Abstract
We have simulated both conventional (V1) and saturation transfer (V'2) electron paramagnetic resonance spectra for the case of Brownian rotational diffusion restricted in angular amplitude. Numerical solutions of the diffusion-coupled Bloch equations were obtained for an axially symmetric 14N nitroxide spin label with its principal axis rotating within a Gaussian angular distribution of full width delta theta at half maximum. Spectra were first calculated for a macroscopically oriented system with cylindrical symmetry (e.g., a bundle of muscle fibers or a stack of membrane bilayers), with the Gaussian angular distribution centered at theta 0 with respect to the magnetic field. These spectra were then summed over theta 0 to obtain the spectrum of a randomly oriented sample (e.g., a dispersion of myofibrils or membrane vesicles). The angular amplitude delta theta was varied from 0 degrees, corresponding to isotropic motion (order parameter = 0). For each value of delta theta, the rotational correlation time, tau r, was varied from 10(-7) to 10(-2) s, spanning the range from maximal to minimal saturation transfer. We provide plots that illustrate the dependence of spectral parameters on delta theta and tau r. For an oriented system, the effects of changing delta theta and tau r are easily distinguishable, and both parameters can be determined unambiguously by comparing simulated and experimental spectra. For a macroscopically disordered system, the simulated spectra are still quite sensitive to delta theta, but a decrease in tau r produces changes similar to those from an increase in delta theta. If delta theta can be determined independently, then the results of the present study can be used to determine tau r from experimental spectra. Similarly, if tau r is known, then delta theta can be determined.
Full text
PDF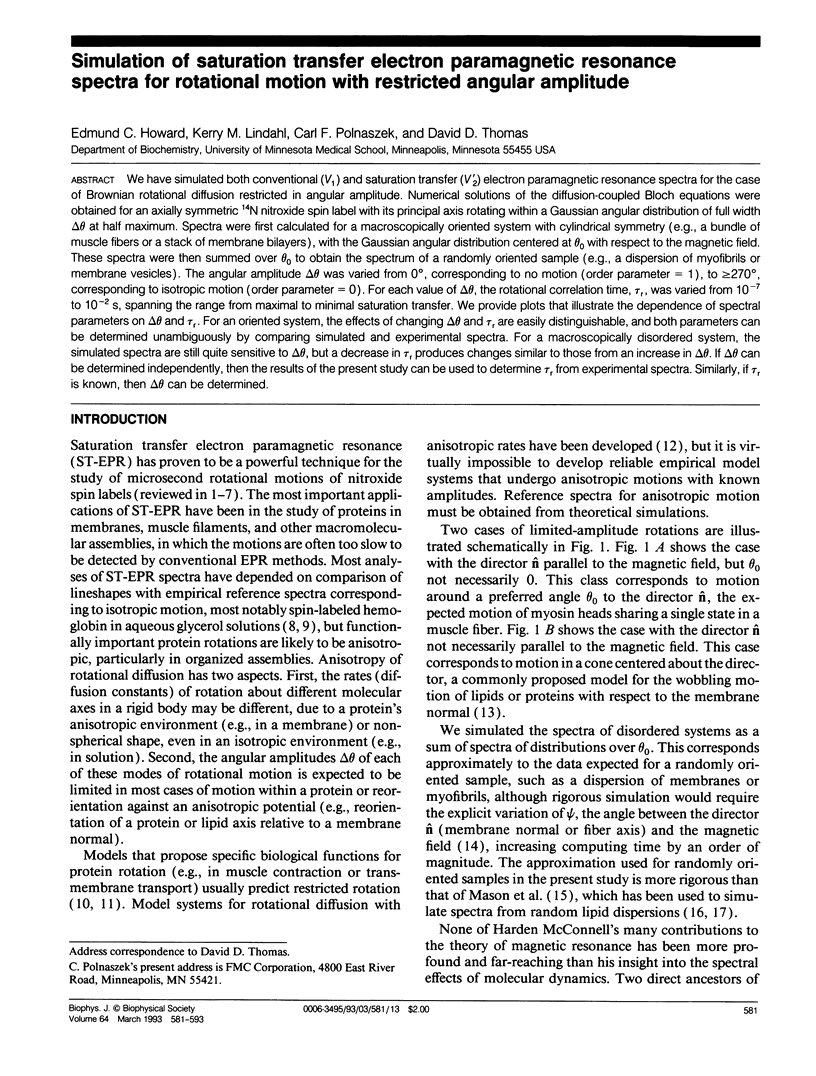
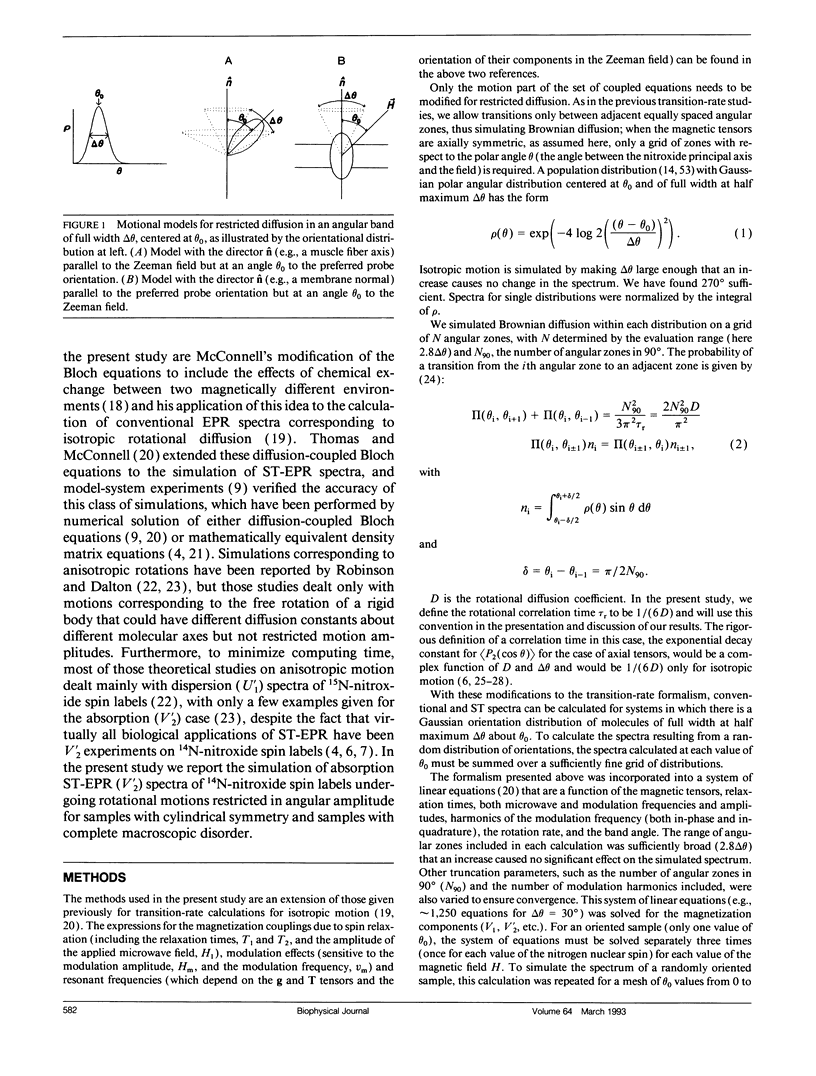
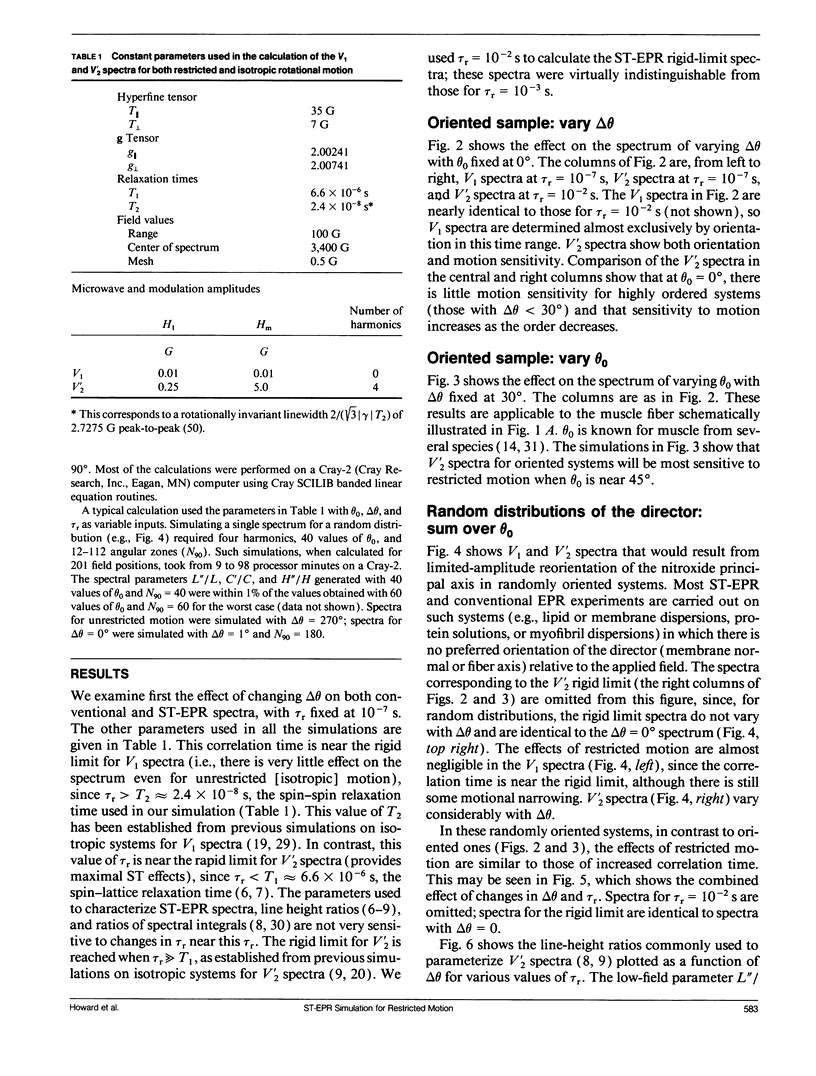
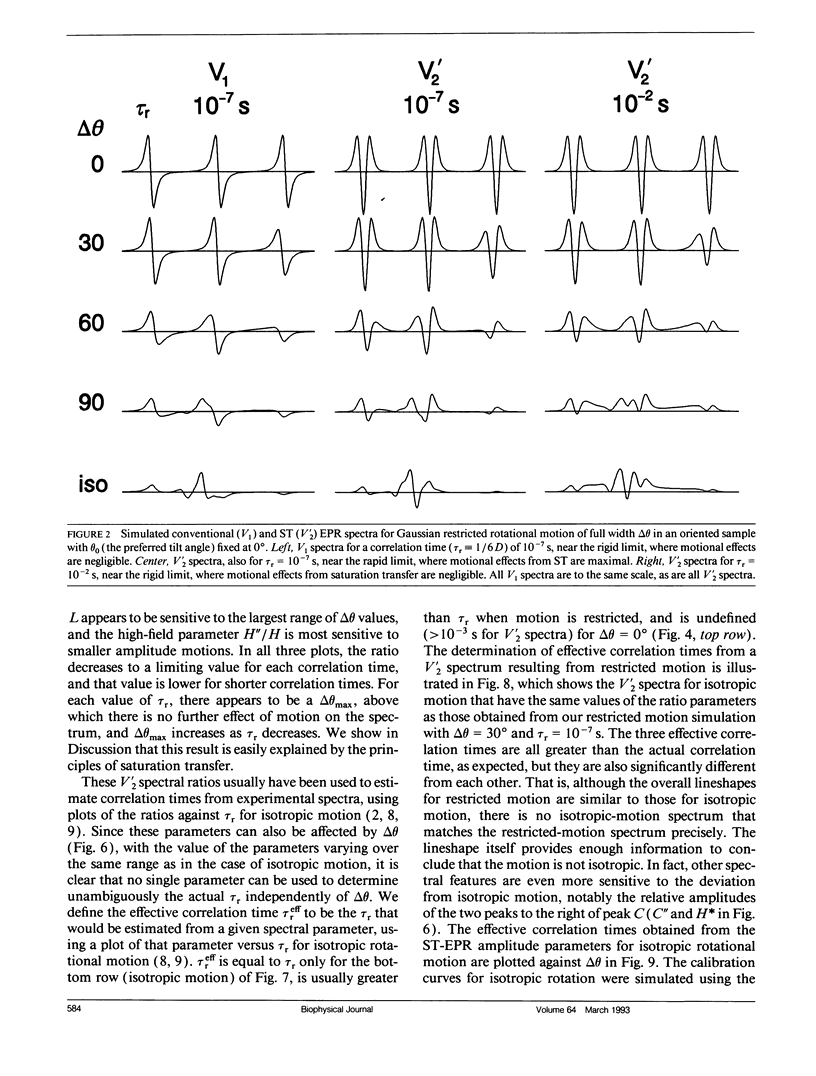
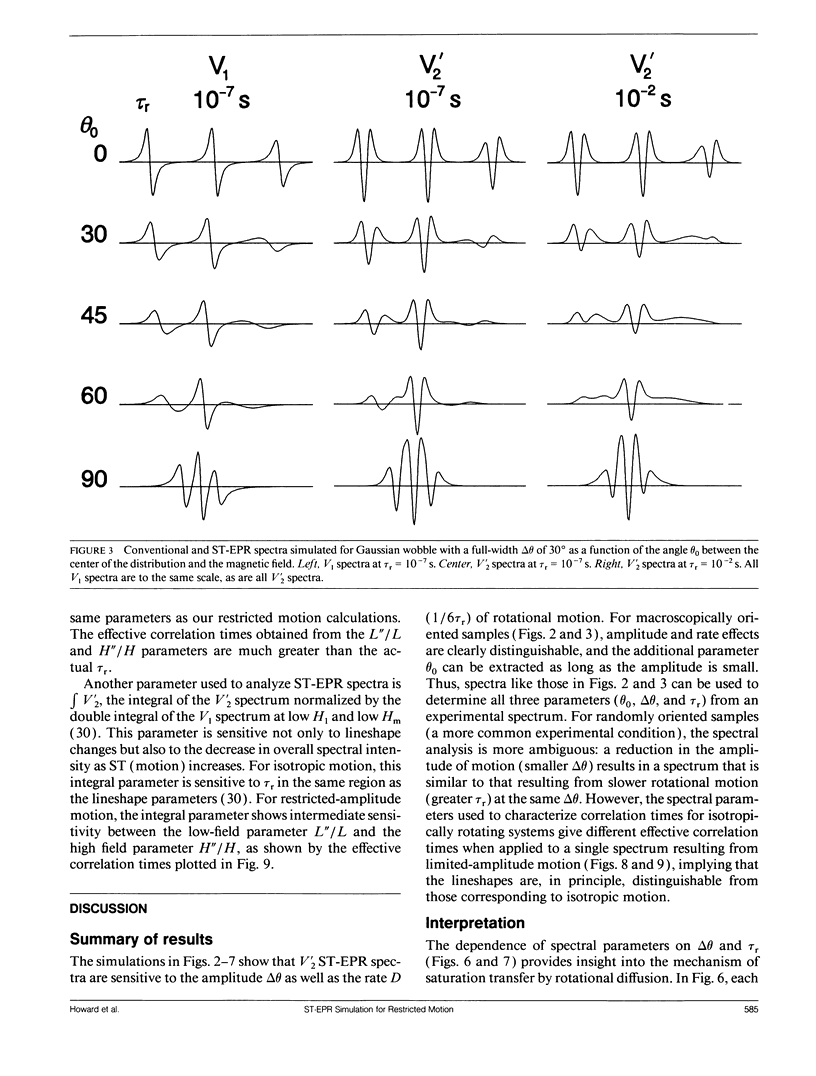
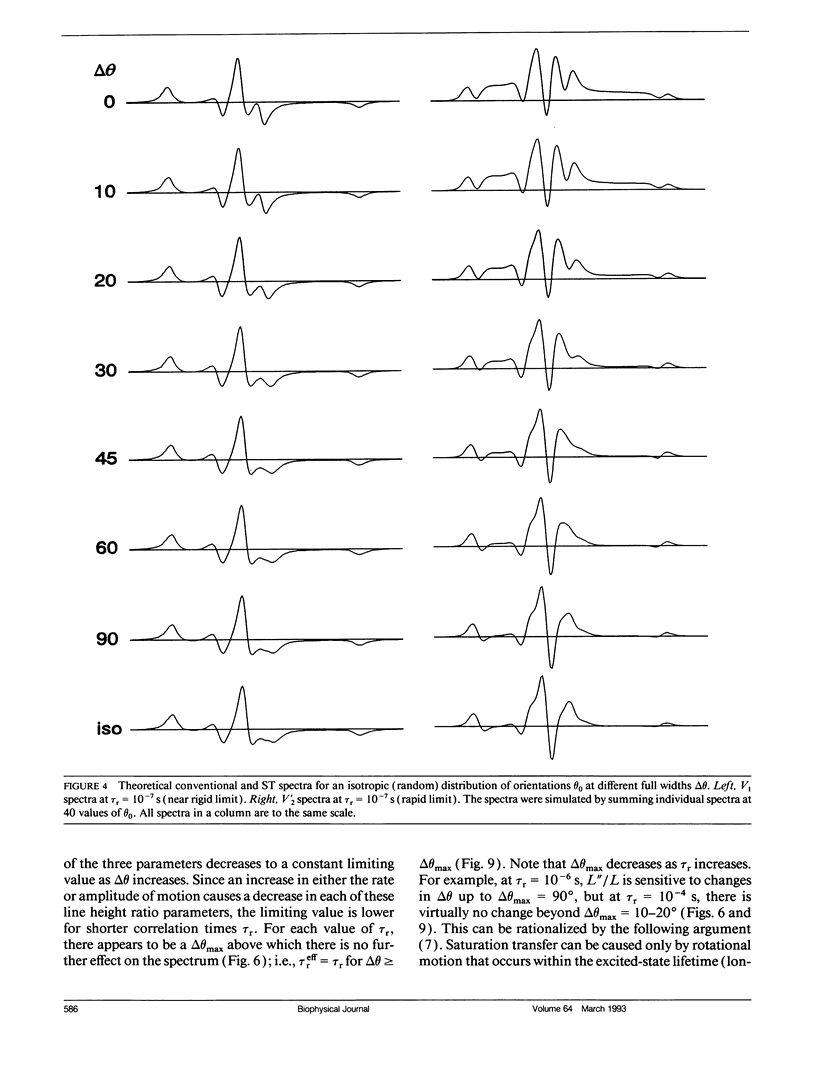
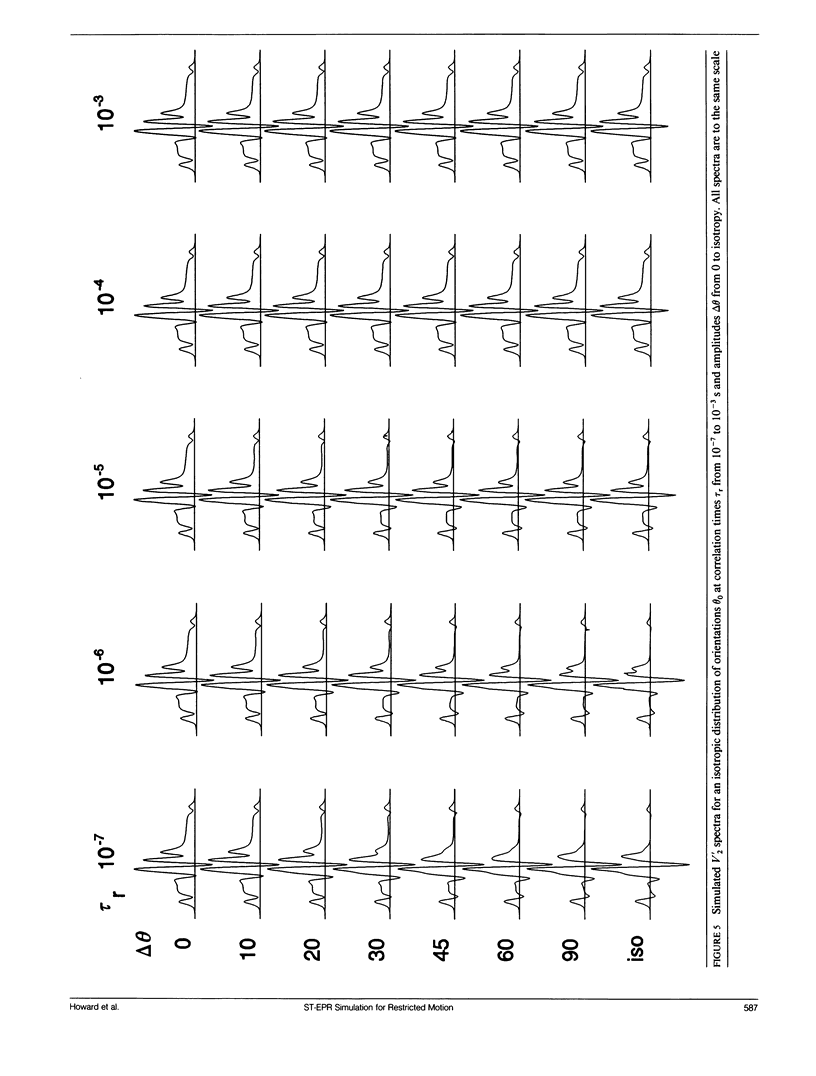
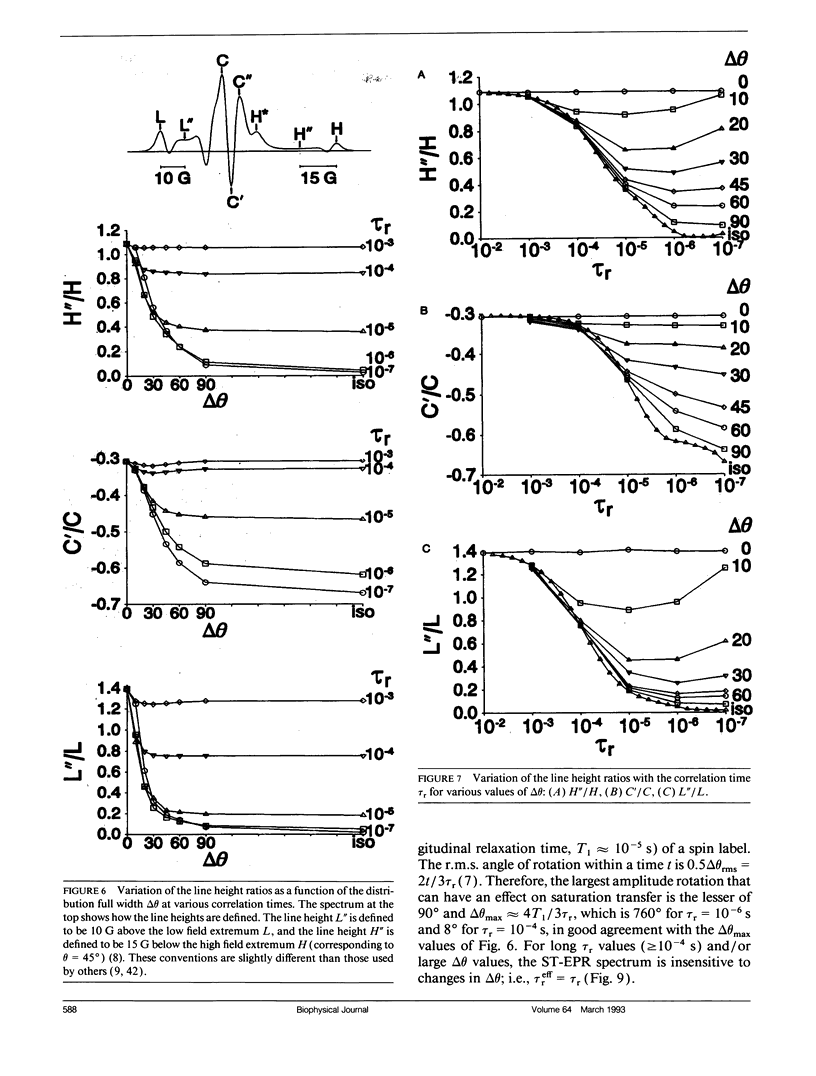
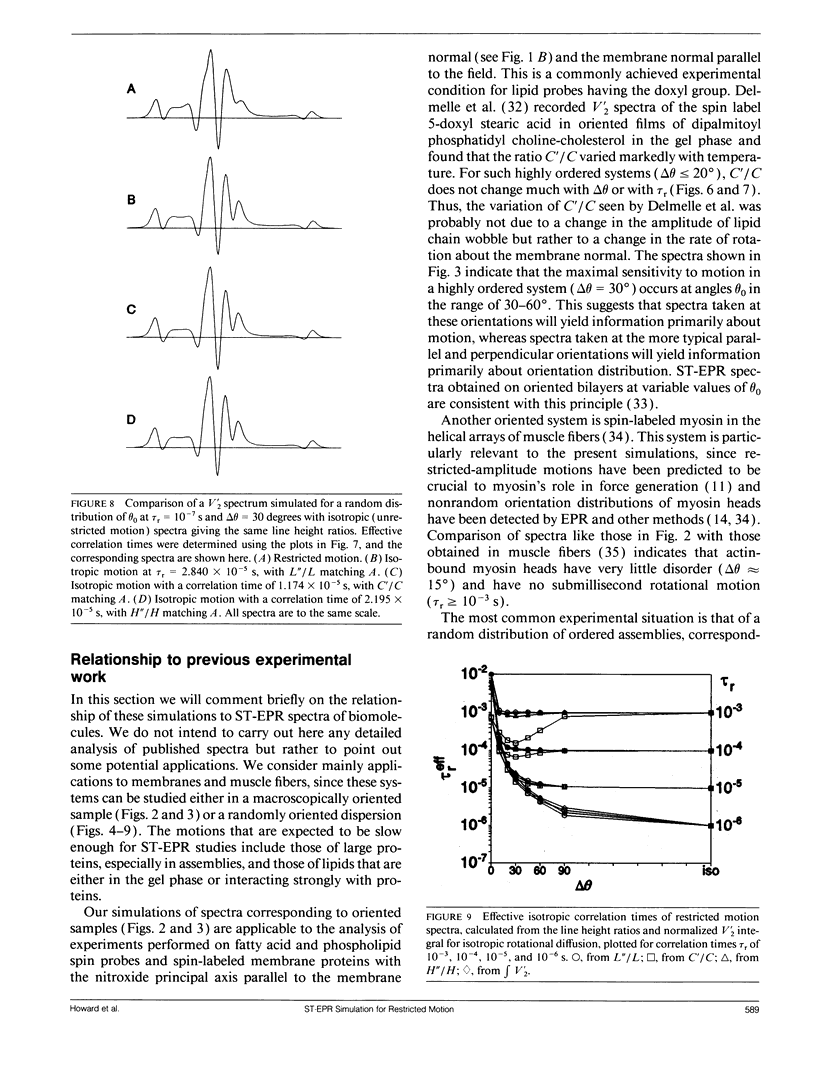
