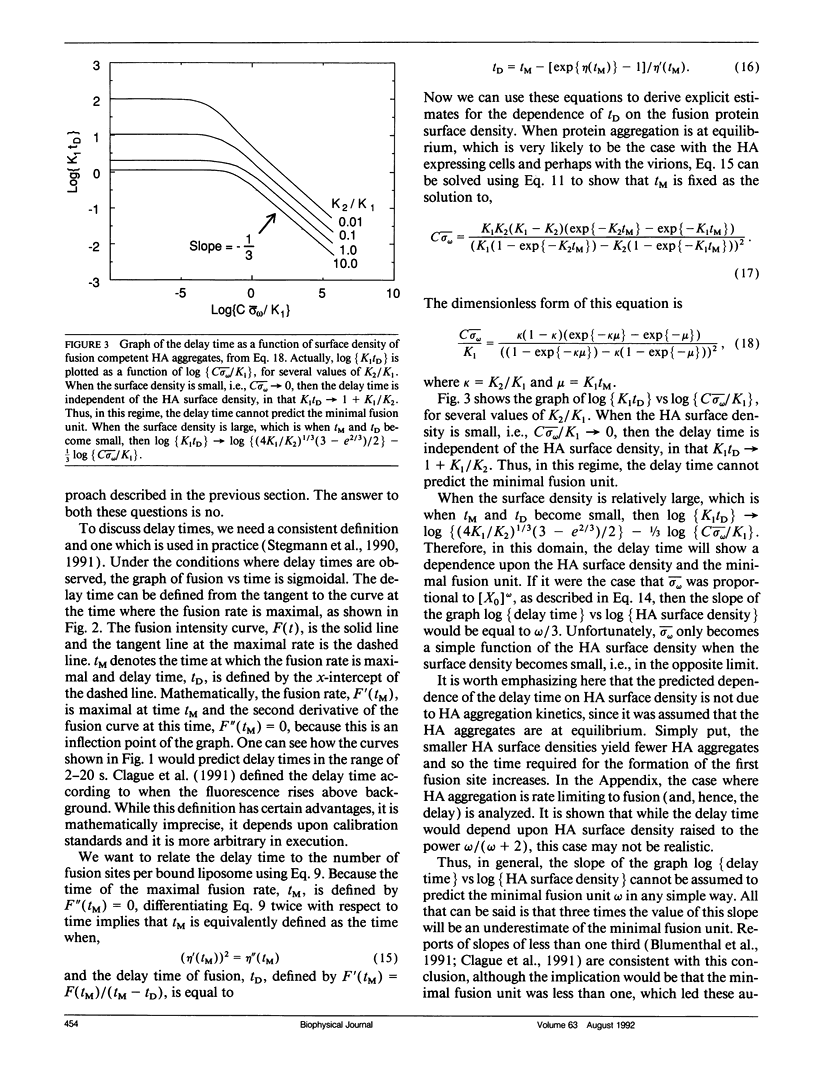
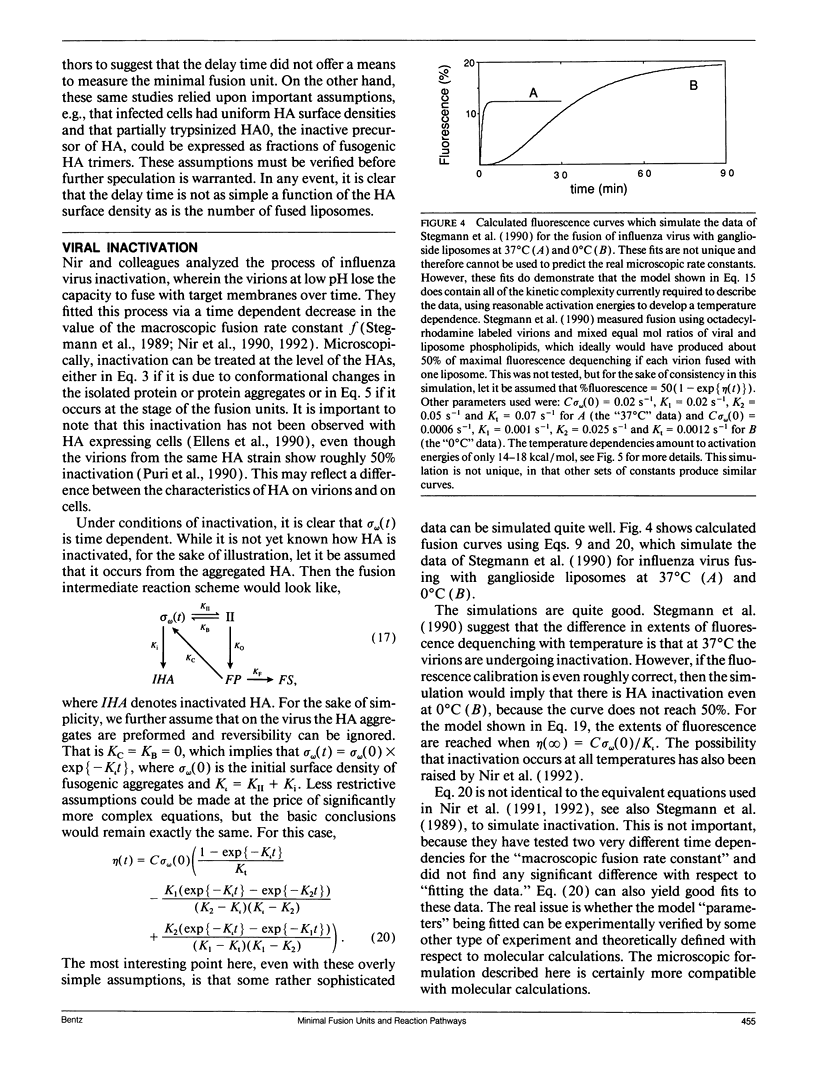
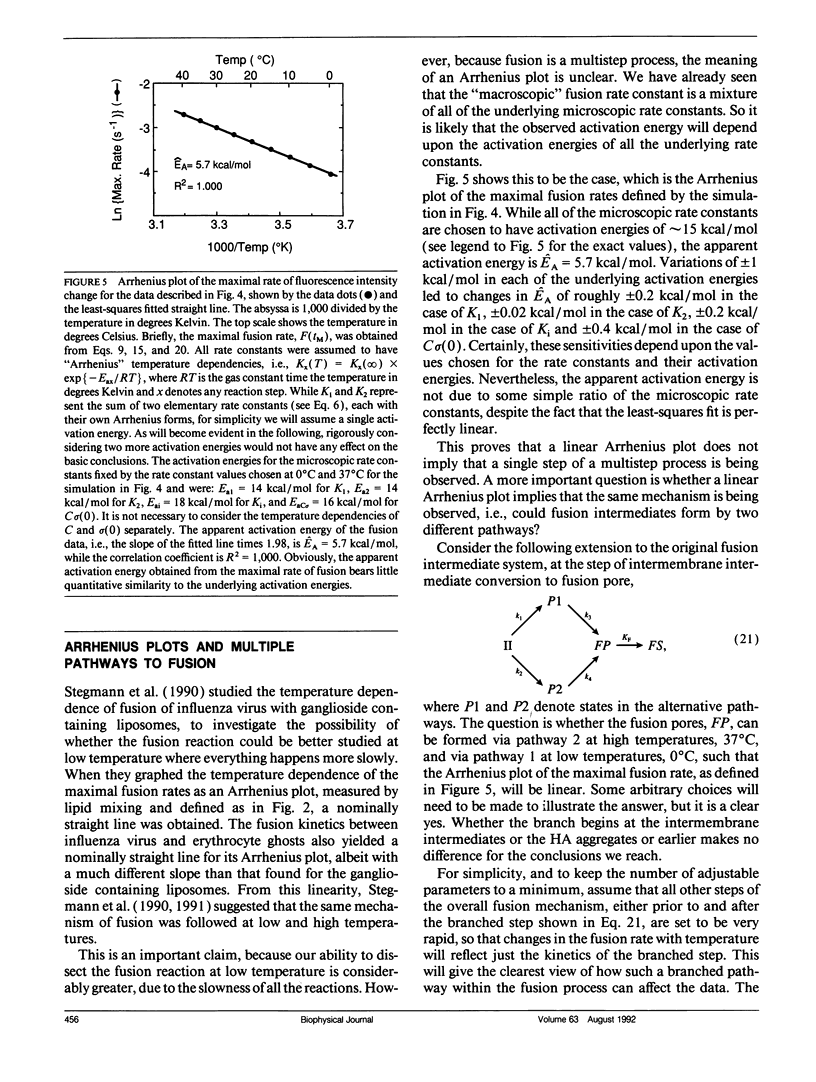
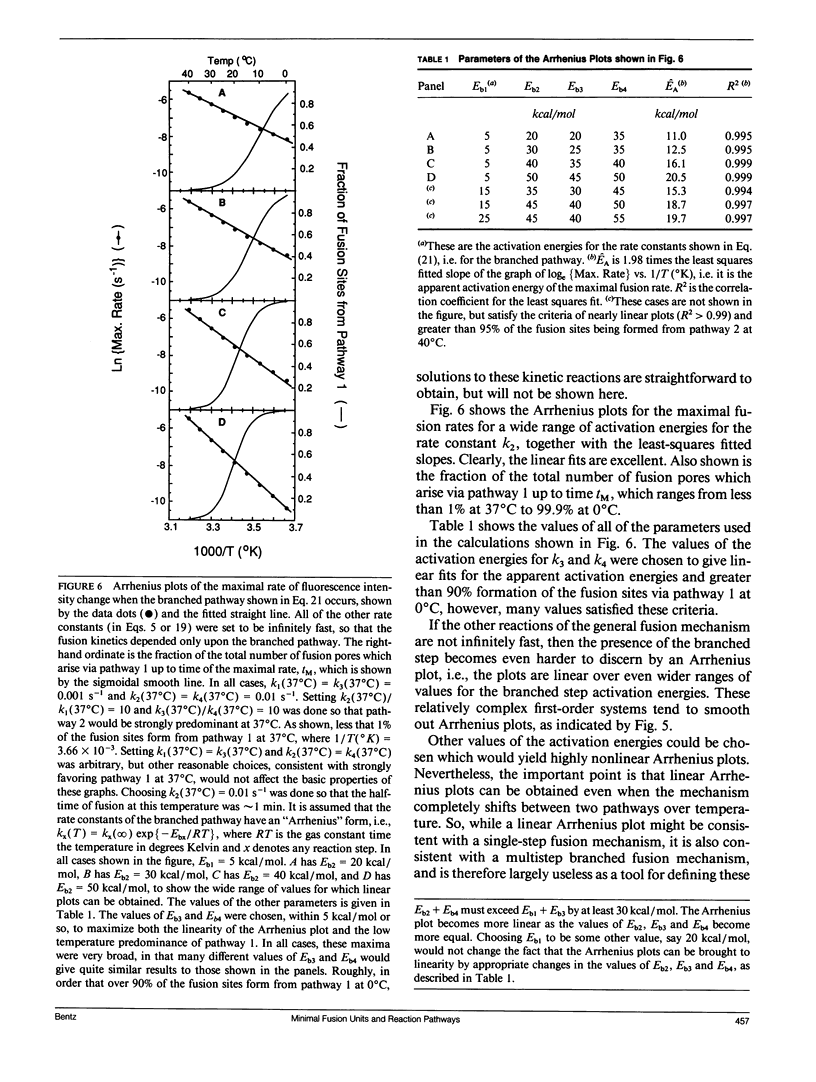
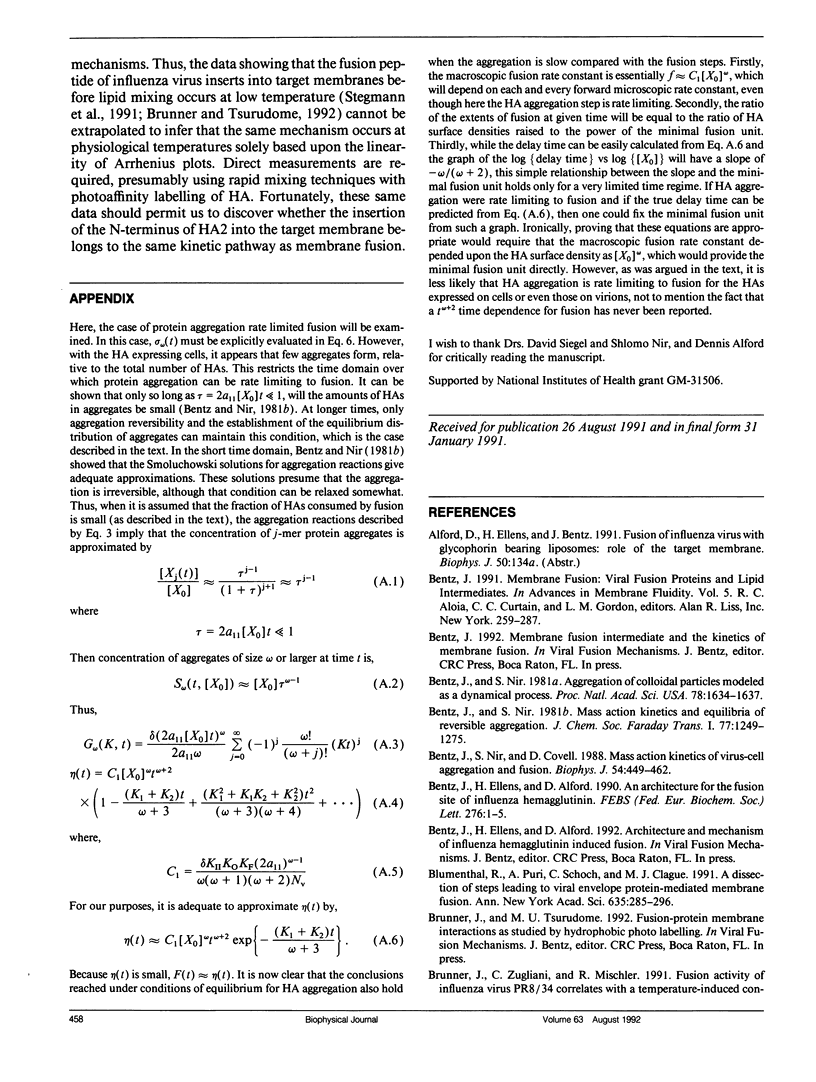
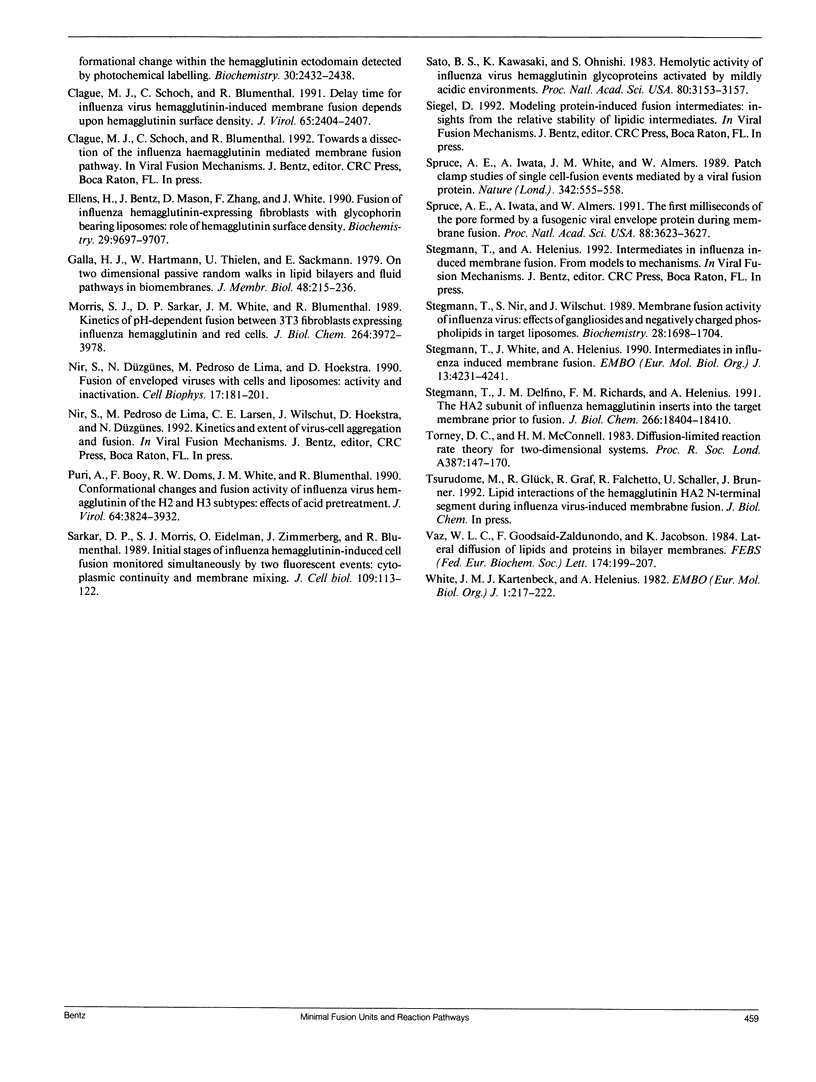
Selected References
These references are in PubMed. This may not be the complete list of references from this article.
- Bentz J., Nir S. Aggregation of colloidal particles modeled as a dynamical process. Proc Natl Acad Sci U S A. 1981 Mar;78(3):1634–1637. doi: 10.1073/pnas.78.3.1634. [DOI] [PMC free article] [PubMed] [Google Scholar]
- Bentz J., Nir S., Covell D. G. Mass action kinetics of virus-cell aggregation and fusion. Biophys J. 1988 Sep;54(3):449–462. doi: 10.1016/S0006-3495(88)82978-3. [DOI] [PMC free article] [PubMed] [Google Scholar]
