Abstract
We investigated the mechanism of the Ca2+ sensitivity of cross-bridge transitions that limit the rate of force development in vertebrate skeletal muscle. The rate of force development increases with Ca2+ concentration in the physiological range. We show here that at low concentrations of Ca2+ the rate of force development increases after partial extraction of the 20-kD light chain 2 subunit of myosin, whereas reconstitution with light chain 2 fully restores native sensitivity to Ca2+ in skinned single skeletal fibers. Furthermore, elevated free Mg2+ concentration reduces Ca2+ sensitivity, an effect that is reversed by extraction of the light chain but not by disruption of thin-filament activation by partial removal of troponin C, the Ca2+ binding protein of the thin filament. Our findings indicate that the Ca2+ sensitivity of the rate of force development in vertebrate skeletal muscle is mediated in part by the light chain 2 subunit of the myosin cross-bridge.
Full text
PDF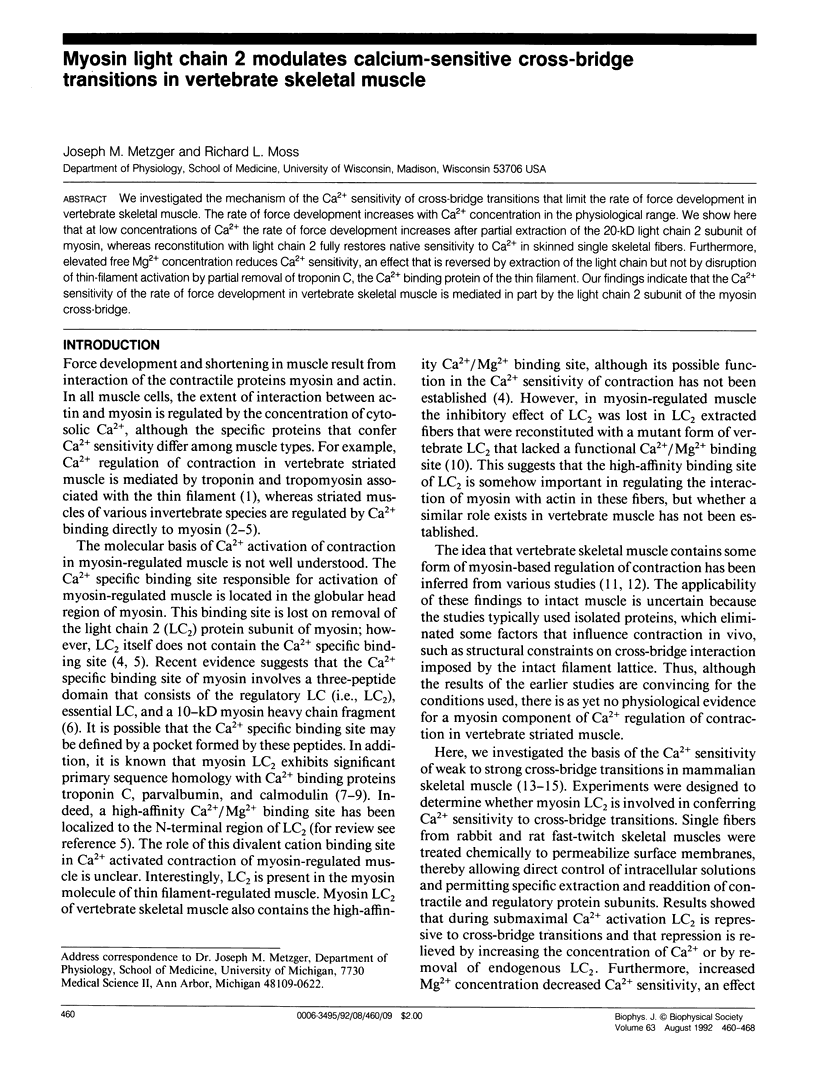
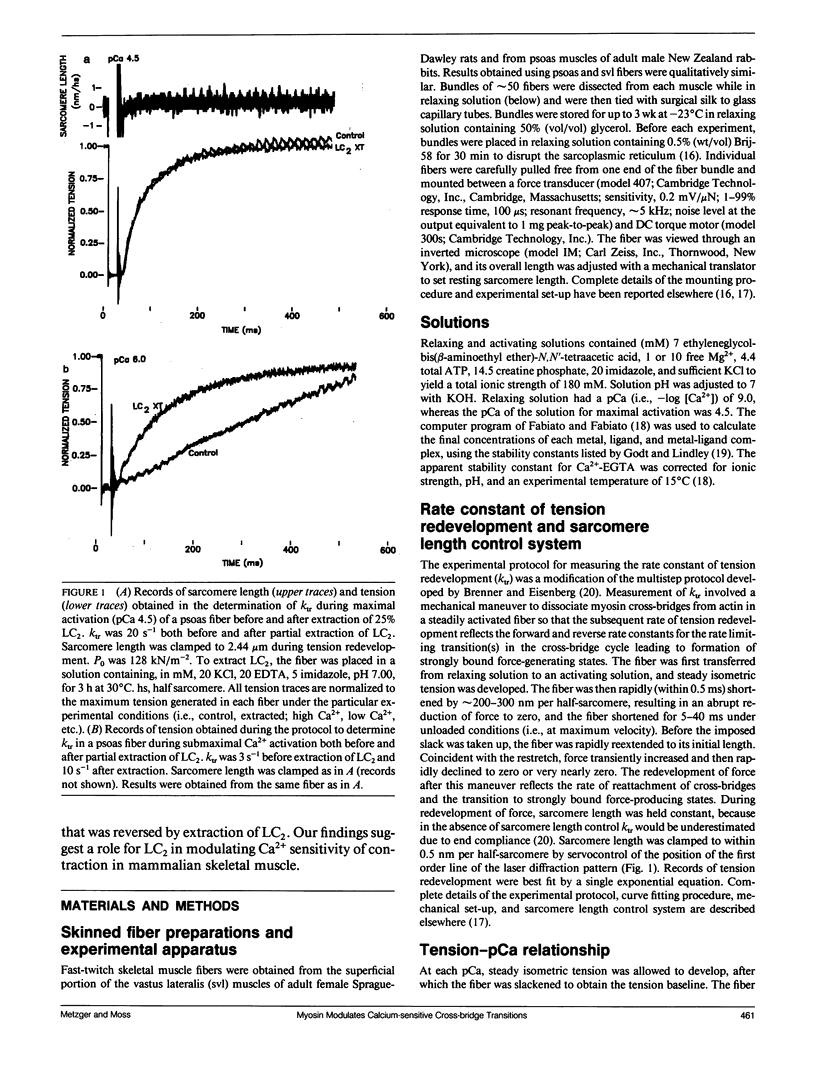
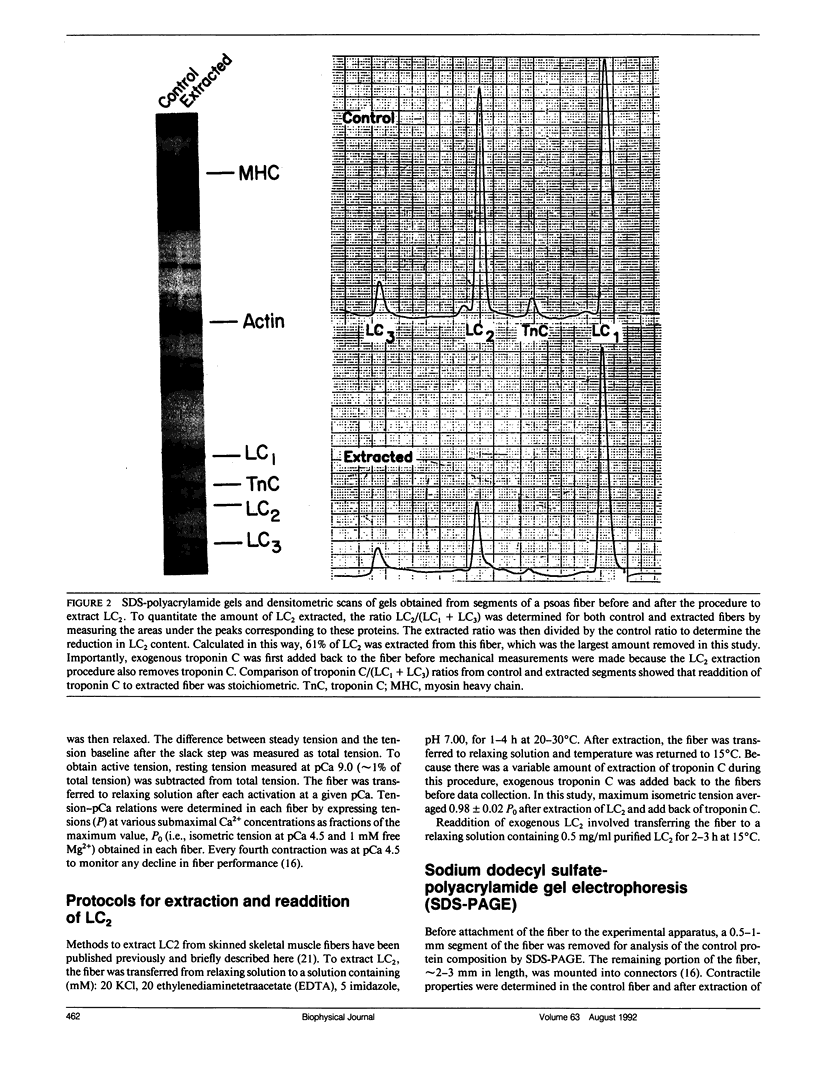
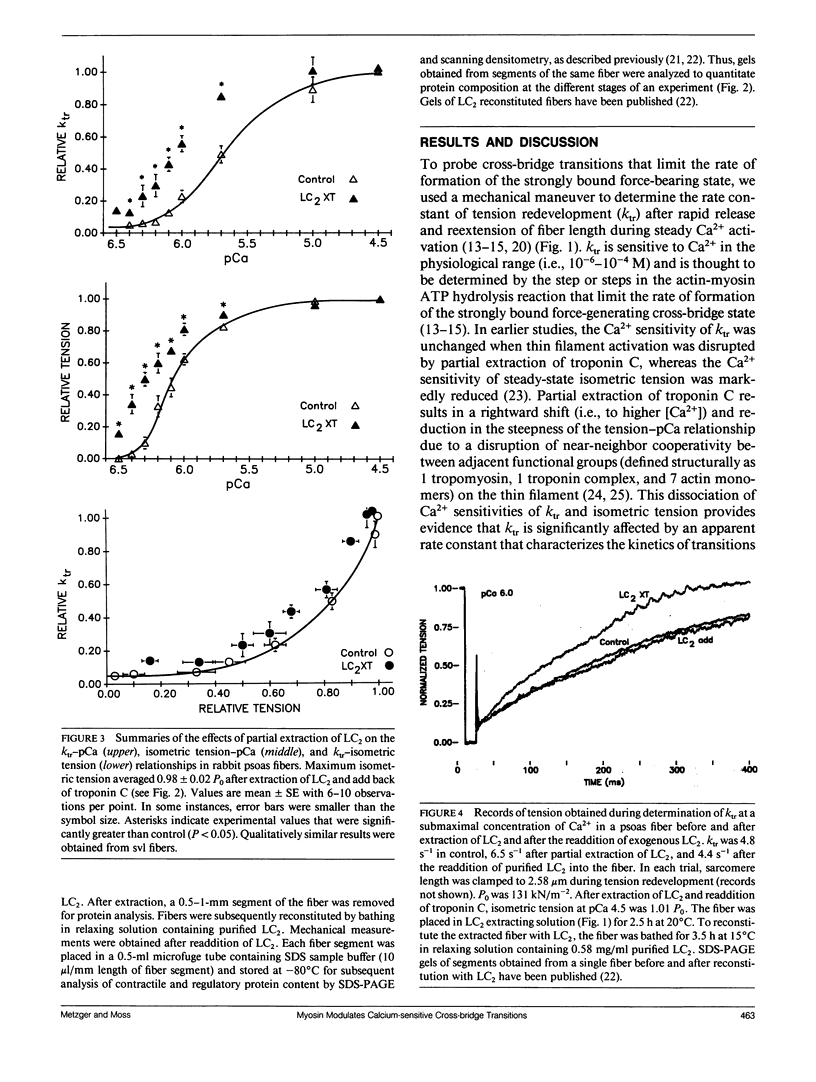
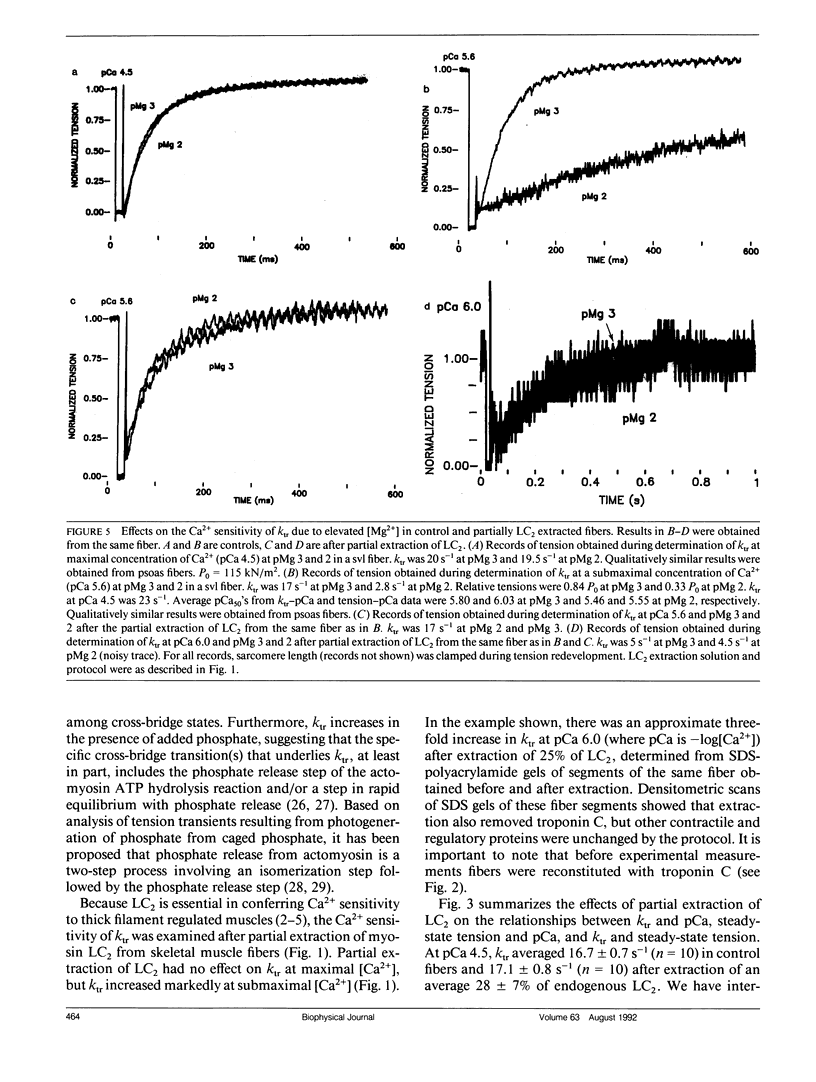
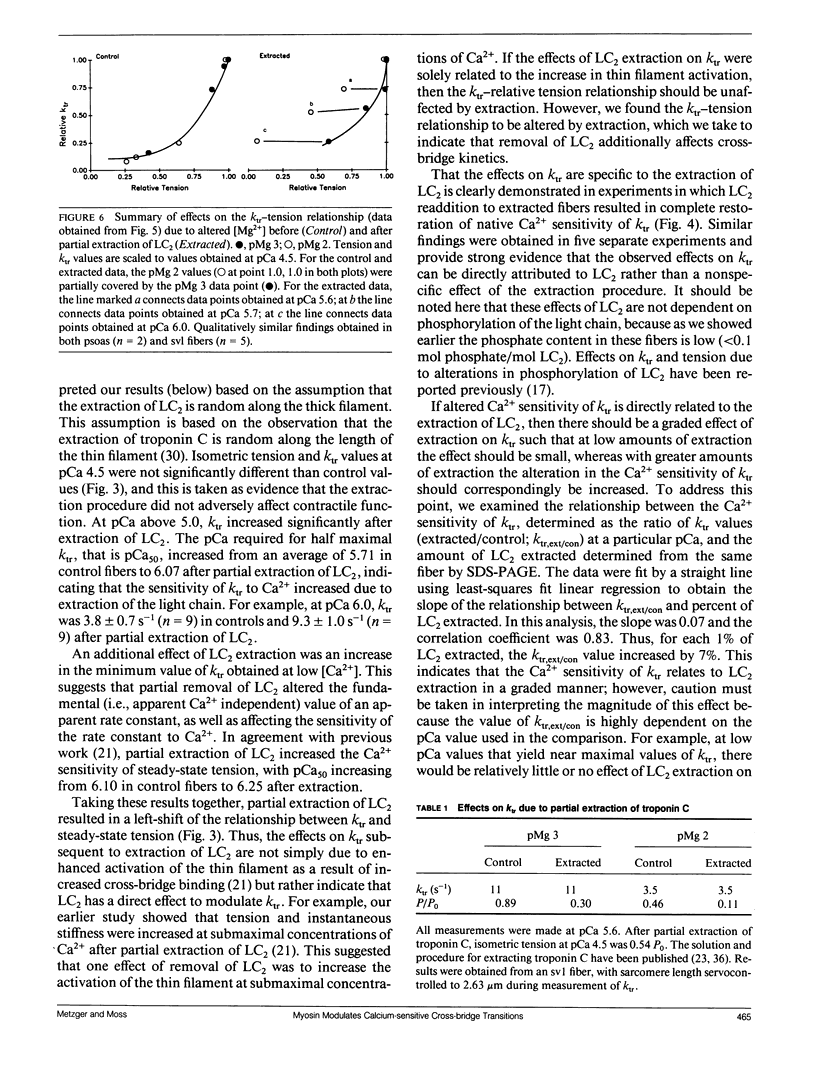