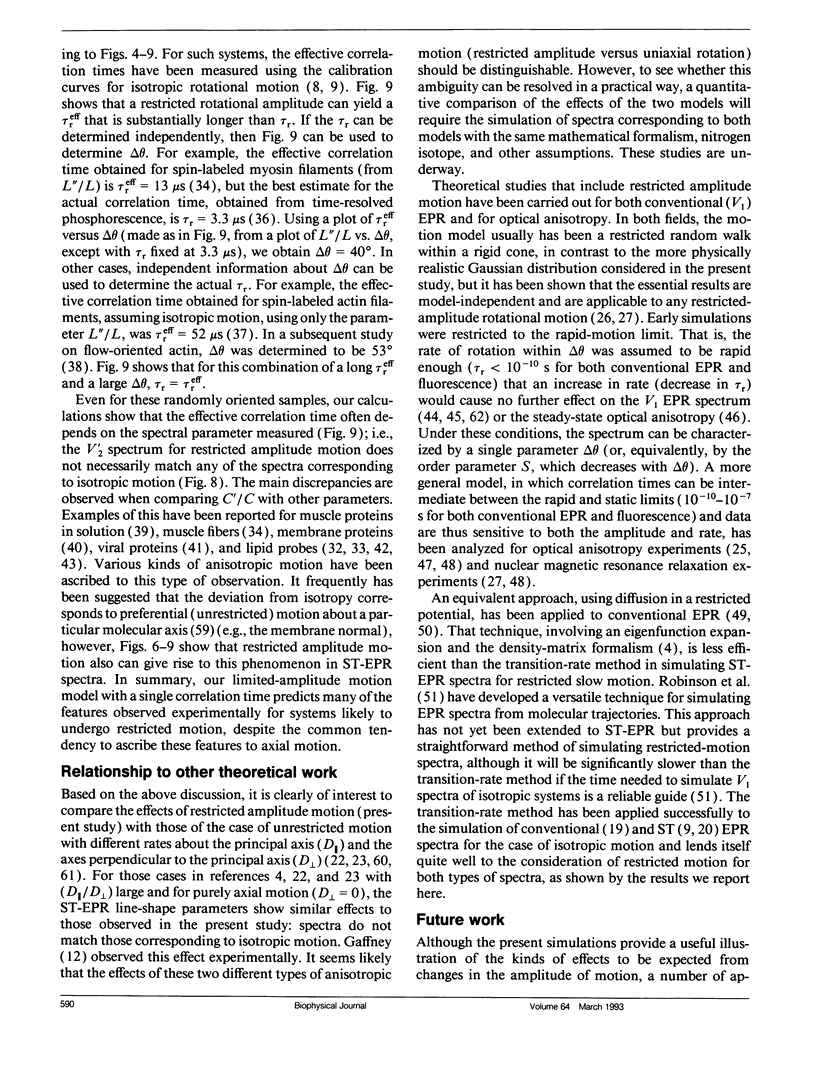
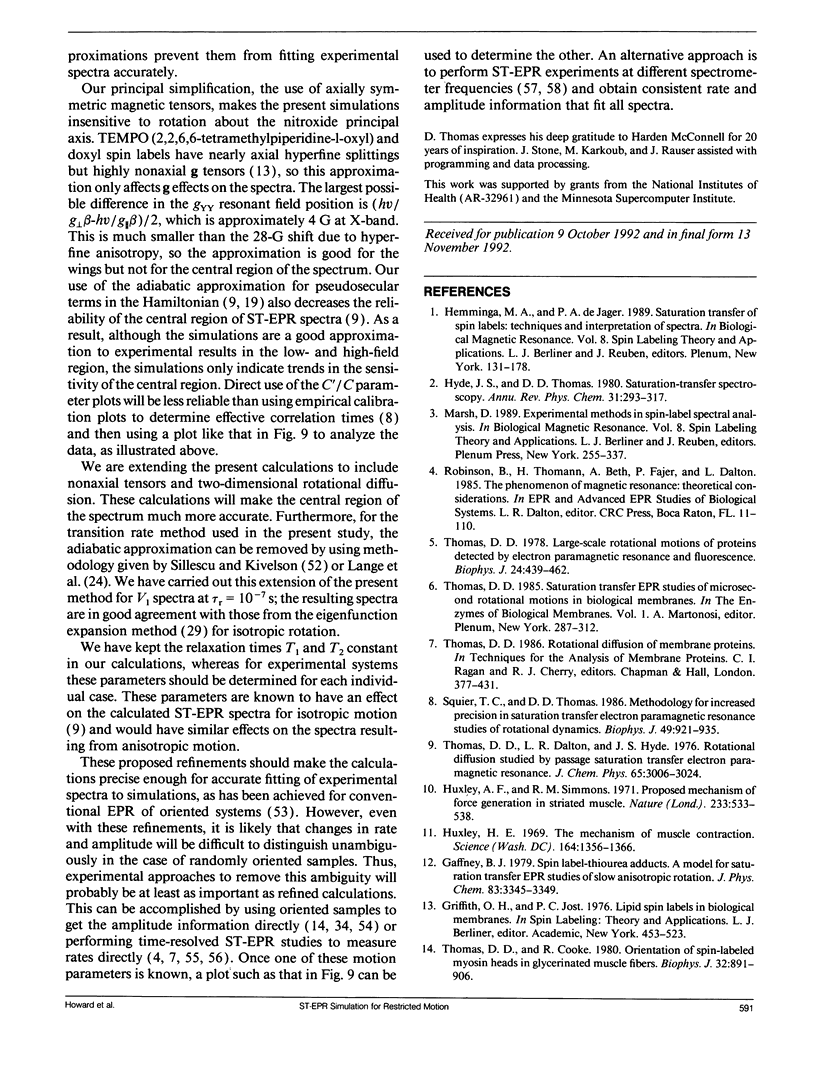
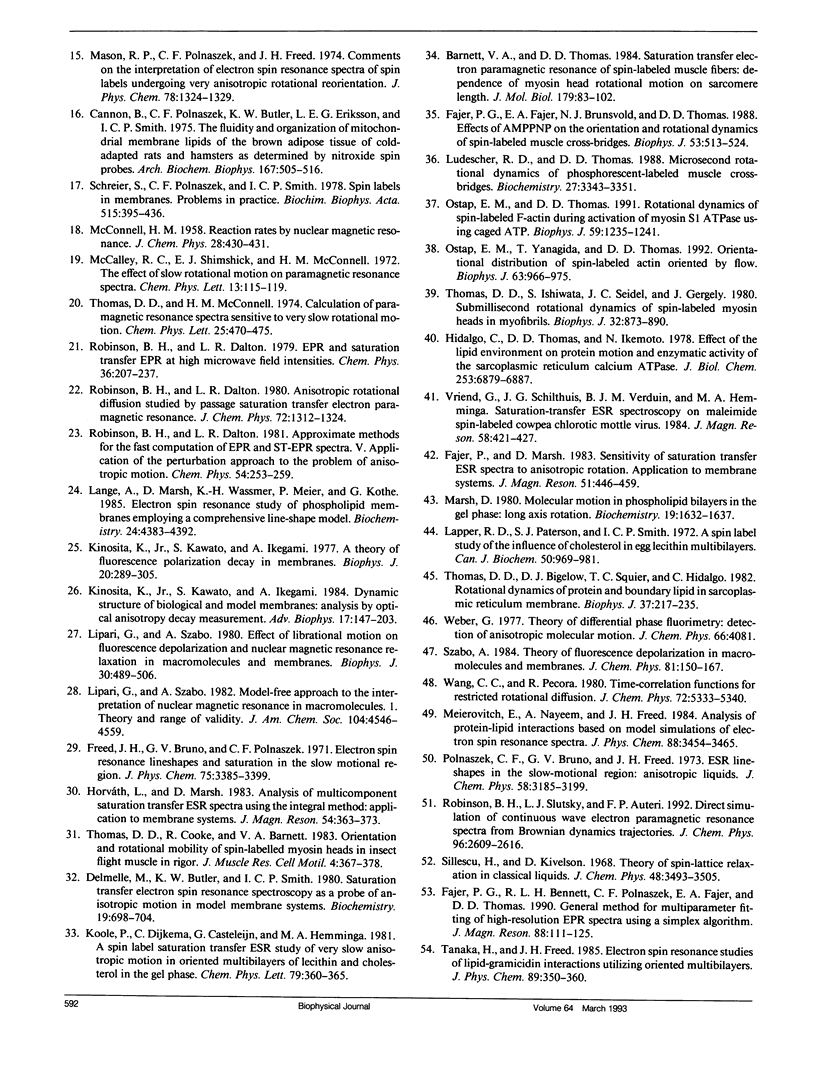
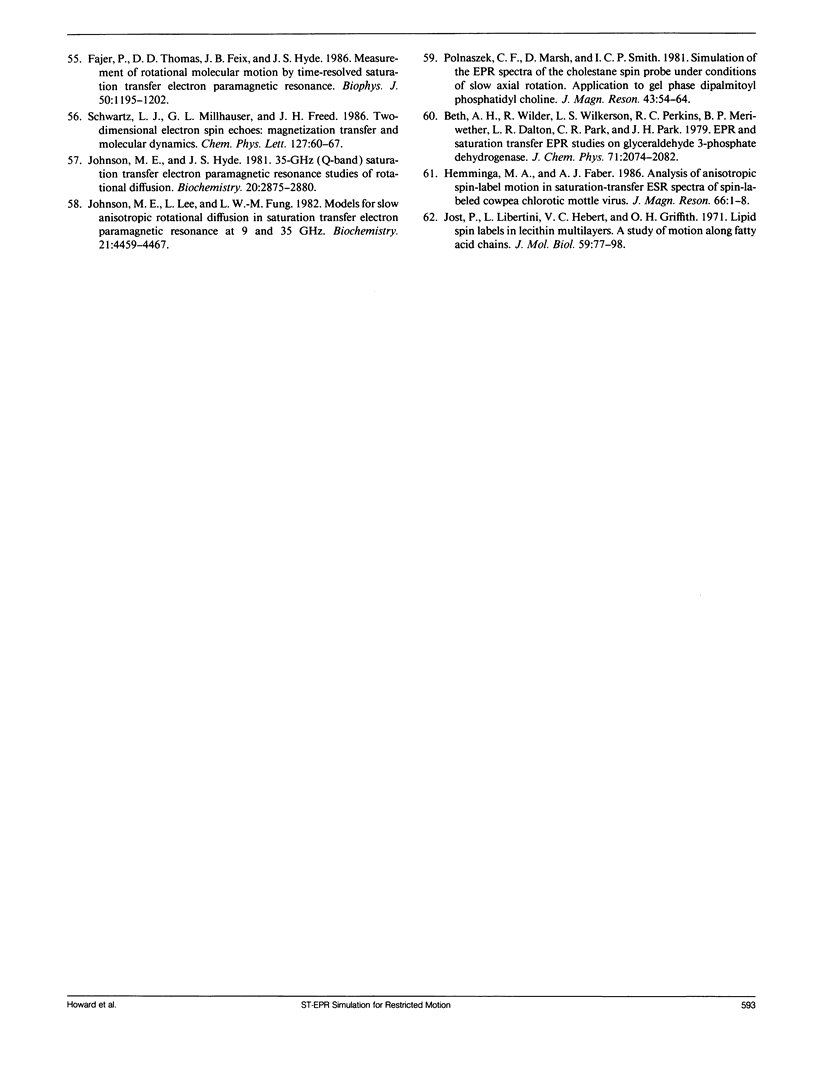
Selected References
These references are in PubMed. This may not be the complete list of references from this article.
- Barnett V. A., Thomas D. D. Saturation transfer electron paramagnetic resonance of spin-labeled muscle fibers. Dependence of myosin head rotational motion on sarcomere length. J Mol Biol. 1984 Oct 15;179(1):83–102. doi: 10.1016/0022-2836(84)90307-3. [DOI] [PubMed] [Google Scholar]
- Cannon B., Polnaszek C. F., Butler K. W., Eriksson L. E., Smith I. C. The fluidity and organization of mitochondrial membrane lipids of the brown adipose tissue of cold-adapted rats and hamsters as determined by nitroxide spin probes. Arch Biochem Biophys. 1975 Apr;167(2):505–518. doi: 10.1016/0003-9861(75)90493-2. [DOI] [PubMed] [Google Scholar]
- Delmelle M., Butler K. W., Smith I. C. Saturation transfer electron spin resonance spectroscopy as a probe of anisotropic motion in model membrane systems. Biochemistry. 1980 Feb 19;19(4):698–704. doi: 10.1021/bi00545a014. [DOI] [PubMed] [Google Scholar]
