Abstract
We have used linear frequency domain techniques to measure impedance at various locations and depths in the intact rat lens. The data are used to obtain best-fit solutions to a new electrical model based on lens structure, allowing us to estimate localized conductances of surface cell membranes (Gs), fiber cell membranes (gm), and gap junctions (Gj) as functions of position. We find that gm is small and fairly uniform throughout the lens (2.02 +/- 0.58 microS/cm2); for the anterior surface-epithelial cells Gs = 1.26 +/- 0.19 mS/cm2; for the posterior surface differentiating fiber cells Gs = 0.46 +/- 0.04 mS/cm2. Thus, Gs varies about the equator in a stepwise fashion. Gj between fiber cells at locations interior to 80% of the radius is fairly uniform (0.75 S/cm2); but in the outer 20% Gj varies smoothly and symmetrically from both poles (0.66 S/cm2) to equator (5.95 S/cm2). This pattern of variation in Gj is similar to the pattern of inward and outward currents reported by Robinson and Patterson (1983. Curr. Eye Res. 2:843-847). We therefore suggest that the nonuniform distribution of functional gap junctions, not the surface cell conductance or Na/K pumps, may be responsible for directing these current flows. Gap junctional uncoupling during exposure to elevated calcium and acidification was also examined. High calcium (20 mM, with the calcium ionophore