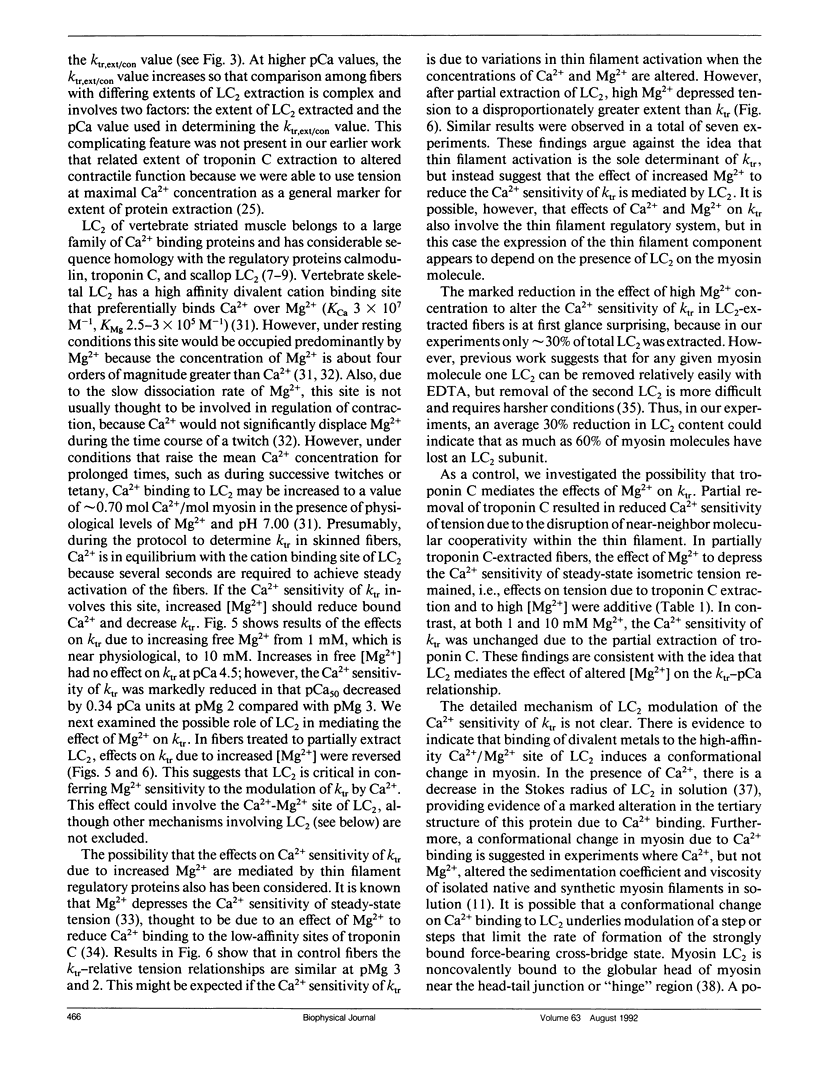
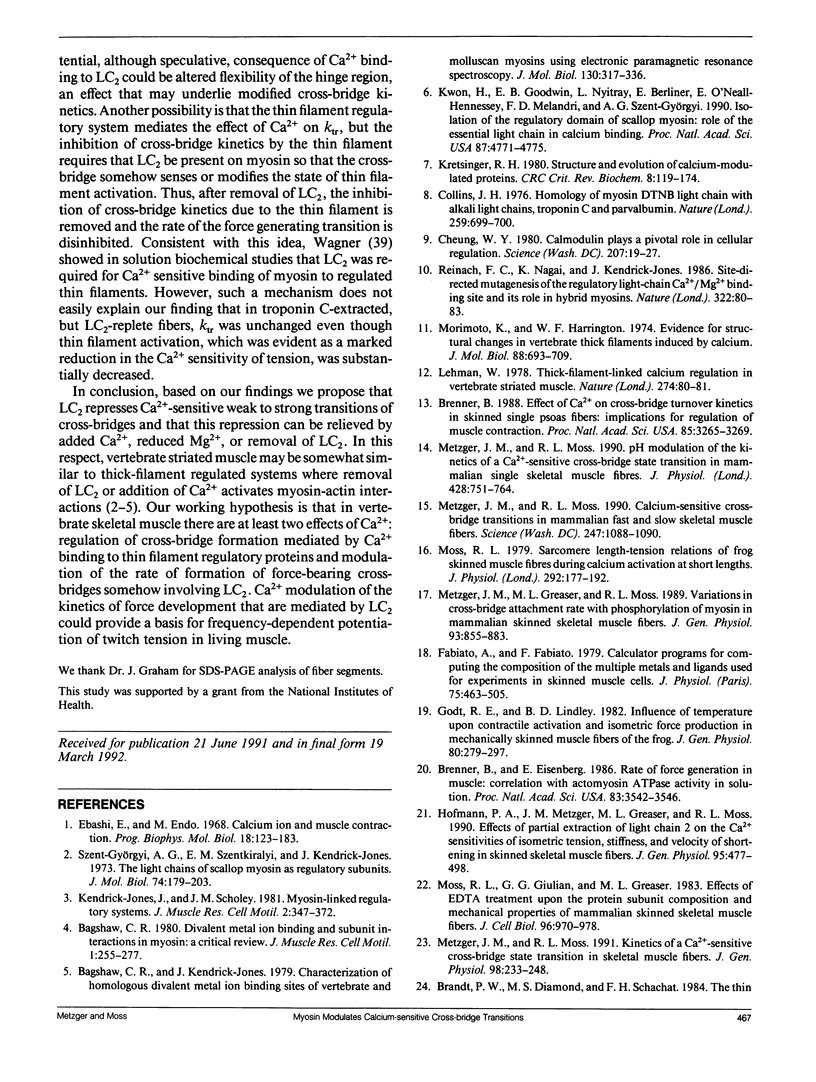
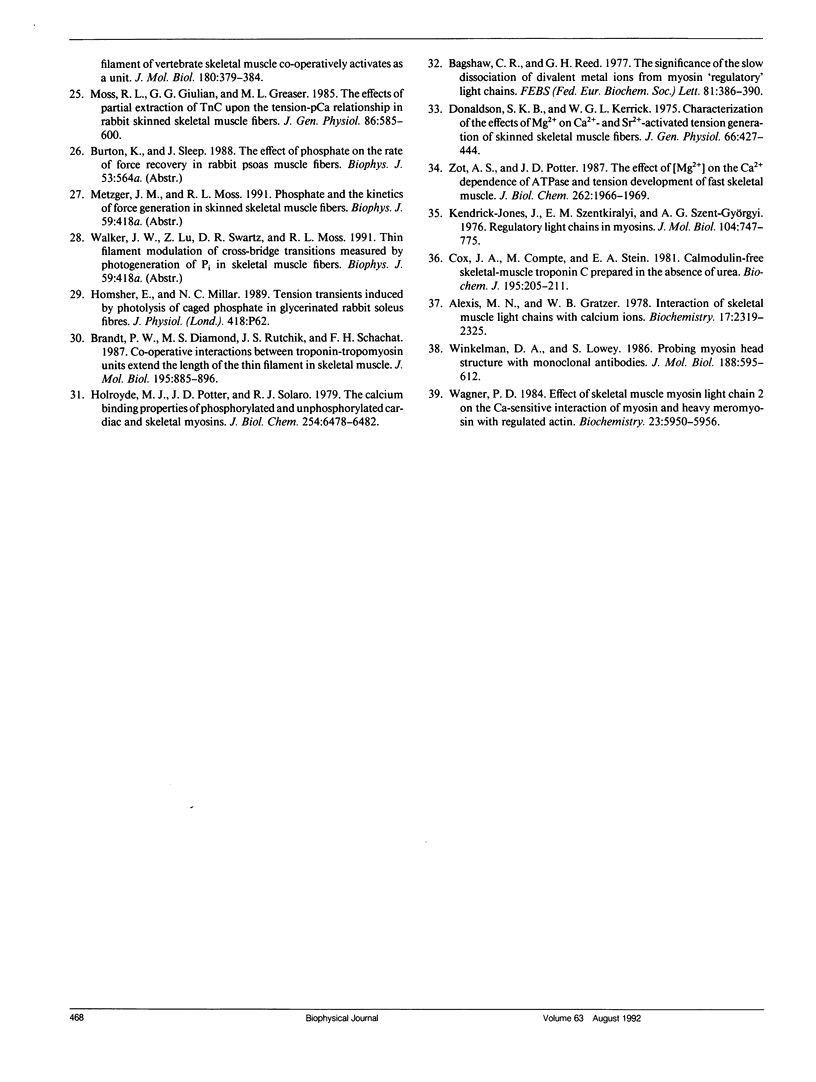
Images in this article
Selected References
These references are in PubMed. This may not be the complete list of references from this article.
