Abstract
The consequences of cardiac excitation-contraction coupling by calcium-induced calcium release were studied theoretically, using a series of idealized models solved by analytic and numerical methods. "Common-pool" models, those in which the trigger calcium and released calcium pass through a common cytosolic pool, gave nearly all-or-none regenerative calcium releases (in disagreement with experiment), unless their loop gain was made sufficiently low that it provided little amplification of the calcium entering through the sarcolemma. In the linear (small trigger) limit, it was proven rigorously that no common-pool model can give graded high amplification unless it is operated on the verge of spontaneous oscillation. To circumvent this problem, we considered two types of "local-control" models. In the first type, the local calcium from a sarcolemmal L-type calcium channel directly stimulates a single, immediately opposed SR calcium release channel. This permits high amplification without regeneration, but requires high conductance of the SR channel. This problem is avoided in the second type of local control model, in which one L-type channel triggers a regenerative cluster of several SR channels. Statistical recruitment of clusters results in graded response with high amplification. In either type of local-control model, the voltage dependence of SR calcium release is not exactly the same as that of the macroscopic sarcolemmal calcium current, even though calcium is the only trigger for SR release. This results from the existence of correlations between the stochastic openings of individual sarcolemmal and SR channels. Propagation of regenerative calcium-release waves (under conditions