- Fajer P. G., Fajer E. A., Brunsvold N. J., Thomas D. D. Effects of AMPPNP on the orientation and rotational dynamics of spin-labeled muscle cross-bridges. Biophys J. 1988 Apr;53(4):513–524. doi: 10.1016/S0006-3495(88)83131-X. [DOI] [PMC free article] [PubMed] [Google Scholar]
- Fajer P., Thomas D. D., Feix J. B., Hyde J. S. Measurement of rotational molecular motion by time-resolved saturation transfer electron paramagnetic resonance. Biophys J. 1986 Dec;50(6):1195–1202. doi: 10.1016/S0006-3495(86)83562-7. [DOI] [PMC free article] [PubMed] [Google Scholar]
- Hidalgo C., Thomas D. D., Ikemoto N. Effect of the lipid environment on protein motion and enzymatic activity of sarcoplasmic reticulum calcium ATPase. J Biol Chem. 1978 Oct 10;253(19):6879–6887. [PubMed] [Google Scholar]
- Huxley A. F., Simmons R. M. Proposed mechanism of force generation in striated muscle. Nature. 1971 Oct 22;233(5321):533–538. doi: 10.1038/233533a0. [DOI] [PubMed] [Google Scholar]
- Huxley H. E. The mechanism of muscular contraction. Science. 1969 Jun 20;164(3886):1356–1365. doi: 10.1126/science.164.3886.1356. [DOI] [PubMed] [Google Scholar]
- Johnson M. E., Hyde J. S. 35-GHz (Q-band) saturation transfer electron paramagnetic resonance studies of rotational diffusion. Biochemistry. 1981 May 12;20(10):2875–2880. doi: 10.1021/bi00513a025. [DOI] [PubMed] [Google Scholar]
- Johnson M. E., Lee L., Fung L. W. Models for slow anisotropic rotational diffusion in saturation transfer electron paramagnetic resonance at 9 and 35 GHz. Biochemistry. 1982 Aug 31;21(18):4459–4467. doi: 10.1021/bi00261a041. [DOI] [PubMed] [Google Scholar]
- Jost P., Libertini L. J., Hebert V. C., Griffith O. H. Lipid spin labels in lecithin multilayers. A study of motion along fatty acid chains. J Mol Biol. 1971 Jul 14;59(1):77–98. doi: 10.1016/0022-2836(71)90414-1. [DOI] [PubMed] [Google Scholar]
- Kinosita K., Jr, Kawato S., Ikegami A. A theory of fluorescence polarization decay in membranes. Biophys J. 1977 Dec;20(3):289–305. doi: 10.1016/S0006-3495(77)85550-1. [DOI] [PMC free article] [PubMed] [Google Scholar]
