Abstract
1. The length dependence of the mechanical latent period and early tension development, uncomplicated by latency relaxation, was determined from responses of single muscle fibres to restimulation at the peak of the isometric twitch at 15 degrees C. 2. The onset of the reactivation response showed little (less than 1.5 X 10(-7) N) or no latency relaxation. 3. Reactivation latency was minimal (2.8 msec) and constant at 1.9-2.1 micrometers sarcomere length and it increased by about 3 msec with sarcomere extension to 3.2 micrometers. 4. Reactivation responses showed two stages of early tension development, an initial phase in which tension acceleration increased, and a phase of maximum responsiveness in which tension acceleration was constant; the transition between the two phases occurred about 4.5 msec after the start of the stimulus at 2.2 micrometers sarcomere length and was delayed about 4 msec with increase in sarcomere length to 3.2 micrometers. 5. The square root of the maximum tension acceleration was directly proportional to the degree of overlap of thick and thin filaments in the sarcomere length range 2.3-3.2 micrometers. 6. It is proposed that the onset of the phase of constant tension acceleration marks the end of the period during which the activator, calcium, is distributed throughout the sarcomere. 7. Analysis of early tension transients in relation to myofibril structure showed that length-dependent changes in reactivation latency and time of onset of constant tension acceleration were probably brought about mainly by alteration of the kinetics of distribution of activator within the myofibril and by changes in the diffusion distance between activator-release sites ner the end of the sarcomere and the tension-generating sites. 8. There was a 2 msec myofibril priming delay in the rise of tension in twitch responses that was not seen in reactivation responses; the