of calcium overload) was analyzed using analytically soluble models in which SR calcium release was treated phenomenalogically. The range of wave velocities observed experimentally is easily explained; however, the observed degree of refractoriness to wave propagation requires either a strong dependence of SR calcium release on the rate of rise of cytosolic calcium or localization of SR release sites to one point in the sarcomere. We conclude that the macroscopic behavior of calcium-induced calcium release depends critically on the spatial relationships among sarcolemmal and SR calcium channels, as well as on their kinetics.
Full text
PDF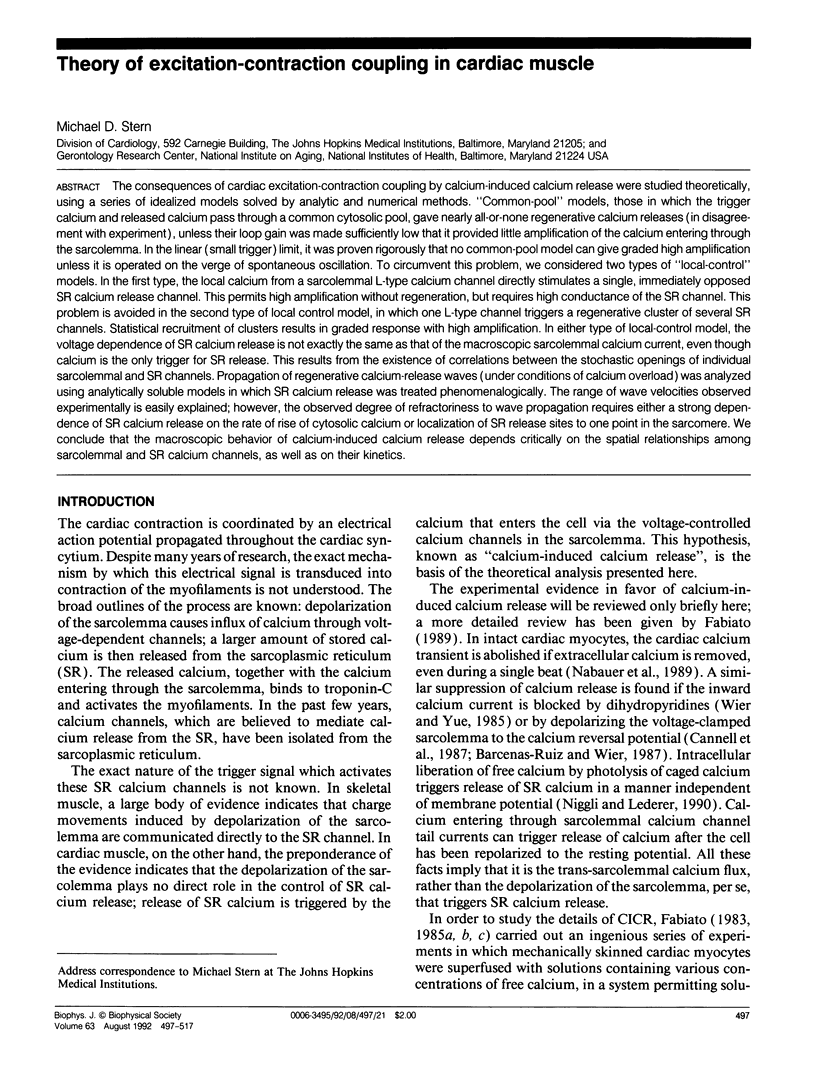
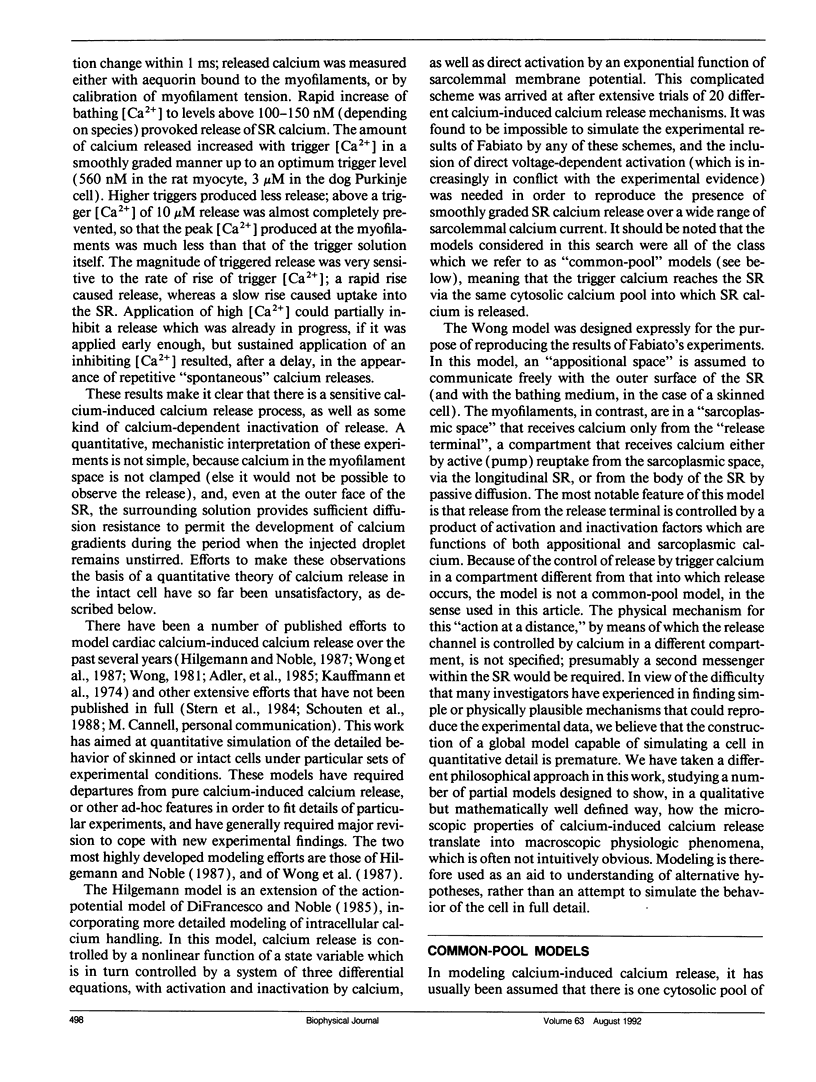
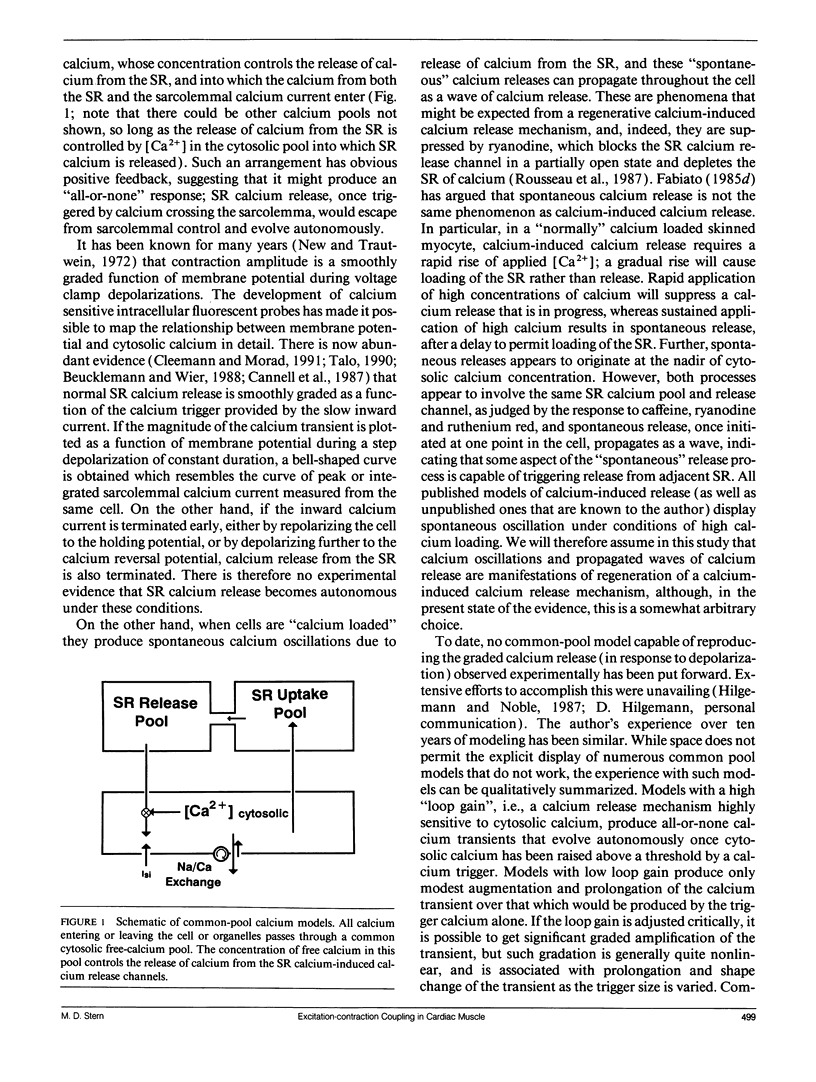
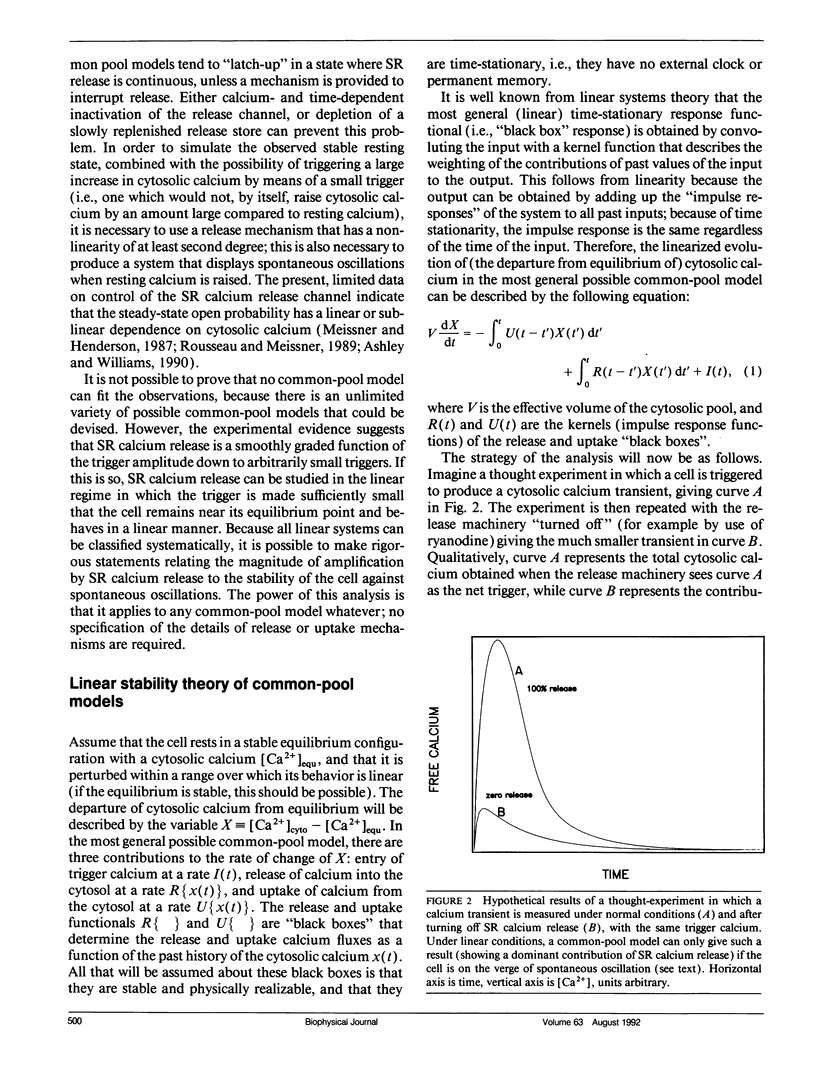
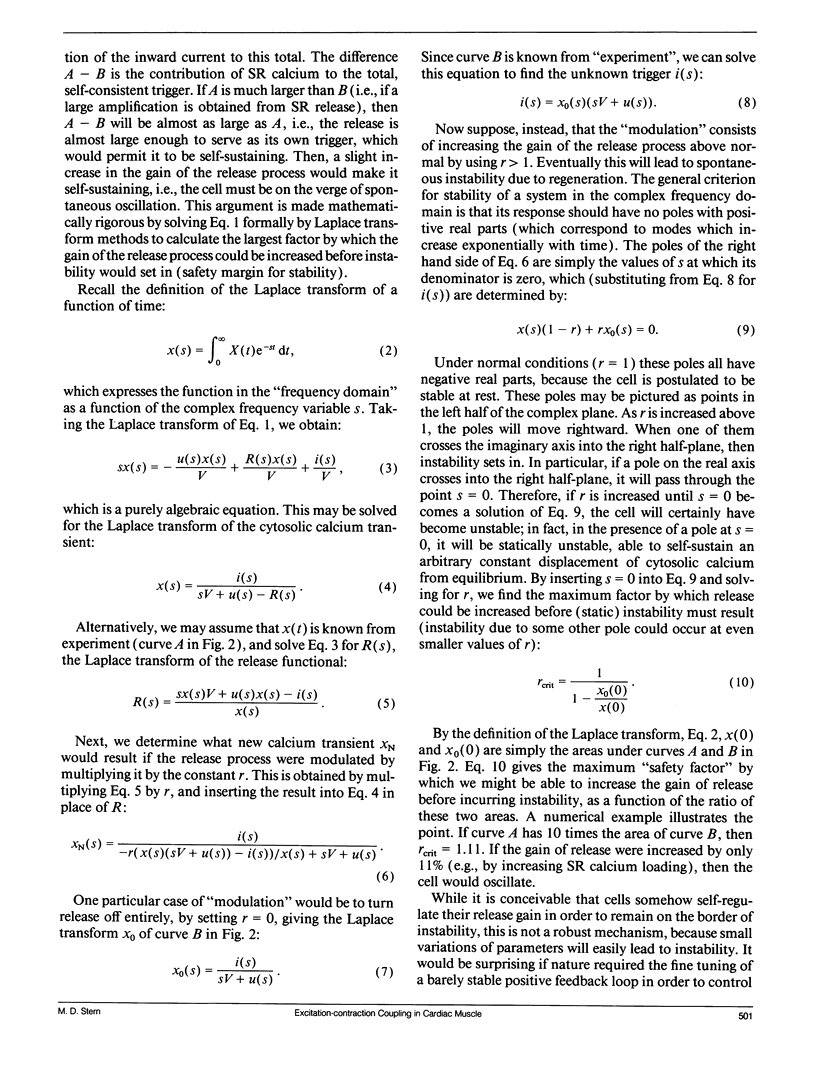
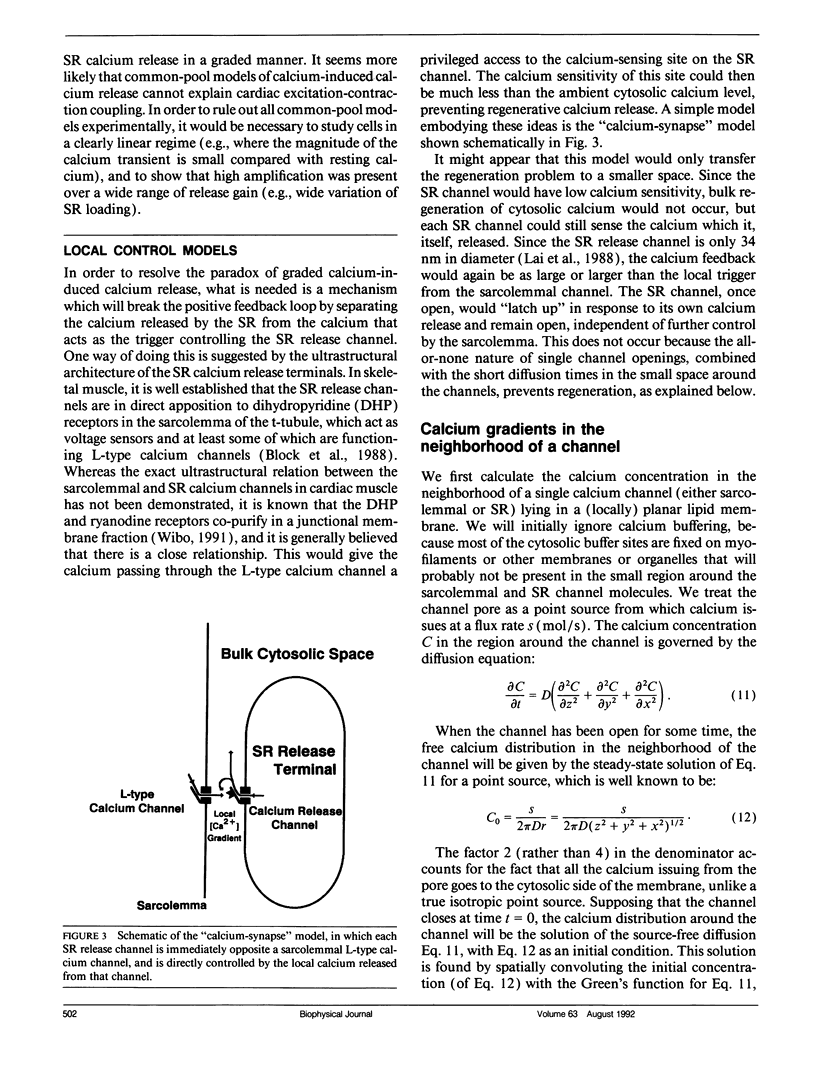
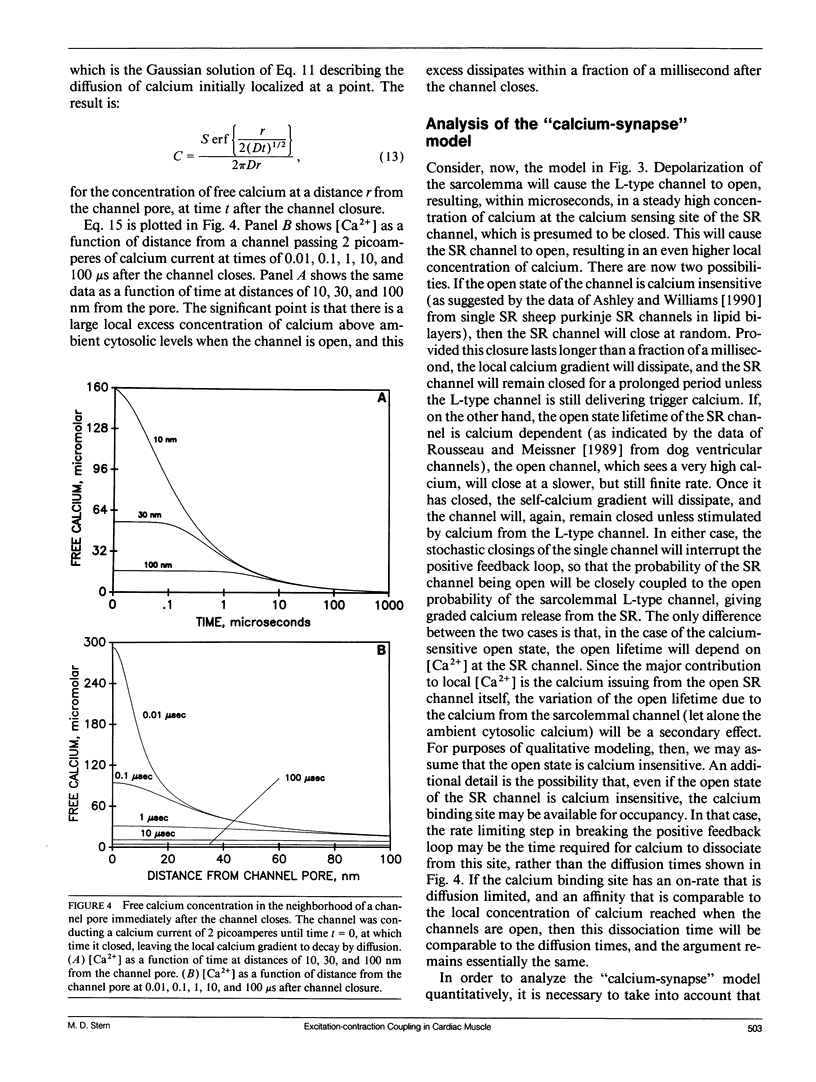
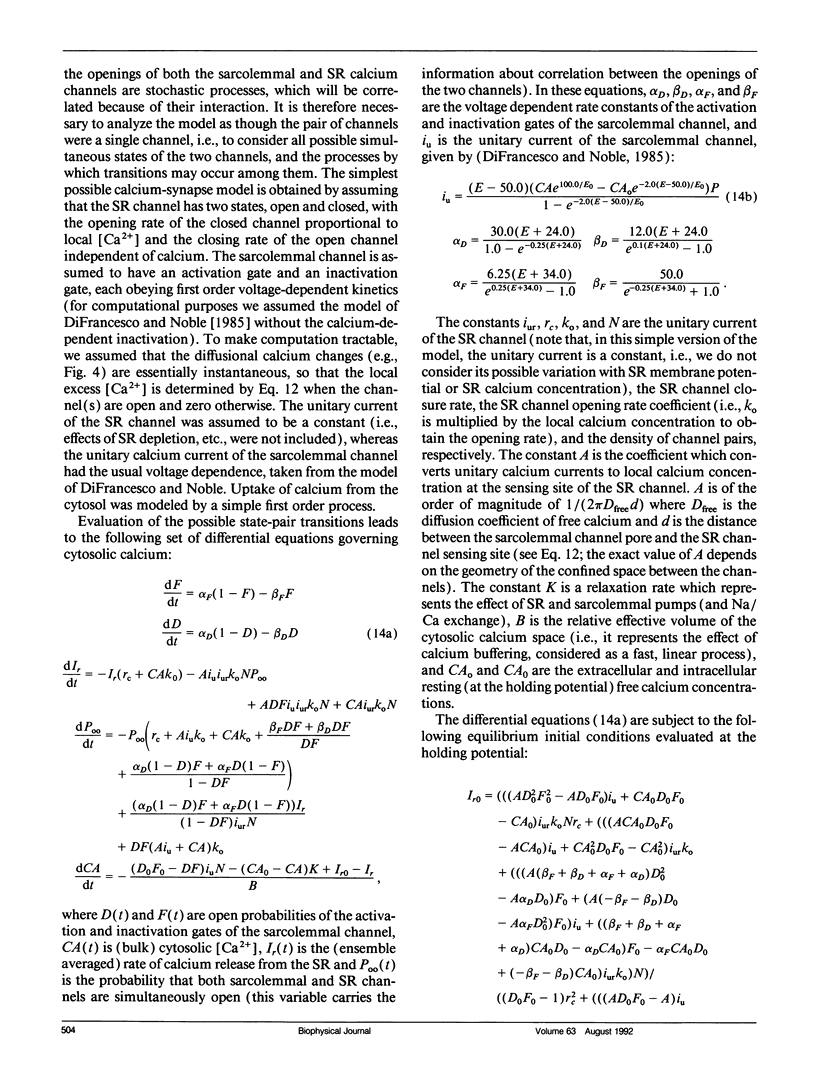
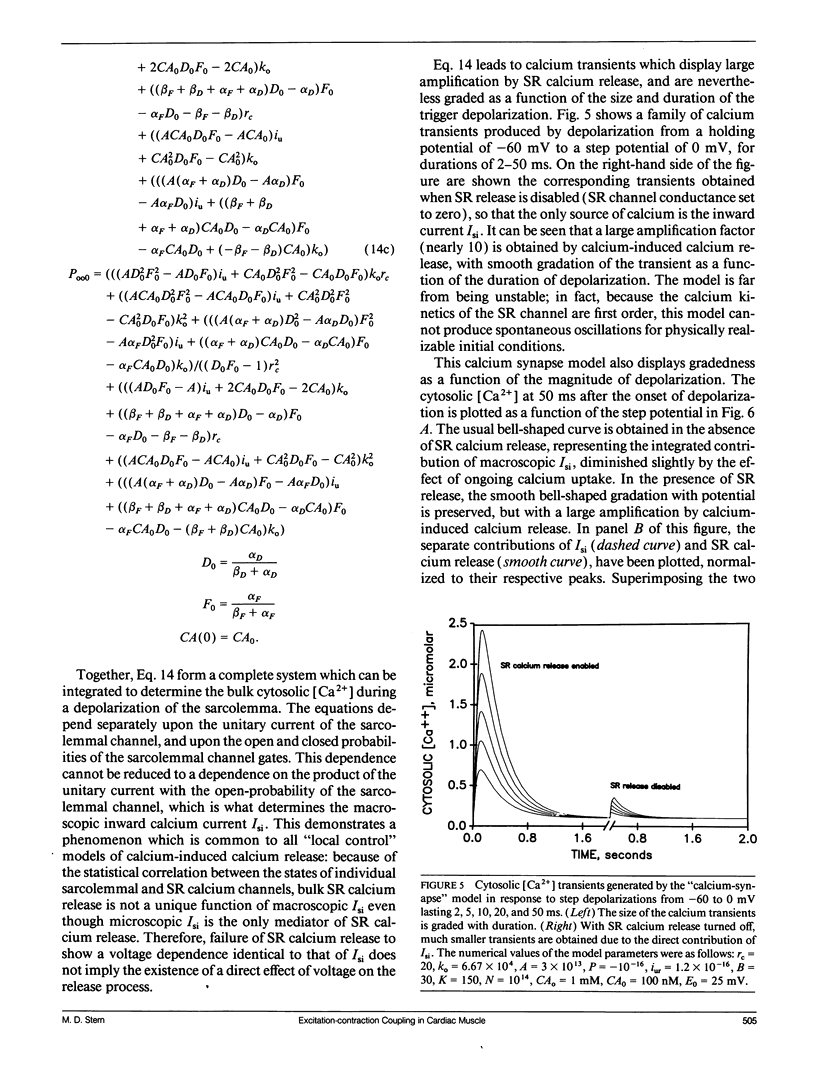
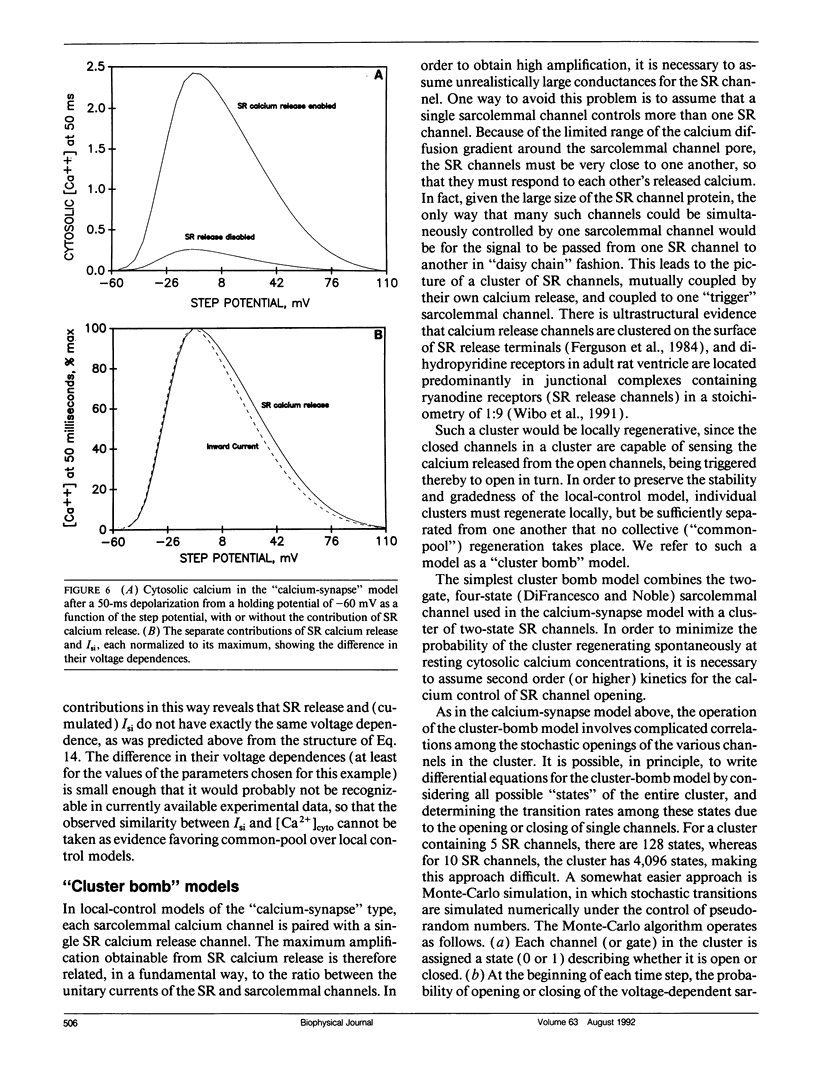
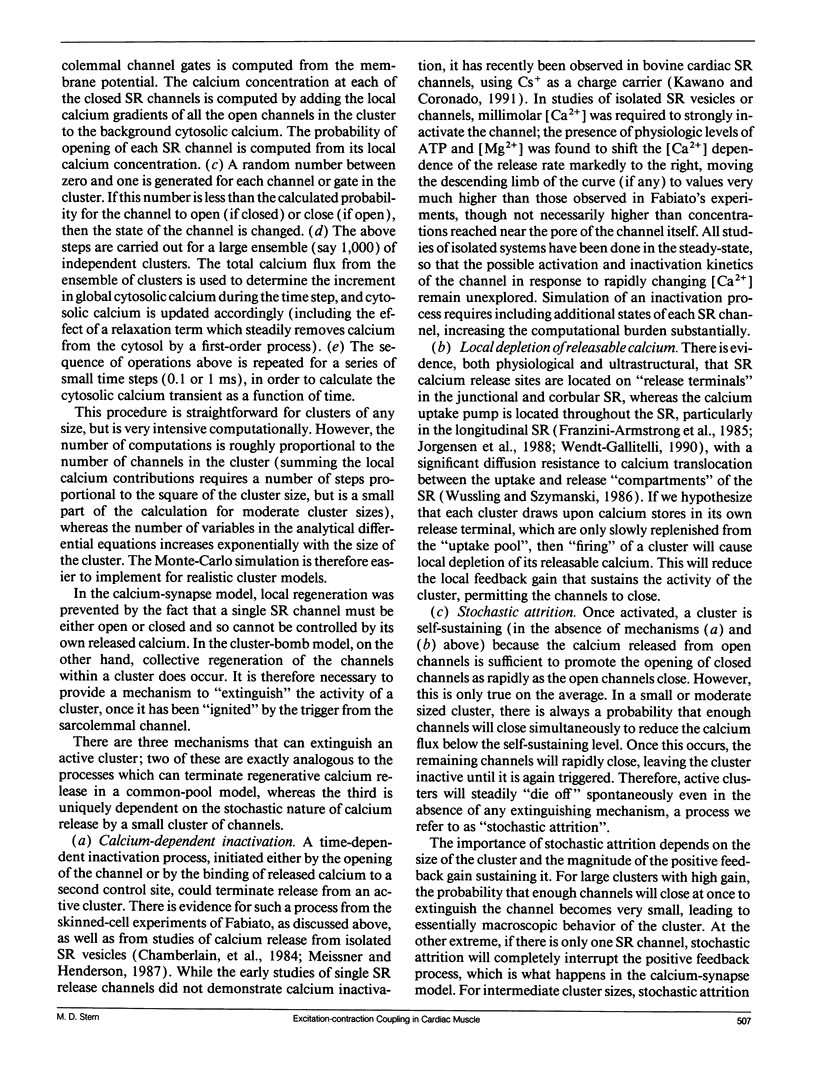
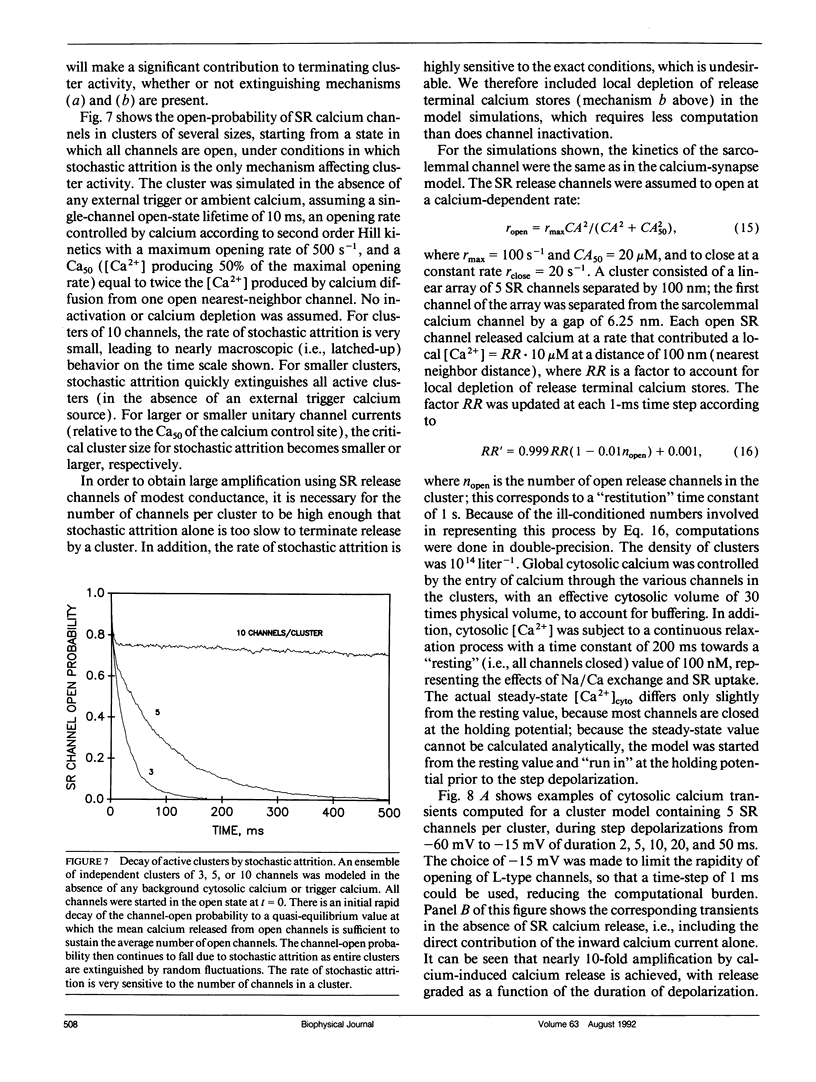
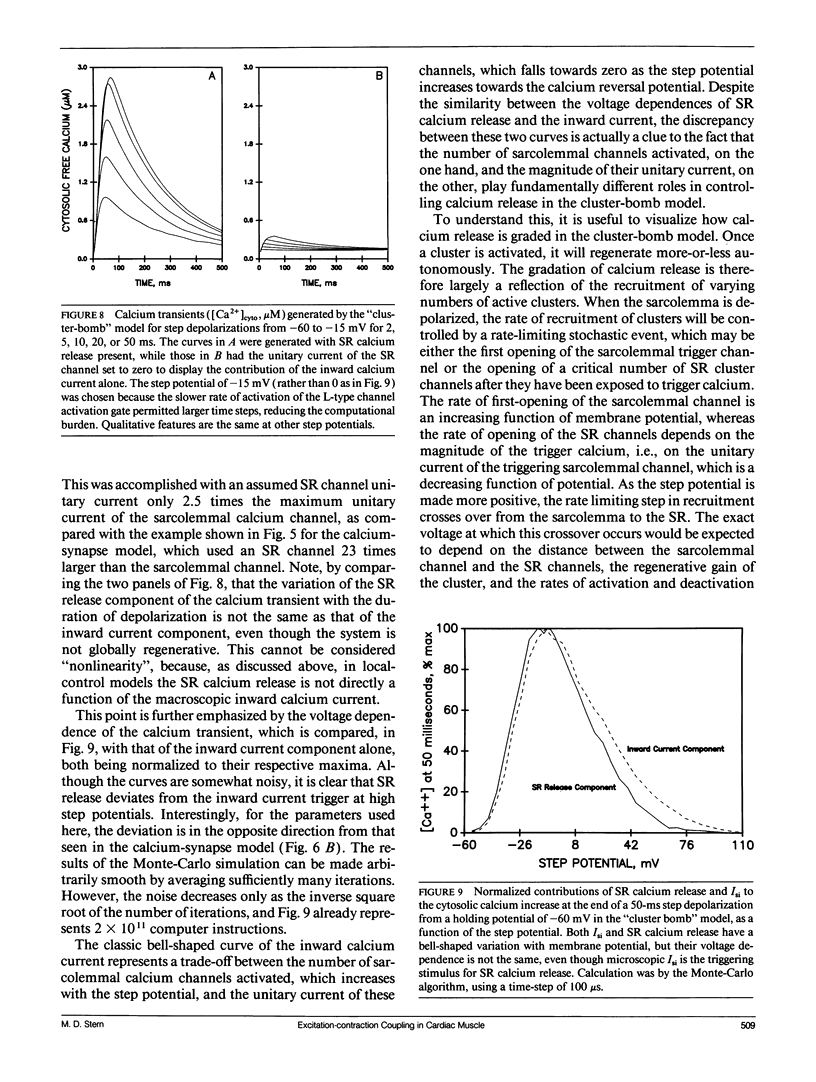
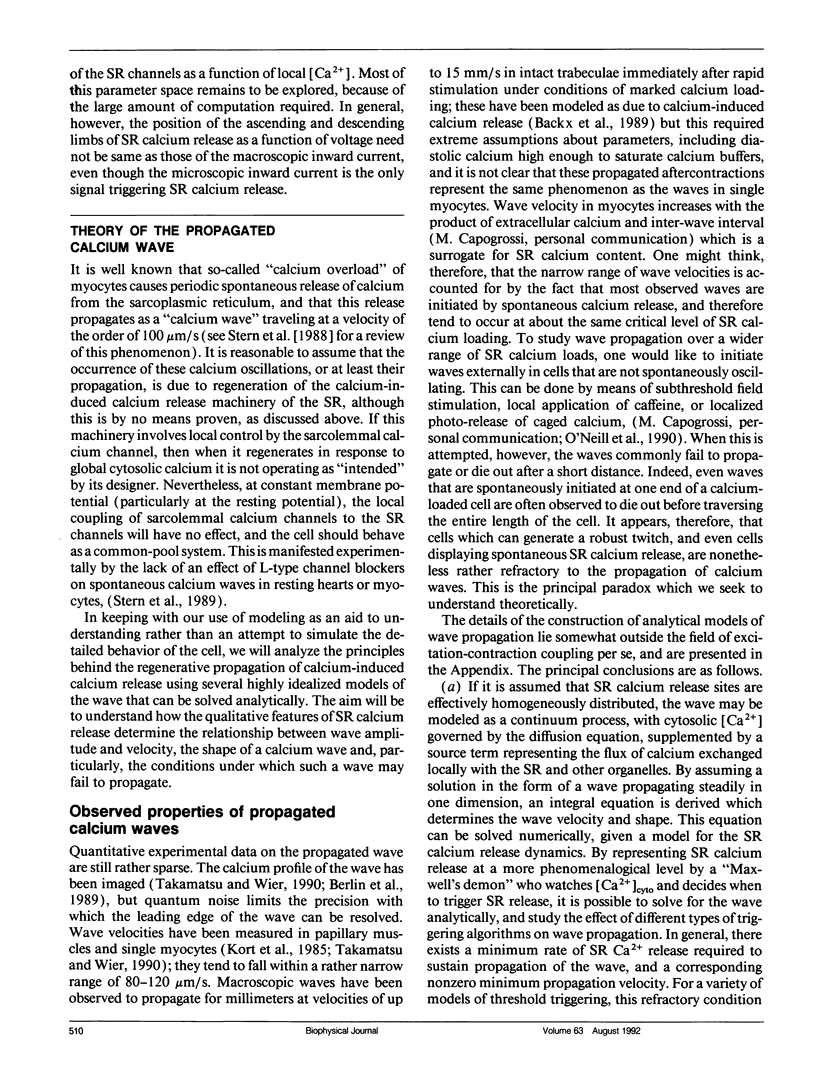
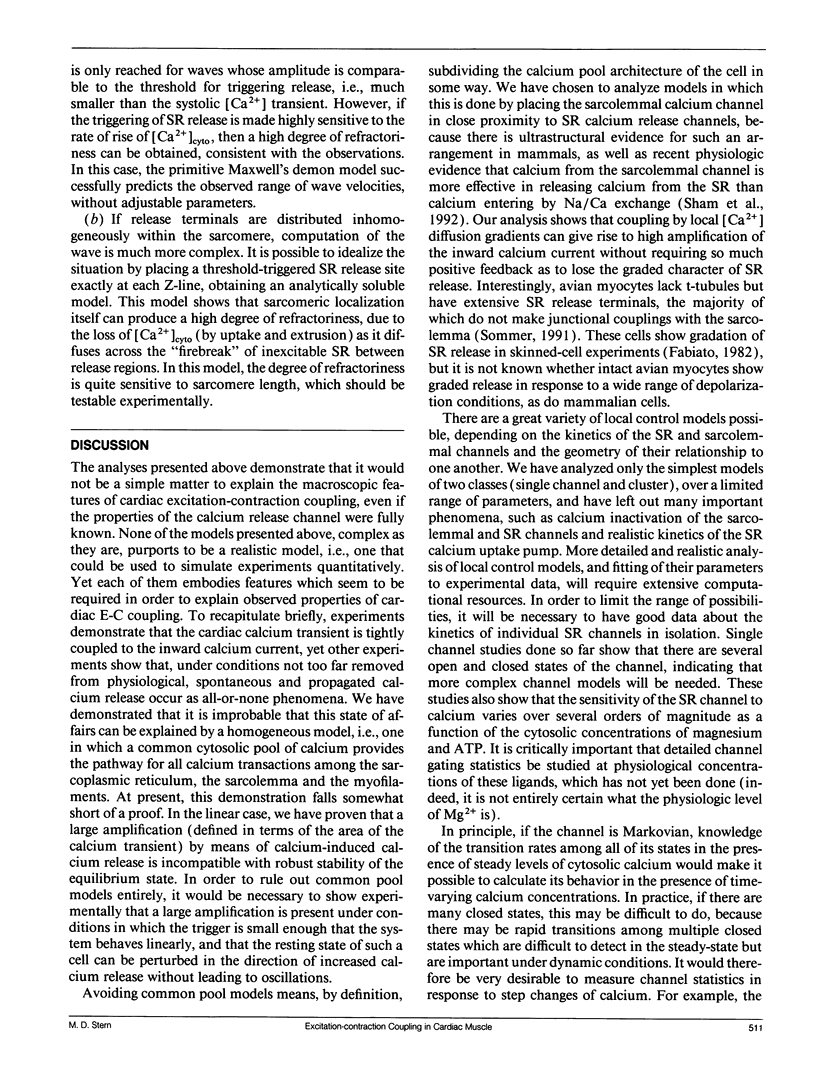
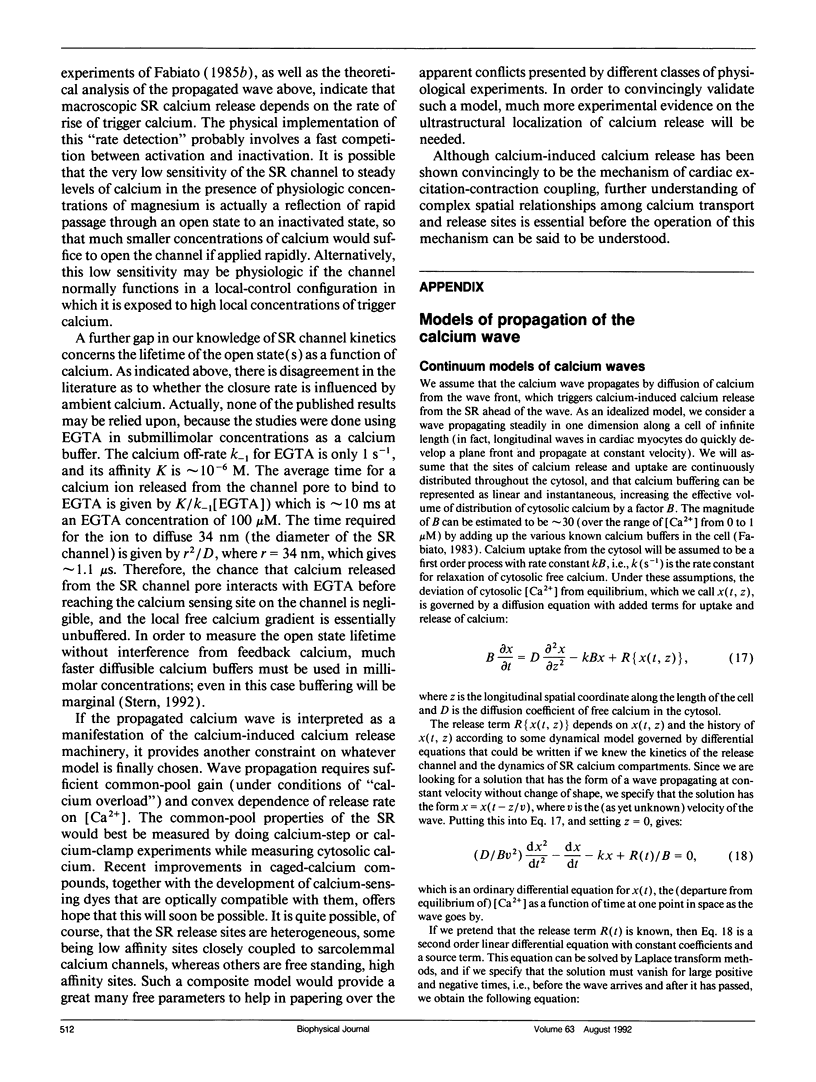
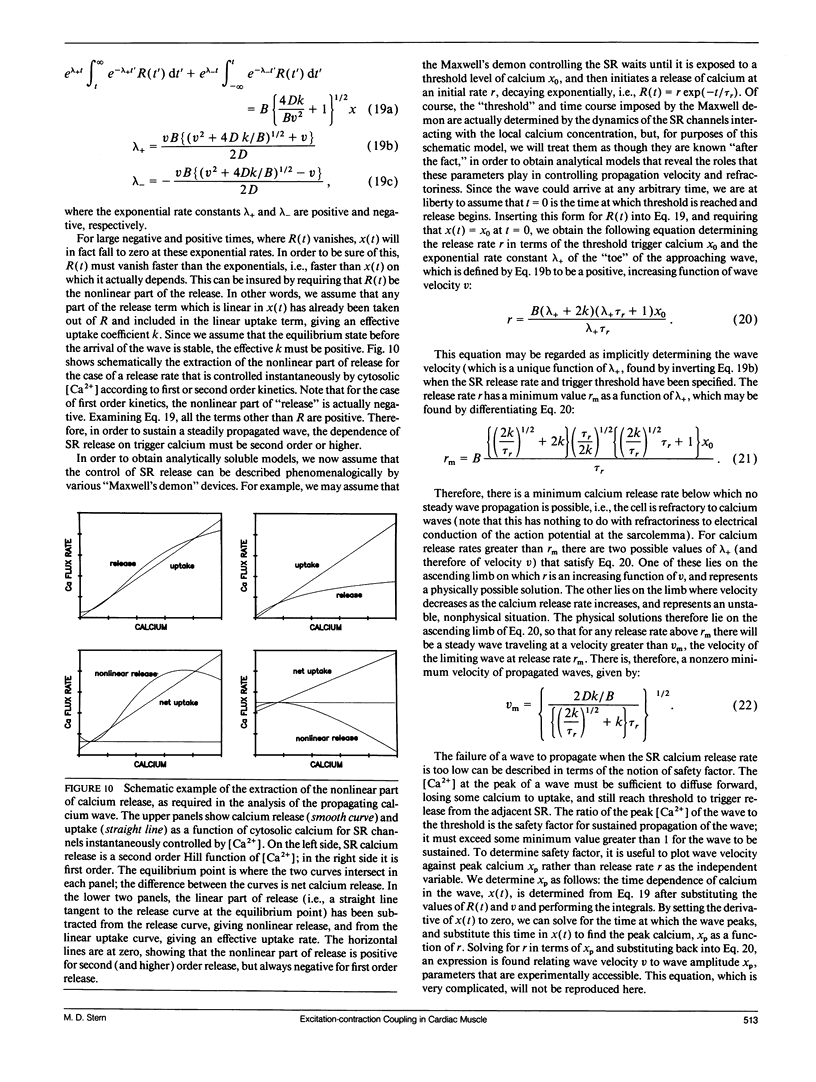
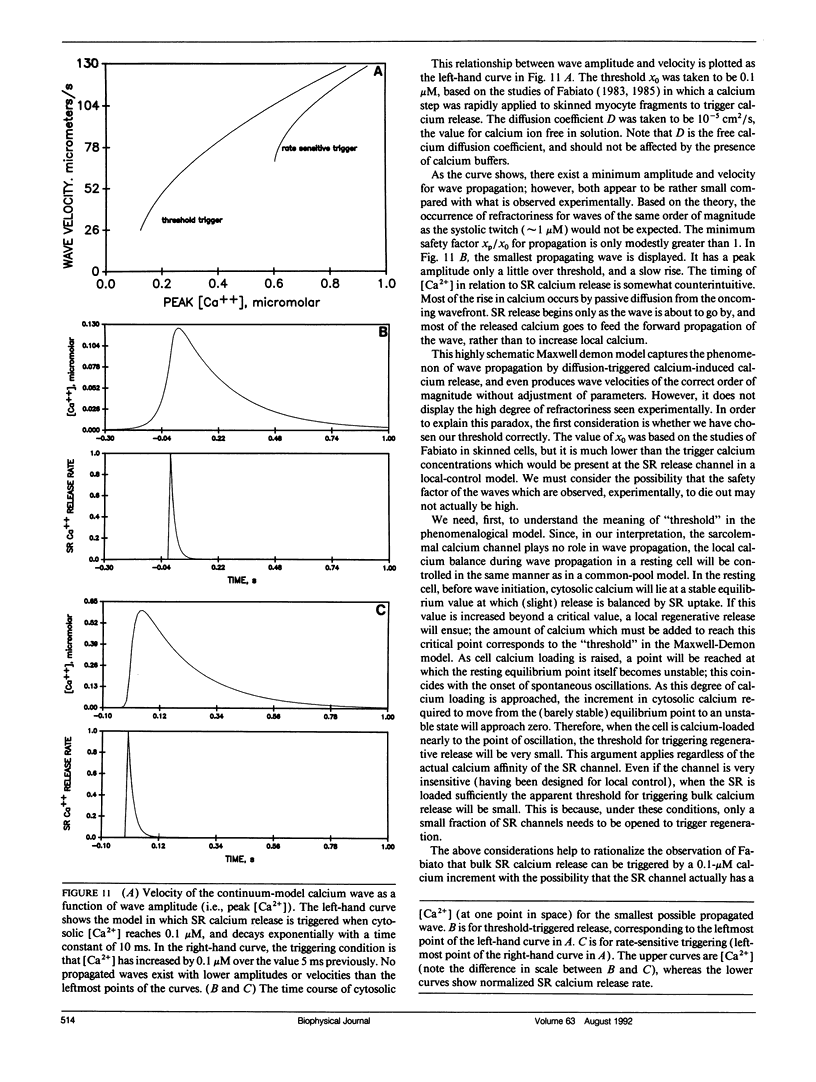
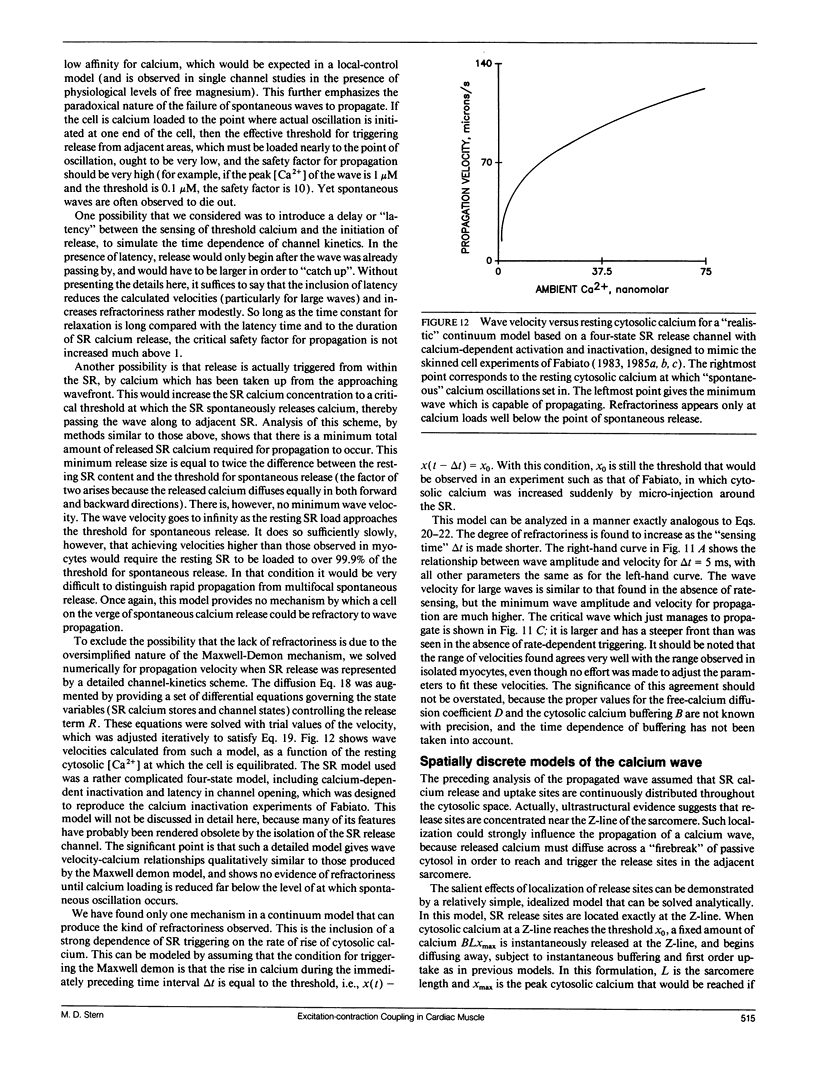
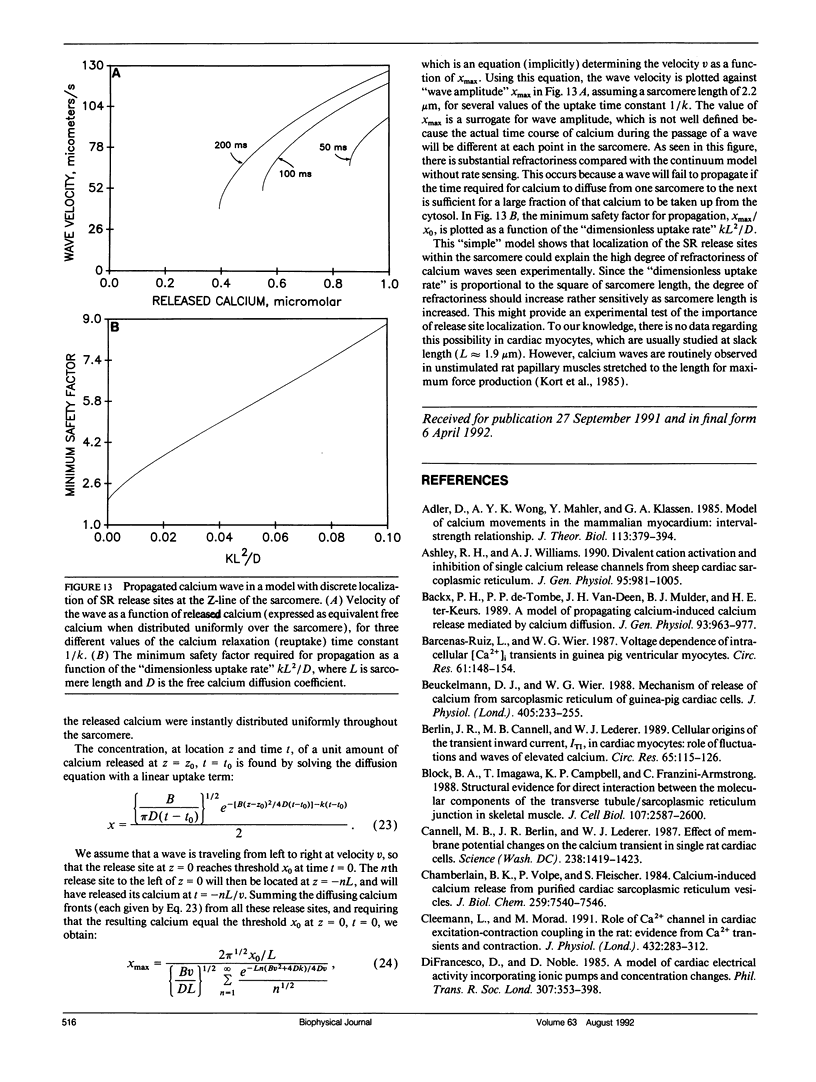
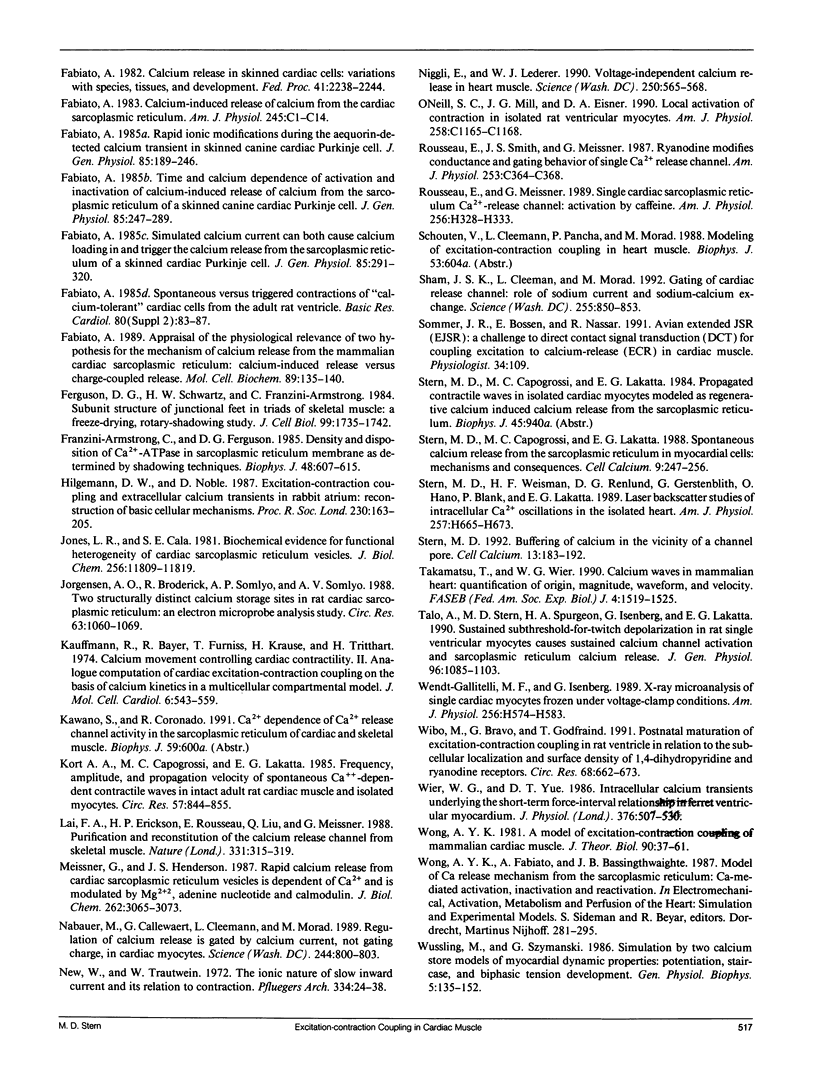
Selected References
These references are in PubMed. This may not be the complete list of references from this article.
