Abstract
The lamellar/inverted hexagonal (L alpha/HII) phase transition can be very fast, despite the drastic change in the topology of the lipid/water interfaces. The first structures to form in this transition may be similar to those that mediate membrane fusion in many lipid systems. To study the transition mechanism and other dynamic phenomena in membrane dispersions, we constructed an apparatus to rapidly trigger the transition and then vitrify the specimens to preserve the structure of transient intermediates. The apparatus applies millisecond-long temperature jumps of variable size to aqueous dispersions of lipids on electron microscope grids at times 9-16 ms before specimen vitrification. The vitrified specimens are then examined by cryo-transmission electron microscopy. Dispersions of egg phosphatidylethanolamine completed the transition within 9 ms when superheated by 20 K. Similar transition times have been observed in dioleoylphosphatidylethanolamine via time-resolved x-ray diffraction. N-monomethylated dioleoylphosphatidylethanolamine dispersions superheated to lesser extent exhibited slower transitions and more complex morphology. The structure of the first intermediates to form in the transition process could not be determined, probably because the intermediates are labile on the time scale of sample cooling and vitrification (< 1 ms) and because of the poor contrast developed by some of these small structures. However, the results are more compatible with a transition mechanism based on "stalk" intermediates than a mechanism involving inverted micellar intermediates. Temperature-jump cryo-transmission electron microscopy should be useful in studying dynamic phenomena in biomembranes, large