possible origin of that delay is discussed in relation to structural changes accompanying activation and to competition between calcium-binding structures. 9. The onset of twitch latency relaxation occurred within about 250 microseconds after the time corresponding to latency of the earliest reactivation responses and appeared to signal the start of a process that took place after the arrival of calcium among the myofilaments. The origin of latency relaxation is discussed.
Full text
PDF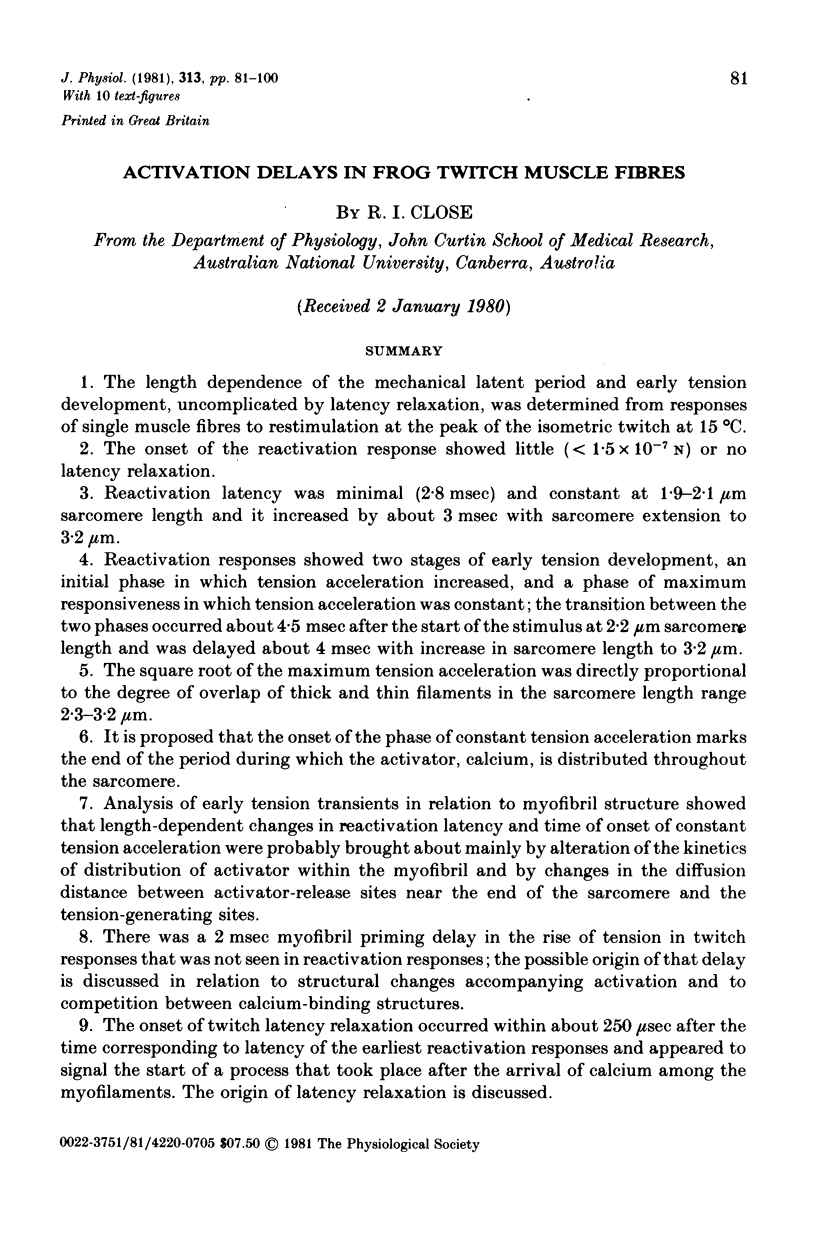
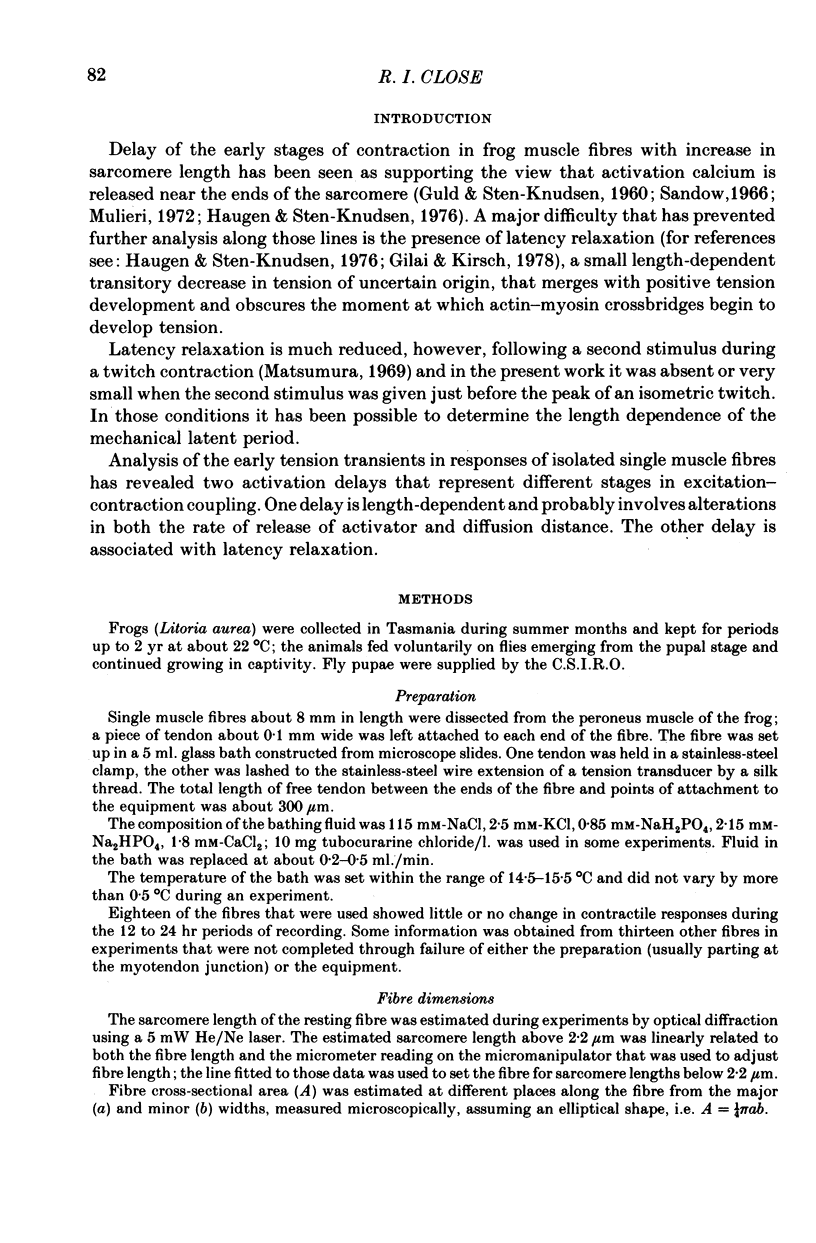
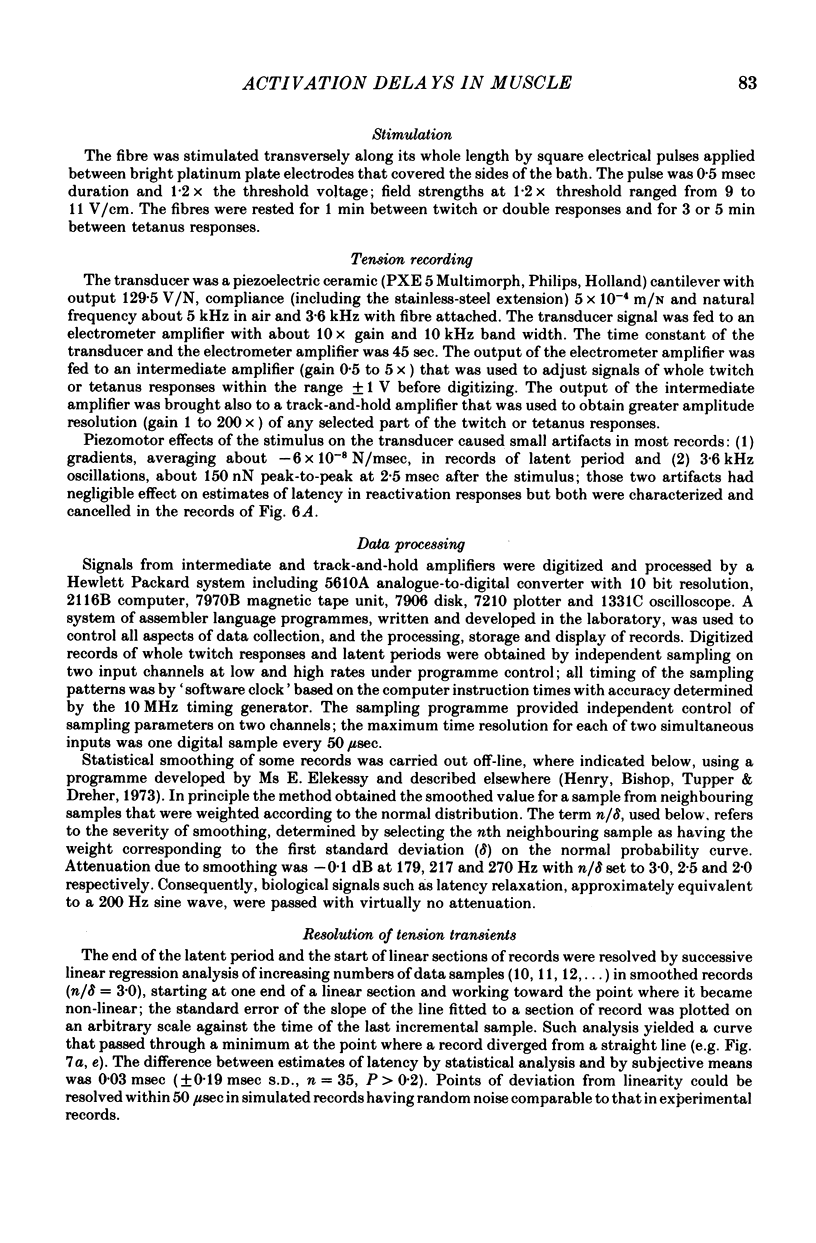
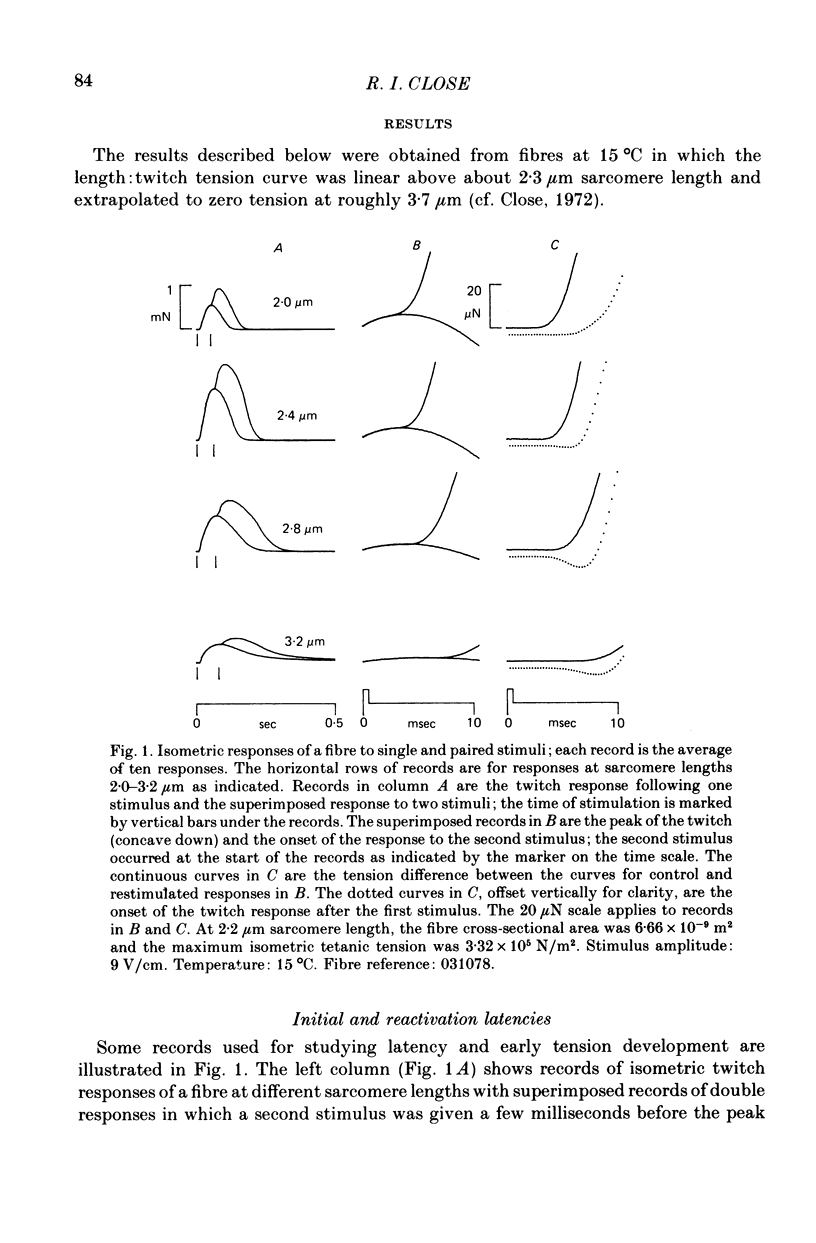
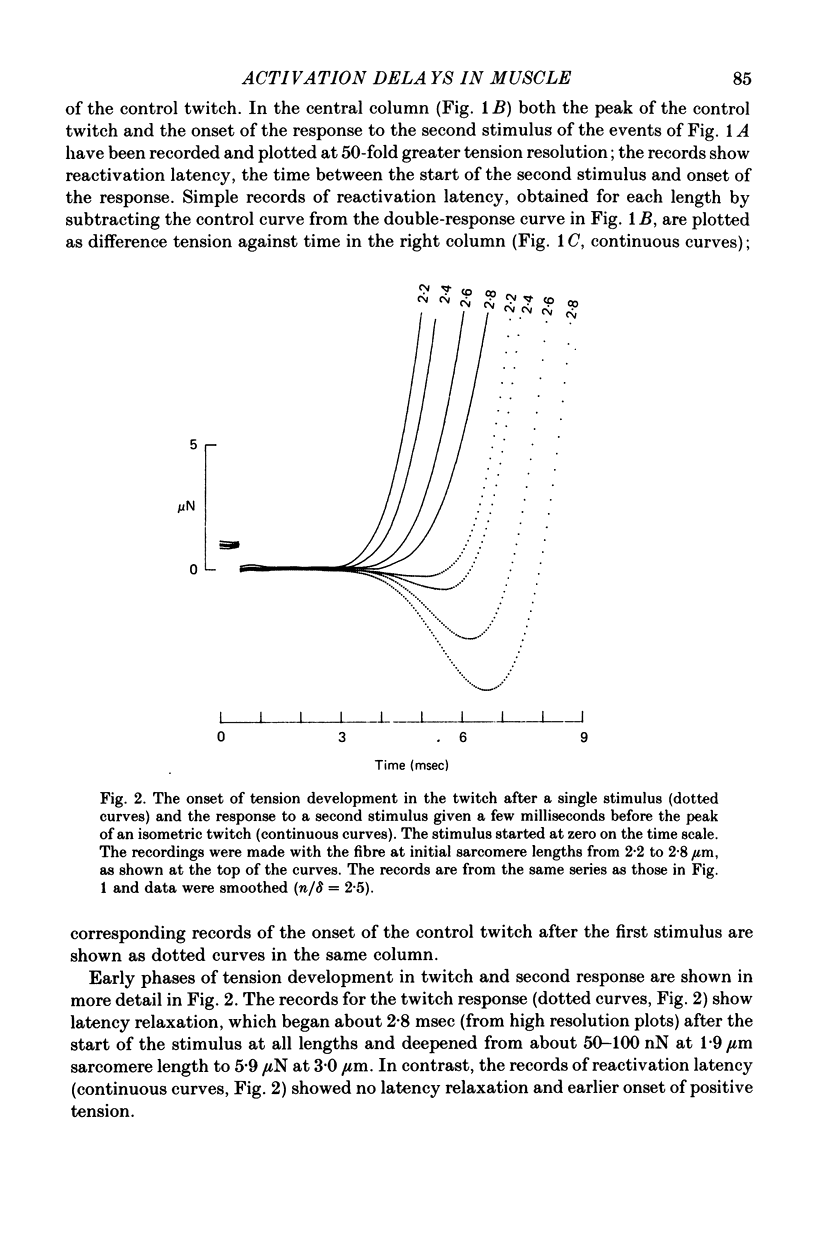
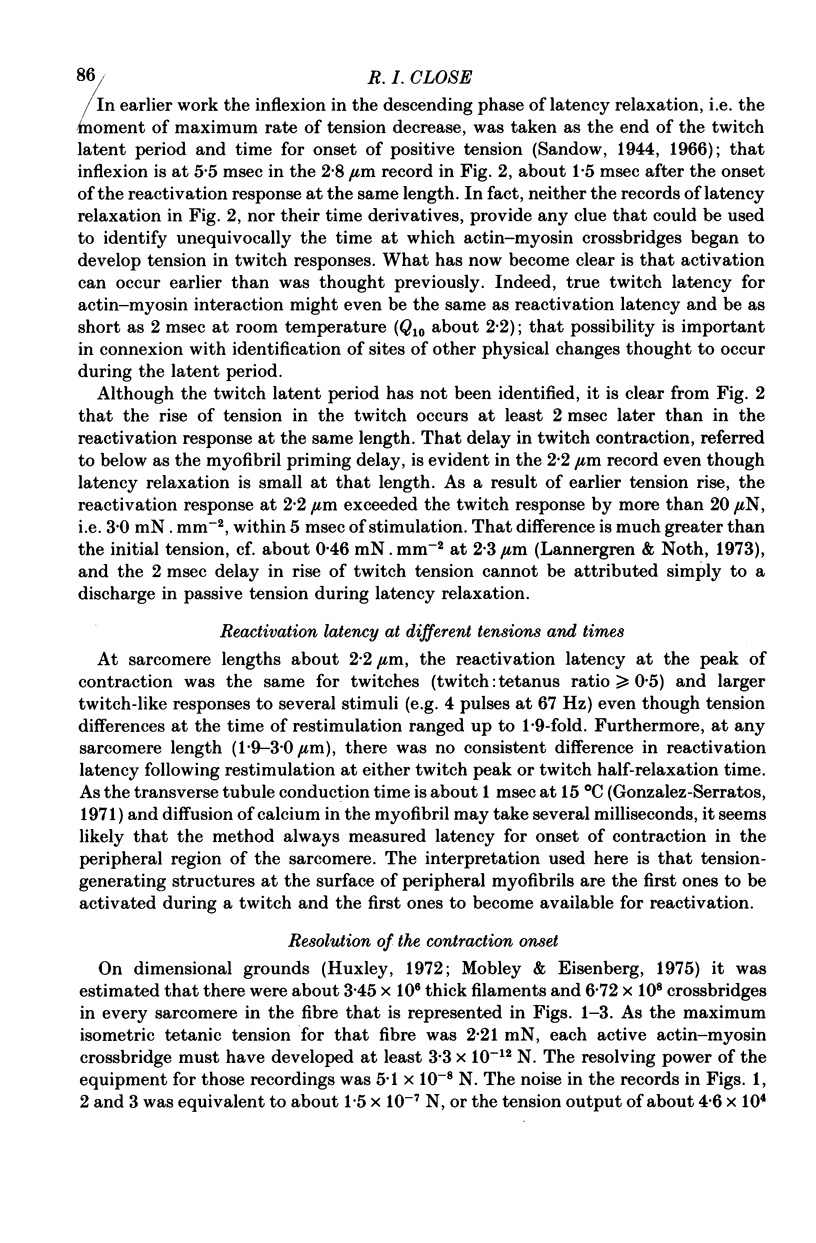
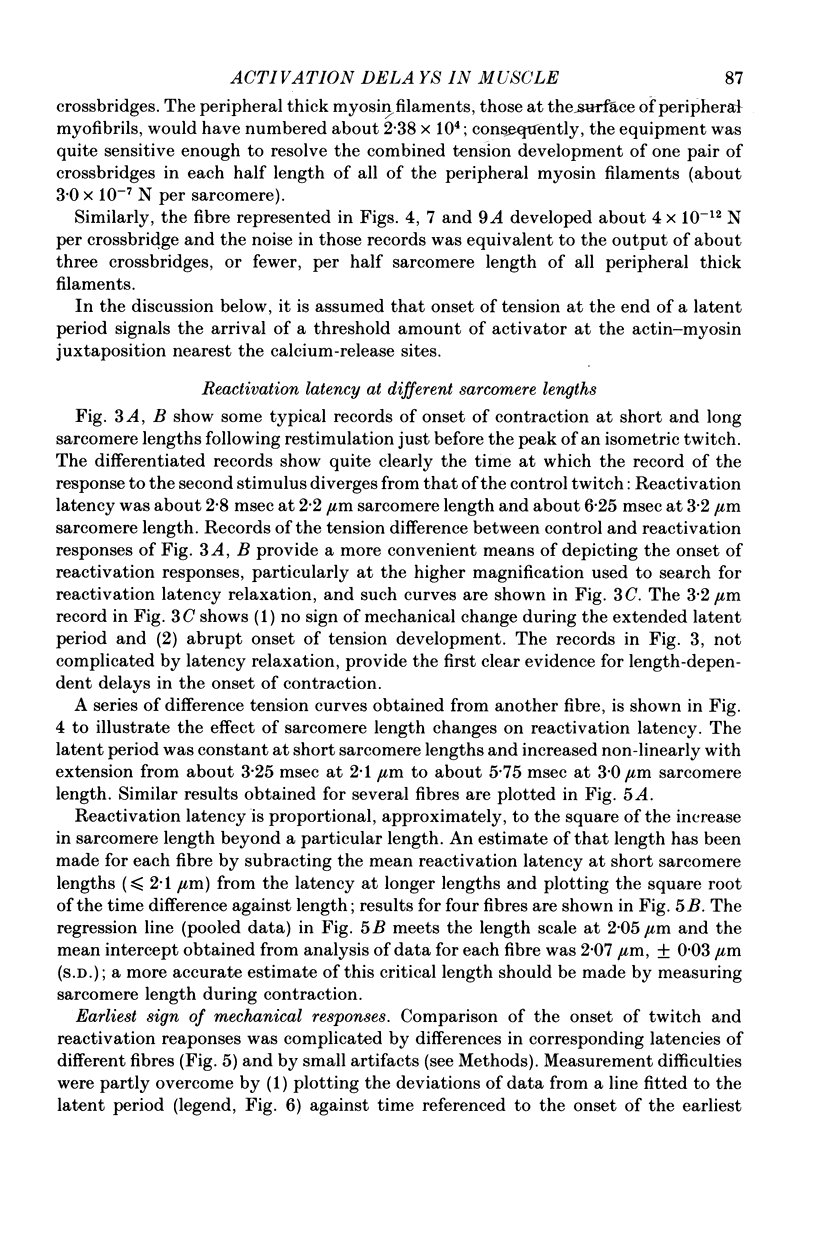
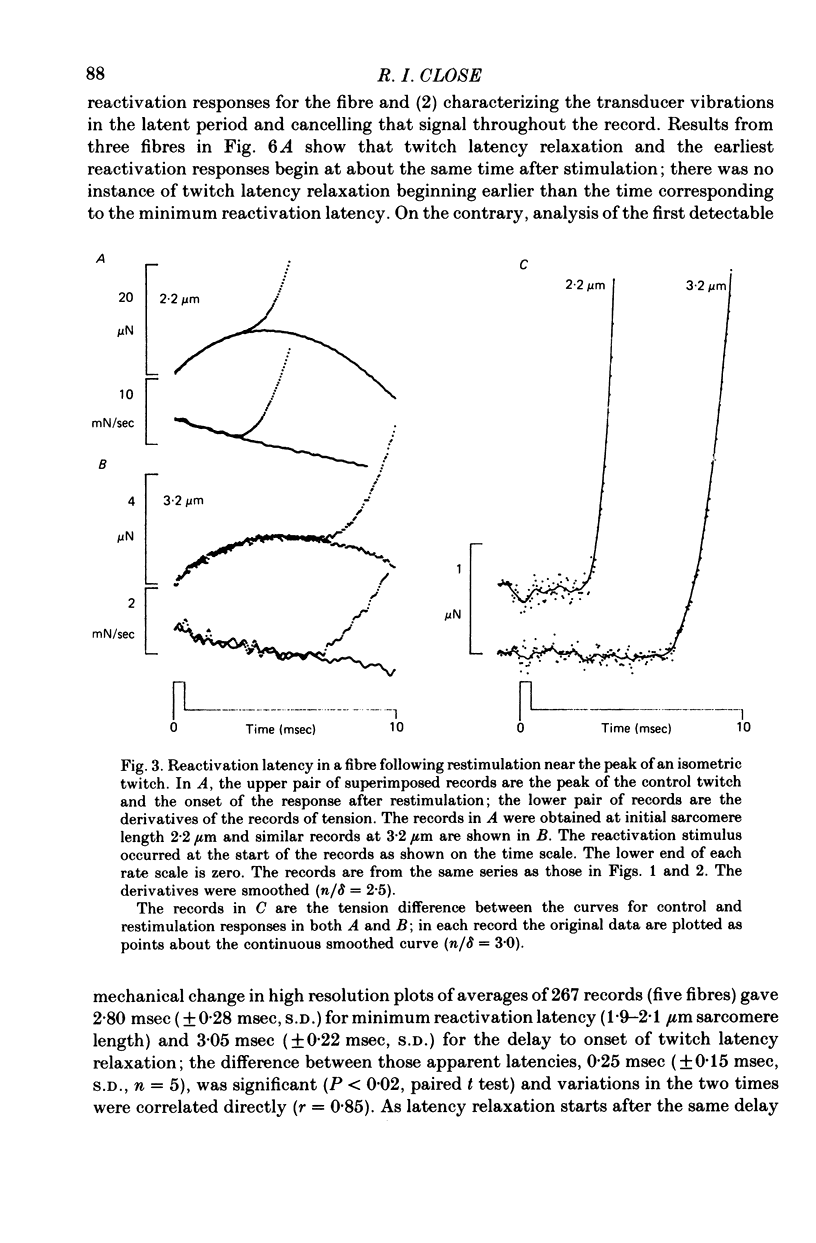
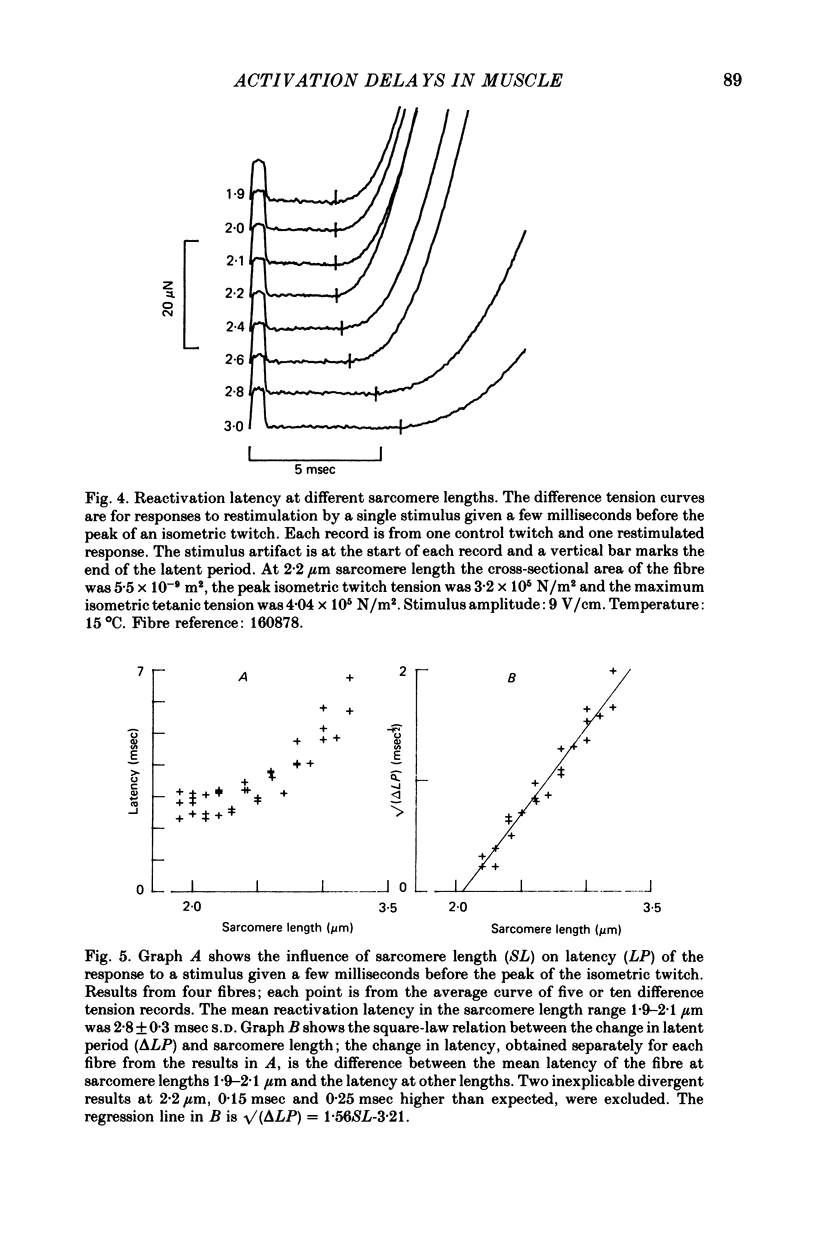
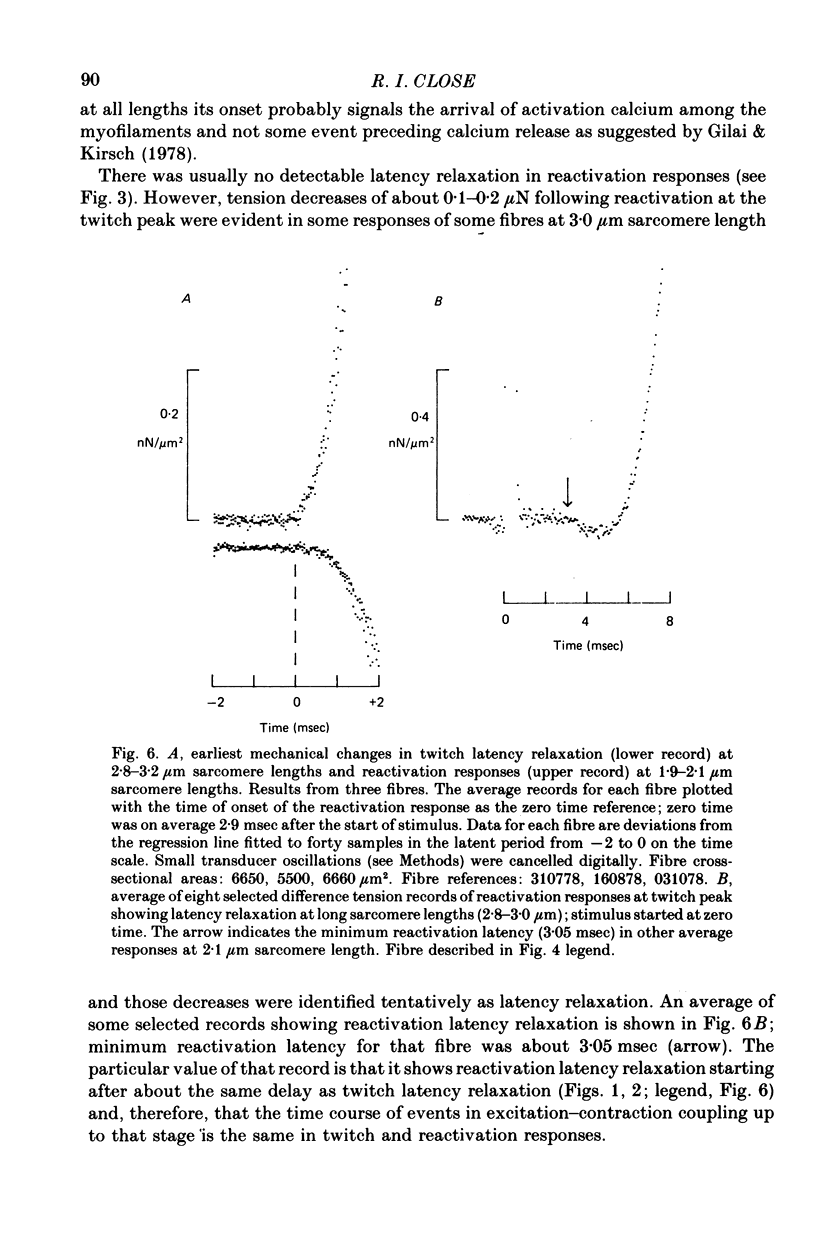
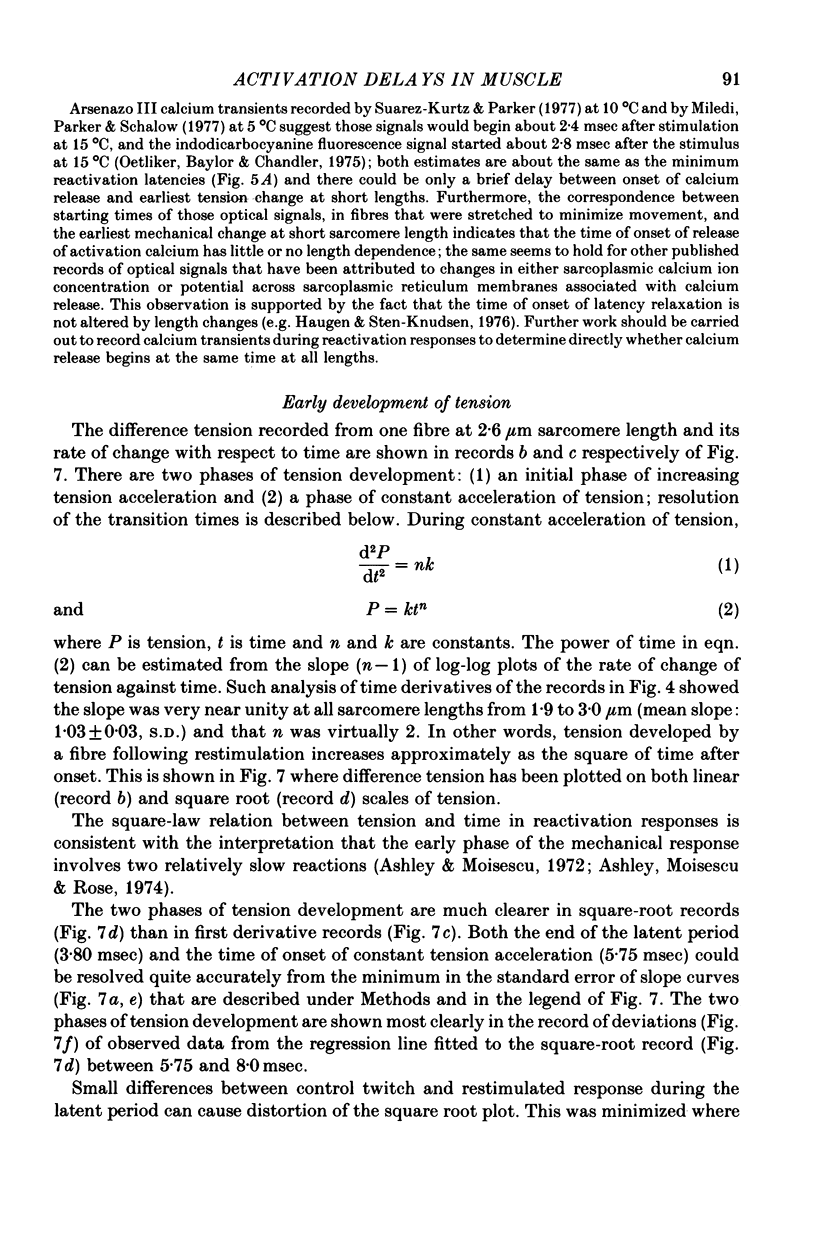