- Adler D., Wong A. Y., Mahler Y., Klassen G. A. Model of calcium movements in the mammalian myocardium: interval-strength relationship. J Theor Biol. 1985 Mar 21;113(2):379–394. doi: 10.1016/s0022-5193(85)80233-2. [DOI] [PubMed] [Google Scholar]
- Ashley R. H., Williams A. J. Divalent cation activation and inhibition of single calcium release channels from sheep cardiac sarcoplasmic reticulum. J Gen Physiol. 1990 May;95(5):981–1005. doi: 10.1085/jgp.95.5.981. [DOI] [PMC free article] [PubMed] [Google Scholar]
- Backx P. H., de Tombe P. P., Van Deen J. H., Mulder B. J., ter Keurs H. E. A model of propagating calcium-induced calcium release mediated by calcium diffusion. J Gen Physiol. 1989 May;93(5):963–977. doi: 10.1085/jgp.93.5.963. [DOI] [PMC free article] [PubMed] [Google Scholar]
- Barcenas-Ruiz L., Wier W. G. Voltage dependence of intracellular [Ca2+]i transients in guinea pig ventricular myocytes. Circ Res. 1987 Jul;61(1):148–154. doi: 10.1161/01.res.61.1.148. [DOI] [PubMed] [Google Scholar]
- Berlin J. R., Cannell M. B., Lederer W. J. Cellular origins of the transient inward current in cardiac myocytes. Role of fluctuations and waves of elevated intracellular calcium. Circ Res. 1989 Jul;65(1):115–126. doi: 10.1161/01.res.65.1.115. [DOI] [PubMed] [Google Scholar]
- Beuckelmann D. J., Wier W. G. Mechanism of release of calcium from sarcoplasmic reticulum of guinea-pig cardiac cells. J Physiol. 1988 Nov;405:233–255. doi: 10.1113/jphysiol.1988.sp017331. [DOI] [PMC free article] [PubMed] [Google Scholar]
- Block B. A., Imagawa T., Campbell K. P., Franzini-Armstrong C. Structural evidence for direct interaction between the molecular components of the transverse tubule/sarcoplasmic reticulum junction in skeletal muscle. J Cell Biol. 1988 Dec;107(6 Pt 2):2587–2600. doi: 10.1083/jcb.107.6.2587. [DOI] [PMC free article] [PubMed] [Google Scholar]