protein complexes, and other colloidal dispersions. It should be especially helpful in studying the mechanism of protein-induced membrane fusion.
Full text
PDF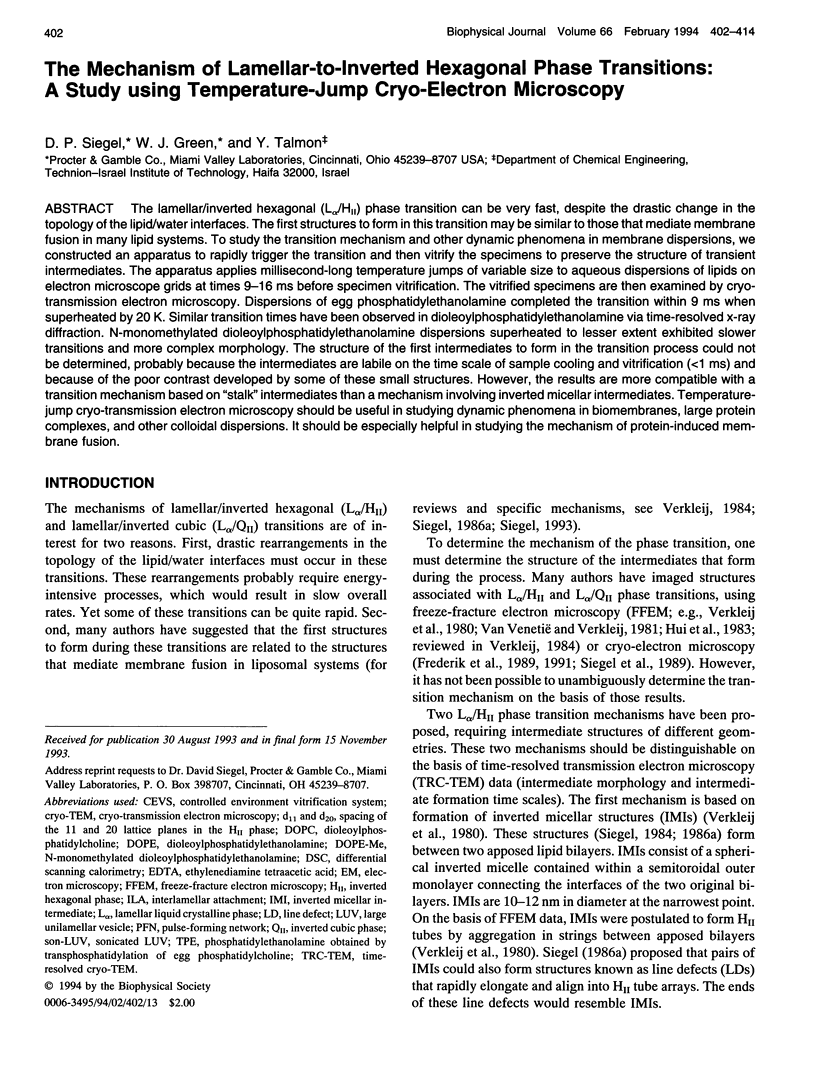
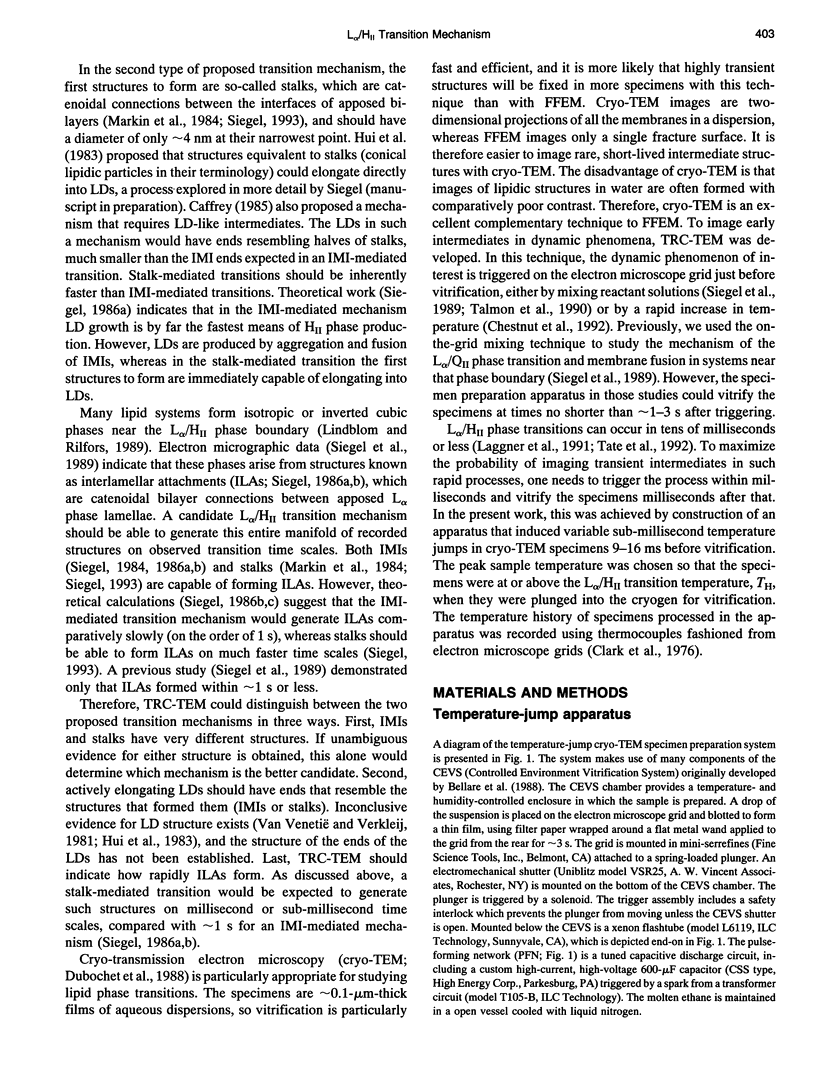
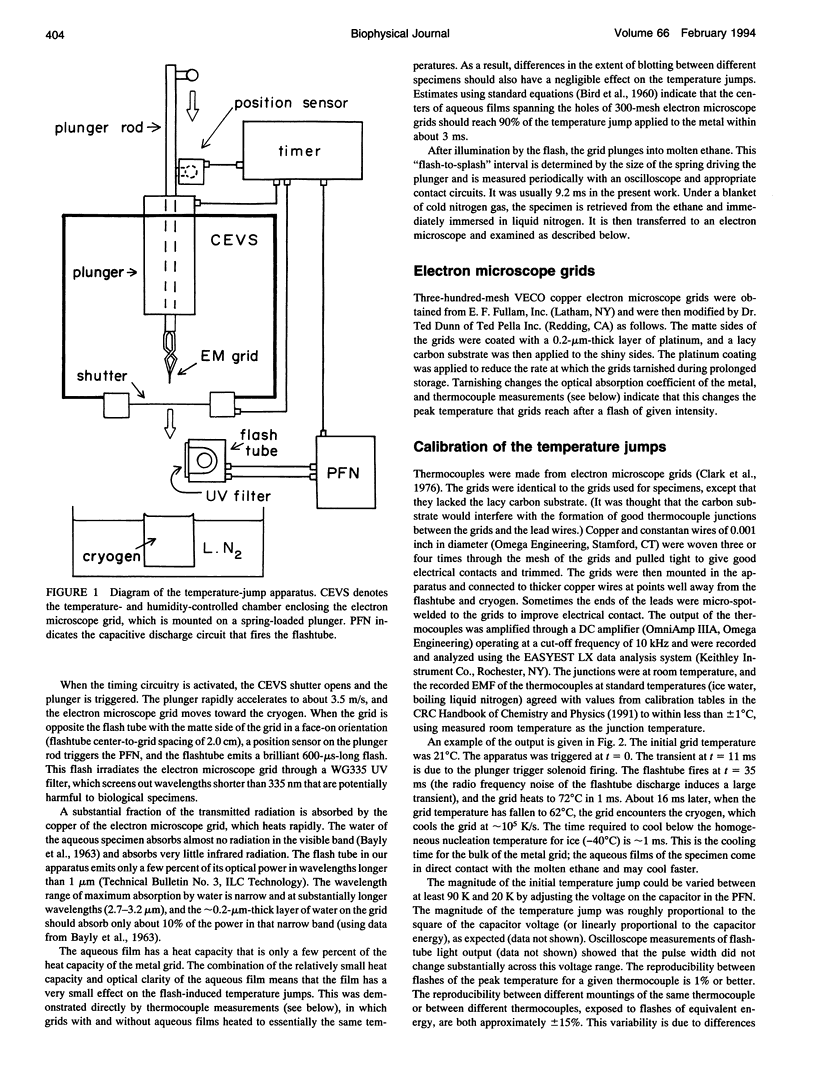
