Abstract
Flux through an open ionic channel is analyzed with Poisson-Nernst-Planck (PNP) theory. The channel protein is described as an unchanging but nonuniform distribution of permanent charge, the charge distribution observed (in principle) in x-ray diffraction. Appropriate boundary conditions are derived and presented in some generality. Three kinds of charge are present: (a) permanent charge on the atoms of the protein, the charge independent of the electric field; (b) free or mobile charge, carried by ions in the pore as they flux through the channel; and (c) induced (sometimes called polarization) charge, in the pore and protein, created by the electric field, zero when the electric field is zero. The permanent charge produces an offset in potential, a built-in Donnan potential at both ends of the channel pore. The system is completely solved for bathing solutions of two ions. Graphs describe the distribution of potential, concentration, free (i.e., mobile) and induced charge, and the potential energy associated with the concentration of charge, as well as the unidirectional flux as a function of concentration of ions in the bath, for a distribution of permanent charge that is uniform. The model shows surprising complexity, exhibiting some (but not all) of the properties usually attributed to single filing and exchange diffusion. The complexity arises because the arrangement of free and induced charge, and thus of potential and potential energy, varies, sometimes substantially, as conditions change, even though the channel structure and conformation (of permanent charge) is strictly constant. Energy barriers and wells, and the concomitant binding sites and binding phenomena, are outputs of the PNP theory: they are computed, not assumed. They vary in size and location as experimental conditions change, while the conformation of permanent charge remains constant, thus giving the model much of its interesting behavior.
Full text
PDF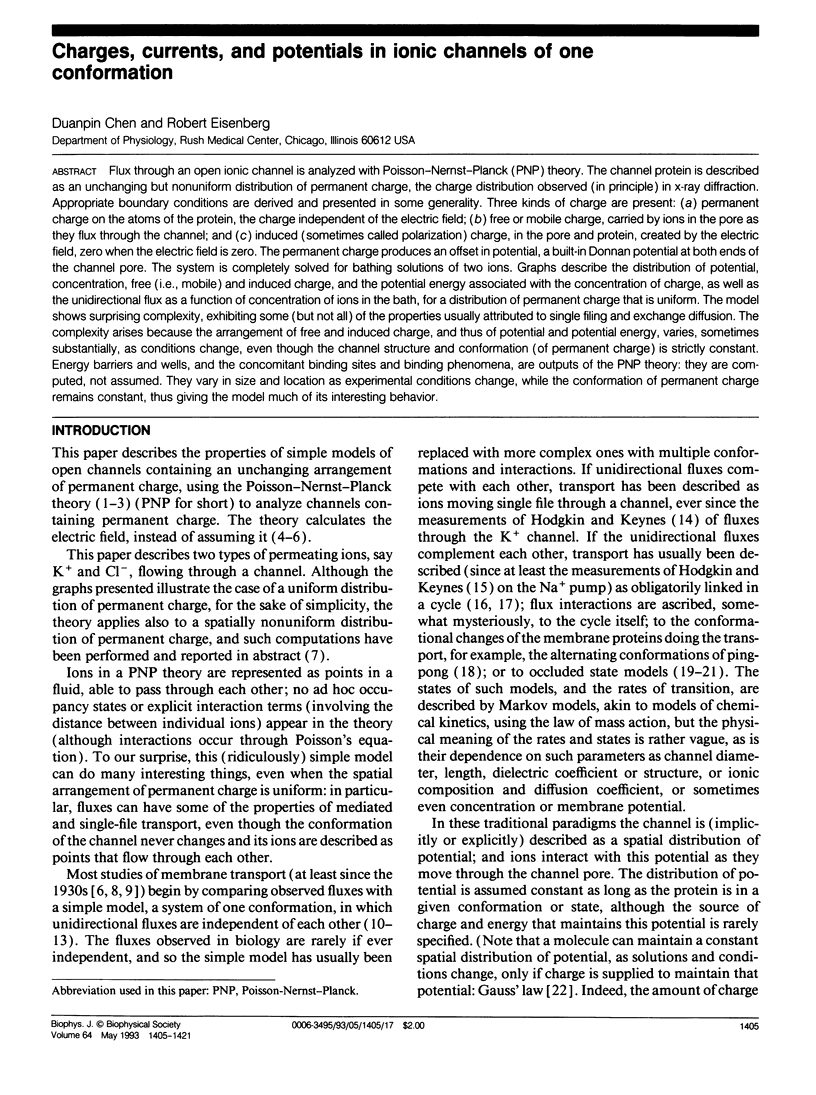
