Abstract
Biophysical evidence has placed the binding site for the naturally occurring marine toxins tetrodotoxin (TTX) and saxitoxin (STX) in the external mouth of the Na+ channel ion permeation pathway. We developed a molecular model of the binding pocket for TTX and STX, composed of antiparallel beta-hairpins formed from peptide segments of the four S5-S6 loops of the voltage-gated Na+ channel. For TTX the guanidinium moiety formed salt bridges with three carboxyls, while two toxin hydroxyls (C9-OH and C10-OH) interacted with a fourth carboxyl on repeats I and II. This alignment also resulted in a hydrophobic interaction with an aromatic ring of phenylalanine or tyrosine residues for the brainII and skeletal Na+ channel isoforms, but not with the cysteine found in the cardiac isoform. In comparison to TTX, there was an additional interaction site for STX through its second guanidinium group with a carboxyl on repeat IV. This model satisfactorily reproduced the effects of mutations in the S5-S6 regions and the differences in affinity by various toxin analogs. However, this model differed in important ways from previously published models for the outer vestibule and the selectivity region of the Na+ channel pore. Removal of the toxins from the pocket formed by the four beta-hairpins revealed a structure resembling a funnel that terminated in a narrowed region suitable as a candidate for the selectivity filter of the channel. This region contained two carboxyls (Asp384 and Glu942) that substituted for molecules of water from the hydrated Na+ ion. Simulation of mutations in this region that have