- Cannell M. B., Berlin J. R., Lederer W. J. Effect of membrane potential changes on the calcium transient in single rat cardiac muscle cells. Science. 1987 Dec 4;238(4832):1419–1423. doi: 10.1126/science.2446391. [DOI] [PubMed] [Google Scholar]
- Chamberlain B. K., Volpe P., Fleischer S. Calcium-induced calcium release from purified cardiac sarcoplasmic reticulum vesicles. General characteristics. J Biol Chem. 1984 Jun 25;259(12):7540–7546. [PubMed] [Google Scholar]
- Cleemann L., Morad M. Role of Ca2+ channel in cardiac excitation-contraction coupling in the rat: evidence from Ca2+ transients and contraction. J Physiol. 1991 Jan;432:283–312. doi: 10.1113/jphysiol.1991.sp018385. [DOI] [PMC free article] [PubMed] [Google Scholar]
- DiFrancesco D., Noble D. A model of cardiac electrical activity incorporating ionic pumps and concentration changes. Philos Trans R Soc Lond B Biol Sci. 1985 Jan 10;307(1133):353–398. doi: 10.1098/rstb.1985.0001. [DOI] [PubMed] [Google Scholar]
- Fabiato A. Appraisal of the physiological relevance of two hypothesis for the mechanism of calcium release from the mammalian cardiac sarcoplasmic reticulum: calcium-induced release versus charge-coupled release. Mol Cell Biochem. 1989 Sep 7;89(2):135–140. doi: 10.1007/BF00220765. [DOI] [PubMed] [Google Scholar]
- Fabiato A. Calcium release in skinned cardiac cells: variations with species, tissues, and development. Fed Proc. 1982 May;41(7):2238–2244. [PubMed] [Google Scholar]
- Fabiato A. Calcium-induced release of calcium from the cardiac sarcoplasmic reticulum. Am J Physiol. 1983 Jul;245(1):C1–14. doi: 10.1152/ajpcell.1983.245.1.C1. [DOI] [PubMed] [Google Scholar]