A23187) produced modest (twofold) irreversible uncoupling along with large, irreversible decreases in membrane potential. We did not pursue this further. Acidification with 20 and 100% CO2-bubbled Tyrode's produced 5- and 15-fold reversible uncoupling, respectively, only in the outer 20% of the lens radius. The remaining inner 80% of the lens gap junctions seemed resistant to the acidification and did not uncouple.
Full text
PDF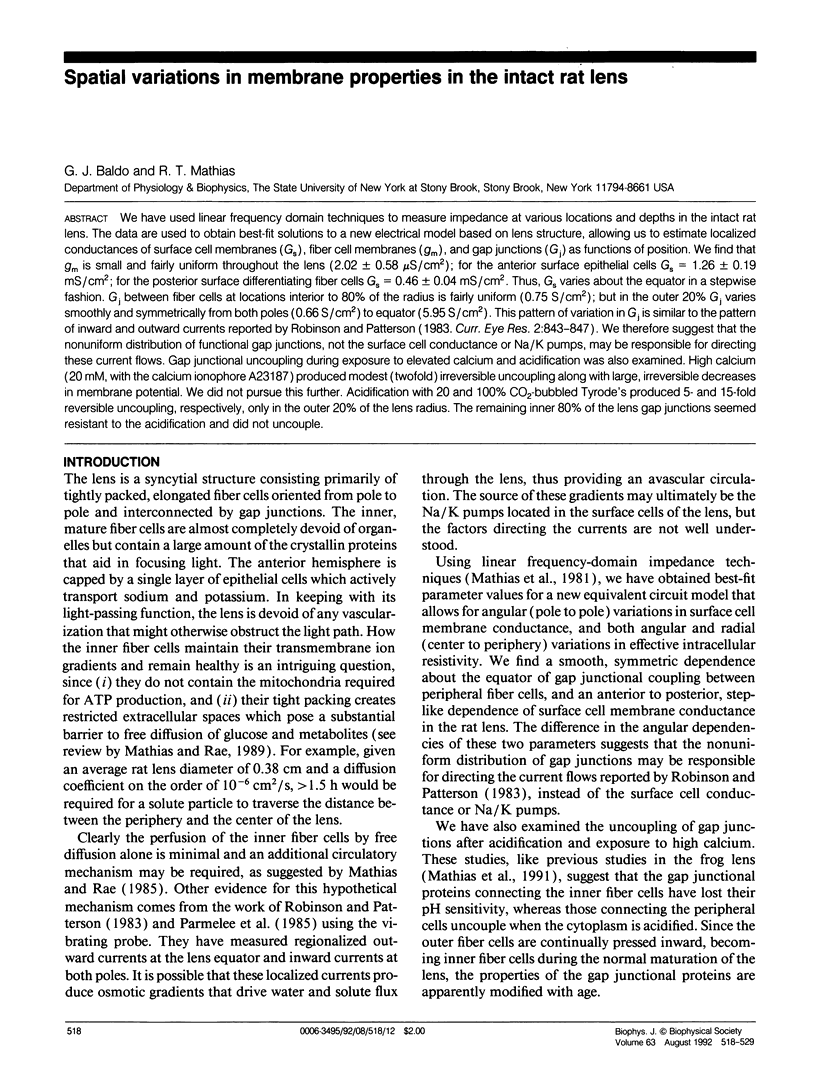
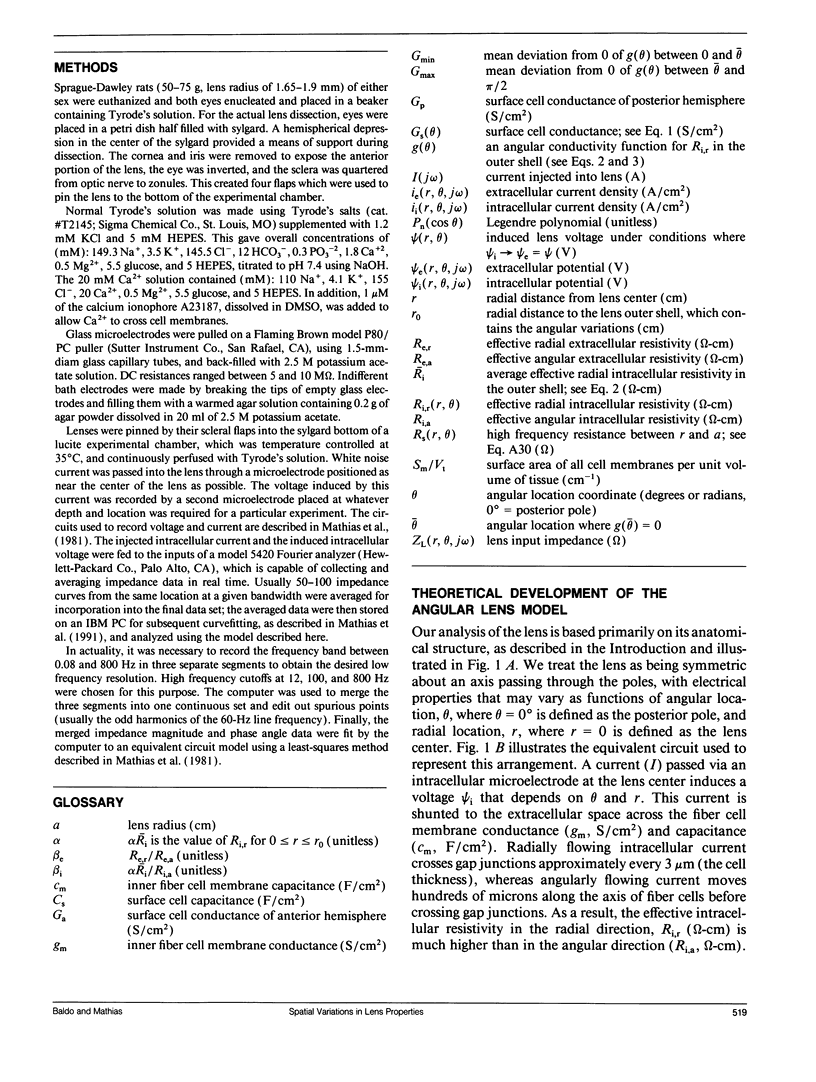
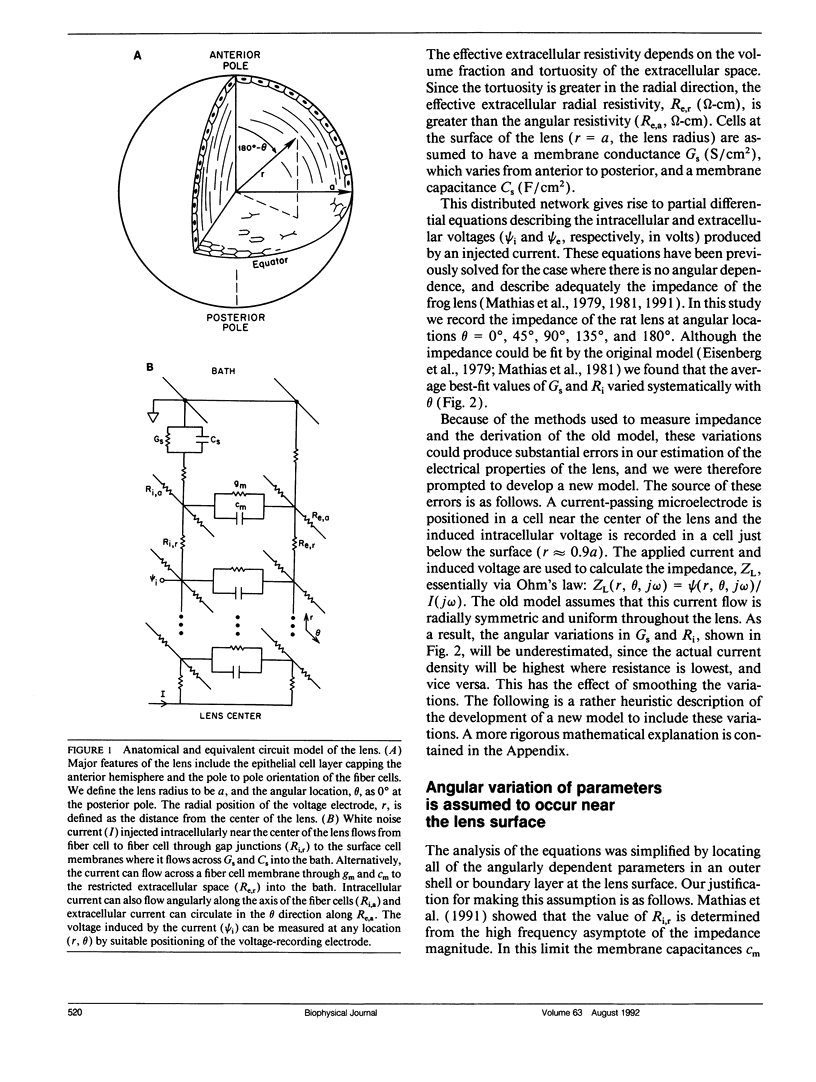
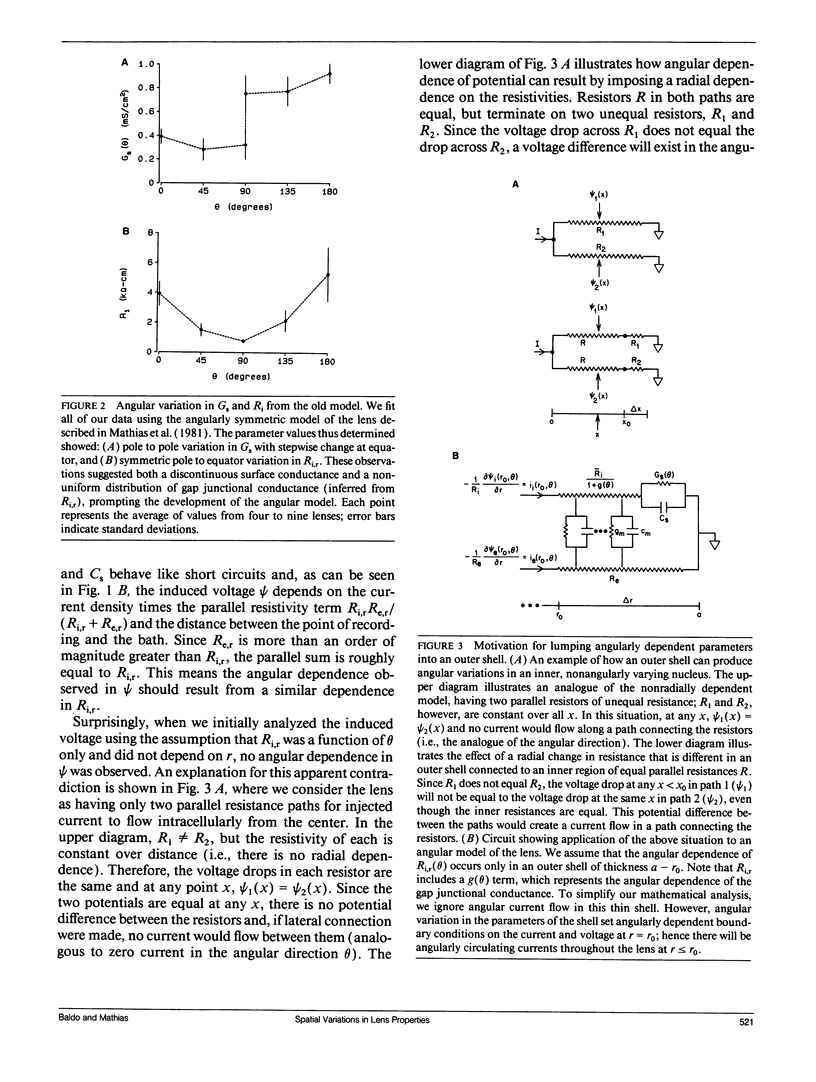
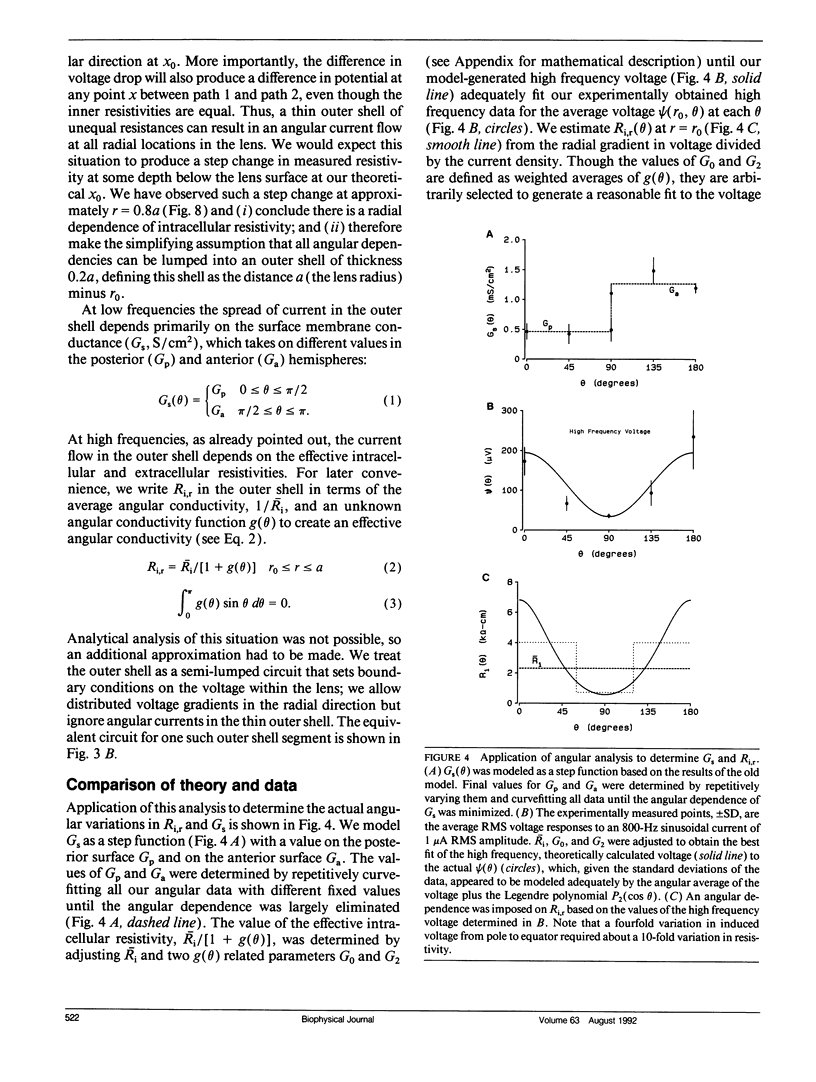
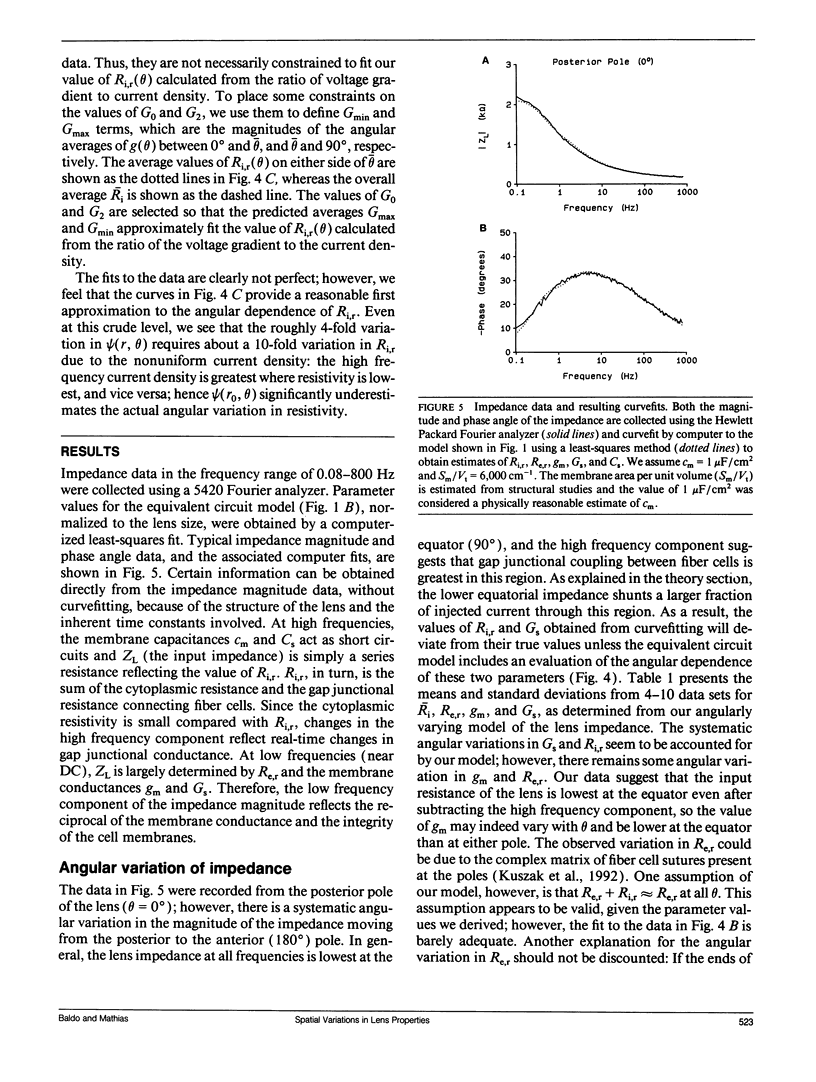