produced Ca2+ permeation of the Na+ channel created a site with three carboxyls (Asp384, Glu942, and Glu1714) in proximity.
Full text
PDF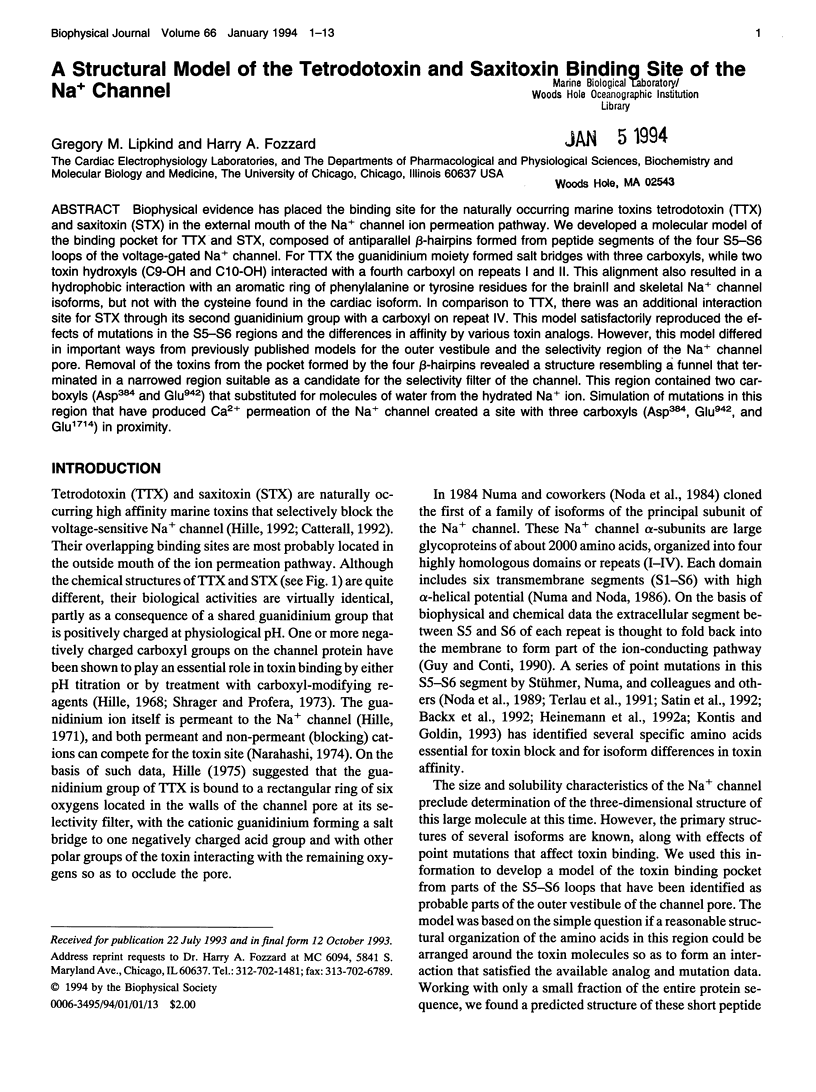
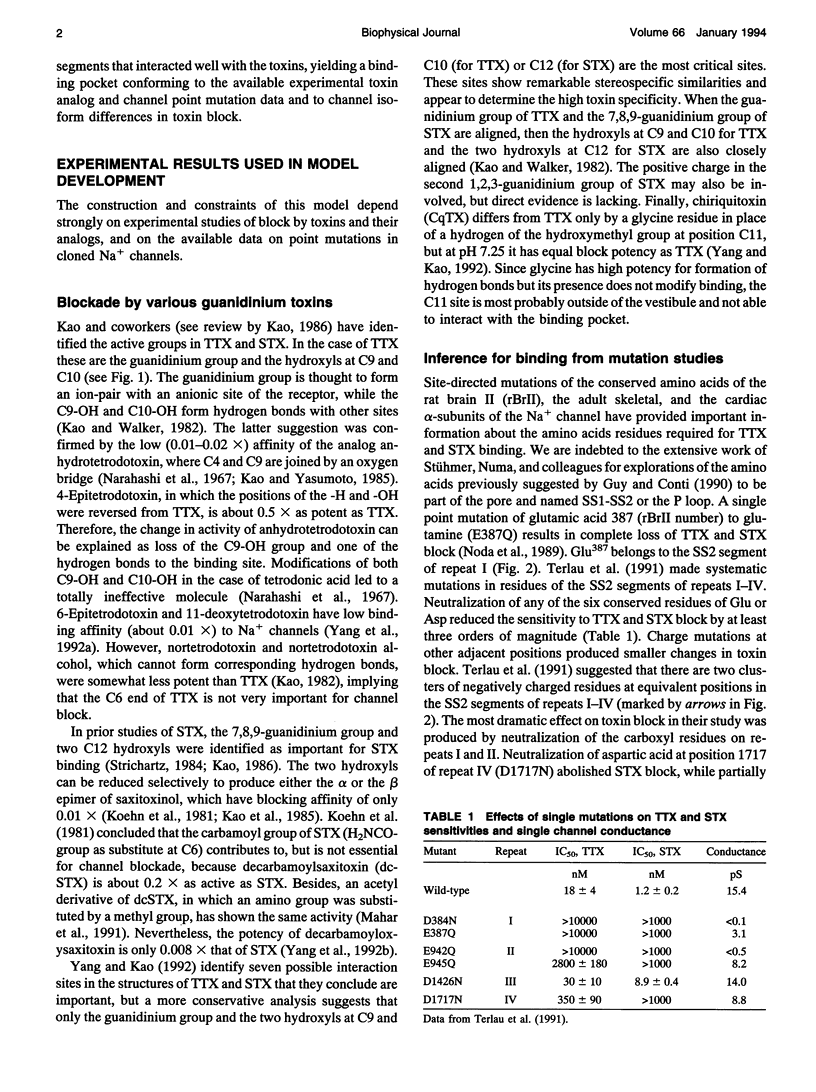
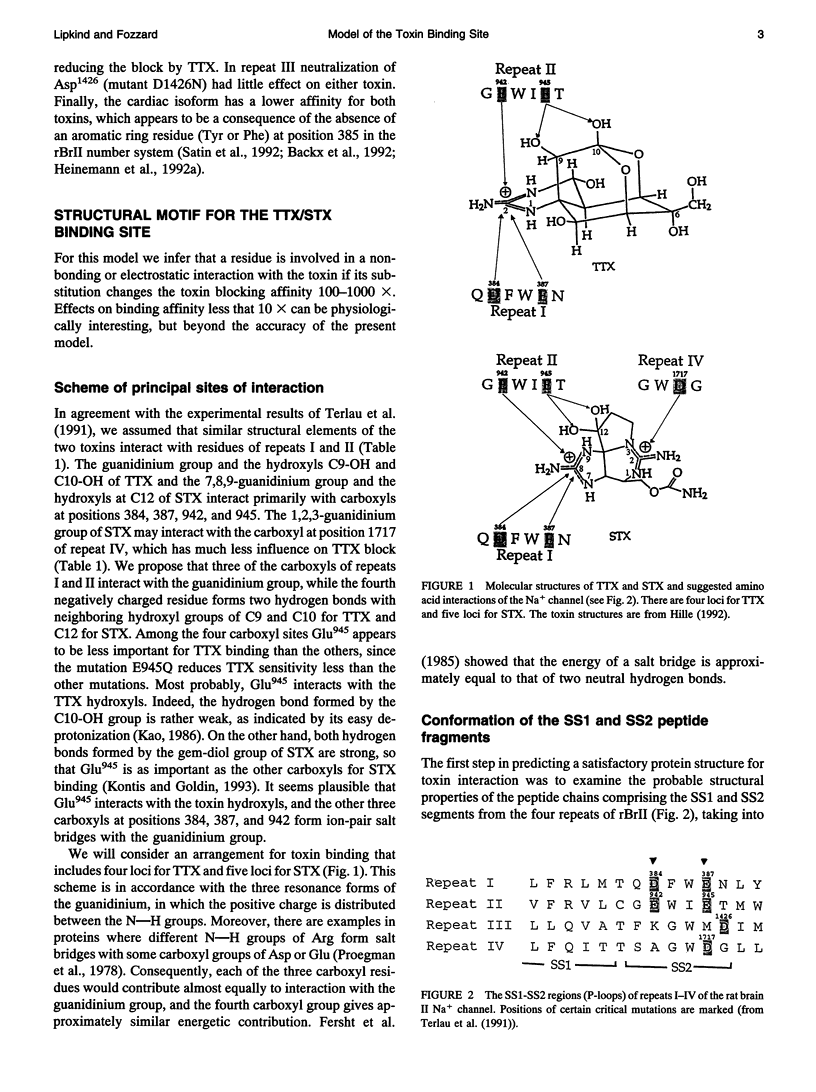
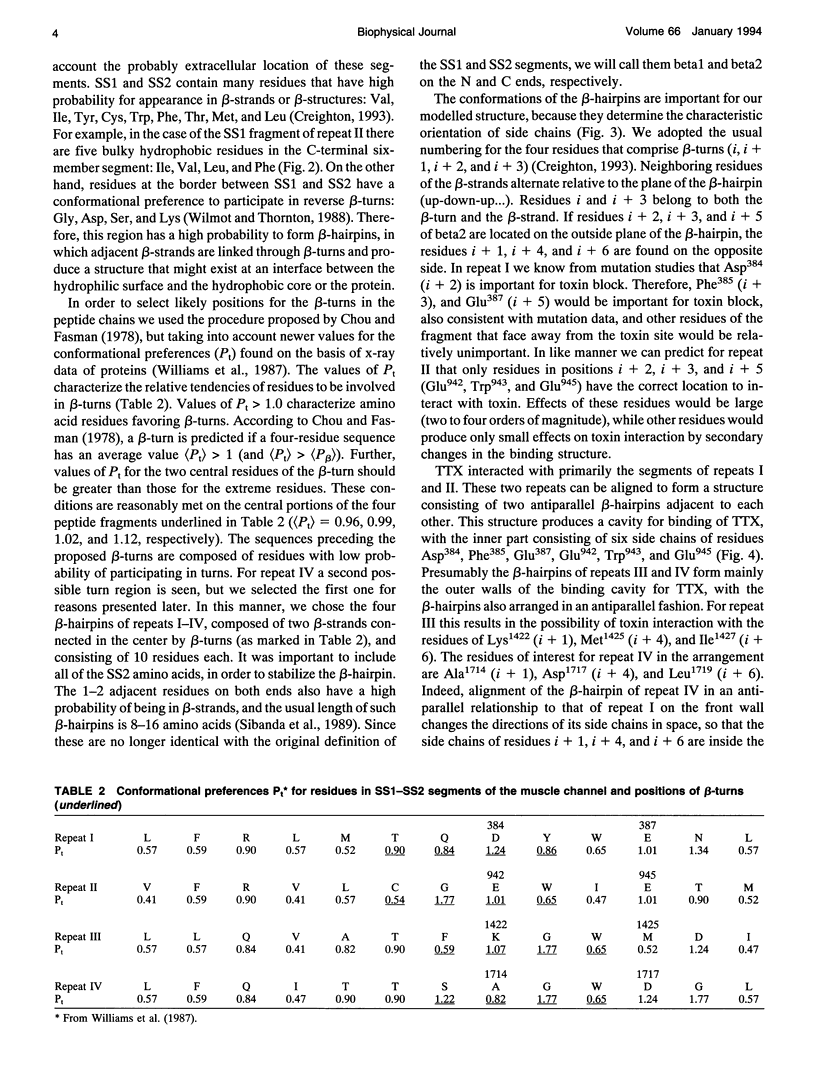
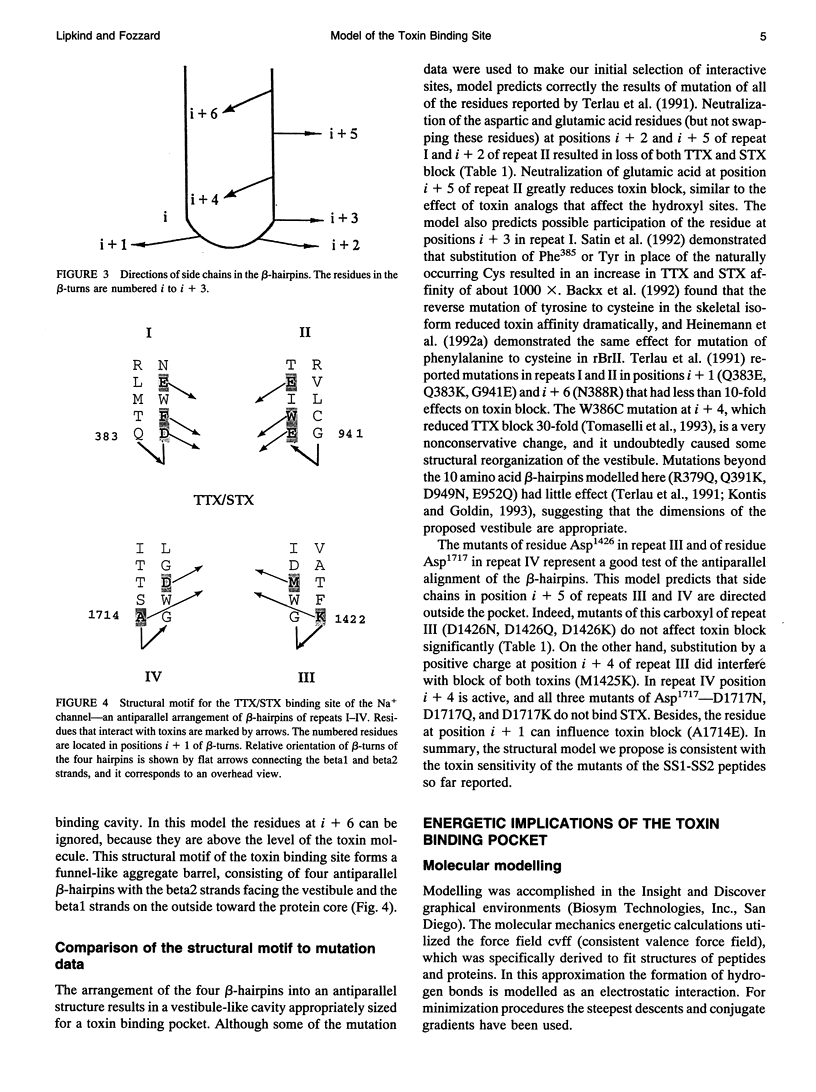
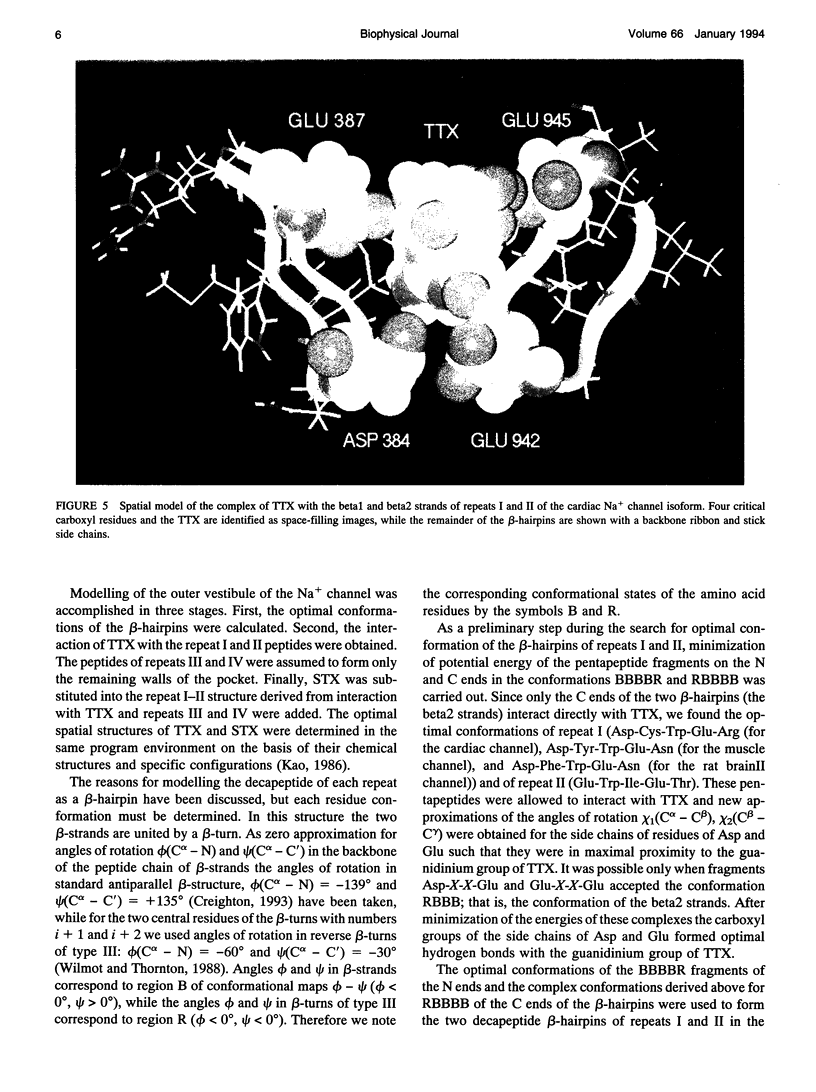
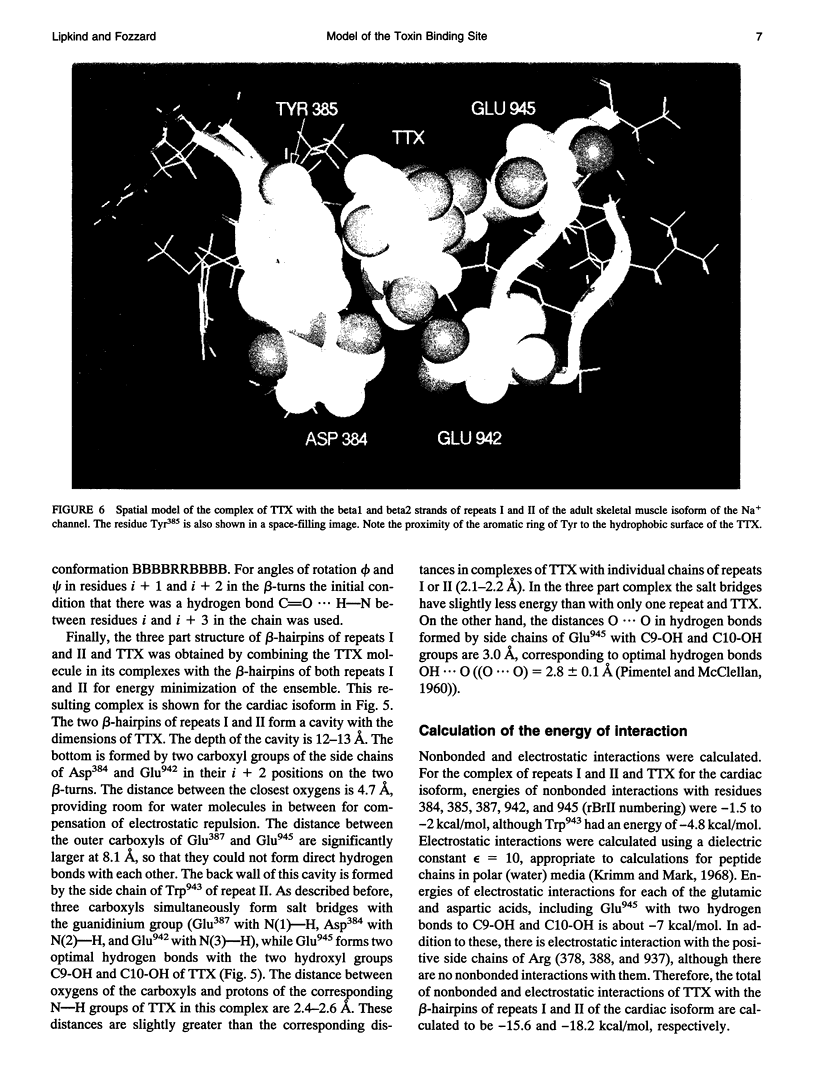