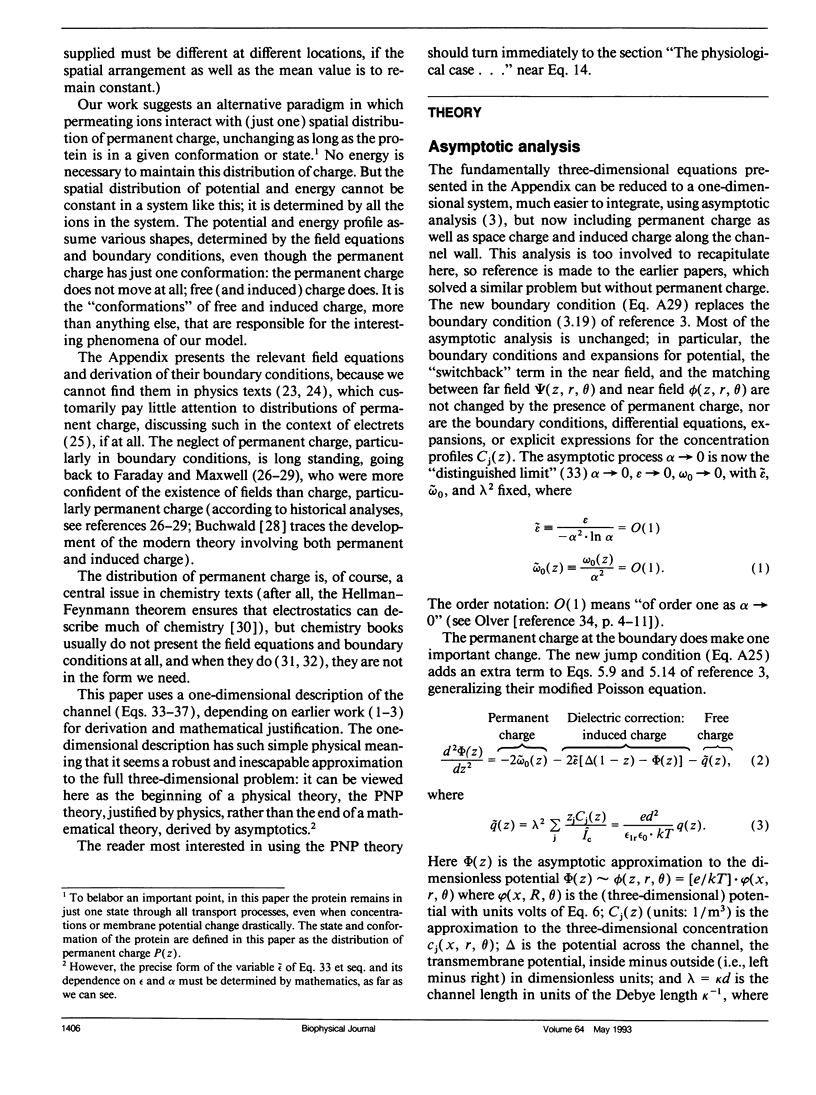
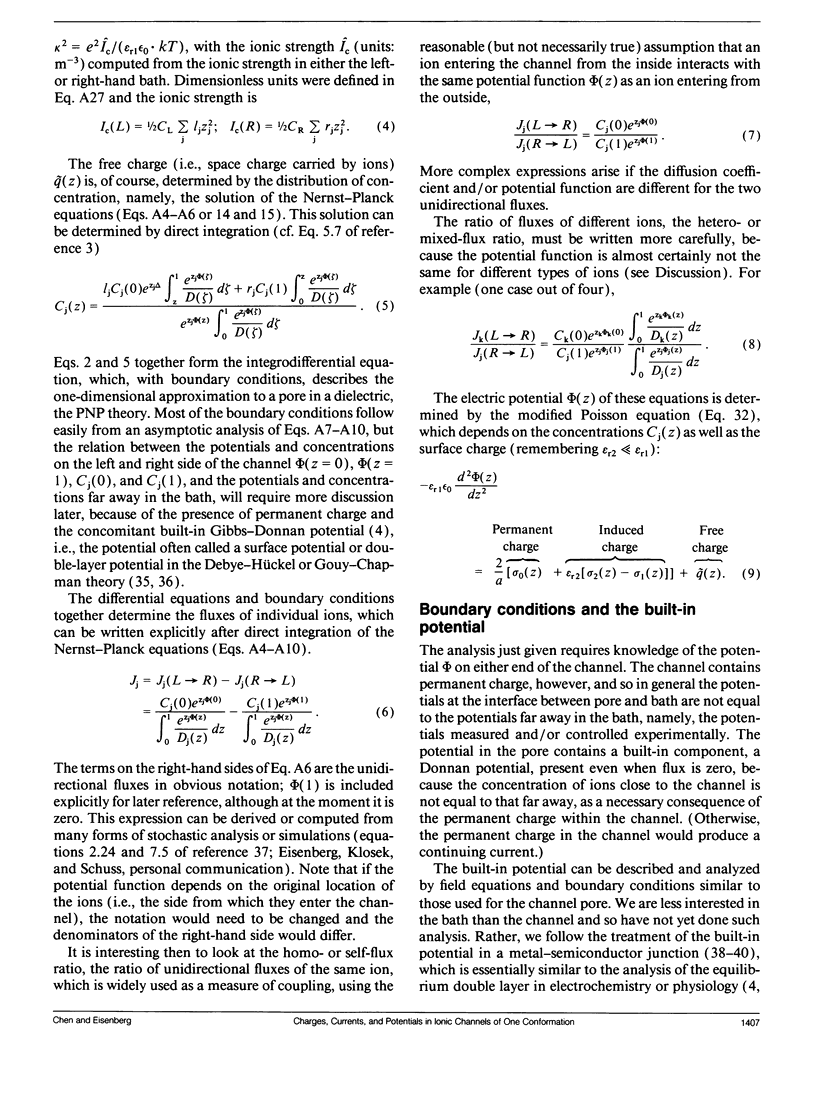
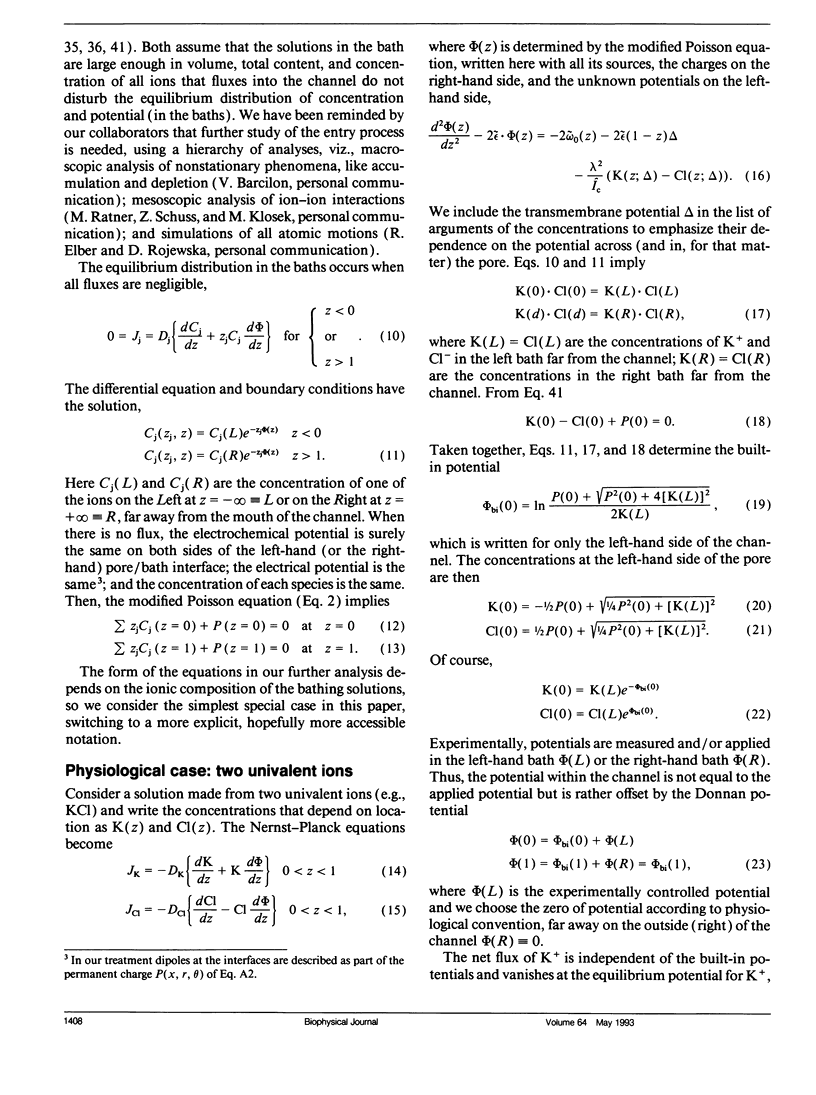
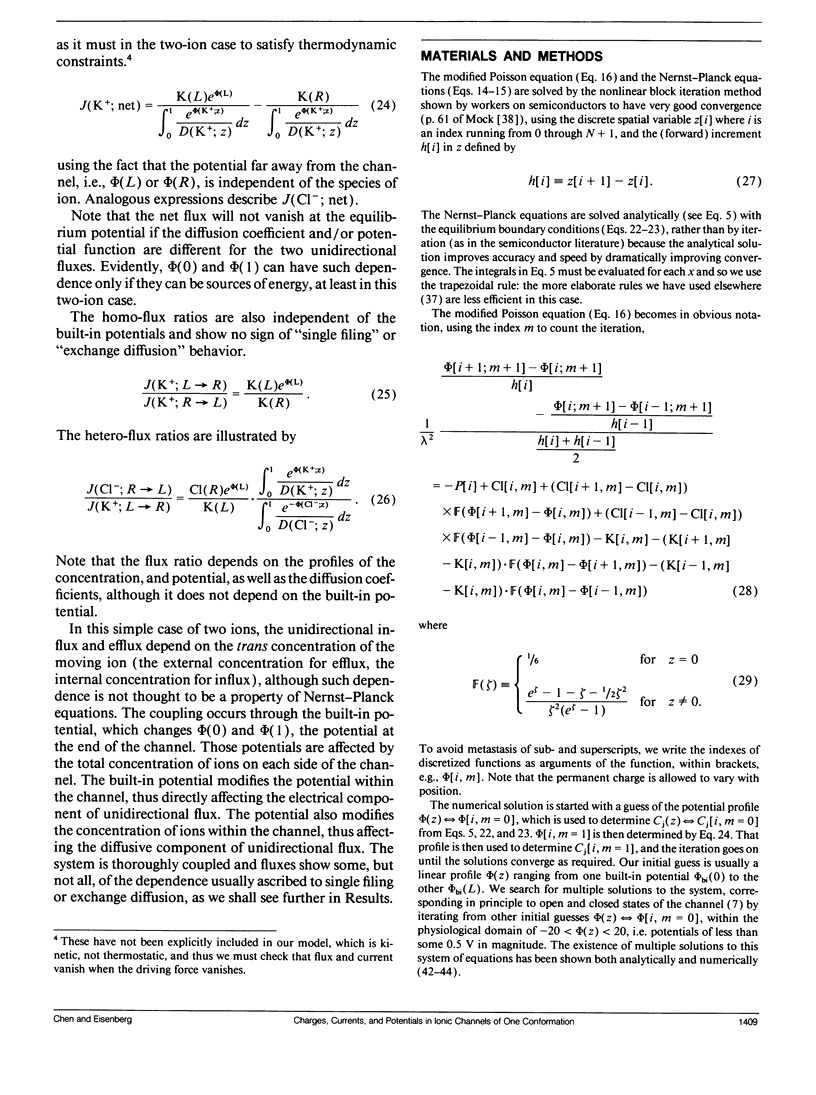
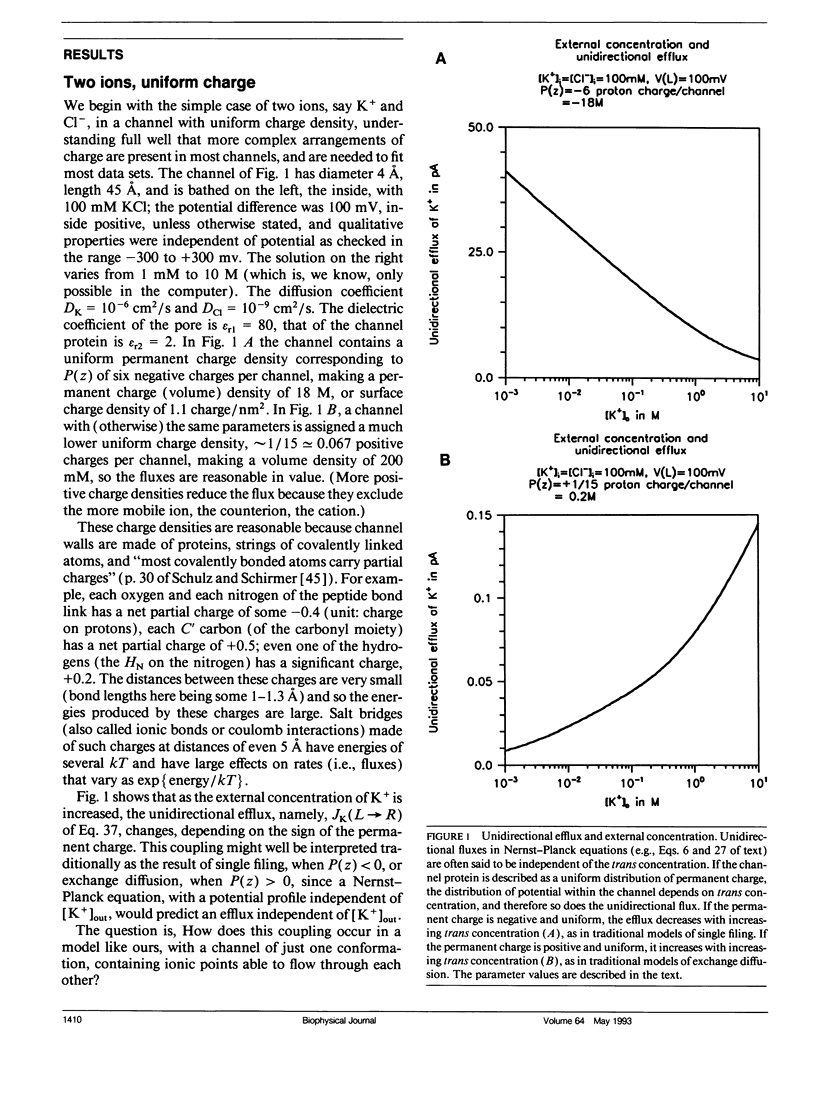
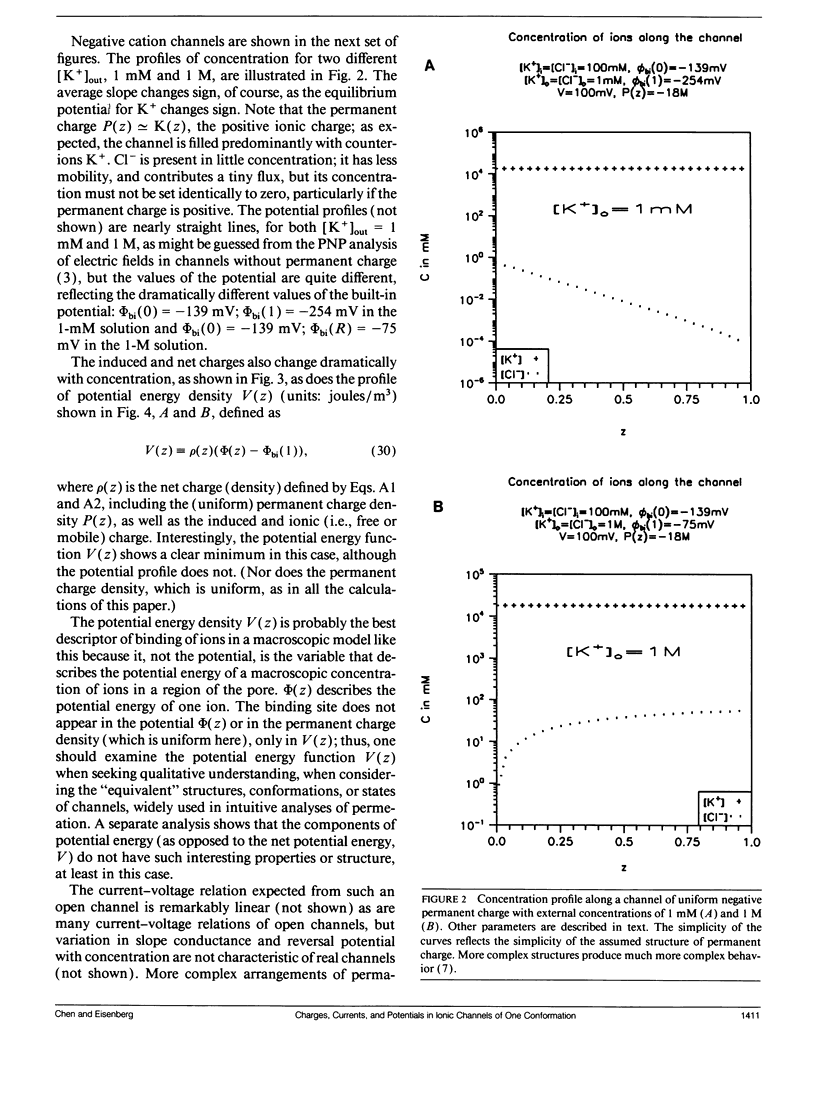
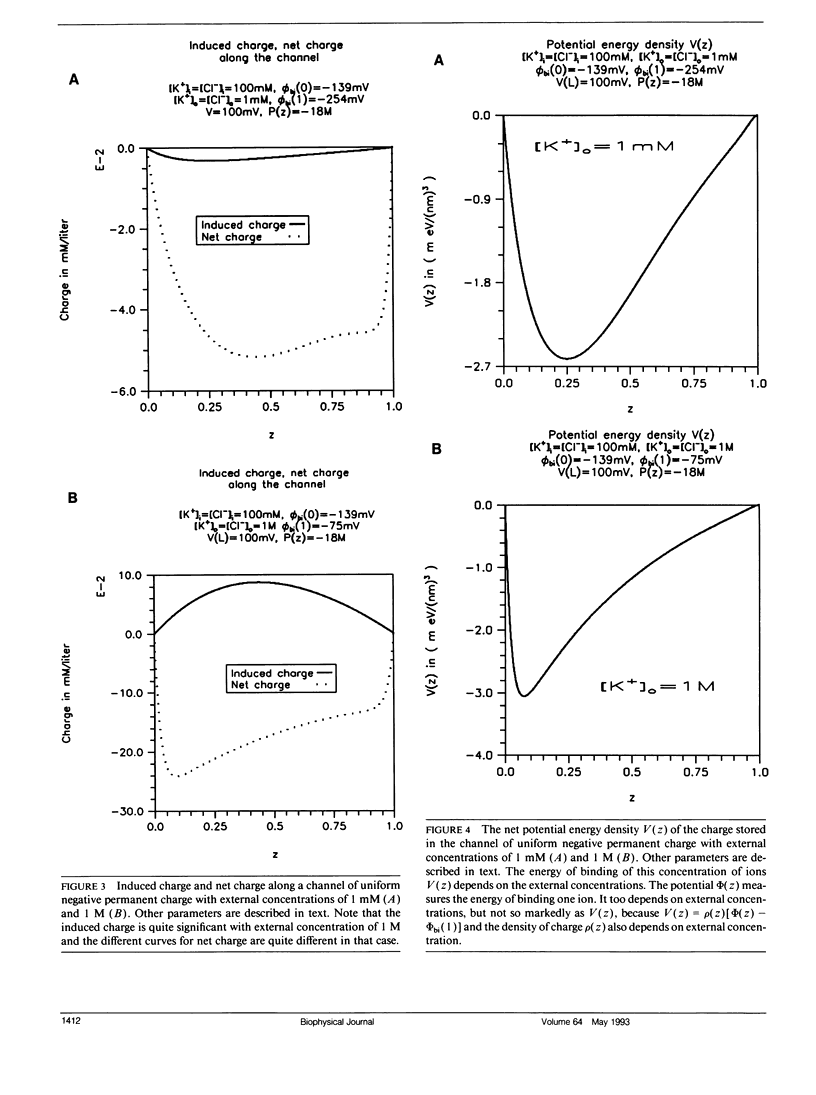
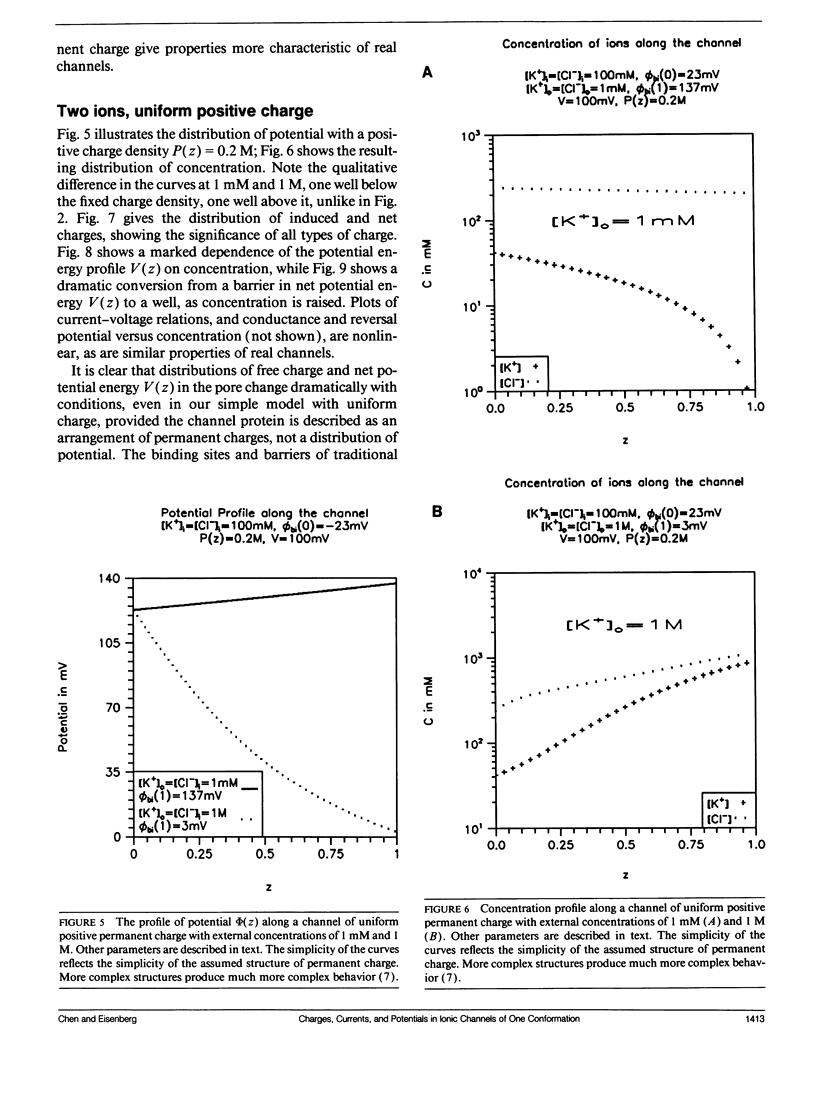
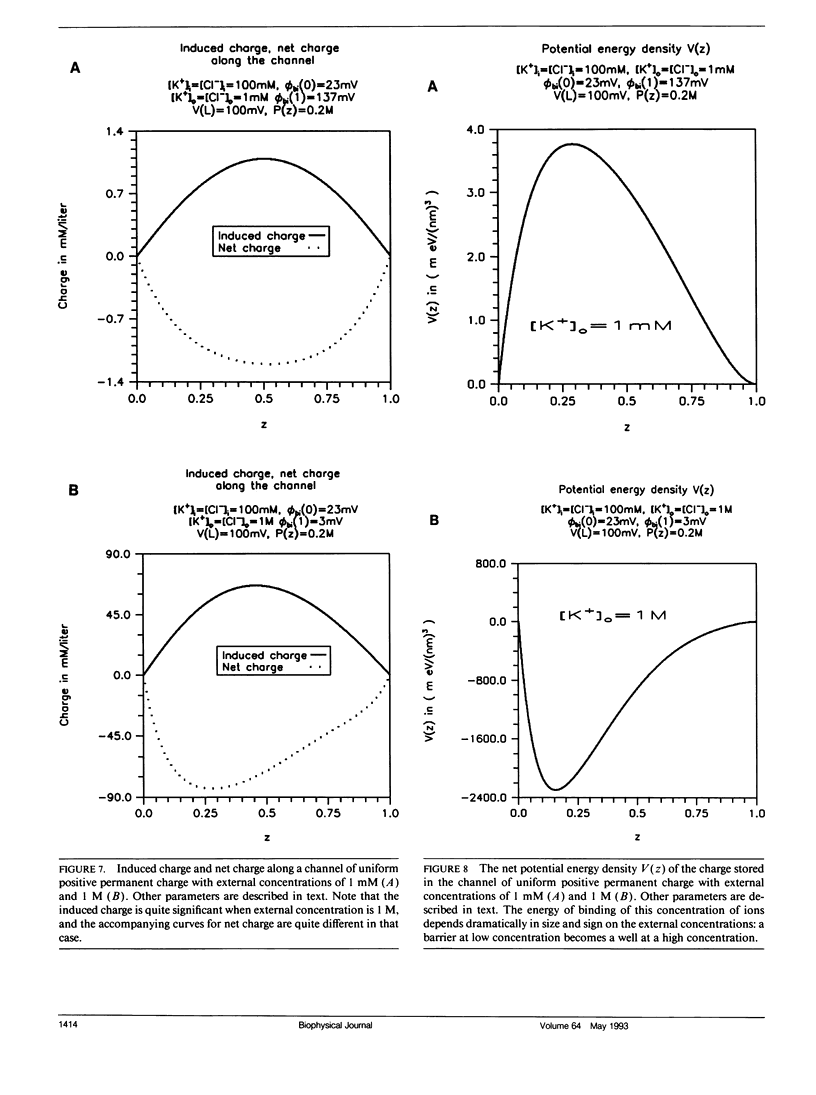