- Fabiato A. Rapid ionic modifications during the aequorin-detected calcium transient in a skinned canine cardiac Purkinje cell. J Gen Physiol. 1985 Feb;85(2):189–246. doi: 10.1085/jgp.85.2.189. [DOI] [PMC free article] [PubMed] [Google Scholar]
- Fabiato A. Simulated calcium current can both cause calcium loading in and trigger calcium release from the sarcoplasmic reticulum of a skinned canine cardiac Purkinje cell. J Gen Physiol. 1985 Feb;85(2):291–320. doi: 10.1085/jgp.85.2.291. [DOI] [PMC free article] [PubMed] [Google Scholar]
- Fabiato A. Time and calcium dependence of activation and inactivation of calcium-induced release of calcium from the sarcoplasmic reticulum of a skinned canine cardiac Purkinje cell. J Gen Physiol. 1985 Feb;85(2):247–289. doi: 10.1085/jgp.85.2.247. [DOI] [PMC free article] [PubMed] [Google Scholar]
- Ferguson D. G., Schwartz H. W., Franzini-Armstrong C. Subunit structure of junctional feet in triads of skeletal muscle: a freeze-drying, rotary-shadowing study. J Cell Biol. 1984 Nov;99(5):1735–1742. doi: 10.1083/jcb.99.5.1735. [DOI] [PMC free article] [PubMed] [Google Scholar]
- Franzini-Armstrong C., Ferguson D. G. Density and disposition of Ca2+-ATPase in sarcoplasmic reticulum membrane as determined by shadowing techniques. Biophys J. 1985 Oct;48(4):607–615. doi: 10.1016/S0006-3495(85)83816-9. [DOI] [PMC free article] [PubMed] [Google Scholar]
- Hilgemann D. W., Noble D. Excitation-contraction coupling and extracellular calcium transients in rabbit atrium: reconstruction of basic cellular mechanisms. Proc R Soc Lond B Biol Sci. 1987 Mar 23;230(1259):163–205. doi: 10.1098/rspb.1987.0015. [DOI] [PubMed] [Google Scholar]