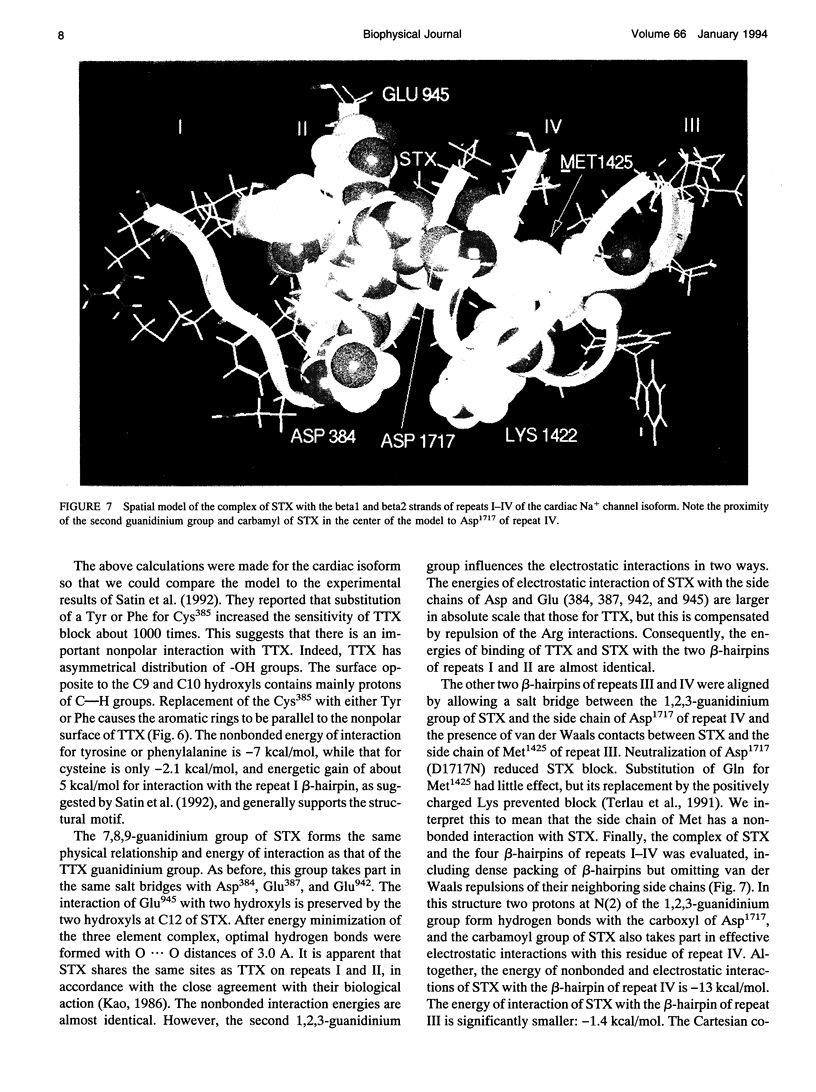
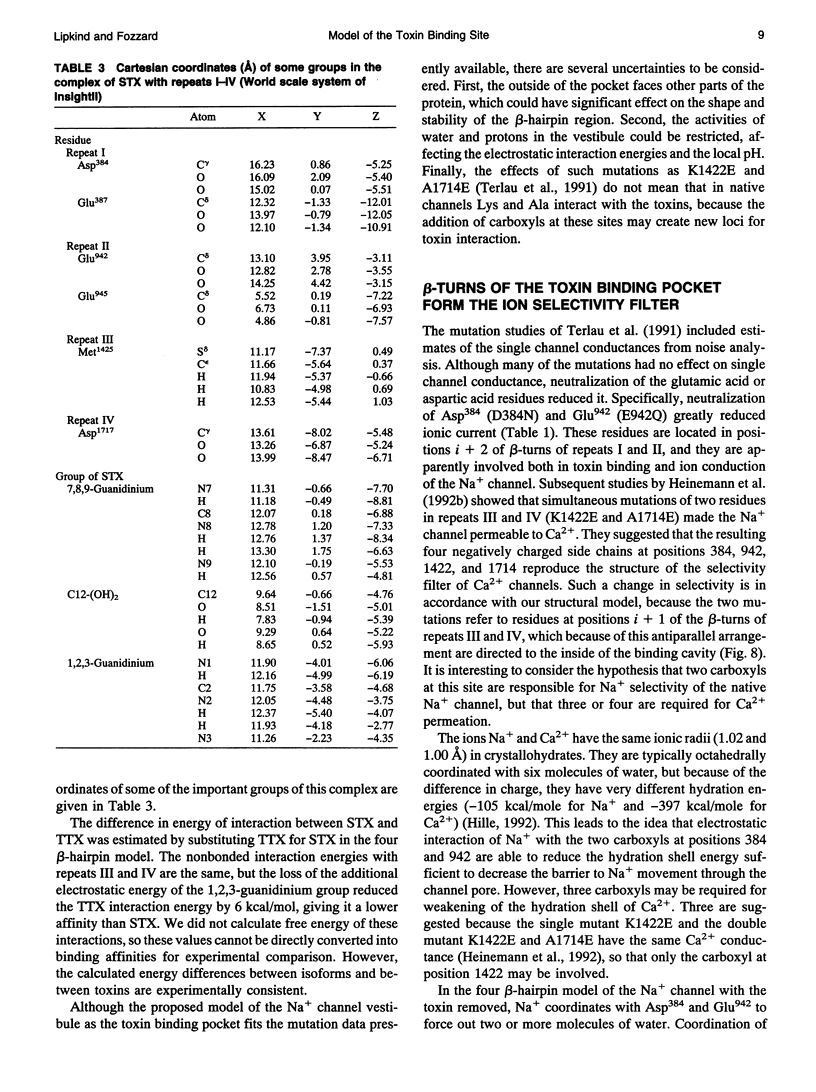
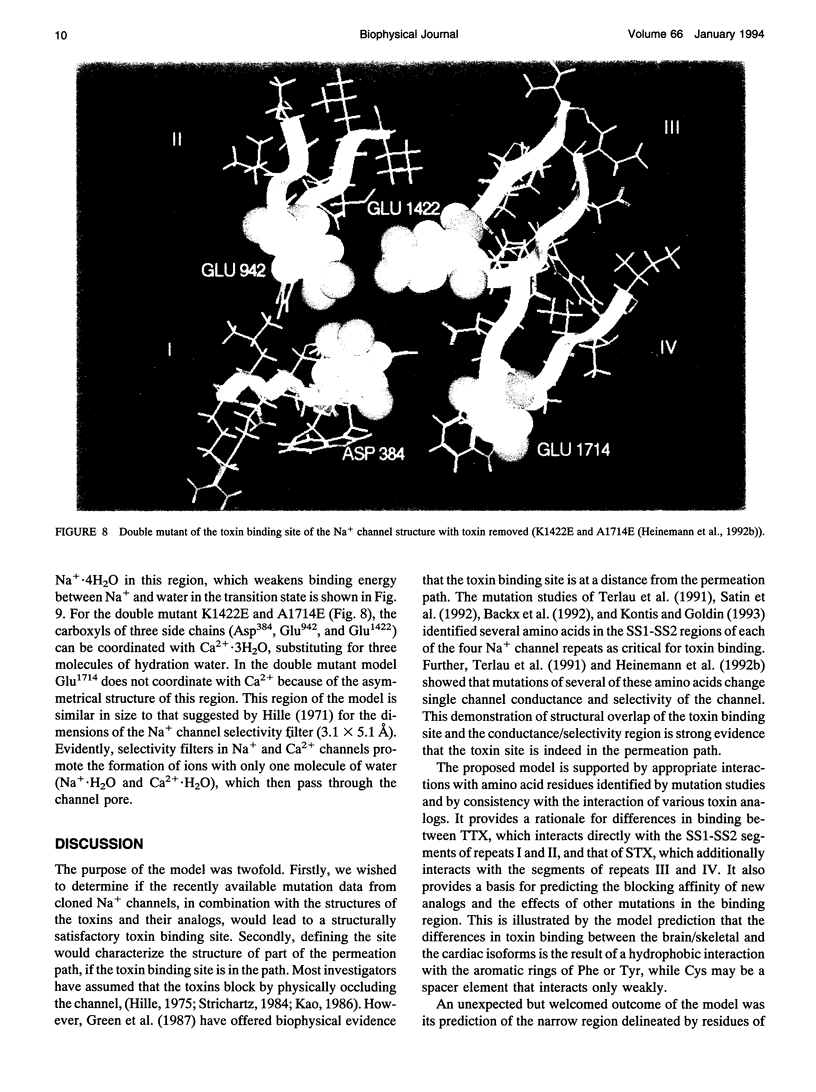
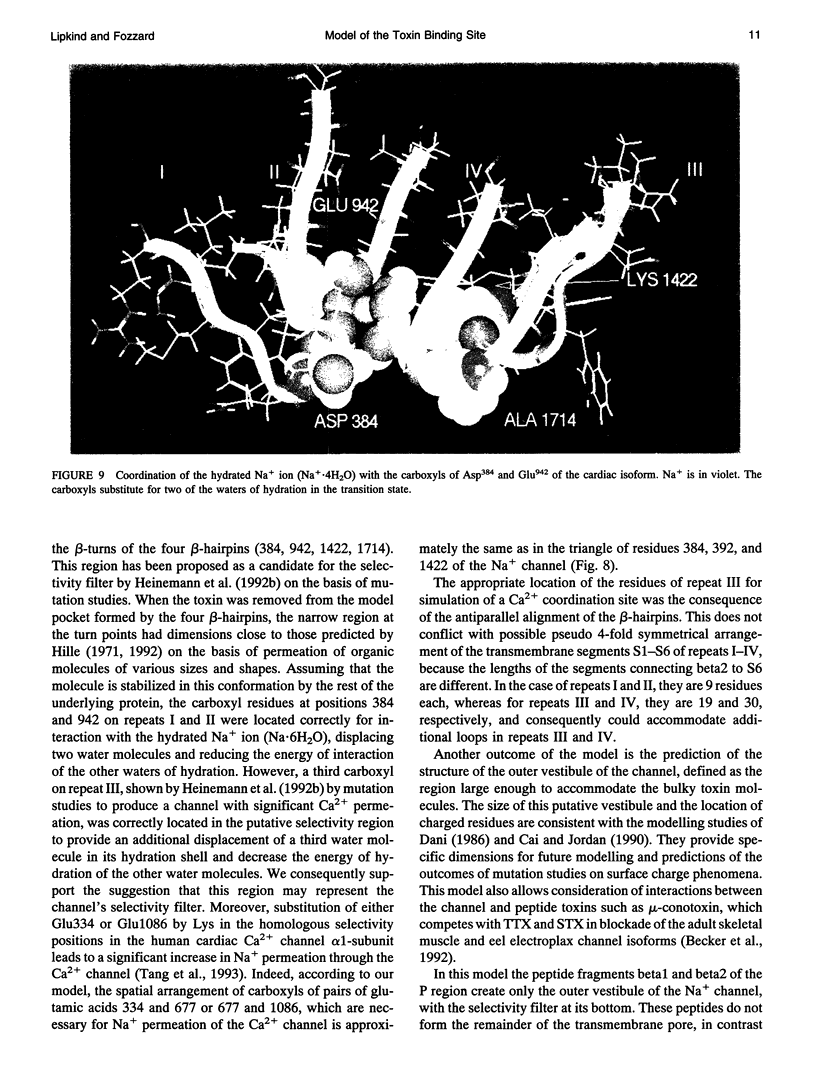
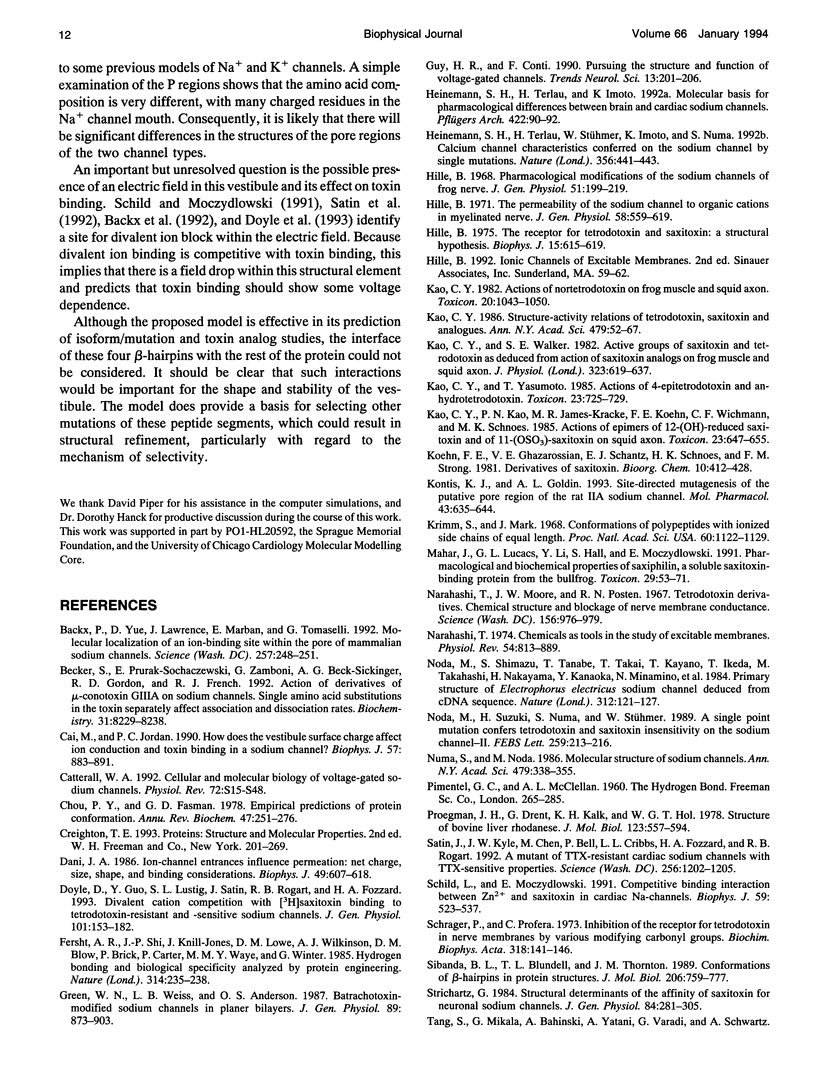
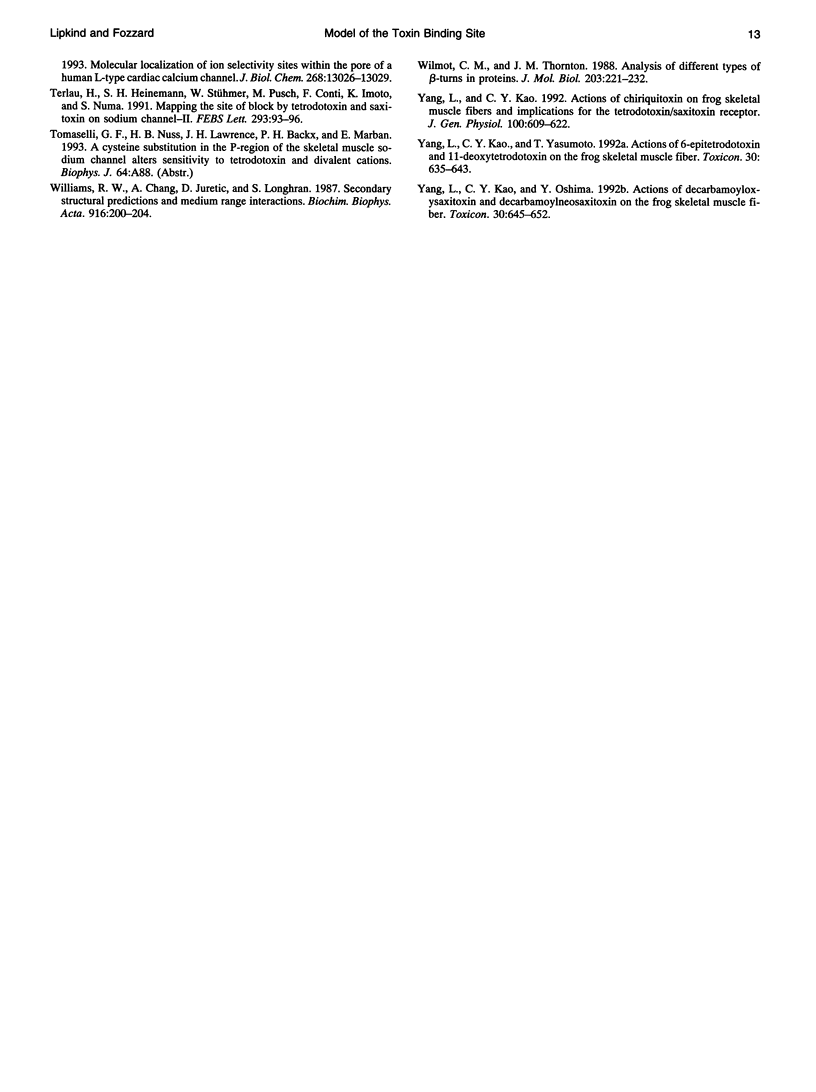
Images in this article
Selected References
These references are in PubMed. This may not be the complete list of references from this article.
