Abstract
At issue is the type of constitutive equation that can be used to describe all possible types of deformation of the neutrophil. Here a neutrophil undergoing small deformations is studied by aspirating it into a glass pipet with a diameter that is only slightly smaller than the diameter of the spherically shaped cell. After being held in the pipet for at least seven seconds, the cell is rapidly expelled and allowed to recover its undeformed, spherical shape. The recovery takes approximately 15 s. An analysis of the recovery process that treats the cell as a simple Newtonian liquid drop with a constant cortical (surface) tension gives a value of 3.3 x 10(-5) cm/s for the ratio of the cortical tension to cytoplasmic viscosity. This value is about twice as large as a previously published value obtained with the same model from studies of large deformations of neutrophils. This discrepancy indicates that the cytoplasmic viscosity decreases as the amount of deformation decreases. An extrapolated value for the cytoplasmic viscosity at zero deformation is approximately 600 poise when a value for the cortical tension of 0.024 dyn/cm is assumed. Clearly the neutrophil does not behave like a simple Newtonian liquid drop in that small deformations are inherently different from large deformations. More complex models consisting either of two or more fluids or multiple shells must be developed. The complex structure inside the neutrophil is shown in scanning electron micrographs of osmotically burst cells and cells whose membrane has been dissolved away.
Full text
PDF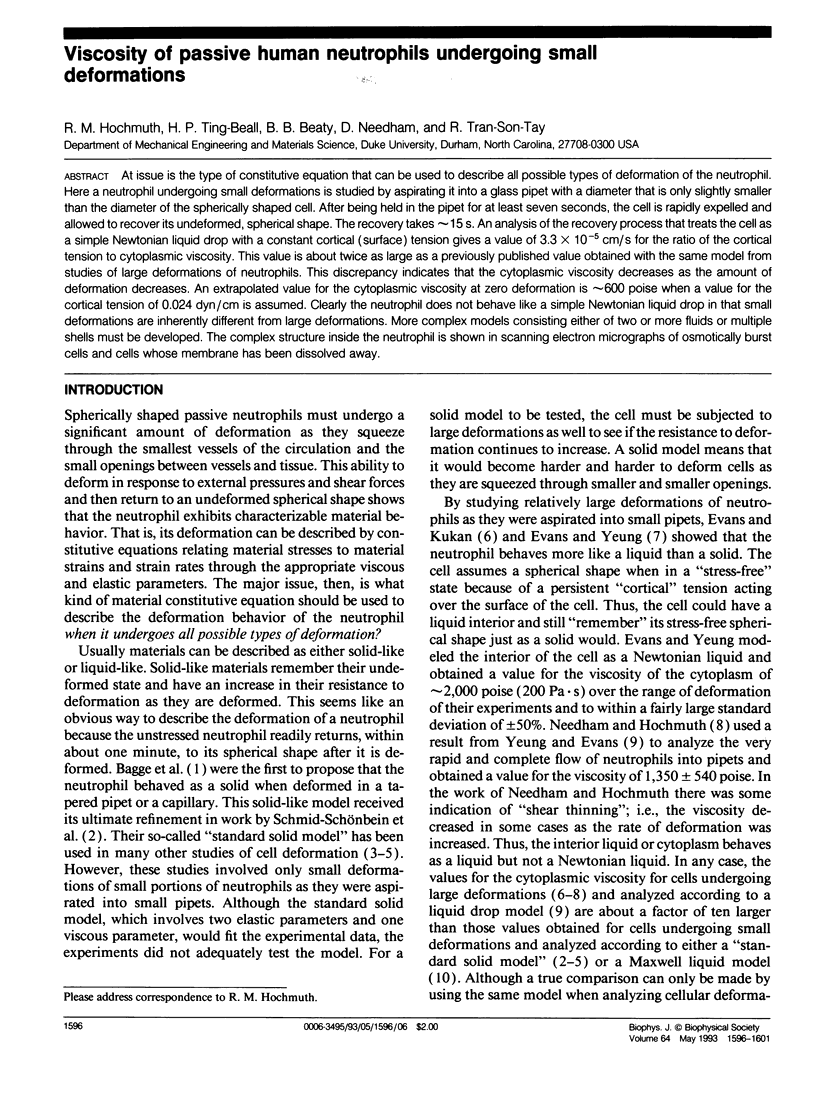
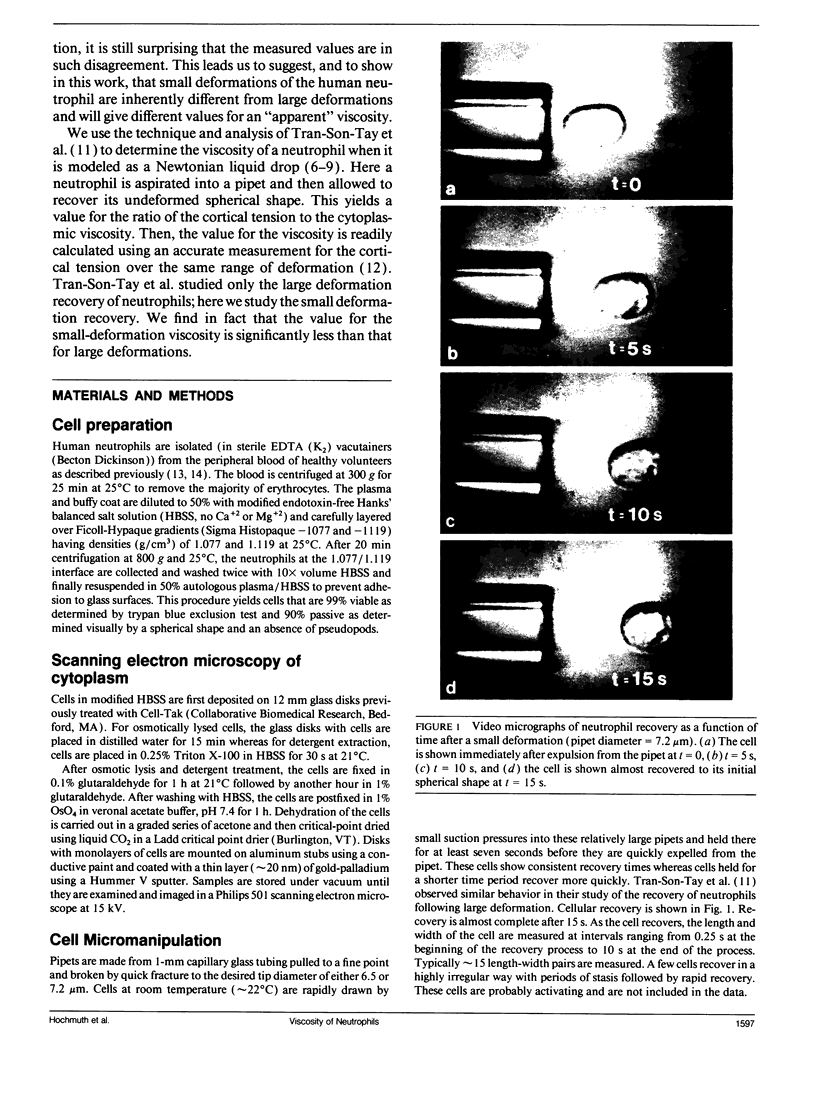
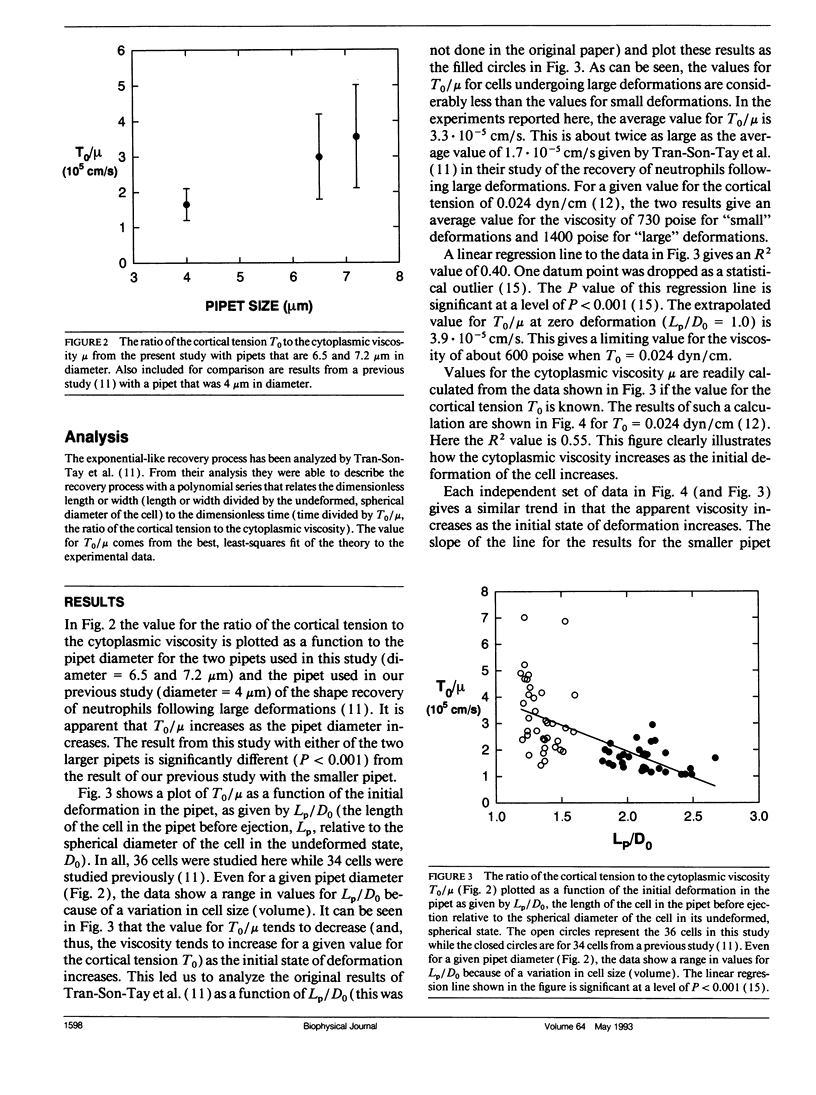
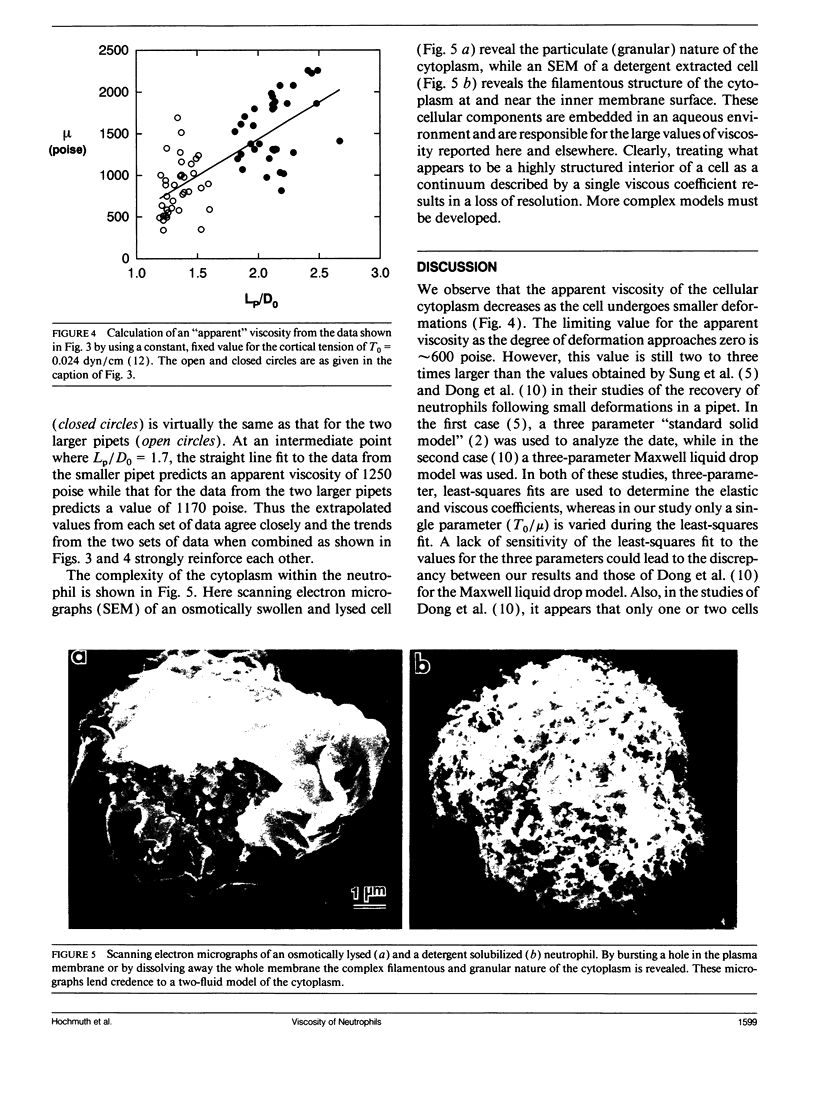
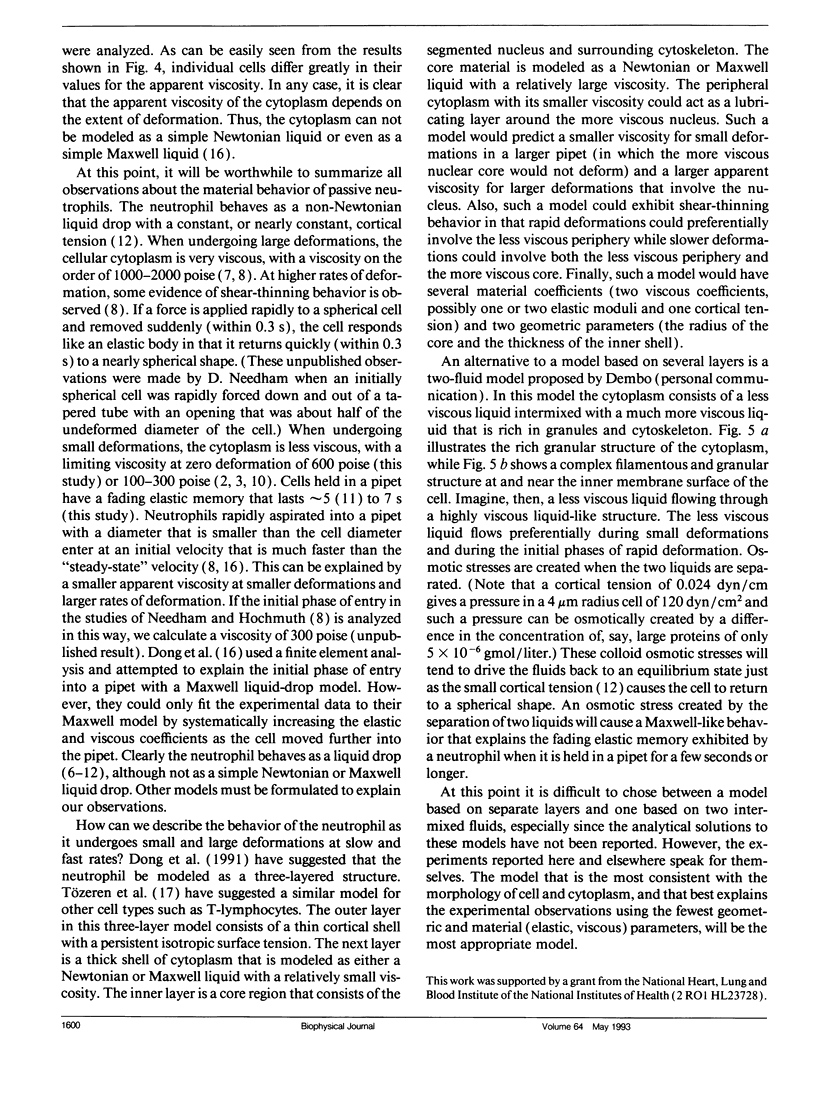

Images in this article
Selected References
These references are in PubMed. This may not be the complete list of references from this article.