- Jones L. R., Cala S. E. Biochemical evidence for functional heterogeneity of cardiac sarcoplasmic reticulum vesicles. J Biol Chem. 1981 Nov 25;256(22):11809–11818. [PubMed] [Google Scholar]
- Jorgensen A. O., Broderick R., Somlyo A. P., Somlyo A. V. Two structurally distinct calcium storage sites in rat cardiac sarcoplasmic reticulum: an electron microprobe analysis study. Circ Res. 1988 Dec;63(6):1060–1069. doi: 10.1161/01.res.63.6.1060. [DOI] [PubMed] [Google Scholar]
- Kaufmann R., Bayer R., Fürniss T., Krause H., Tritthart H. Calcium-movement controlling cardiac contractility II. Analog computation of cardiac excitation-contraction coupling on the basis of calcium kinetics in a multi-compartment model. J Mol Cell Cardiol. 1974 Dec;6(6):543–559. doi: 10.1016/0022-2828(74)90035-2. [DOI] [PubMed] [Google Scholar]
- Kort A. A., Capogrossi M. C., Lakatta E. G. Frequency, amplitude, and propagation velocity of spontaneous Ca++-dependent contractile waves in intact adult rat cardiac muscle and isolated myocytes. Circ Res. 1985 Dec;57(6):844–855. doi: 10.1161/01.res.57.6.844. [DOI] [PubMed] [Google Scholar]
- Lai F. A., Erickson H. P., Rousseau E., Liu Q. Y., Meissner G. Purification and reconstitution of the calcium release channel from skeletal muscle. Nature. 1988 Jan 28;331(6154):315–319. doi: 10.1038/331315a0. [DOI] [PubMed] [Google Scholar]
- Meissner G., Henderson J. S. Rapid calcium release from cardiac sarcoplasmic reticulum vesicles is dependent on Ca2+ and is modulated by Mg2+, adenine nucleotide, and calmodulin. J Biol Chem. 1987 Mar 5;262(7):3065–3073. [PubMed] [Google Scholar]
- New W., Trautwein W. The ionic nature of slow inward current and its relation to contraction. Pflugers Arch. 1972;334(1):24–38. doi: 10.1007/BF00585998. [DOI] [PubMed] [Google Scholar]