- Blumenthal R., Schoch C., Puri A., Clague M. J. A dissection of steps leading to viral envelope protein-mediated membrane fusion. Ann N Y Acad Sci. 1991;635:285–296. doi: 10.1111/j.1749-6632.1991.tb36499.x. [DOI] [PubMed] [Google Scholar]
- Brunner J., Zugliani C., Mischler R. Fusion activity of influenza virus PR8/34 correlates with a temperature-induced conformational change within the hemagglutinin ectodomain detected by photochemical labeling. Biochemistry. 1991 Mar 5;30(9):2432–2438. doi: 10.1021/bi00223a019. [DOI] [PubMed] [Google Scholar]
- Clague M. J., Schoch C., Blumenthal R. Delay time for influenza virus hemagglutinin-induced membrane fusion depends on hemagglutinin surface density. J Virol. 1991 May;65(5):2402–2407. doi: 10.1128/jvi.65.5.2402-2407.1991. [DOI] [PMC free article] [PubMed] [Google Scholar]
- Ellens H., Bentz J., Mason D., Zhang F., White J. M. Fusion of influenza hemagglutinin-expressing fibroblasts with glycophorin-bearing liposomes: role of hemagglutinin surface density. Biochemistry. 1990 Oct 16;29(41):9697–9707. doi: 10.1021/bi00493a027. [DOI] [PubMed] [Google Scholar]
- Galla H. J., Hartmann W., Theilen U., Sackmann E. On two-dimensional passive random walk in lipid bilayers and fluid pathways in biomembranes. J Membr Biol. 1979 Jul 31;48(3):215–236. doi: 10.1007/BF01872892. [DOI] [PubMed] [Google Scholar]
- Morris S. J., Sarkar D. P., White J. M., Blumenthal R. Kinetics of pH-dependent fusion between 3T3 fibroblasts expressing influenza hemagglutinin and red blood cells. Measurement by dequenching of fluorescence. J Biol Chem. 1989 Mar 5;264(7):3972–3978. [PubMed] [Google Scholar]