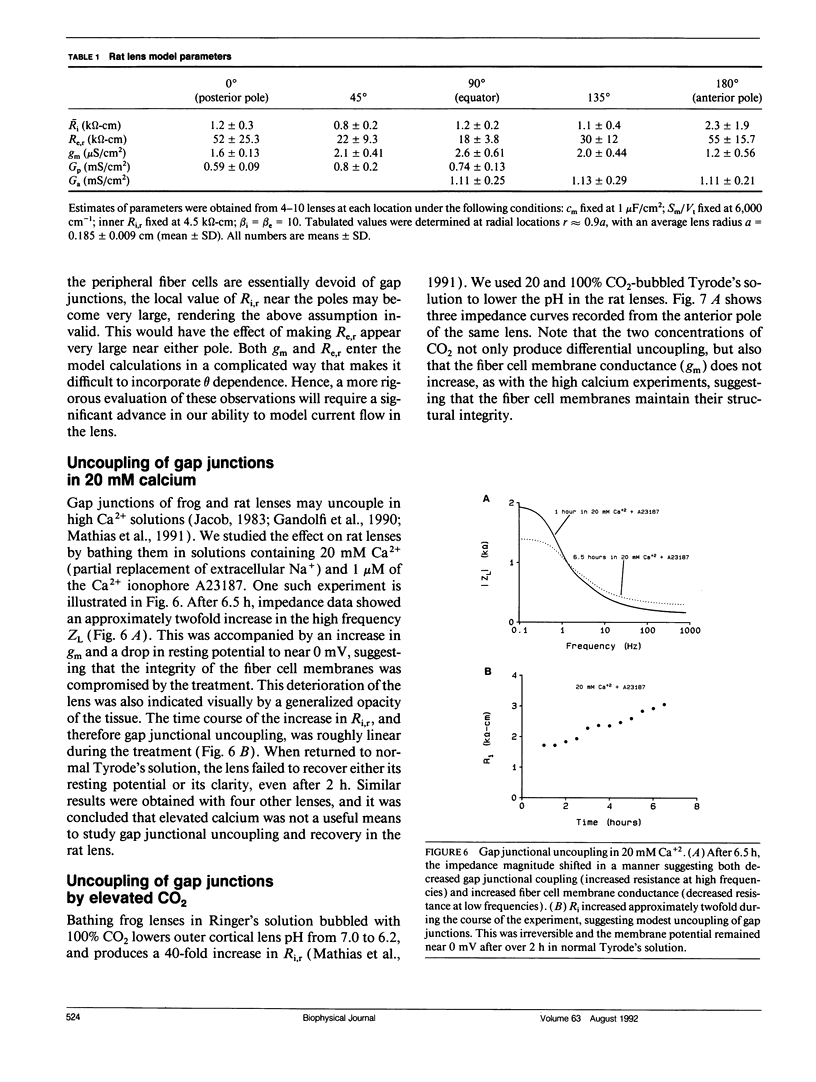
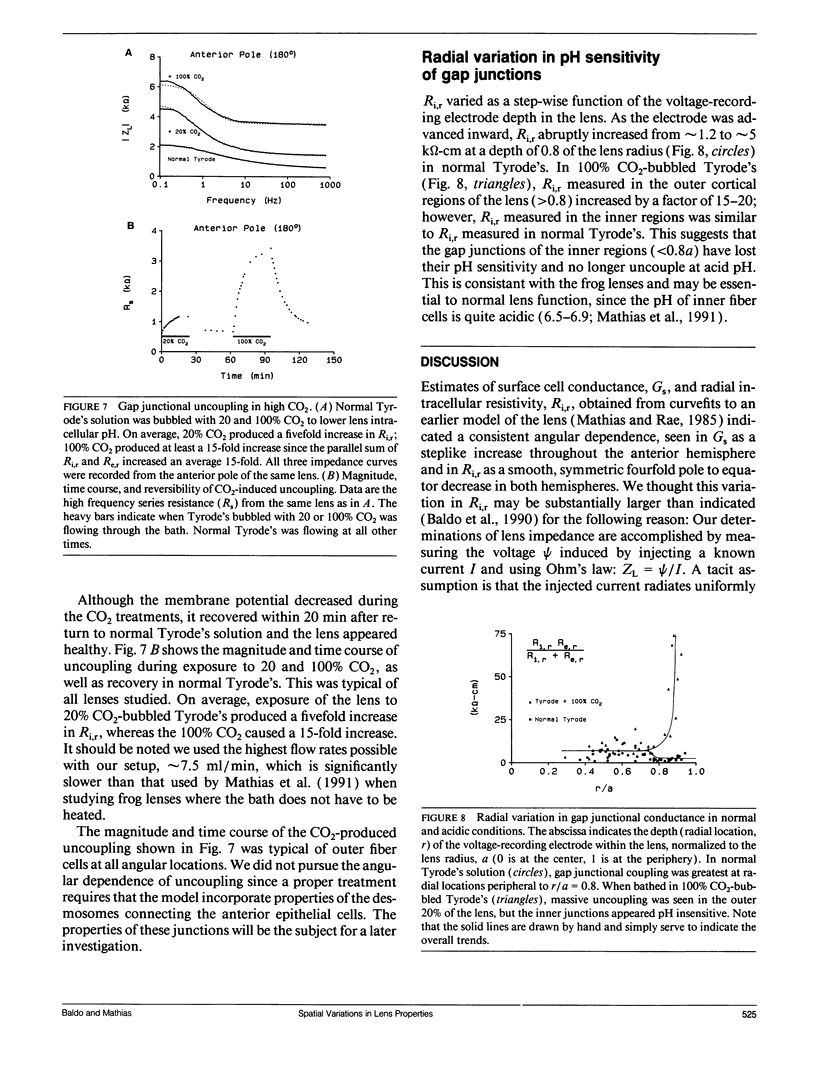
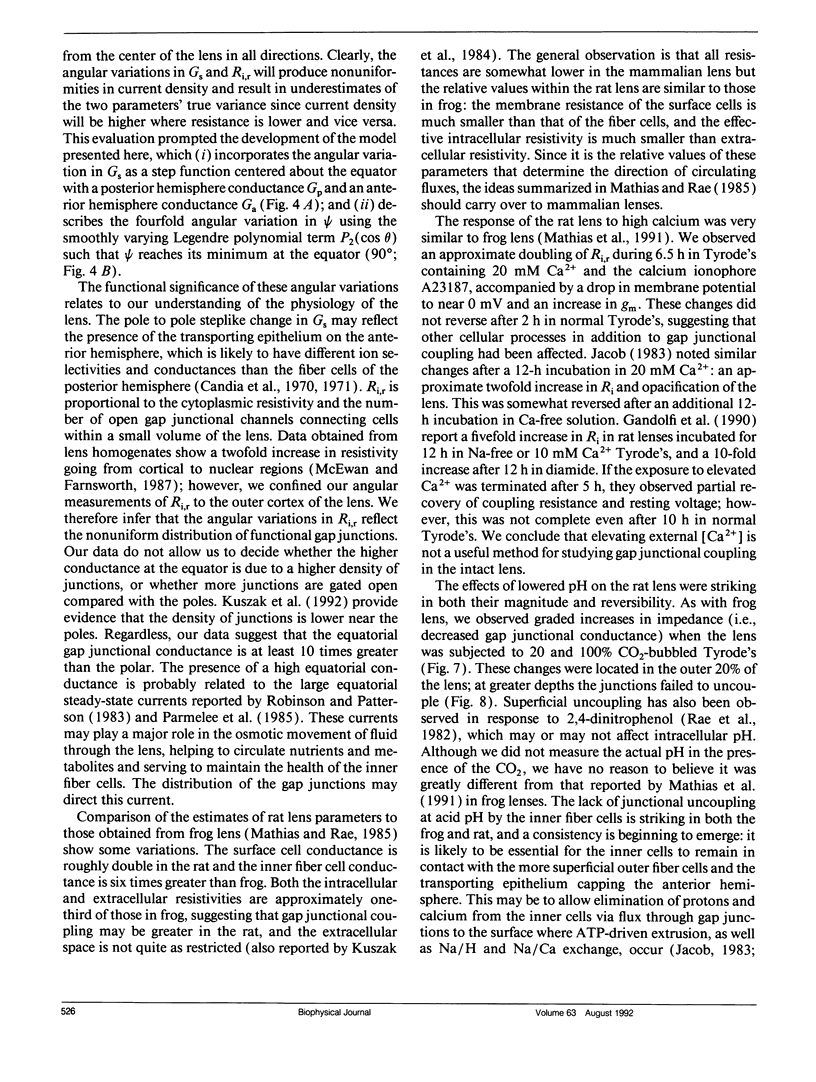
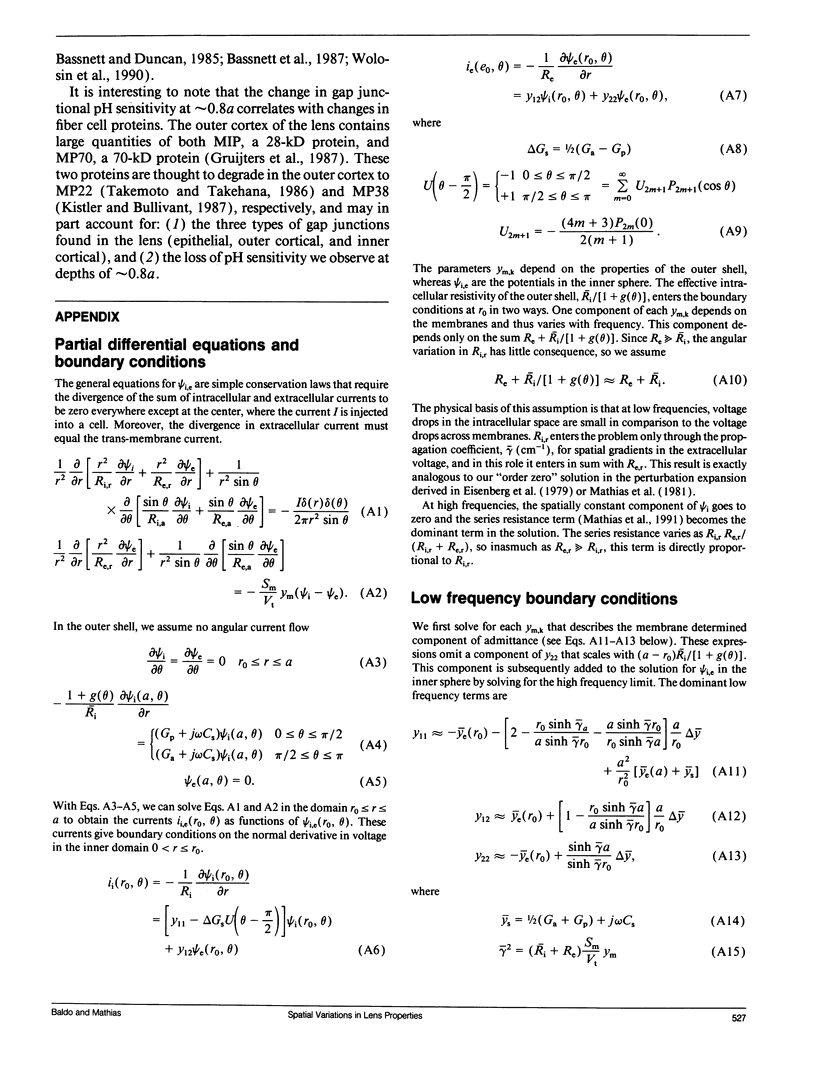
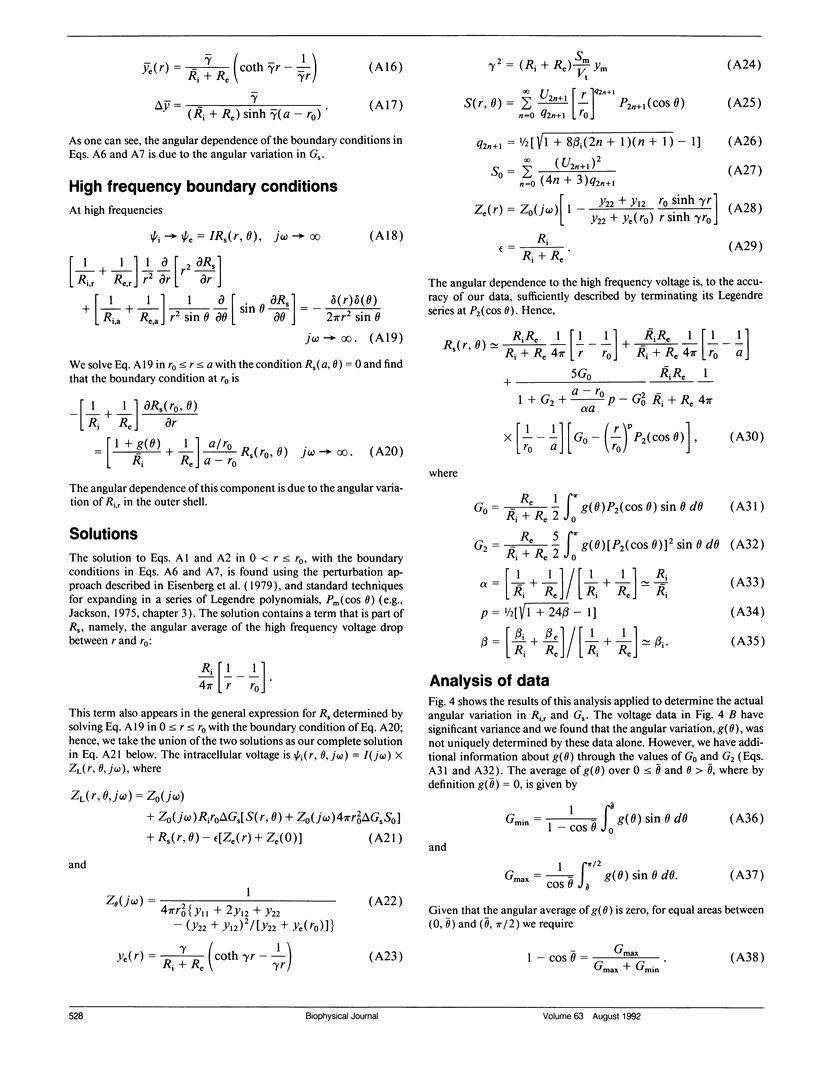
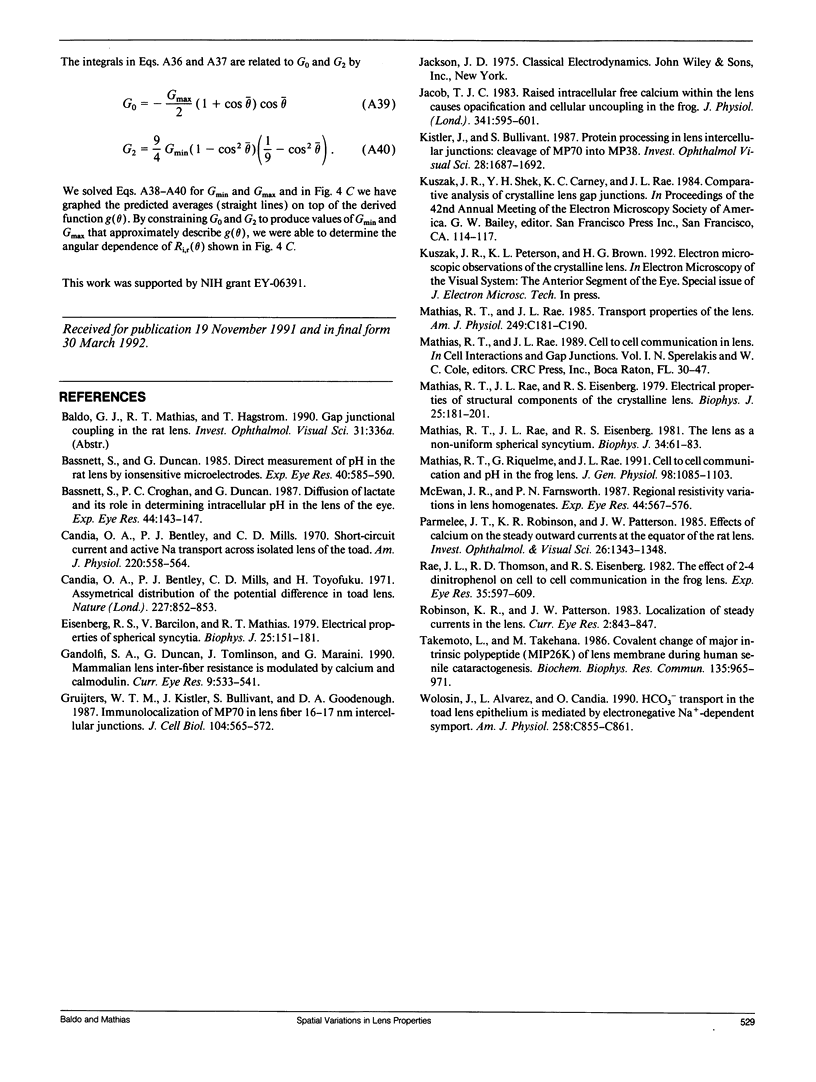
Selected References
These references are in PubMed. This may not be the complete list of references from this article.