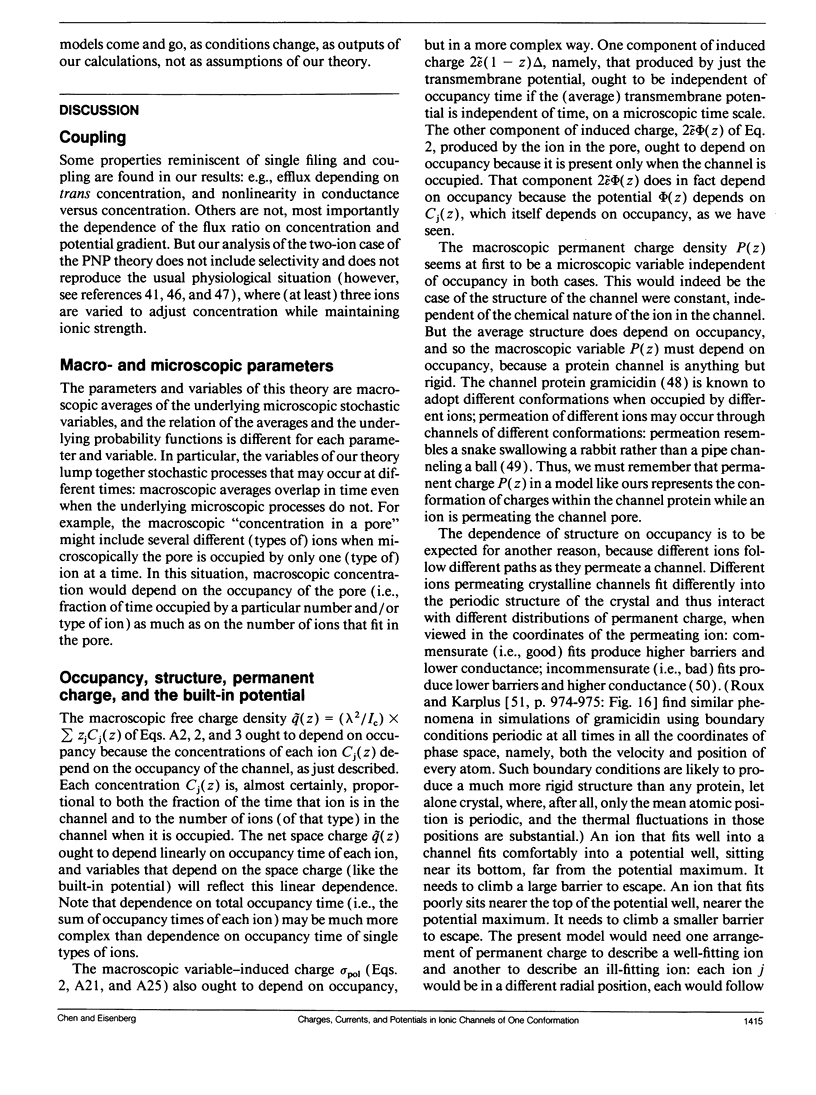
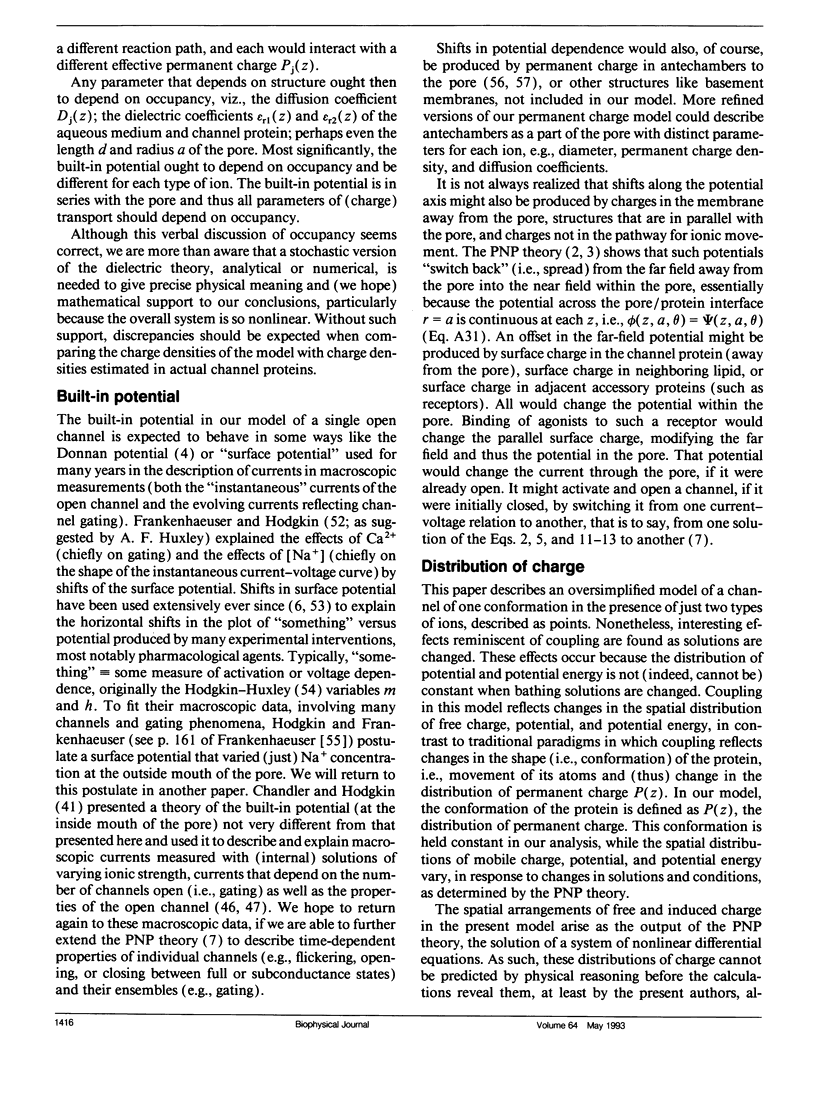
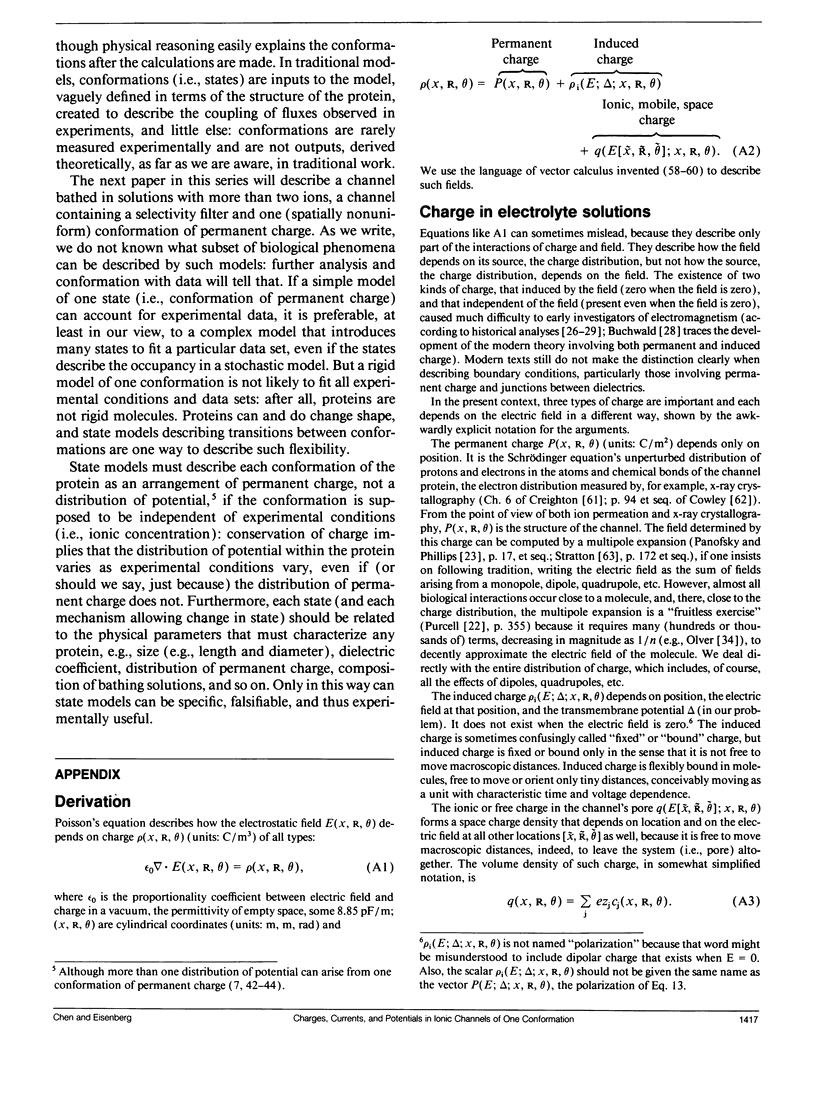
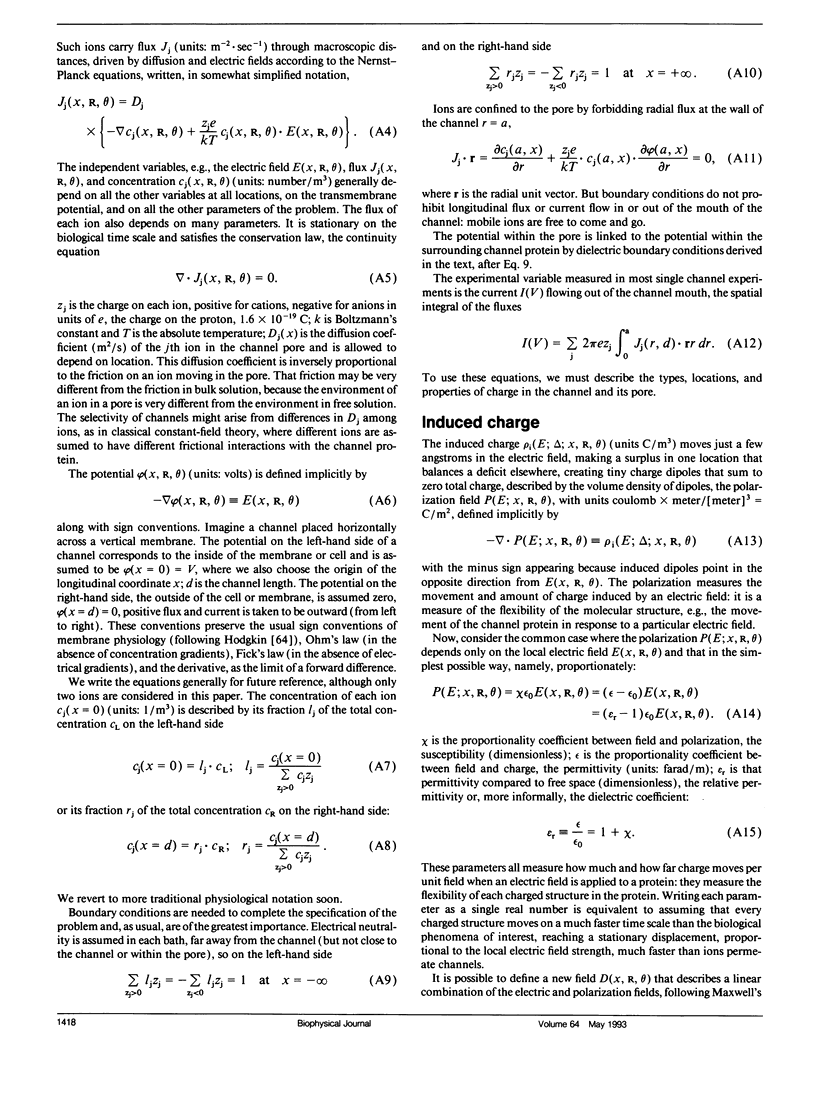
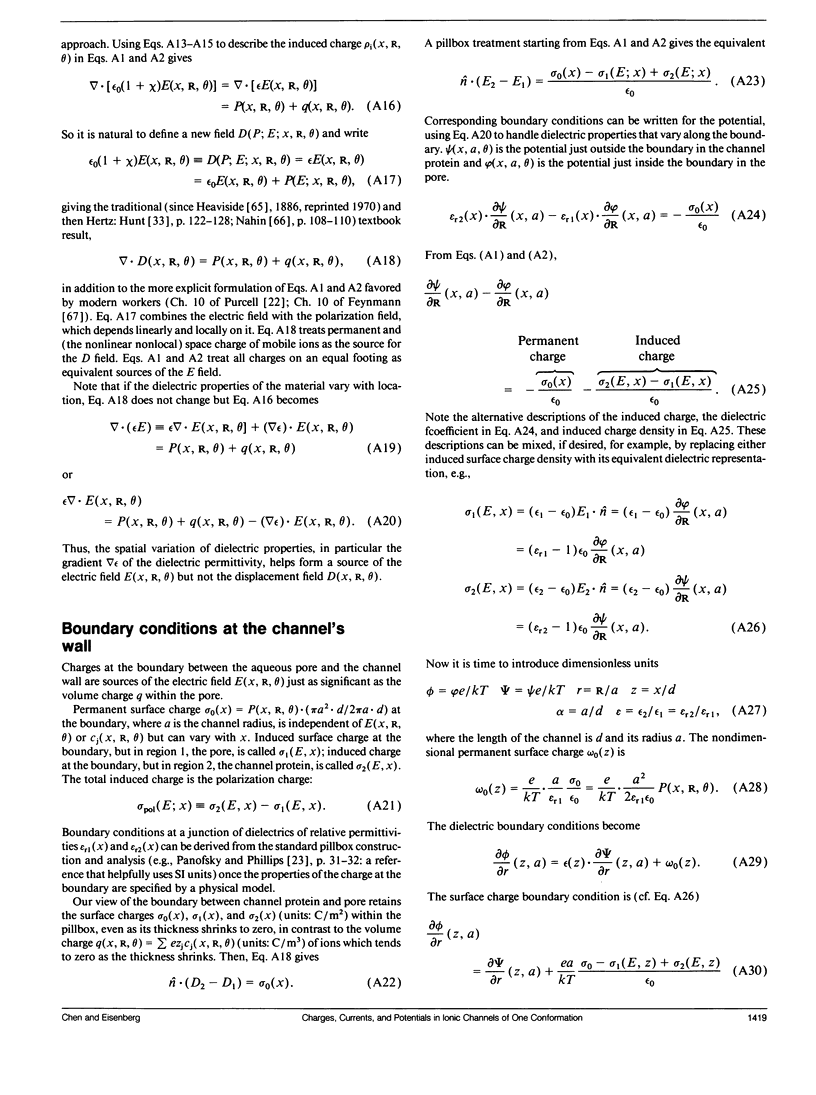
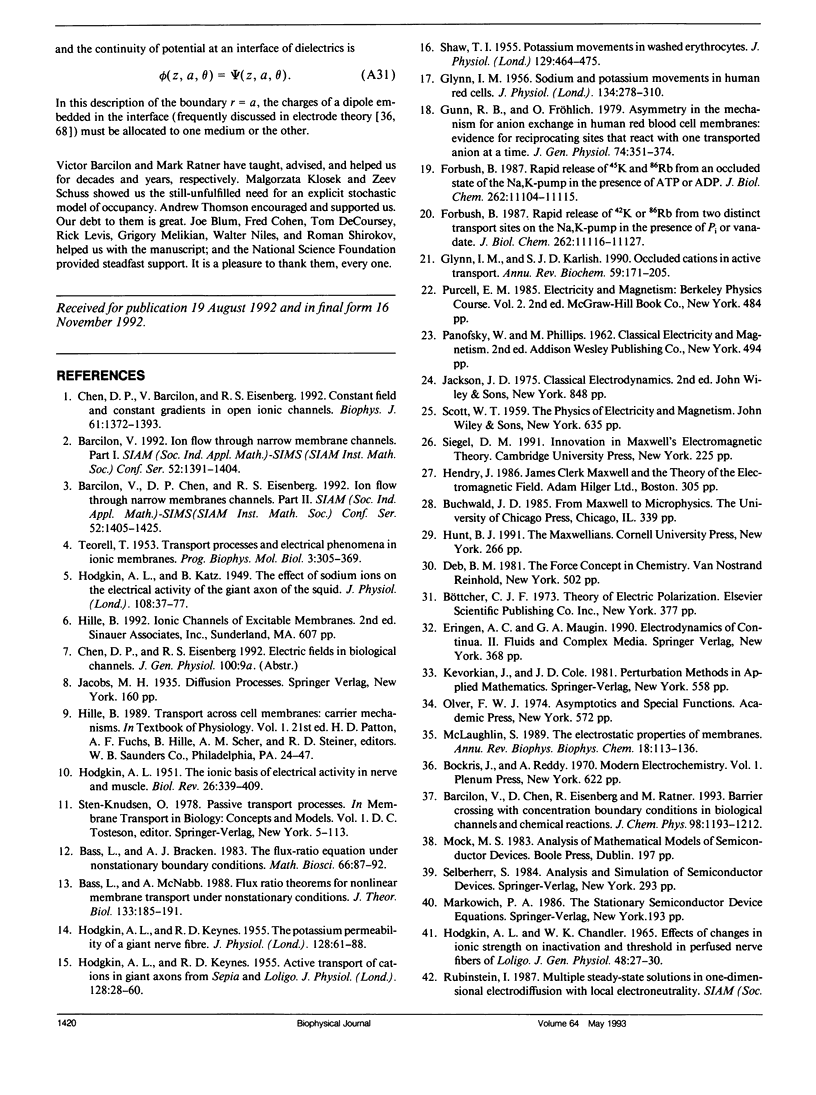
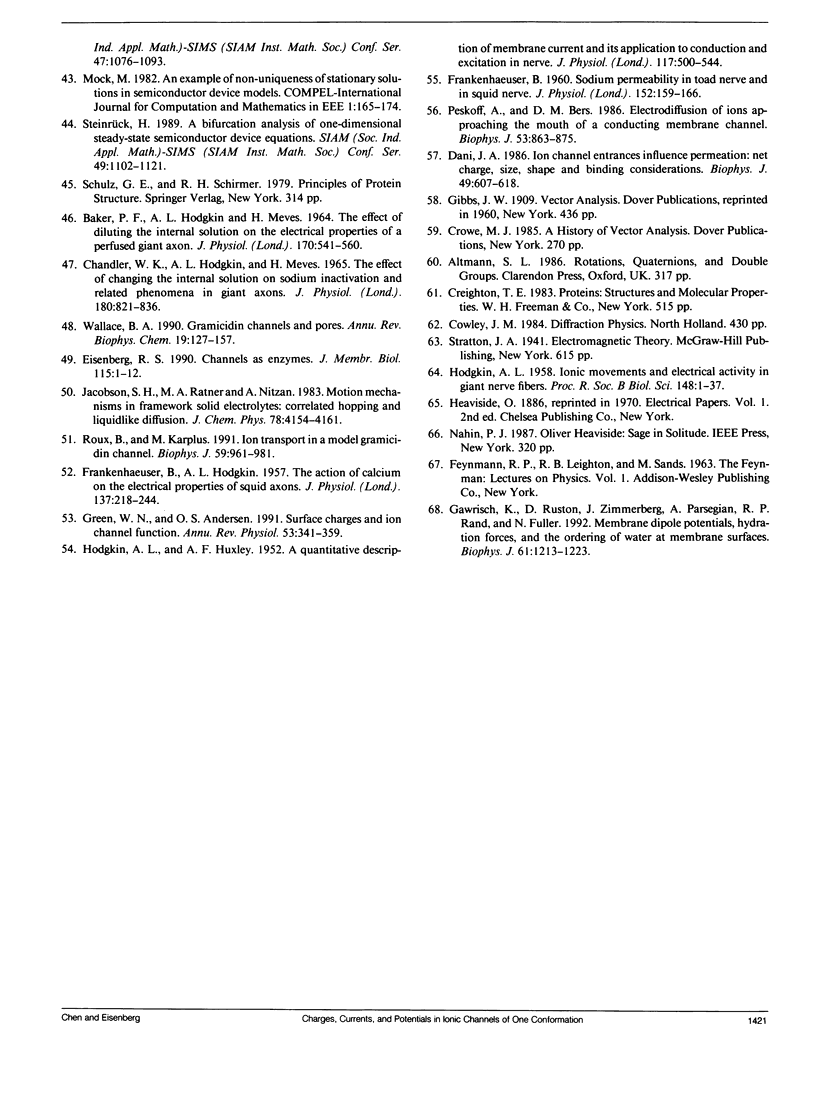
Selected References
These references are in PubMed. This may not be the complete list of references from this article.