- Armstrong M., Jr, Needham D., Hatchell D. L., Nunn R. S. Effect of pentoxifylline on the flow of polymorphonuclear leukocytes through a model capillary. Angiology. 1990 Apr;41(4):253–262. doi: 10.1177/000331979004100401. [DOI] [PubMed] [Google Scholar]
- Chien S., Sung K. L. Effect of colchicine on viscoelastic properties of neutrophils. Biophys J. 1984 Sep;46(3):383–386. doi: 10.1016/S0006-3495(84)84034-5. [DOI] [PMC free article] [PubMed] [Google Scholar]
- Dong C., Skalak R., Sung K. L. Cytoplasmic rheology of passive neutrophils. Biorheology. 1991;28(6):557–567. doi: 10.3233/bir-1991-28607. [DOI] [PubMed] [Google Scholar]
- Dong C., Skalak R., Sung K. L., Schmid-Schönbein G. W., Chien S. Passive deformation analysis of human leukocytes. J Biomech Eng. 1988 Feb;110(1):27–36. doi: 10.1115/1.3108402. [DOI] [PubMed] [Google Scholar]
- English D., Andersen B. R. Single-step separation of red blood cells. Granulocytes and mononuclear leukocytes on discontinuous density gradients of Ficoll-Hypaque. J Immunol Methods. 1974 Aug;5(3):249–252. doi: 10.1016/0022-1759(74)90109-4. [DOI] [PubMed] [Google Scholar]
- Evans E., Kukan B. Passive material behavior of granulocytes based on large deformation and recovery after deformation tests. Blood. 1984 Nov;64(5):1028–1035. [PubMed] [Google Scholar]
- Evans E., Yeung A. Apparent viscosity and cortical tension of blood granulocytes determined by micropipet aspiration. Biophys J. 1989 Jul;56(1):151–160. doi: 10.1016/S0006-3495(89)82660-8. [DOI] [PMC free article] [PubMed] [Google Scholar]
- Needham D., Hochmuth R. M. A sensitive measure of surface stress in the resting neutrophil. Biophys J. 1992 Jun;61(6):1664–1670. doi: 10.1016/S0006-3495(92)81970-7. [DOI] [PMC free article] [PubMed] [Google Scholar]
- Needham D., Hochmuth R. M. Rapid flow of passive neutrophils into a 4 microns pipet and measurement of cytoplasmic viscosity. J Biomech Eng. 1990 Aug;112(3):269–276. doi: 10.1115/1.2891184. [DOI] [PubMed] [Google Scholar]
- Schmid-Schönbein G. W., Sung K. L., Tözeren H., Skalak R., Chien S. Passive mechanical properties of human leukocytes. Biophys J. 1981 Oct;36(1):243–256. doi: 10.1016/S0006-3495(81)84726-1. [DOI] [PMC free article] [PubMed] [Google Scholar]
- Sung K. L., Dong C., Schmid-Schönbein G. W., Chien S., Skalak R. Leukocyte relaxation properties. Biophys J. 1988 Aug;54(2):331–336. doi: 10.1016/S0006-3495(88)82963-1. [DOI] [PMC free article] [PubMed] [Google Scholar]
- Sung K. L., Schmid-Schönbein G. W., Skalak R., Schuessler G. B., Usami S., Chien S. Influence of physicochemical factors on rheology of human neutrophils. Biophys J. 1982 Jul;39(1):101–106. doi: 10.1016/S0006-3495(82)84495-0. [DOI] [PMC free article] [PubMed] [Google Scholar]
- Tran-Son-Tay R., Needham D., Yeung A., Hochmuth R. M. Time-dependent recovery of passive neutrophils after large deformation. Biophys J. 1991 Oct;60(4):856–866. doi: 10.1016/S0006-3495(91)82119-1. [DOI] [PMC free article] [PubMed] [Google Scholar]
- Tözeren A., Mackie L. H., Lawrence M. B., Chan P. Y., Dustin M. L., Springer T. A. Micromanipulation of adhesion of phorbol 12-myristate-13-acetate-stimulated T lymphocytes to planar membranes containing intercellular adhesion molecule-1. Biophys J. 1992 Jul;63(1):247–258. doi: 10.1016/S0006-3495(92)81578-3. [DOI] [PMC free article] [PubMed] [Google Scholar]
- Yeung A., Evans E. Cortical shell-liquid core model for passive flow of liquid-like spherical cells into micropipets. Biophys J. 1989 Jul;56(1):139–149. doi: 10.1016/S0006-3495(89)82659-1. [DOI] [PMC free article] [PubMed] [Google Scholar]