- Bassnett S., Croghan P. C., Duncan G. Diffusion of lactate and its role in determining intracellular pH in the lens of the eye. Exp Eye Res. 1987 Jan;44(1):143–147. doi: 10.1016/s0014-4835(87)80032-5. [DOI] [PubMed] [Google Scholar]
- Bassnett S., Duncan G. Direct measurement of pH in the rat lens by ion-sensitive microelectrodes. Exp Eye Res. 1985 Apr;40(4):585–590. doi: 10.1016/0014-4835(85)90080-6. [DOI] [PubMed] [Google Scholar]
- Candia O. A., Bentley P. J., Mills C. D. Short-circuit current and active Na transport across isolated lens of the toad. Am J Physiol. 1971 Feb;220(2):558–564. doi: 10.1152/ajplegacy.1971.220.2.558. [DOI] [PubMed] [Google Scholar]
- Candia O. A., Bentley P. J., Mills C. D., Toyofuku H. Asymmetrical distribution of the potential difference in the toad lens. Nature. 1970 Aug 22;227(5260):852–853. doi: 10.1038/227852a0. [DOI] [PubMed] [Google Scholar]
- Eisenberg R. S., Barcilon V., Mathias R. T. Electrical properties of spherical syncytia. Biophys J. 1979 Jan;25(1):151–180. doi: 10.1016/S0006-3495(79)85283-2. [DOI] [PMC free article] [PubMed] [Google Scholar]
- Gandolfi S. A., Duncan G., Tomlinson J., Maraini G. Mammalian lens inter-fiber resistance is modulated by calcium and calmodulin. Curr Eye Res. 1990 Jun;9(6):533–541. doi: 10.3109/02713689008999593. [DOI] [PubMed] [Google Scholar]
- Gruijters W. T., Kistler J., Bullivant S., Goodenough D. A. Immunolocalization of MP70 in lens fiber 16-17-nm intercellular junctions. J Cell Biol. 1987 Mar;104(3):565–572. doi: 10.1083/jcb.104.3.565. [DOI] [PMC free article] [PubMed] [Google Scholar]
- Jacob T. J. Raised intracellular free calcium within the lens causes opacification and cellular uncoupling in the frog. J Physiol. 1983 Aug;341:595–601. doi: 10.1113/jphysiol.1983.sp014826. [DOI] [PMC free article] [PubMed] [Google Scholar]
- Kistler J., Bullivant S. Protein processing in lens intercellular junctions: cleavage of MP70 to MP38. Invest Ophthalmol Vis Sci. 1987 Oct;28(10):1687–1692. [PubMed] [Google Scholar]
- Mathias R. T., Rae J. L., Eisenberg R. S. Electrical properties of structural components of the crystalline lens. Biophys J. 1979 Jan;25(1):181–201. doi: 10.1016/S0006-3495(79)85284-4. [DOI] [PMC free article] [PubMed] [Google Scholar]
- Mathias R. T., Rae J. L., Eisenberg R. S. The lens as a nonuniform spherical syncytium. Biophys J. 1981 Apr;34(1):61–83. doi: 10.1016/S0006-3495(81)84837-0. [DOI] [PMC free article] [PubMed] [Google Scholar]
- Mathias R. T., Rae J. L. Transport properties of the lens. Am J Physiol. 1985 Sep;249(3 Pt 1):C181–C190. doi: 10.1152/ajpcell.1985.249.3.C181. [DOI] [PubMed] [Google Scholar]
- Mathias R. T., Riquelme G., Rae J. L. Cell to cell communication and pH in the frog lens. J Gen Physiol. 1991 Dec;98(6):1085–1103. doi: 10.1085/jgp.98.6.1085. [DOI] [PMC free article] [PubMed] [Google Scholar]
- McEwan J. R., Farnsworth P. N. Regional resistivity variations in lens homogenates. Exp Eye Res. 1987 Apr;44(4):567–576. doi: 10.1016/s0014-4835(87)80164-1. [DOI] [PubMed] [Google Scholar]
- Parmelee J. T., Robinson K. R., Patterson J. W. Effects of calcium on the steady outward currents at the equator of the rat lens. Invest Ophthalmol Vis Sci. 1985 Oct;26(10):1343–1348. [PubMed] [Google Scholar]
- Rae J. L., Thomson R. D., Eisenberg R. S. The effect of 2-4 dinitrophenol on cell to cell communication in the frog lens. Exp Eye Res. 1982 Dec;35(6):597–609. doi: 10.1016/s0014-4835(82)80073-0. [DOI] [PubMed] [Google Scholar]
- Robinson K. R., Patterson J. W. Localization of steady currents in the lens. Curr Eye Res. 1982;2(12):843–847. doi: 10.3109/02713688209020020. [DOI] [PubMed] [Google Scholar]
- Takemoto L., Takehana M. Covalent change of major intrinsic polypeptide (MIP26K) of lens membrane during human senile cataractogenesis. Biochem Biophys Res Commun. 1986 Mar 28;135(3):965–971. doi: 10.1016/0006-291x(86)91022-3. [DOI] [PubMed] [Google Scholar]
- Wolosin J. M., Alvarez L. J., Candia O. A. HCO3- transport in the toad lens epithelium is mediated by an electronegative Na(+)-dependent symport. Am J Physiol. 1990 May;258(5 Pt 1):C855–C861. doi: 10.1152/ajpcell.1990.258.5.C855. [DOI] [PubMed] [Google Scholar]