- Kinosita K., Jr, Kawato S., Ikegami A. Dynamic structure of biological and model membranes: analysis by optical anisotropy decay measurement. Adv Biophys. 1984;17:147–203. doi: 10.1016/0065-227x(84)90027-3. [DOI] [PubMed] [Google Scholar]
- Lange A., Marsh D., Wassmer K. H., Meier P., Kothe G. Electron spin resonance study of phospholipid membranes employing a comprehensive line-shape model. Biochemistry. 1985 Jul 30;24(16):4383–4392. doi: 10.1021/bi00337a020. [DOI] [PubMed] [Google Scholar]
- Lipari G., Szabo A. Effect of librational motion on fluorescence depolarization and nuclear magnetic resonance relaxation in macromolecules and membranes. Biophys J. 1980 Jun;30(3):489–506. doi: 10.1016/S0006-3495(80)85109-5. [DOI] [PMC free article] [PubMed] [Google Scholar]
- Ludescher R. D., Thomas D. D. Microsecond rotational dynamics of phosphorescent-labeled muscle cross-bridges. Biochemistry. 1988 May 3;27(9):3343–3351. doi: 10.1021/bi00409a034. [DOI] [PubMed] [Google Scholar]
- Marsh D. Molecular motion in phospholipid bilayers in the gel phase: long axis rotation. Biochemistry. 1980 Apr 15;19(8):1632–1637. doi: 10.1021/bi00549a017. [DOI] [PubMed] [Google Scholar]