- Alexis M. N., Gratzer W. B. Interaction of skeletal myosin light chains with calcium ions. Biochemistry. 1978 Jun 13;17(12):2319–2325. doi: 10.1021/bi00605a010. [DOI] [PubMed] [Google Scholar]
- Bagshaw C. R. Divalent metal ion binding and subunit interactions in myosins: a critical review. J Muscle Res Cell Motil. 1980 Sep;1(3):255–277. doi: 10.1007/BF00711931. [DOI] [PubMed] [Google Scholar]
- Bagshaw C. R., Kendrick-Jones J. Characterization of homologous divalent metal ion binding sites of vertebrate and molluscan myosins using electron paramagnetic resonance spectroscopy. J Mol Biol. 1979 May 25;130(3):317–336. doi: 10.1016/0022-2836(79)90544-8. [DOI] [PubMed] [Google Scholar]
- Bagshaw C. R., Reed G. H. The significance of the slow dissociation of divalent metal ions from myosin 'regulatory' light chains. FEBS Lett. 1977 Sep 15;81(2):386–390. doi: 10.1016/0014-5793(77)80560-7. [DOI] [PubMed] [Google Scholar]
- Brandt P. W., Diamond M. S., Rutchik J. S., Schachat F. H. Co-operative interactions between troponin-tropomyosin units extend the length of the thin filament in skeletal muscle. J Mol Biol. 1987 Jun 20;195(4):885–896. doi: 10.1016/0022-2836(87)90492-x. [DOI] [PubMed] [Google Scholar]
- Brandt P. W., Diamond M. S., Schachat F. H. The thin filament of vertebrate skeletal muscle co-operatively activates as a unit. J Mol Biol. 1984 Dec 5;180(2):379–384. doi: 10.1016/s0022-2836(84)80010-8. [DOI] [PubMed] [Google Scholar]
- Brenner B. Effect of Ca2+ on cross-bridge turnover kinetics in skinned single rabbit psoas fibers: implications for regulation of muscle contraction. Proc Natl Acad Sci U S A. 1988 May;85(9):3265–3269. doi: 10.1073/pnas.85.9.3265. [DOI] [PMC free article] [PubMed] [Google Scholar]
- Brenner B., Eisenberg E. Rate of force generation in muscle: correlation with actomyosin ATPase activity in solution. Proc Natl Acad Sci U S A. 1986 May;83(10):3542–3546. doi: 10.1073/pnas.83.10.3542. [DOI] [PMC free article] [PubMed] [Google Scholar]
- Cheung W. Y. Calmodulin plays a pivotal role in cellular regulation. Science. 1980 Jan 4;207(4426):19–27. doi: 10.1126/science.6243188. [DOI] [PubMed] [Google Scholar]
- Collins J. H. Homology of myosin DTNB light chain with alkali light chains, troponin C and parvalbumin. Nature. 1976 Feb 26;259(5545):699–700. doi: 10.1038/259699a0. [DOI] [PubMed] [Google Scholar]
- Cox J. A., Comte M., Stein E. A. Calmodulin-free skeletal-muscle troponin C prepared in the absence of urea. Biochem J. 1981 Apr 1;195(1):205–211. doi: 10.1042/bj1950205. [DOI] [PMC free article] [PubMed] [Google Scholar]
- Donaldson S. K., Kerrick W. G. Characterization of the effects of Mg2+ on Ca2+- and Sr2+-activated tension generation of skinned skeletal muscle fibers. J Gen Physiol. 1975 Oct;66(4):427–444. doi: 10.1085/jgp.66.4.427. [DOI] [PMC free article] [PubMed] [Google Scholar]
- Ebashi S., Endo M. Calcium ion and muscle contraction. Prog Biophys Mol Biol. 1968;18:123–183. doi: 10.1016/0079-6107(68)90023-0. [DOI] [PubMed] [Google Scholar]
- Fabiato A., Fabiato F. Calculator programs for computing the composition of the solutions containing multiple metals and ligands used for experiments in skinned muscle cells. J Physiol (Paris) 1979;75(5):463–505. [PubMed] [Google Scholar]
- Godt R. E., Lindley B. D. Influence of temperature upon contractile activation and isometric force production in mechanically skinned muscle fibers of the frog. J Gen Physiol. 1982 Aug;80(2):279–297. doi: 10.1085/jgp.80.2.279. [DOI] [PMC free article] [PubMed] [Google Scholar]
- Hofmann P. A., Metzger J. M., Greaser M. L., Moss R. L. Effects of partial extraction of light chain 2 on the Ca2+ sensitivities of isometric tension, stiffness, and velocity of shortening in skinned skeletal muscle fibers. J Gen Physiol. 1990 Mar;95(3):477–498. doi: 10.1085/jgp.95.3.477. [DOI] [PMC free article] [PubMed] [Google Scholar]
- Holroyde M. J., Potter J. D., Solaro R. J. The calcium binding properties of phosphorylated and unphosphorylated cardiac and skeletal myosins. J Biol Chem. 1979 Jul 25;254(14):6478–6482. [PubMed] [Google Scholar]