- Backx P. H., Yue D. T., Lawrence J. H., Marban E., Tomaselli G. F. Molecular localization of an ion-binding site within the pore of mammalian sodium channels. Science. 1992 Jul 10;257(5067):248–251. doi: 10.1126/science.1321496. [DOI] [PubMed] [Google Scholar]
- Becker S., Prusak-Sochaczewski E., Zamponi G., Beck-Sickinger A. G., Gordon R. D., French R. J. Action of derivatives of mu-conotoxin GIIIA on sodium channels. Single amino acid substitutions in the toxin separately affect association and dissociation rates. Biochemistry. 1992 Sep 8;31(35):8229–8238. doi: 10.1021/bi00150a016. [DOI] [PubMed] [Google Scholar]
- Cai M., Jordan P. C. How does vestibule surface charge affect ion conduction and toxin binding in a sodium channel? Biophys J. 1990 Apr;57(4):883–891. doi: 10.1016/S0006-3495(90)82608-4. [DOI] [PMC free article] [PubMed] [Google Scholar]
- Catterall W. A. Cellular and molecular biology of voltage-gated sodium channels. Physiol Rev. 1992 Oct;72(4 Suppl):S15–S48. doi: 10.1152/physrev.1992.72.suppl_4.S15. [DOI] [PubMed] [Google Scholar]
- Chou P. Y., Fasman G. D. Empirical predictions of protein conformation. Annu Rev Biochem. 1978;47:251–276. doi: 10.1146/annurev.bi.47.070178.001343. [DOI] [PubMed] [Google Scholar]
- Dani J. A. Ion-channel entrances influence permeation. Net charge, size, shape, and binding considerations. Biophys J. 1986 Mar;49(3):607–618. doi: 10.1016/S0006-3495(86)83688-8. [DOI] [PMC free article] [PubMed] [Google Scholar]
- Doyle D. D., Guo Y., Lustig S. L., Satin J., Rogart R. B., Fozzard H. A. Divalent cation competition with [3H]saxitoxin binding to tetrodotoxin-resistant and -sensitive sodium channels. A two-site structural model of ion/toxin interaction. J Gen Physiol. 1993 Feb;101(2):153–182. doi: 10.1085/jgp.101.2.153. [DOI] [PMC free article] [PubMed] [Google Scholar]
- Fersht A. R., Shi J. P., Knill-Jones J., Lowe D. M., Wilkinson A. J., Blow D. M., Brick P., Carter P., Waye M. M., Winter G. Hydrogen bonding and biological specificity analysed by protein engineering. Nature. 1985 Mar 21;314(6008):235–238. doi: 10.1038/314235a0. [DOI] [PubMed] [Google Scholar]
- Green W. N., Weiss L. B., Andersen O. S. Batrachotoxin-modified sodium channels in planar lipid bilayers. Characterization of saxitoxin- and tetrodotoxin-induced channel closures. J Gen Physiol. 1987 Jun;89(6):873–903. doi: 10.1085/jgp.89.6.873. [DOI] [PMC free article] [PubMed] [Google Scholar]
- Guy H. R., Conti F. Pursuing the structure and function of voltage-gated channels. Trends Neurosci. 1990 Jun;13(6):201–206. doi: 10.1016/0166-2236(90)90160-c. [DOI] [PubMed] [Google Scholar]
- Heinemann S. H., Terlau H., Imoto K. Molecular basis for pharmacological differences between brain and cardiac sodium channels. Pflugers Arch. 1992 Oct;422(1):90–92. doi: 10.1007/BF00381519. [DOI] [PubMed] [Google Scholar]
- Heinemann S. H., Terlau H., Stühmer W., Imoto K., Numa S. Calcium channel characteristics conferred on the sodium channel by single mutations. Nature. 1992 Apr 2;356(6368):441–443. doi: 10.1038/356441a0. [DOI] [PubMed] [Google Scholar]
- Hille B. Pharmacological modifications of the sodium channels of frog nerve. J Gen Physiol. 1968 Feb;51(2):199–219. doi: 10.1085/jgp.51.2.199. [DOI] [PMC free article] [PubMed] [Google Scholar]
- Hille B. The permeability of the sodium channel to organic cations in myelinated nerve. J Gen Physiol. 1971 Dec;58(6):599–619. doi: 10.1085/jgp.58.6.599. [DOI] [PMC free article] [PubMed] [Google Scholar]
- Hille B. The receptor for tetrodotoxin and saxitoxin. A structural hypothesis. Biophys J. 1975 Jun;15(6):615–619. doi: 10.1016/S0006-3495(75)85842-5. [DOI] [PMC free article] [PubMed] [Google Scholar]
- Kao C. Y. Actions of nortetrodotoxin on frog muscle and squid axon. Toxicon. 1982;20(6):1043–1050. doi: 10.1016/0041-0101(82)90106-4. [DOI] [PubMed] [Google Scholar]
- Kao C. Y., Kao P. N., James-Kracke M. R., Koehn F. E., Wichmann C. F., Schnoes H. K. Actions of epimers of 12-(OH)-reduced saxitoxin and of 11-(OSO3)-saxitoxin on squid axon. Toxicon. 1985;23(4):647–655. doi: 10.1016/0041-0101(85)90369-1. [DOI] [PubMed] [Google Scholar]
- Kao C. Y. Structure-activity relations of tetrodotoxin, saxitoxin, and analogues. Ann N Y Acad Sci. 1986;479:52–67. doi: 10.1111/j.1749-6632.1986.tb15561.x. [DOI] [PubMed] [Google Scholar]
- Kao C. Y., Walker S. E. Active groups of saxitoxin and tetrodotoxin as deduced from actions of saxitoxin analogues on frog muscle and squid axon. J Physiol. 1982 Feb;323:619–637. doi: 10.1113/jphysiol.1982.sp014095. [DOI] [PMC free article] [PubMed] [Google Scholar]
- Kao C. Y., Yasumoto T. Actions of 4-epitetrodotoxin and anhydrotetrodotoxin on the squid axon. Toxicon. 1985;23(5):725–729. doi: 10.1016/0041-0101(85)90002-9. [DOI] [PubMed] [Google Scholar]