- Ostap E. M., Thomas D. D. Rotational dynamics of spin-labeled F-actin during activation of myosin S1 ATPase using caged ATP. Biophys J. 1991 Jun;59(6):1235–1241. doi: 10.1016/S0006-3495(91)82338-4. [DOI] [PMC free article] [PubMed] [Google Scholar]
- Ostap E. M., Yanagida T., Thomas D. D. Orientational distribution of spin-labeled actin oriented by flow. Biophys J. 1992 Oct;63(4):966–975. doi: 10.1016/S0006-3495(92)81684-3. [DOI] [PMC free article] [PubMed] [Google Scholar]
- Schreier S., Polnaszek C. F., Smith I. C. Spin labels in membranes. Problems in practice. Biochim Biophys Acta. 1978 Dec 15;515(4):395–436. doi: 10.1016/0304-4157(78)90011-4. [DOI] [PubMed] [Google Scholar]
- Squier T. C., Thomas D. D. Methodology for increased precision in saturation transfer electron paramagnetic resonance studies of rotational dynamics. Biophys J. 1986 Apr;49(4):921–935. doi: 10.1016/S0006-3495(86)83720-1. [DOI] [PMC free article] [PubMed] [Google Scholar]
- Thomas D. D., Bigelow D. J., Squier T. C., Hidalgo C. Rotational dynamics of protein and boundary lipid in sarcoplasmic reticulum membrane. Biophys J. 1982 Jan;37(1):217–225. doi: 10.1016/S0006-3495(82)84671-7. [DOI] [PMC free article] [PubMed] [Google Scholar]
- Thomas D. D., Cooke R., Barnett V. A. Orientation and rotational mobility of spin-labelled myosin heads in insect flight muscle in rigor. J Muscle Res Cell Motil. 1983 Jun;4(3):367–378. doi: 10.1007/BF00712002. [DOI] [PubMed] [Google Scholar]
- Thomas D. D., Cooke R. Orientation of spin-labeled myosin heads in glycerinated muscle fibers. Biophys J. 1980 Dec;32(3):891–906. doi: 10.1016/S0006-3495(80)85024-7. [DOI] [PMC free article] [PubMed] [Google Scholar]
- Thomas D. D., Ishiwata S., Seidel J. C., Gergely J. Submillisecond rotational dynamics of spin-labeled myosin heads in myofibrils. Biophys J. 1980 Dec;32(3):873–889. doi: 10.1016/S0006-3495(80)85023-5. [DOI] [PMC free article] [PubMed] [Google Scholar]
- Thomas D. D. Large-scale rotational motions of proteins detected by electron paramagnetic resonance and fluorescence. Biophys J. 1978 Nov;24(2):439–462. doi: 10.1016/S0006-3495(78)85394-6. [DOI] [PMC free article] [PubMed] [Google Scholar]