- Kendrick-Jones J., Szentkiralyi E. M., Szent-Györgyi A. G. Regulatory light chains in myosins. J Mol Biol. 1976 Jul 15;104(4):747–775. doi: 10.1016/0022-2836(76)90180-7. [DOI] [PubMed] [Google Scholar]
- Kretsinger R. H. Structure and evolution of calcium-modulated proteins. CRC Crit Rev Biochem. 1980;8(2):119–174. doi: 10.3109/10409238009105467. [DOI] [PubMed] [Google Scholar]
- Kwon H., Goodwin E. B., Nyitray L., Berliner E., O'Neall-Hennessey E., Melandri F. D., Szent-Györgyi A. G. Isolation of the regulatory domain of scallop myosin: role of the essential light chain in calcium binding. Proc Natl Acad Sci U S A. 1990 Jun;87(12):4771–4775. doi: 10.1073/pnas.87.12.4771. [DOI] [PMC free article] [PubMed] [Google Scholar]
- Lehman W. Thick-filament-linked calcium regulation in vertebrate striated muscle. Nature. 1978 Jul 6;274(5666):80–81. doi: 10.1038/274080a0. [DOI] [PubMed] [Google Scholar]
- Metzger J. M., Greaser M. L., Moss R. L. Variations in cross-bridge attachment rate and tension with phosphorylation of myosin in mammalian skinned skeletal muscle fibers. Implications for twitch potentiation in intact muscle. J Gen Physiol. 1989 May;93(5):855–883. doi: 10.1085/jgp.93.5.855. [DOI] [PMC free article] [PubMed] [Google Scholar]
- Metzger J. M., Moss R. L. Calcium-sensitive cross-bridge transitions in mammalian fast and slow skeletal muscle fibers. Science. 1990 Mar 2;247(4946):1088–1090. doi: 10.1126/science.2309121. [DOI] [PubMed] [Google Scholar]
- Metzger J. M., Moss R. L. Kinetics of a Ca(2+)-sensitive cross-bridge state transition in skeletal muscle fibers. Effects due to variations in thin filament activation by extraction of troponin C. J Gen Physiol. 1991 Aug;98(2):233–248. doi: 10.1085/jgp.98.2.233. [DOI] [PMC free article] [PubMed] [Google Scholar]
- Metzger J. M., Moss R. L. pH modulation of the kinetics of a Ca2(+)-sensitive cross-bridge state transition in mammalian single skeletal muscle fibres. J Physiol. 1990 Sep;428:751–764. doi: 10.1113/jphysiol.1990.sp018239. [DOI] [PMC free article] [PubMed] [Google Scholar]
- Morimoto K., Harrington W. F. Evidence for structural changes in vertebrate thick filaments induced by calcium. J Mol Biol. 1974 Sep 25;88(3):693–709. doi: 10.1016/0022-2836(74)90417-3. [DOI] [PubMed] [Google Scholar]
- Moss R. L., Giulian G. G., Greaser M. L. Effects of EDTA treatment upon the protein subunit composition and mechanical properties of mammalian single skeletal muscle fibers. J Cell Biol. 1983 Apr;96(4):970–978. doi: 10.1083/jcb.96.4.970. [DOI] [PMC free article] [PubMed] [Google Scholar]
- Moss R. L., Giulian G. G., Greaser M. L. The effects of partial extraction of TnC upon the tension-pCa relationship in rabbit skinned skeletal muscle fibers. J Gen Physiol. 1985 Oct;86(4):585–600. doi: 10.1085/jgp.86.4.585. [DOI] [PMC free article] [PubMed] [Google Scholar]
- Moss R. L. Sarcomere length-tension relations of frog skinned muscle fibres during calcium activation at short lengths. J Physiol. 1979 Jul;292:177–192. doi: 10.1113/jphysiol.1979.sp012845. [DOI] [PMC free article] [PubMed] [Google Scholar]
- Reinach F. C., Nagai K., Kendrick-Jones J. Site-directed mutagenesis of the regulatory light-chain Ca2+/Mg2+ binding site and its role in hybrid myosins. Nature. 1986 Jul 3;322(6074):80–83. doi: 10.1038/322080a0. [DOI] [PubMed] [Google Scholar]
- Szent-Györgyi A. G., Szentkiralyi E. M., Kendrick-Jonas J. The light chains of scallop myosin as regulatory subunits. J Mol Biol. 1973 Feb 25;74(2):179–203. doi: 10.1016/0022-2836(73)90106-x. [DOI] [PubMed] [Google Scholar]
- Wagner P. D. Effect of skeletal muscle myosin light chain 2 on the Ca2+-sensitive interaction of myosin and heavy meromyosin with regulated actin. Biochemistry. 1984 Dec 4;23(25):5950–5956. doi: 10.1021/bi00320a010. [DOI] [PubMed] [Google Scholar]
- Winkelmann D. A., Lowey S. Probing myosin head structure with monoclonal antibodies. J Mol Biol. 1986 Apr 20;188(4):595–612. doi: 10.1016/s0022-2836(86)80009-2. [DOI] [PubMed] [Google Scholar]
- Zot A. S., Potter J. D. The effect of [Mg2+] on the Ca2+ dependence of ATPase and tension development of fast skeletal muscle. The role of the Ca2+-specific sites of troponin C. J Biol Chem. 1987 Feb 15;262(5):1966–1969. [PubMed] [Google Scholar]