- Niggli E., Lederer W. J. Voltage-independent calcium release in heart muscle. Science. 1990 Oct 26;250(4980):565–568. doi: 10.1126/science.2173135. [DOI] [PubMed] [Google Scholar]
- Näbauer M., Callewaert G., Cleemann L., Morad M. Regulation of calcium release is gated by calcium current, not gating charge, in cardiac myocytes. Science. 1989 May 19;244(4906):800–803. doi: 10.1126/science.2543067. [DOI] [PubMed] [Google Scholar]
- Rousseau E., Meissner G. Single cardiac sarcoplasmic reticulum Ca2+-release channel: activation by caffeine. Am J Physiol. 1989 Feb;256(2 Pt 2):H328–H333. doi: 10.1152/ajpheart.1989.256.2.H328. [DOI] [PubMed] [Google Scholar]
- Rousseau E., Smith J. S., Meissner G. Ryanodine modifies conductance and gating behavior of single Ca2+ release channel. Am J Physiol. 1987 Sep;253(3 Pt 1):C364–C368. doi: 10.1152/ajpcell.1987.253.3.C364. [DOI] [PubMed] [Google Scholar]
- Sham J. S., Cleemann L., Morad M. Gating of the cardiac Ca2+ release channel: the role of Na+ current and Na(+)-Ca2+ exchange. Science. 1992 Feb 14;255(5046):850–853. doi: 10.1126/science.1311127. [DOI] [PubMed] [Google Scholar]
- Stern M. D. Buffering of calcium in the vicinity of a channel pore. Cell Calcium. 1992 Mar;13(3):183–192. doi: 10.1016/0143-4160(92)90046-u. [DOI] [PubMed] [Google Scholar]
- Stern M. D., Capogrossi M. C., Lakatta E. G. Spontaneous calcium release from the sarcoplasmic reticulum in myocardial cells: mechanisms and consequences. Cell Calcium. 1988 Dec;9(5-6):247–256. doi: 10.1016/0143-4160(88)90005-x. [DOI] [PubMed] [Google Scholar]