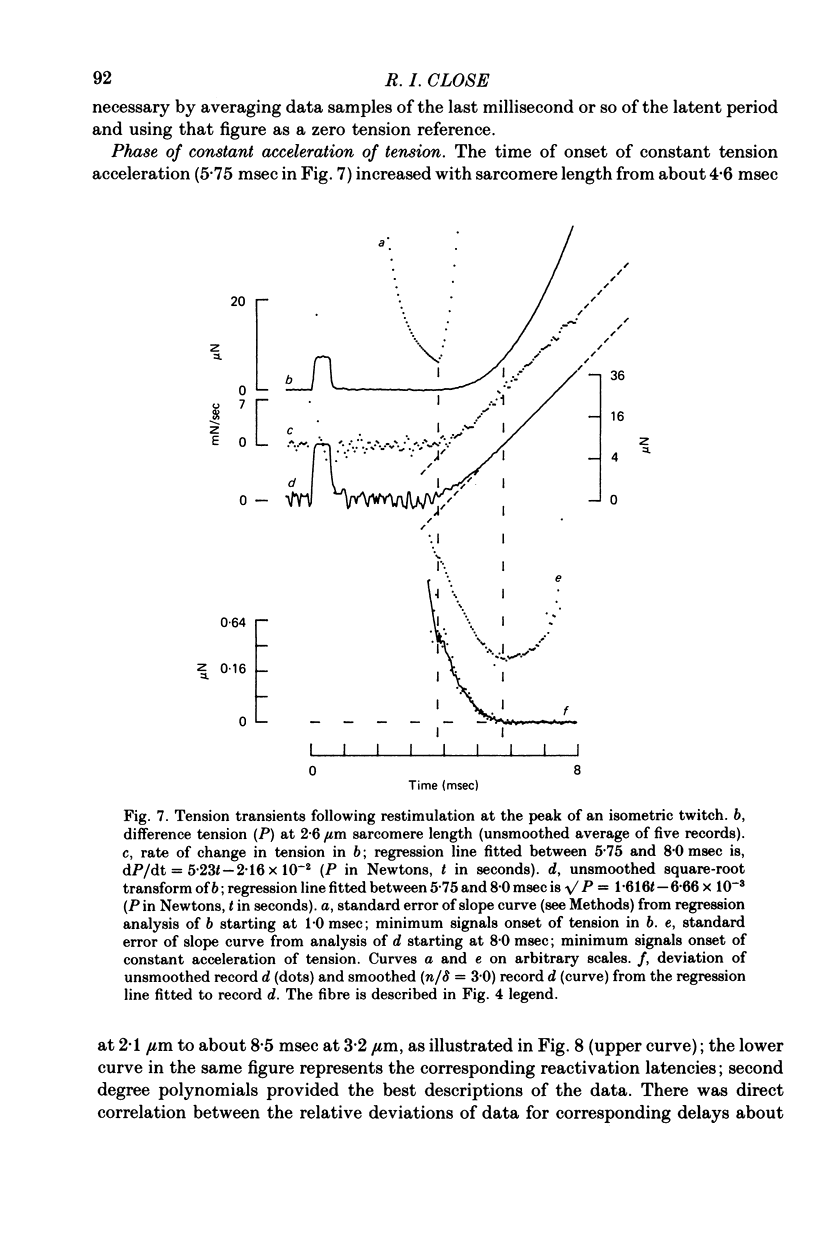
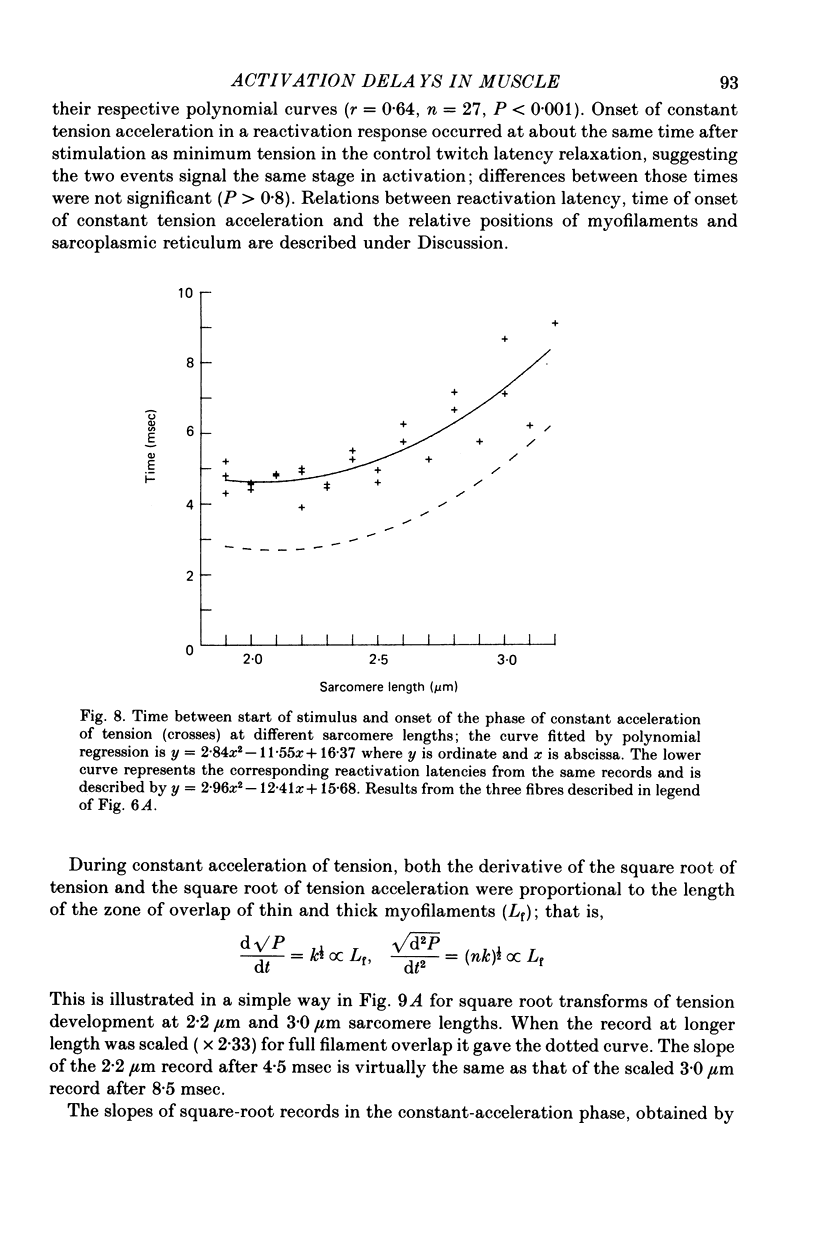
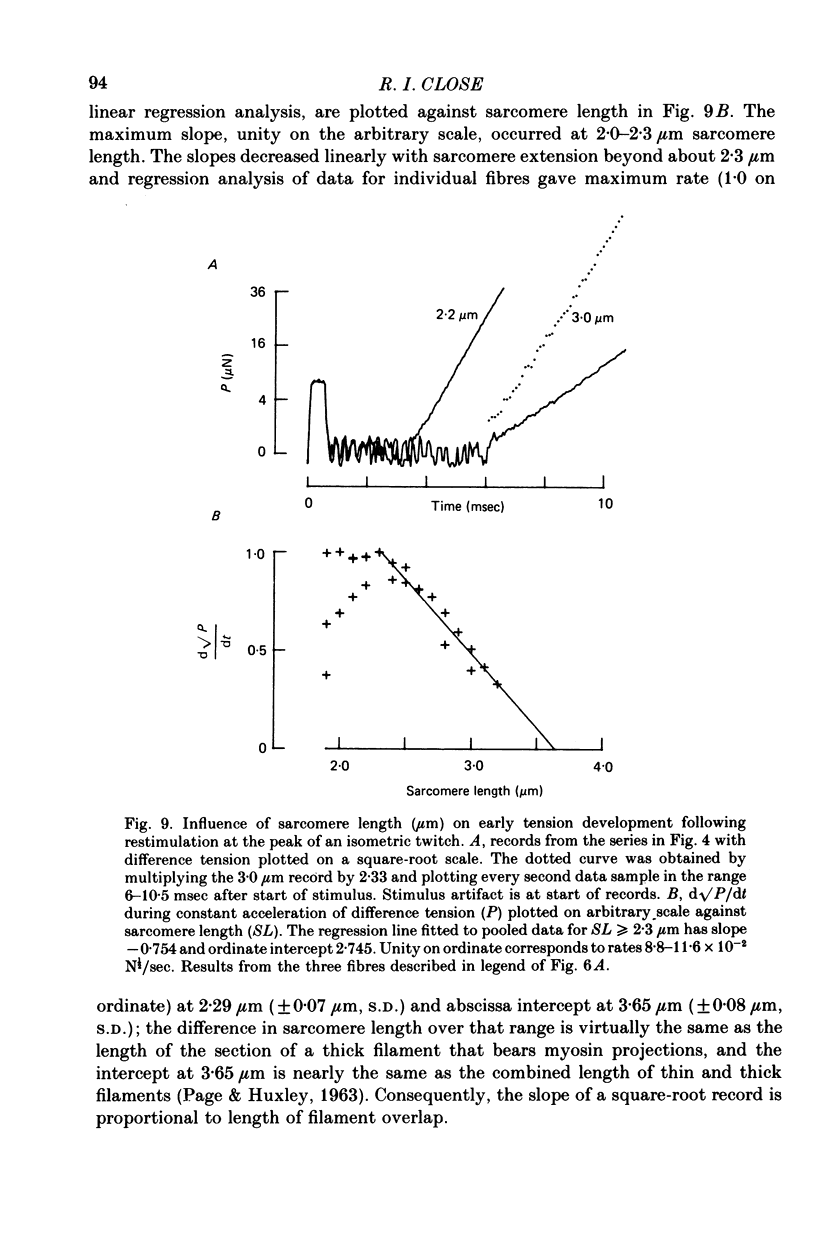
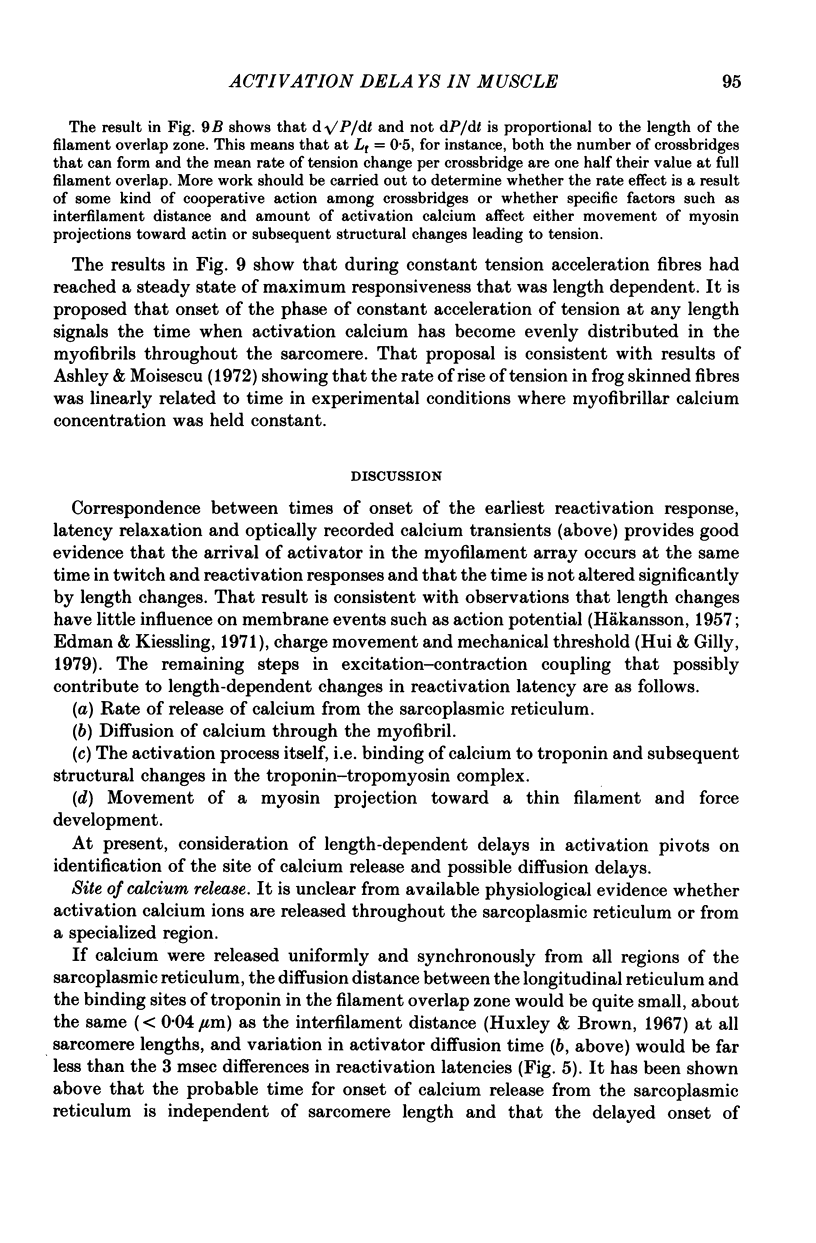
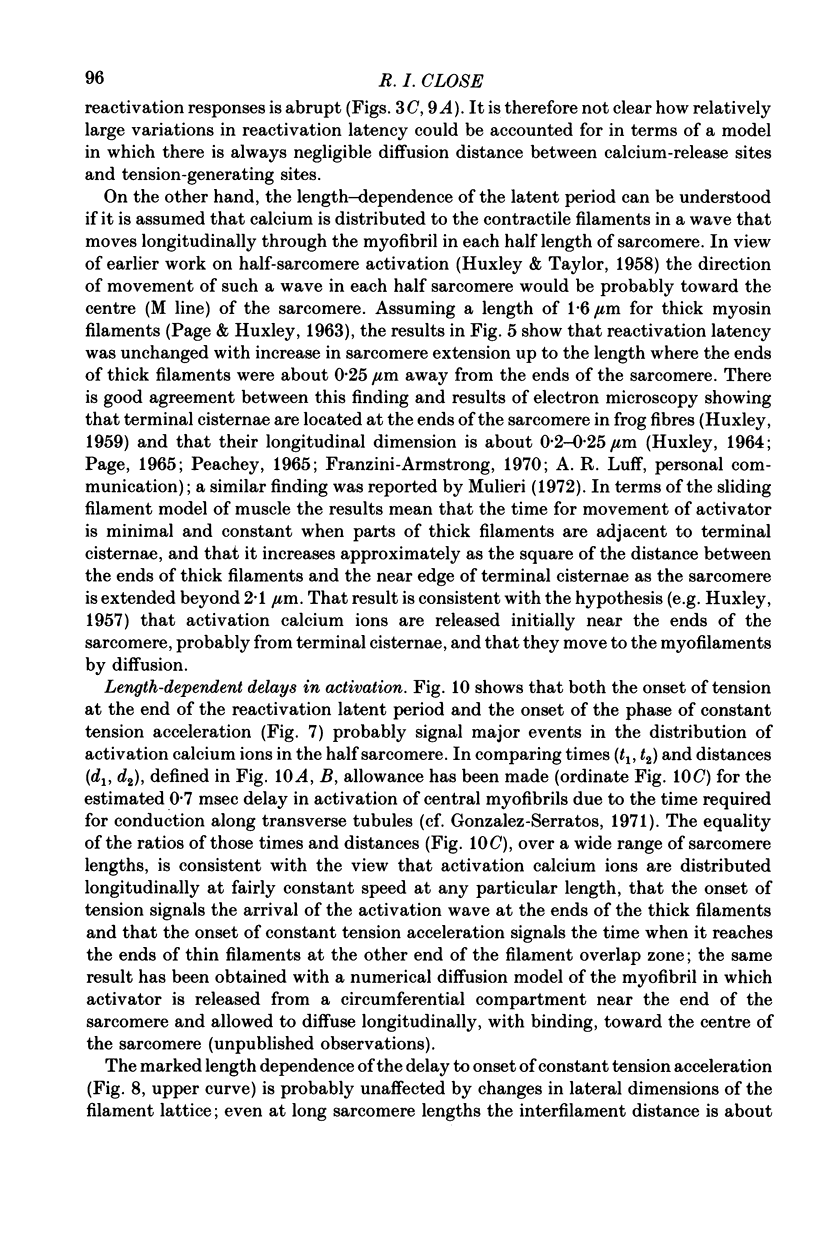
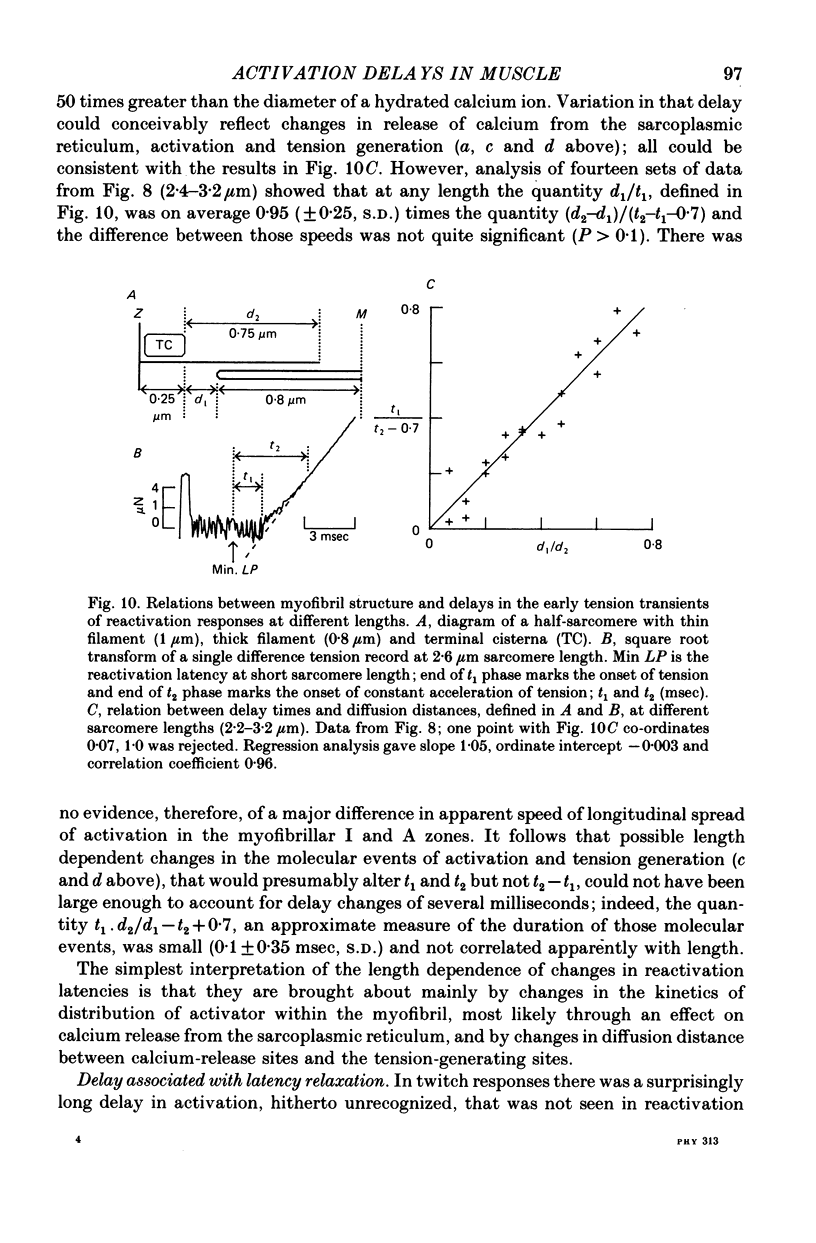
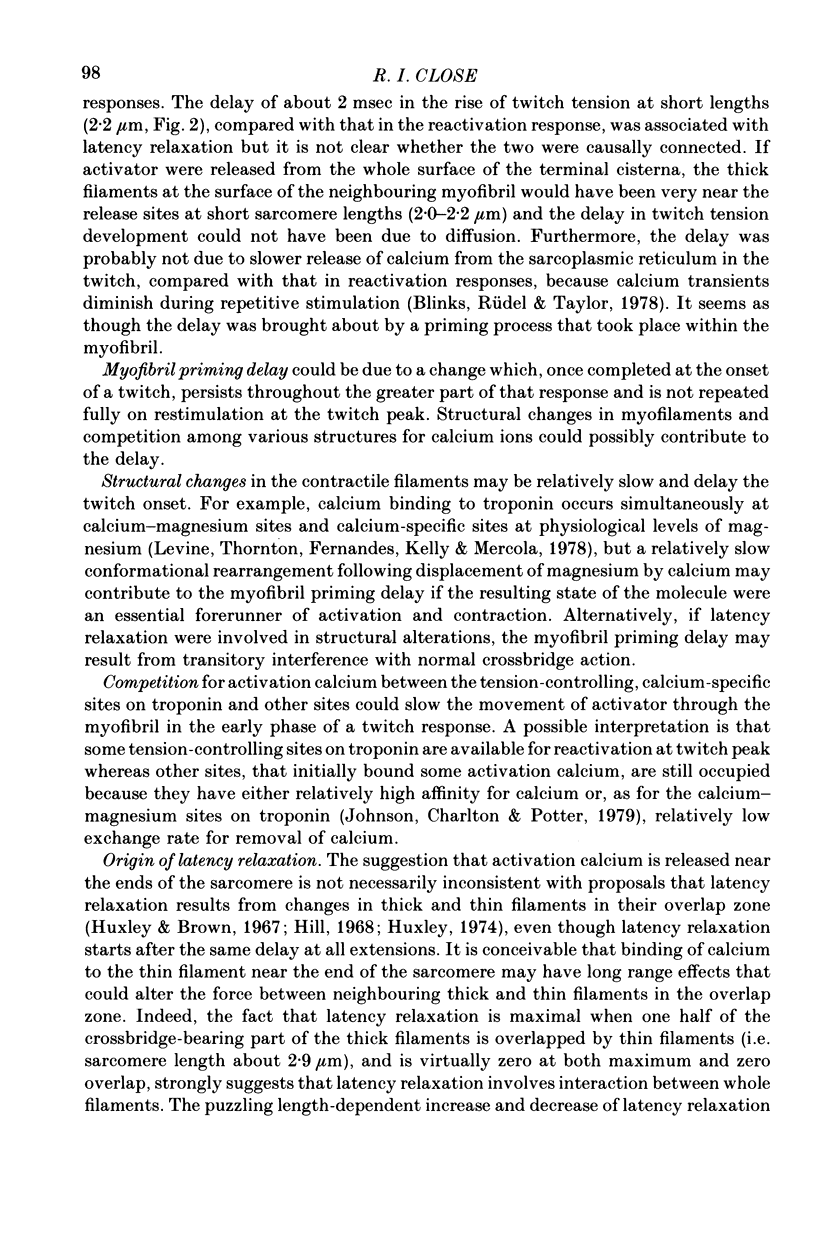
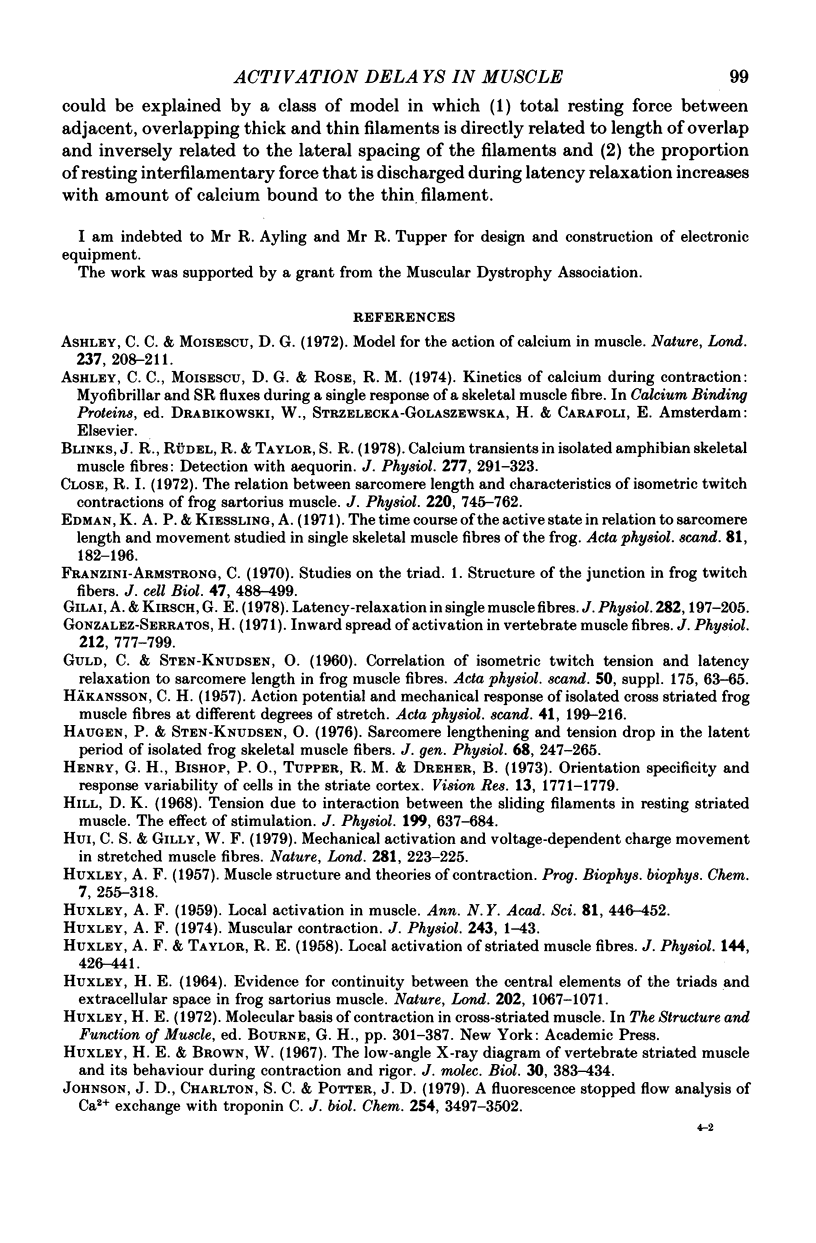
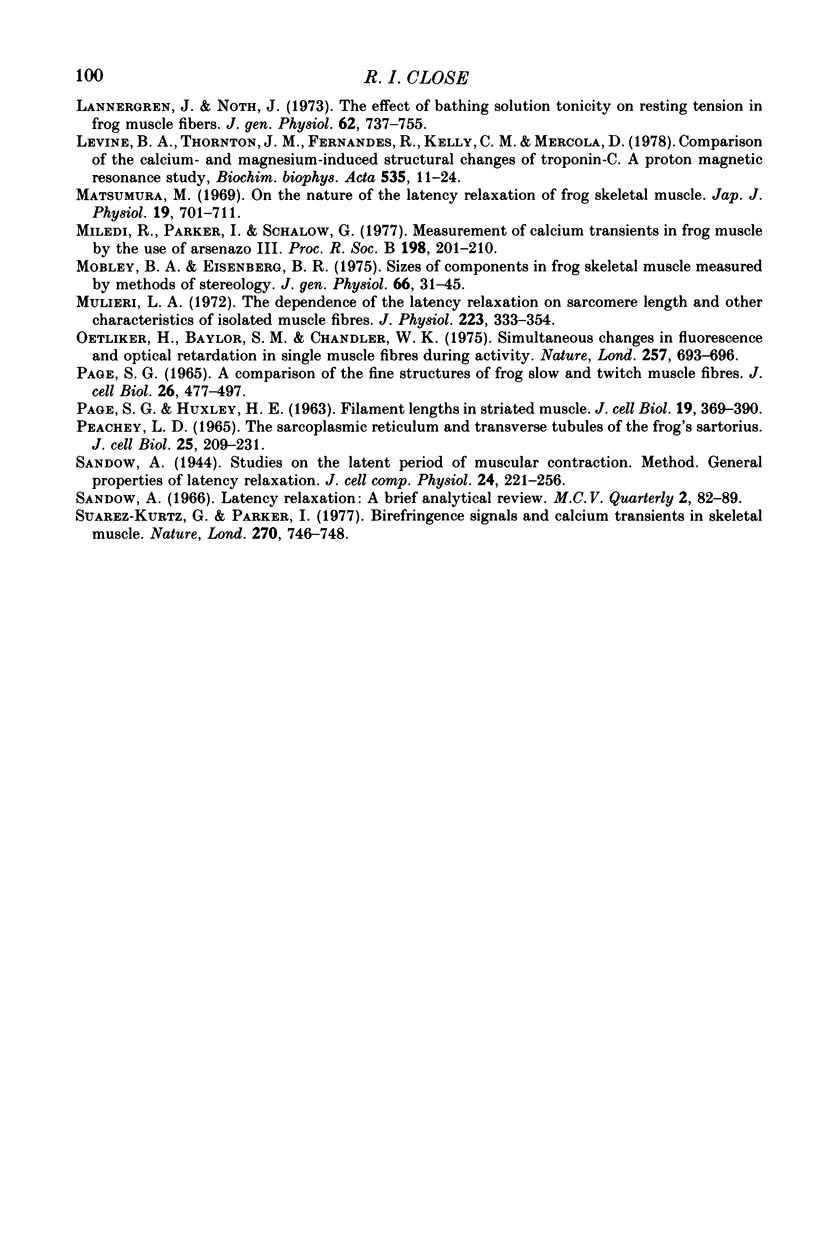
Selected References
These references are in PubMed. This may not be the complete list of references from this article.