- BAKER P. F., HODGKIN A. L., MEVES H. THE EFFECT OF DILUTING THE INTERNAL SOLUTION ON THE ELECTRICAL PROPERTIES OF A PERFUSED GIANT AXON. J Physiol. 1964 Apr;170:541–560. doi: 10.1113/jphysiol.1964.sp007348. [DOI] [PMC free article] [PubMed] [Google Scholar]
- Bass L., McNabb A. Flux ratio theorems for nonlinear membrane transport under nonstationary conditions. J Theor Biol. 1988 Jul 21;133(2):185–191. doi: 10.1016/s0022-5193(88)80004-3. [DOI] [PubMed] [Google Scholar]
- Chandler W. K., Hodgkin A. L., Meves H. The effect of changing the internal solution on sodium inactivation and related phenomena in giant axons. J Physiol. 1965 Oct;180(4):821–836. doi: 10.1113/jphysiol.1965.sp007733. [DOI] [PMC free article] [PubMed] [Google Scholar]
- Chen D. P., Barcilon V., Eisenberg R. S. Constant fields and constant gradients in open ionic channels. Biophys J. 1992 May;61(5):1372–1393. doi: 10.1016/S0006-3495(92)81944-6. [DOI] [PMC free article] [PubMed] [Google Scholar]
- Dani J. A. Ion-channel entrances influence permeation. Net charge, size, shape, and binding considerations. Biophys J. 1986 Mar;49(3):607–618. doi: 10.1016/S0006-3495(86)83688-8. [DOI] [PMC free article] [PubMed] [Google Scholar]
- Eisenberg R. S. Channels as enzymes. J Membr Biol. 1990 Apr;115(1):1–12. doi: 10.1007/BF01869101. [DOI] [PubMed] [Google Scholar]
- FRANKENHAEUSER B., HODGKIN A. L. The action of calcium on the electrical properties of squid axons. J Physiol. 1957 Jul 11;137(2):218–244. doi: 10.1113/jphysiol.1957.sp005808. [DOI] [PMC free article] [PubMed] [Google Scholar]
- FRANKENHAEUSER B. Sodium permeability in toad nerve and in squid nerve. J Physiol. 1960 Jun;152:159–166. doi: 10.1113/jphysiol.1960.sp006477. [DOI] [PMC free article] [PubMed] [Google Scholar]
- Forbush B., 3rd Rapid release of 42K and 86Rb from an occluded state of the Na,K-pump in the presence of ATP or ADP. J Biol Chem. 1987 Aug 15;262(23):11104–11115. [PubMed] [Google Scholar]
- Forbush B., 3rd Rapid release of 42K or 86Rb from two distinct transport sites on the Na,K-pump in the presence of Pi or vanadate. J Biol Chem. 1987 Aug 15;262(23):11116–11127. [PubMed] [Google Scholar]
- GLYNN I. M. Sodium and potassium movements in human red cells. J Physiol. 1956 Nov 28;134(2):278–310. doi: 10.1113/jphysiol.1956.sp005643. [DOI] [PMC free article] [PubMed] [Google Scholar]
- Gawrisch K., Ruston D., Zimmerberg J., Parsegian V. A., Rand R. P., Fuller N. Membrane dipole potentials, hydration forces, and the ordering of water at membrane surfaces. Biophys J. 1992 May;61(5):1213–1223. doi: 10.1016/S0006-3495(92)81931-8. [DOI] [PMC free article] [PubMed] [Google Scholar]
- Glynn I. M., Karlish S. J. Occluded cations in active transport. Annu Rev Biochem. 1990;59:171–205. doi: 10.1146/annurev.bi.59.070190.001131. [DOI] [PubMed] [Google Scholar]
- Green W. N., Andersen O. S. Surface charges and ion channel function. Annu Rev Physiol. 1991;53:341–359. doi: 10.1146/annurev.ph.53.030191.002013. [DOI] [PubMed] [Google Scholar]
- Gunn R. B., Fröhlich O. Asymmetry in the mechanism for anion exchange in human red blood cell membranes. Evidence for reciprocating sites that react with one transported anion at a time. J Gen Physiol. 1979 Sep;74(3):351–374. doi: 10.1085/jgp.74.3.351. [DOI] [PMC free article] [PubMed] [Google Scholar]
- HODGKIN A. L., CHANDLER W. K. EFFECTS OF CHANGES IN IONIC STRENGTH ON INACTIVATION AND THRESHOLD IN PERFUSED NERVE FIBERS OF LOLIGO. J Gen Physiol. 1965 May;48:SUPPL–SUPPL:30. doi: 10.1085/jgp.48.5.27. [DOI] [PMC free article] [PubMed] [Google Scholar]
- HODGKIN A. L., HUXLEY A. F. A quantitative description of membrane current and its application to conduction and excitation in nerve. J Physiol. 1952 Aug;117(4):500–544. doi: 10.1113/jphysiol.1952.sp004764. [DOI] [PMC free article] [PubMed] [Google Scholar]
- HODGKIN A. L. Ionic movements and electrical activity in giant nerve fibres. Proc R Soc Lond B Biol Sci. 1958 Jan 1;148(930):1–37. doi: 10.1098/rspb.1958.0001. [DOI] [PubMed] [Google Scholar]
- HODGKIN A. L., KATZ B. The effect of sodium ions on the electrical activity of giant axon of the squid. J Physiol. 1949 Mar 1;108(1):37–77. doi: 10.1113/jphysiol.1949.sp004310. [DOI] [PMC free article] [PubMed] [Google Scholar]
- HODGKIN A. L., KEYNES R. D. Active transport of cations in giant axons from Sepia and Loligo. J Physiol. 1955 Apr 28;128(1):28–60. doi: 10.1113/jphysiol.1955.sp005290. [DOI] [PMC free article] [PubMed] [Google Scholar]
- HODGKIN A. L., KEYNES R. D. The potassium permeability of a giant nerve fibre. J Physiol. 1955 Apr 28;128(1):61–88. doi: 10.1113/jphysiol.1955.sp005291. [DOI] [PMC free article] [PubMed] [Google Scholar]
- McLaughlin S. The electrostatic properties of membranes. Annu Rev Biophys Biophys Chem. 1989;18:113–136. doi: 10.1146/annurev.bb.18.060189.000553. [DOI] [PubMed] [Google Scholar]
- Peskoff A., Bers D. M. Electrodiffusion of ions approaching the mouth of a conducting membrane channel. Biophys J. 1988 Jun;53(6):863–875. doi: 10.1016/S0006-3495(88)83167-9. [DOI] [PMC free article] [PubMed] [Google Scholar]
- Roux B., Karplus M. Ion transport in a model gramicidin channel. Structure and thermodynamics. Biophys J. 1991 May;59(5):961–981. doi: 10.1016/S0006-3495(91)82311-6. [DOI] [PMC free article] [PubMed] [Google Scholar]
- SHAW T. I. Potassium movements in washed erythrocytes. J Physiol. 1955 Sep 28;129(3):464–475. doi: 10.1113/jphysiol.1955.sp005371. [DOI] [PMC free article] [PubMed] [Google Scholar]
- Wallace B. A. Gramicidin channels and pores. Annu Rev Biophys Biophys Chem. 1990;19:127–157. doi: 10.1146/annurev.bb.19.060190.001015. [DOI] [PubMed] [Google Scholar]