- Stern M. D., Weisman H. F., Renlund D. G., Gerstenblith G., Hano O., Blank P. S., Lakatta E. G. Laser backscatter studies of intracellular Ca2+ oscillations in isolated hearts. Am J Physiol. 1989 Aug;257(2 Pt 2):H665–H673. doi: 10.1152/ajpheart.1989.257.2.H665. [DOI] [PubMed] [Google Scholar]
- Takamatsu T., Wier W. G. Calcium waves in mammalian heart: quantification of origin, magnitude, waveform, and velocity. FASEB J. 1990 Mar;4(5):1519–1525. doi: 10.1096/fasebj.4.5.2307330. [DOI] [PubMed] [Google Scholar]
- Talo A., Stern M. D., Spurgeon H. A., Isenberg G., Lakatta E. G. Sustained subthreshold-for-twitch depolarization in rat single ventricular myocytes causes sustained calcium channel activation and sarcoplasmic reticulum calcium release. J Gen Physiol. 1990 Nov;96(5):1085–1103. doi: 10.1085/jgp.96.5.1085. [DOI] [PMC free article] [PubMed] [Google Scholar]
- Wendt-Gallitelli M. F., Isenberg G. X-ray microanalysis of single cardiac myocytes frozen under voltage-clamp conditions. Am J Physiol. 1989 Feb;256(2 Pt 2):H574–H583. doi: 10.1152/ajpheart.1989.256.2.H574. [DOI] [PubMed] [Google Scholar]
- Wibo M., Bravo G., Godfraind T. Postnatal maturation of excitation-contraction coupling in rat ventricle in relation to the subcellular localization and surface density of 1,4-dihydropyridine and ryanodine receptors. Circ Res. 1991 Mar;68(3):662–673. doi: 10.1161/01.res.68.3.662. [DOI] [PubMed] [Google Scholar]
- Wong A. Y. A model of excitation-contraction coupling of mammalian cardiac muscle. J Theor Biol. 1981 May 7;90(1):37–61. doi: 10.1016/0022-5193(81)90121-1. [DOI] [PubMed] [Google Scholar]
- Wussling M., Szymanski G. Simulation by two calcium store models of myocardial dynamic properties: potentiation, staircase, and biphasic tension development. Gen Physiol Biophys. 1986 Apr;5(2):135–152. [PubMed] [Google Scholar]