- Ashley C. C., Moisescu D. G. Model for the action of calcium in muscle. Nat New Biol. 1972 Jun 14;237(76):208–211. doi: 10.1038/newbio237208a0. [DOI] [PubMed] [Google Scholar]
- Blinks J. R., Rüdel R., Taylor S. R. Calcium transients in isolated amphibian skeletal muscle fibres: detection with aequorin. J Physiol. 1978 Apr;277:291–323. doi: 10.1113/jphysiol.1978.sp012273. [DOI] [PMC free article] [PubMed] [Google Scholar]
- Close R. I. The relations between sarcomere length and characteristics of isometric twitch contractions of frog sartorius muscle. J Physiol. 1972 Feb;220(3):745–762. doi: 10.1113/jphysiol.1972.sp009733. [DOI] [PMC free article] [PubMed] [Google Scholar]
- Edman K. A., Kiessling A. The time course of the active state in relation to sarcomere length and movement studied in single skeletal muscle fibres of the frog. Acta Physiol Scand. 1971 Feb;81(2):182–196. doi: 10.1111/j.1748-1716.1971.tb04891.x. [DOI] [PubMed] [Google Scholar]
- Franzini-Armstrong C. STUDIES OF THE TRIAD : I. Structure of the Junction in Frog Twitch Fibers. J Cell Biol. 1970 Nov 1;47(2):488–499. doi: 10.1083/jcb.47.2.488. [DOI] [PMC free article] [PubMed] [Google Scholar]
- Gilai A., Kirsch G. E. Latency-relaxation in single muscle fibres. J Physiol. 1978 Sep;282:197–205. doi: 10.1113/jphysiol.1978.sp012457. [DOI] [PMC free article] [PubMed] [Google Scholar]
- González-Serratos H. Inward spread of activation in vertebrate muscle fibres. J Physiol. 1971 Feb;212(3):777–799. doi: 10.1113/jphysiol.1971.sp009356. [DOI] [PMC free article] [PubMed] [Google Scholar]
- HUXLEY A. F. Local activation of muscle. Ann N Y Acad Sci. 1959 Aug 28;81:446–452. doi: 10.1111/j.1749-6632.1959.tb49326.x. [DOI] [PubMed] [Google Scholar]
- HUXLEY A. F. Muscle structure and theories of contraction. Prog Biophys Biophys Chem. 1957;7:255–318. [PubMed] [Google Scholar]
- HUXLEY A. F., TAYLOR R. E. Local activation of striated muscle fibres. J Physiol. 1958 Dec 30;144(3):426–441. doi: 10.1113/jphysiol.1958.sp006111. [DOI] [PMC free article] [PubMed] [Google Scholar]
- HUXLEY H. E. EVIDENCE FOR CONTINUITY BETWEEN THE CENTRAL ELEMENTS OF THE TRIADS AND EXTRACELLULAR SPACE IN FROG SARTORIUS MUSCLE. Nature. 1964 Jun 13;202:1067–1071. doi: 10.1038/2021067b0. [DOI] [PubMed] [Google Scholar]
- Haugen P., Sten-Knudsen O. Sarcomere lengthening and tension drop in the latent period of isolated frog skeletal muscle fibers. J Gen Physiol. 1976 Sep;68(3):247–265. doi: 10.1085/jgp.68.3.247. [DOI] [PMC free article] [PubMed] [Google Scholar]
- Henry G. H., Bishop P. O., Tupper R. M., Dreher B. Orientation specificity and response variability of cells in the striate cortex. Vision Res. 1973 Sep;13(9):1771–1779. doi: 10.1016/0042-6989(73)90094-1. [DOI] [PubMed] [Google Scholar]
- Hill D. K. Tension due to interaction between the sliding filaments in resting striated muscle. The effect of stimulation. J Physiol. 1968 Dec;199(3):637–684. doi: 10.1113/jphysiol.1968.sp008672. [DOI] [PMC free article] [PubMed] [Google Scholar]
- Hui C. S., Gilly W. F. Mechanical activation and voltage-dependent charge movement in stretched muscle fibres. Nature. 1979 Sep 20;281(5728):223–225. doi: 10.1038/281223a0. [DOI] [PubMed] [Google Scholar]
- Huxley A. F. Muscular contraction. J Physiol. 1974 Nov;243(1):1–43. [PMC free article] [PubMed] [Google Scholar]
- Huxley H. E., Brown W. The low-angle x-ray diagram of vertebrate striated muscle and its behaviour during contraction and rigor. J Mol Biol. 1967 Dec 14;30(2):383–434. doi: 10.1016/s0022-2836(67)80046-9. [DOI] [PubMed] [Google Scholar]
- Johnson J. D., Charlton S. C., Potter J. D. A fluorescence stopped flow analysis of Ca2+ exchange with troponin C. J Biol Chem. 1979 May 10;254(9):3497–3502. [PubMed] [Google Scholar]
- Levine B. A., Thornton J. M., Fernandes R., Kelly C. M., Mercola D. Comparison of the calcium- and magnesium-induced structural changes of troponin--C. A proton magnetic resonance study. Biochim Biophys Acta. 1978 Jul 21;535(1):11–24. doi: 10.1016/0005-2795(78)90028-4. [DOI] [PubMed] [Google Scholar]
- Lännergren J., Noth J. The effect of bathing solution tonicity on resting tension in frog muscle fibers. J Gen Physiol. 1973 Dec;62(6):737–755. doi: 10.1085/jgp.62.6.737. [DOI] [PMC free article] [PubMed] [Google Scholar]
- Matsumura M. On the nature of the latency relaxation of frog skeletal muscle. Jpn J Physiol. 1969 Dec;19(6):701–711. doi: 10.2170/jjphysiol.19.701. [DOI] [PubMed] [Google Scholar]
- Miledi R., Parker I., Schalow G. Measurement of calcium transients in frog muscle by the use of arsenazo III. Proc R Soc Lond B Biol Sci. 1977 Aug 22;198(1131):201–210. doi: 10.1098/rspb.1977.0094. [DOI] [PubMed] [Google Scholar]
- Mobley B. A., Eisenberg B. R. Sizes of components in frog skeletal muscle measured by methods of stereology. J Gen Physiol. 1975 Jul;66(1):31–45. doi: 10.1085/jgp.66.1.31. [DOI] [PMC free article] [PubMed] [Google Scholar]
- Mulieri L. A. The dependence of the latency relaxation on sarcomere length and other characteristics of isolated muscle fibres. J Physiol. 1972 Jun;223(2):333–354. doi: 10.1113/jphysiol.1972.sp009850. [DOI] [PMC free article] [PubMed] [Google Scholar]
- Oetliker H., Baylor S. M., Chandler W. K. Simultaneous changes in fluorescence and optical retardation in single muscle fibres during activity. Nature. 1975 Oct 23;257(5528):693–696. doi: 10.1038/257693a0. [DOI] [PubMed] [Google Scholar]
- PAGE S. G., HUXLEY H. E. FILAMENT LENGTHS IN STRIATED MUSCLE. J Cell Biol. 1963 Nov;19:369–390. doi: 10.1083/jcb.19.2.369. [DOI] [PMC free article] [PubMed] [Google Scholar]
- Page S. G. A comparison of the fine structures of frog slow and twitch muscle fibers. J Cell Biol. 1965 Aug;26(2):477–497. doi: 10.1083/jcb.26.2.477. [DOI] [PMC free article] [PubMed] [Google Scholar]
- Peachey L. D. The sarcoplasmic reticulum and transverse tubules of the frog's sartorius. J Cell Biol. 1965 Jun;25(3 Suppl):209–231. doi: 10.1083/jcb.25.3.209. [DOI] [PubMed] [Google Scholar]
- Suarez-Kurtz G., Parker I. Birefringence signals and calcium transients in skeletal muscle. Nature. 1977 Dec 22;270(5639):746–748. doi: 10.1038/270746a0. [DOI] [PubMed] [Google Scholar]