- Nir S., Düzgünes N., de Lima M. C., Hoekstra D. Fusion of enveloped viruses with cells and liposomes. Activity and inactivation. Cell Biophys. 1990 Oct;17(2):181–201. doi: 10.1007/BF02990496. [DOI] [PubMed] [Google Scholar]
- Puri A., Booy F. P., Doms R. W., White J. M., Blumenthal R. Conformational changes and fusion activity of influenza virus hemagglutinin of the H2 and H3 subtypes: effects of acid pretreatment. J Virol. 1990 Aug;64(8):3824–3832. doi: 10.1128/jvi.64.8.3824-3832.1990. [DOI] [PMC free article] [PubMed] [Google Scholar]
- Sarkar D. P., Morris S. J., Eidelman O., Zimmerberg J., Blumenthal R. Initial stages of influenza hemagglutinin-induced cell fusion monitored simultaneously by two fluorescent events: cytoplasmic continuity and lipid mixing. J Cell Biol. 1989 Jul;109(1):113–122. doi: 10.1083/jcb.109.1.113. [DOI] [PMC free article] [PubMed] [Google Scholar]
- Sato S. B., Kawasaki K., Ohnishi S. Hemolytic activity of influenza virus hemagglutinin glycoproteins activated in mildly acidic environments. Proc Natl Acad Sci U S A. 1983 Jun;80(11):3153–3157. doi: 10.1073/pnas.80.11.3153. [DOI] [PMC free article] [PubMed] [Google Scholar]
- Spruce A. E., Iwata A., Almers W. The first milliseconds of the pore formed by a fusogenic viral envelope protein during membrane fusion. Proc Natl Acad Sci U S A. 1991 May 1;88(9):3623–3627. doi: 10.1073/pnas.88.9.3623. [DOI] [PMC free article] [PubMed] [Google Scholar]
- Spruce A. E., Iwata A., White J. M., Almers W. Patch clamp studies of single cell-fusion events mediated by a viral fusion protein. Nature. 1989 Nov 30;342(6249):555–558. doi: 10.1038/342555a0. [DOI] [PubMed] [Google Scholar]
- Stegmann T., Delfino J. M., Richards F. M., Helenius A. The HA2 subunit of influenza hemagglutinin inserts into the target membrane prior to fusion. J Biol Chem. 1991 Sep 25;266(27):18404–18410. [PubMed] [Google Scholar]
- Stegmann T., Nir S., Wilschut J. Membrane fusion activity of influenza virus. Effects of gangliosides and negatively charged phospholipids in target liposomes. Biochemistry. 1989 Feb 21;28(4):1698–1704. doi: 10.1021/bi00430a041. [DOI] [PubMed] [Google Scholar]
- Stegmann T., White J. M., Helenius A. Intermediates in influenza induced membrane fusion. EMBO J. 1990 Dec;9(13):4231–4241. doi: 10.1002/j.1460-2075.1990.tb07871.x. [DOI] [PMC free article] [PubMed] [Google Scholar]
- White J., Kartenbeck J., Helenius A. Membrane fusion activity of influenza virus. EMBO J. 1982;1(2):217–222. doi: 10.1002/j.1460-2075.1982.tb01150.x. [DOI] [PMC free article] [PubMed] [Google Scholar]