- Kontis K. J., Goldin A. L. Site-directed mutagenesis of the putative pore region of the rat IIA sodium channel. Mol Pharmacol. 1993 Apr;43(4):635–644. [PubMed] [Google Scholar]
- Krimm S., Mark J. E. Conformations of polypeptides with ionized side chains of equal length. Proc Natl Acad Sci U S A. 1968 Aug;60(4):1122–1129. doi: 10.1073/pnas.60.4.1122. [DOI] [PMC free article] [PubMed] [Google Scholar]
- Mahar J., Lukács G. L., Li Y., Hall S., Moczydlowski E. Pharmacological and biochemical properties of saxiphilin, a soluble saxitoxin-binding protein from the bullfrog (Rana catesbeiana). Toxicon. 1991;29(1):53–71. doi: 10.1016/0041-0101(91)90039-t. [DOI] [PubMed] [Google Scholar]
- Narahashi T. Chemicals as tools in the study of excitable membranes. Physiol Rev. 1974 Oct;54(4):813–889. doi: 10.1152/physrev.1974.54.4.813. [DOI] [PubMed] [Google Scholar]
- Narahashi T., Moore J. W., Poston R. N. Tetrodotoxin derivatives: chemical structure and blockage of nerve membrane conductance. Science. 1967 May 19;156(3777):976–979. doi: 10.1126/science.156.3777.976. [DOI] [PubMed] [Google Scholar]
- Noda M., Shimizu S., Tanabe T., Takai T., Kayano T., Ikeda T., Takahashi H., Nakayama H., Kanaoka Y., Minamino N. Primary structure of Electrophorus electricus sodium channel deduced from cDNA sequence. Nature. 1984 Nov 8;312(5990):121–127. doi: 10.1038/312121a0. [DOI] [PubMed] [Google Scholar]
- Noda M., Suzuki H., Numa S., Stühmer W. A single point mutation confers tetrodotoxin and saxitoxin insensitivity on the sodium channel II. FEBS Lett. 1989 Dec 18;259(1):213–216. doi: 10.1016/0014-5793(89)81531-5. [DOI] [PubMed] [Google Scholar]
- Numa S., Noda M. Molecular structure of sodium channels. Ann N Y Acad Sci. 1986;479:338–355. doi: 10.1111/j.1749-6632.1986.tb15580.x. [DOI] [PubMed] [Google Scholar]
- Ploegman J. H., Drent G., Kalk K. H., Hol W. G. Structure of bovine liver rhodanese. I. Structure determination at 2.5 A resolution and a comparison of the conformation and sequence of its two domains. J Mol Biol. 1978 Aug 25;123(4):557–594. [PubMed] [Google Scholar]
- Satin J., Kyle J. W., Chen M., Bell P., Cribbs L. L., Fozzard H. A., Rogart R. B. A mutant of TTX-resistant cardiac sodium channels with TTX-sensitive properties. Science. 1992 May 22;256(5060):1202–1205. doi: 10.1126/science.256.5060.1202. [DOI] [PubMed] [Google Scholar]
- Schild L., Moczydlowski E. Competitive binding interaction between Zn2+ and saxitoxin in cardiac Na+ channels. Evidence for a sulfhydryl group in the Zn2+/saxitoxin binding site. Biophys J. 1991 Mar;59(3):523–537. doi: 10.1016/S0006-3495(91)82269-X. [DOI] [PMC free article] [PubMed] [Google Scholar]
- Shrager P., Profera C. Inhibition of the receptor for tetrodotoxin in nerve membranes by reagents modifying carboxyl groups. Biochim Biophys Acta. 1973 Aug 9;318(1):141–146. doi: 10.1016/0005-2736(73)90343-x. [DOI] [PubMed] [Google Scholar]
- Sibanda B. L., Blundell T. L., Thornton J. M. Conformation of beta-hairpins in protein structures. A systematic classification with applications to modelling by homology, electron density fitting and protein engineering. J Mol Biol. 1989 Apr 20;206(4):759–777. doi: 10.1016/0022-2836(89)90583-4. [DOI] [PubMed] [Google Scholar]
- Strichartz G. Structural determinants of the affinity of saxitoxin for neuronal sodium channels. Electrophysiological studies on frog peripheral nerve. J Gen Physiol. 1984 Aug;84(2):281–305. doi: 10.1085/jgp.84.2.281. [DOI] [PMC free article] [PubMed] [Google Scholar]
- Terlau H., Heinemann S. H., Stühmer W., Pusch M., Conti F., Imoto K., Numa S. Mapping the site of block by tetrodotoxin and saxitoxin of sodium channel II. FEBS Lett. 1991 Nov 18;293(1-2):93–96. doi: 10.1016/0014-5793(91)81159-6. [DOI] [PubMed] [Google Scholar]
- Williams R. W., Chang A., Juretić D., Loughran S. Secondary structure predictions and medium range interactions. Biochim Biophys Acta. 1987 Nov 26;916(2):200–204. doi: 10.1016/0167-4838(87)90109-9. [DOI] [PubMed] [Google Scholar]
- Wilmot C. M., Thornton J. M. Analysis and prediction of the different types of beta-turn in proteins. J Mol Biol. 1988 Sep 5;203(1):221–232. doi: 10.1016/0022-2836(88)90103-9. [DOI] [PubMed] [Google Scholar]
- Yang L., Kao C. Y. Actions of chiriquitoxin on frog skeletal muscle fibers and implications for the tetrodotoxin/saxitoxin receptor. J Gen Physiol. 1992 Oct;100(4):609–622. doi: 10.1085/jgp.100.4.609. [DOI] [PMC free article] [PubMed] [Google Scholar]
- Yang L., Kao C. Y., Oshima Y. Actions of decarbamoyloxysaxitoxin and decarbamoylneosaxitoxin on the frog skeletal muscle fiber. Toxicon. 1992 May-Jun;30(5-6):645–652. doi: 10.1016/0041-0101(92)90858-3. [DOI] [PubMed] [Google Scholar]
- Yang L., Kao C. Y., Yasumoto T. Actions of 6-epitetrodotoxin and 11-deoxytetrodotoxin on the frog skeletal muscle fiber. Toxicon. 1992 May-Jun;30(5-6):635–643. doi: 10.1016/0041-0101(92)90857-2. [DOI] [PubMed] [Google Scholar]