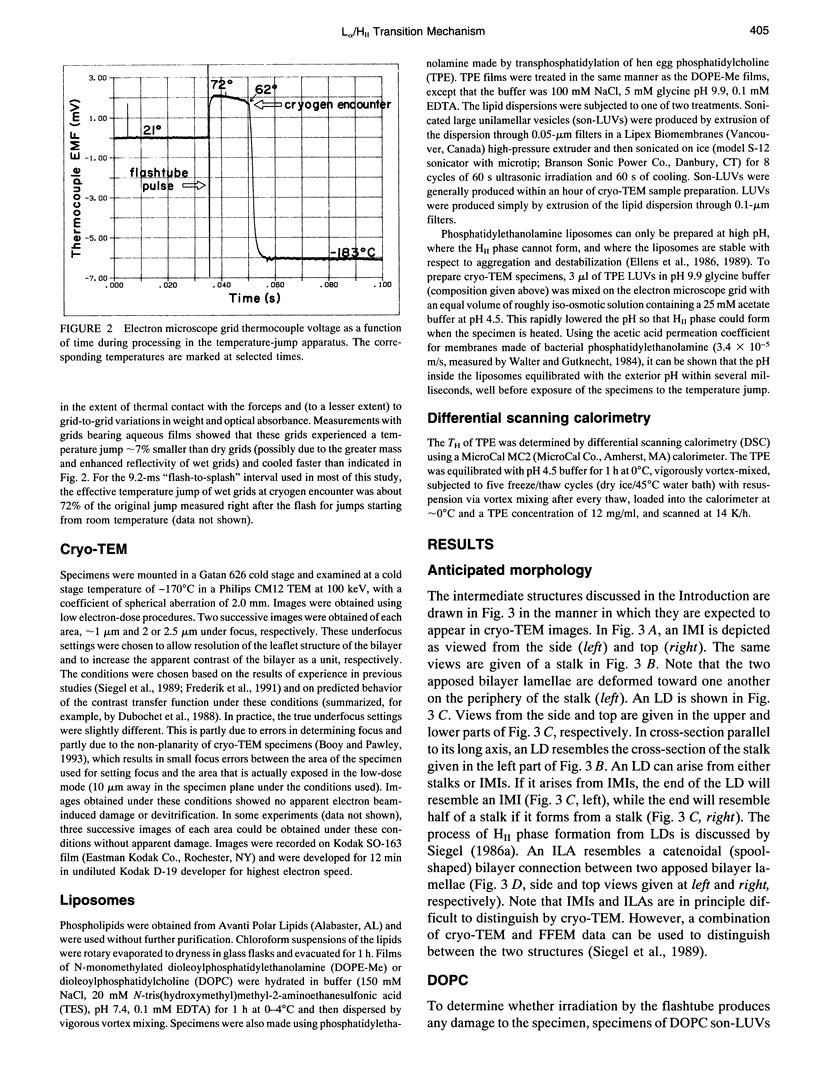
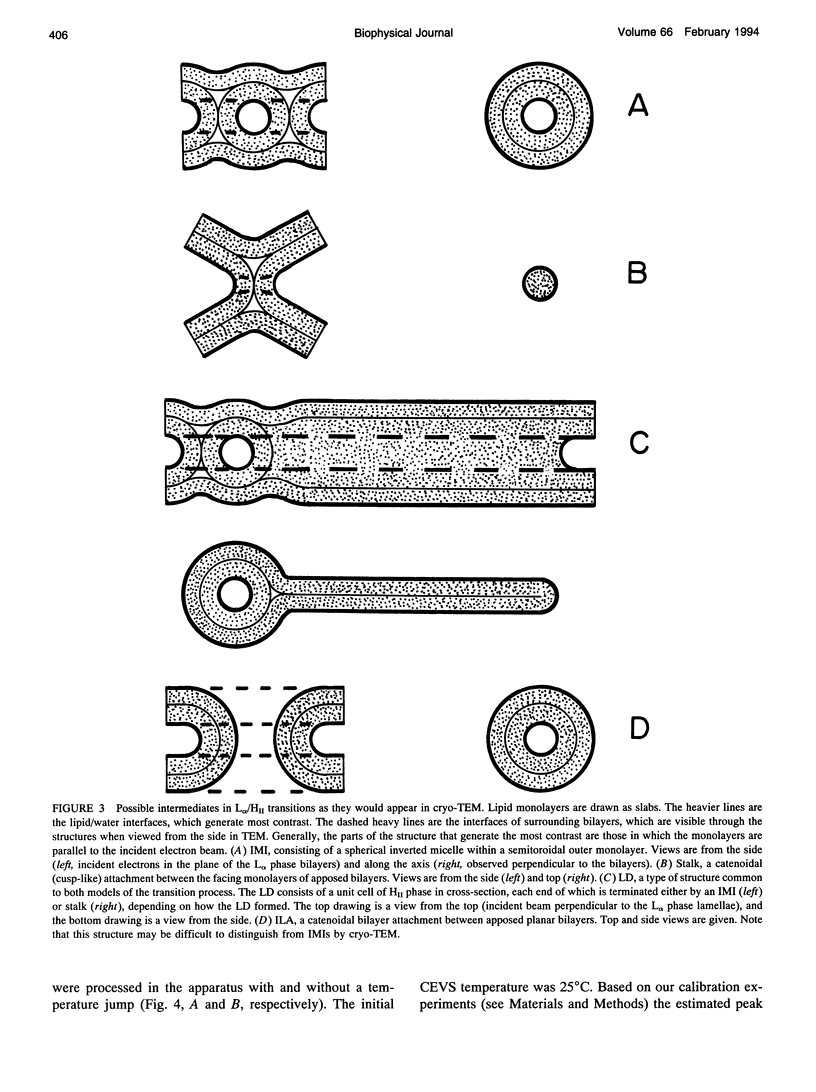
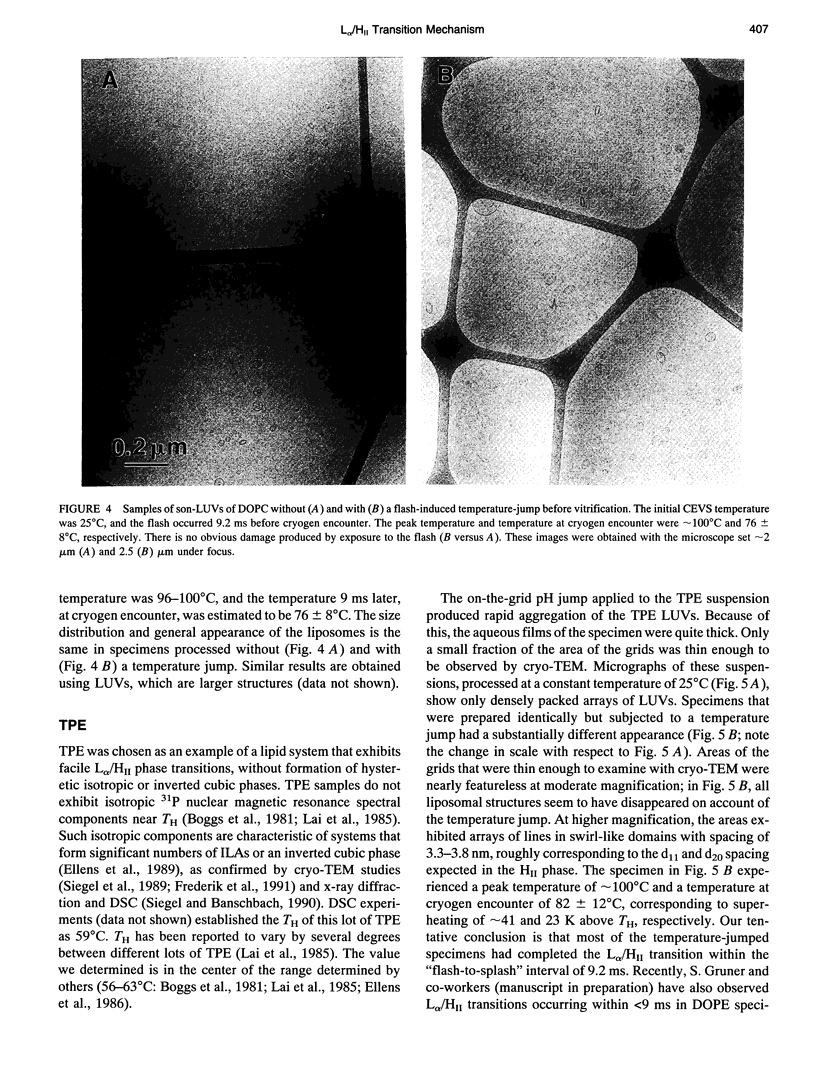
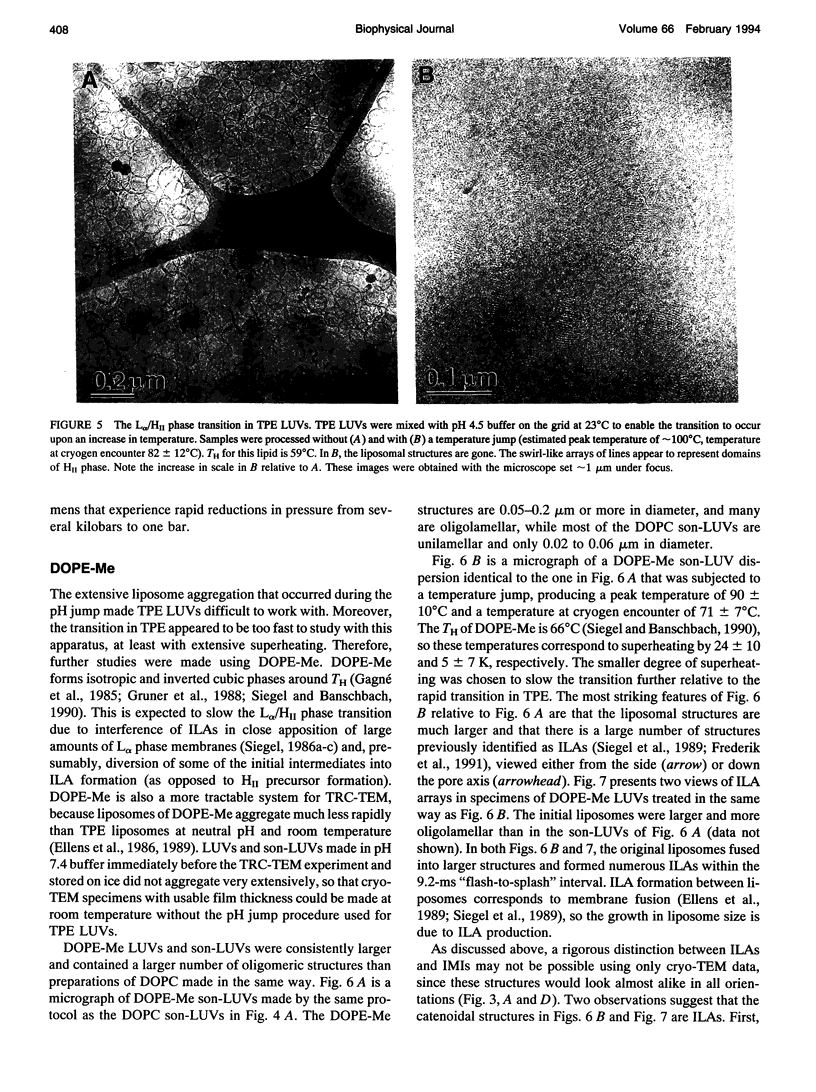
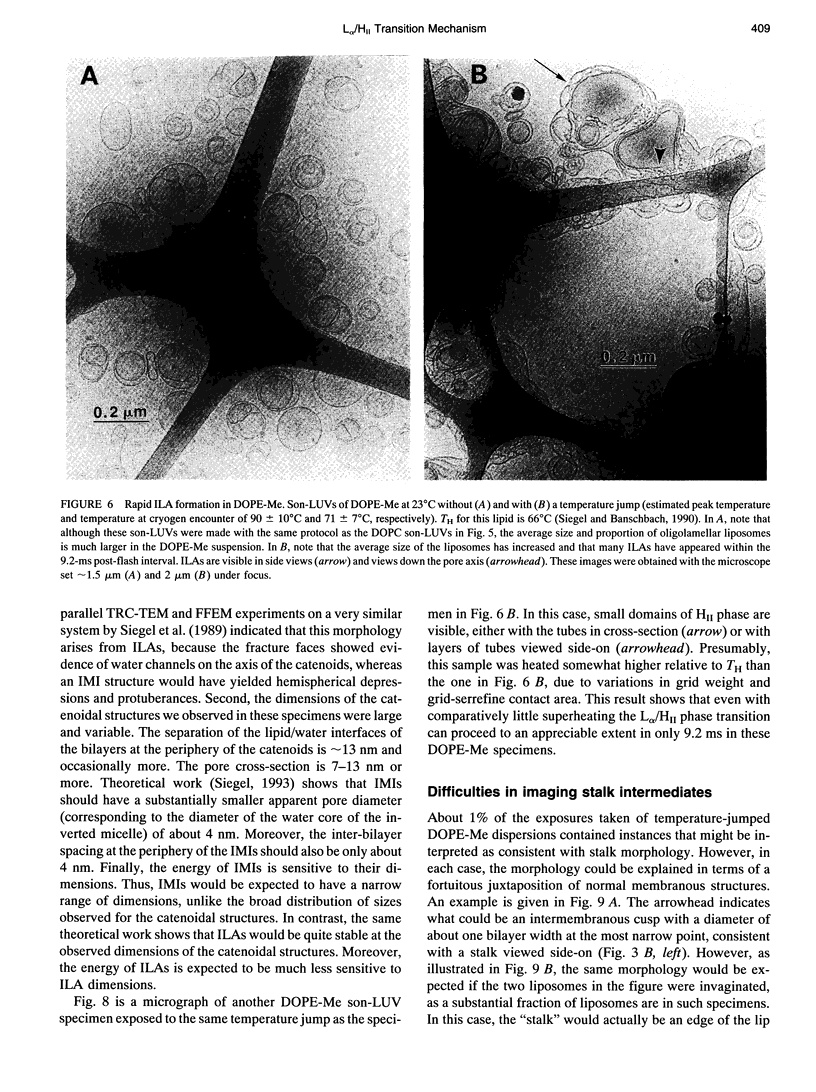
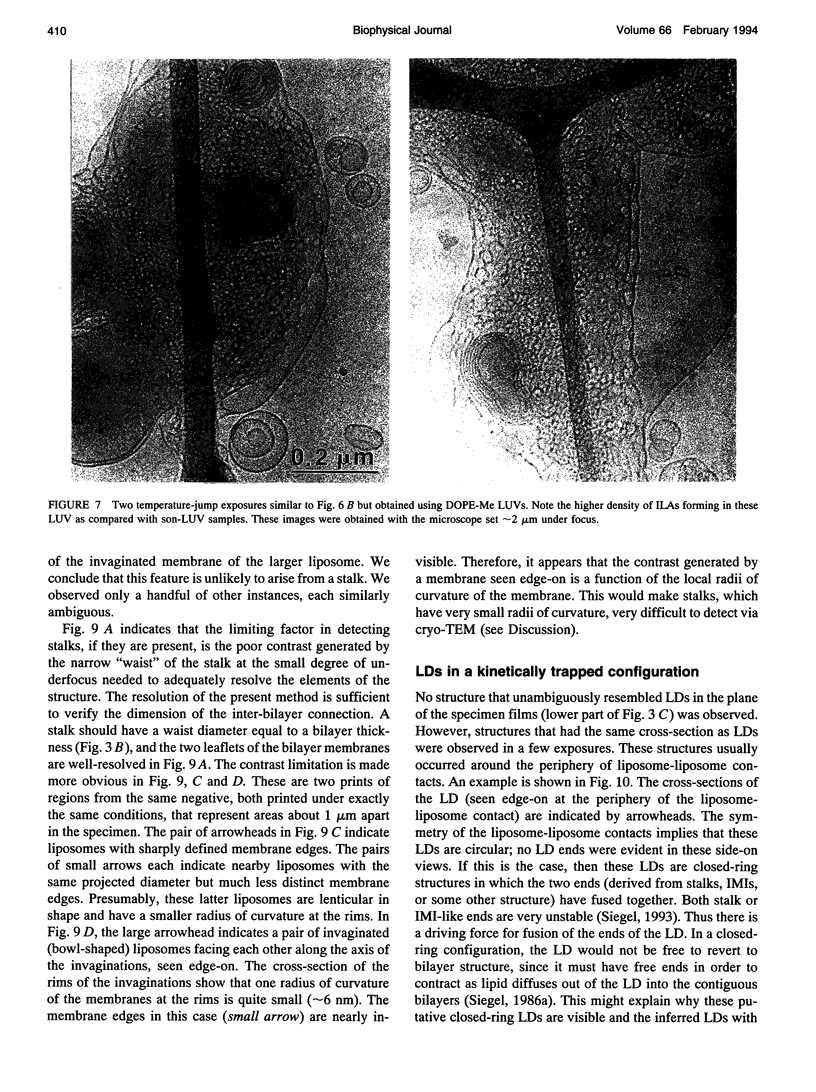
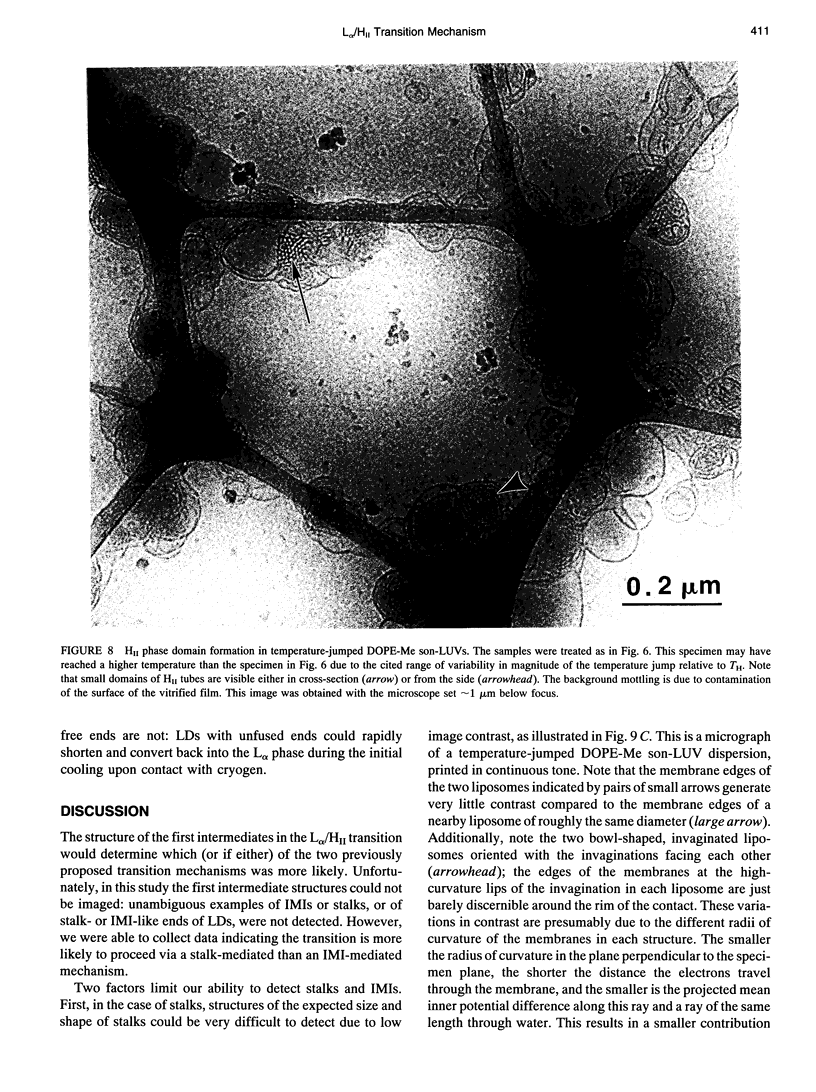
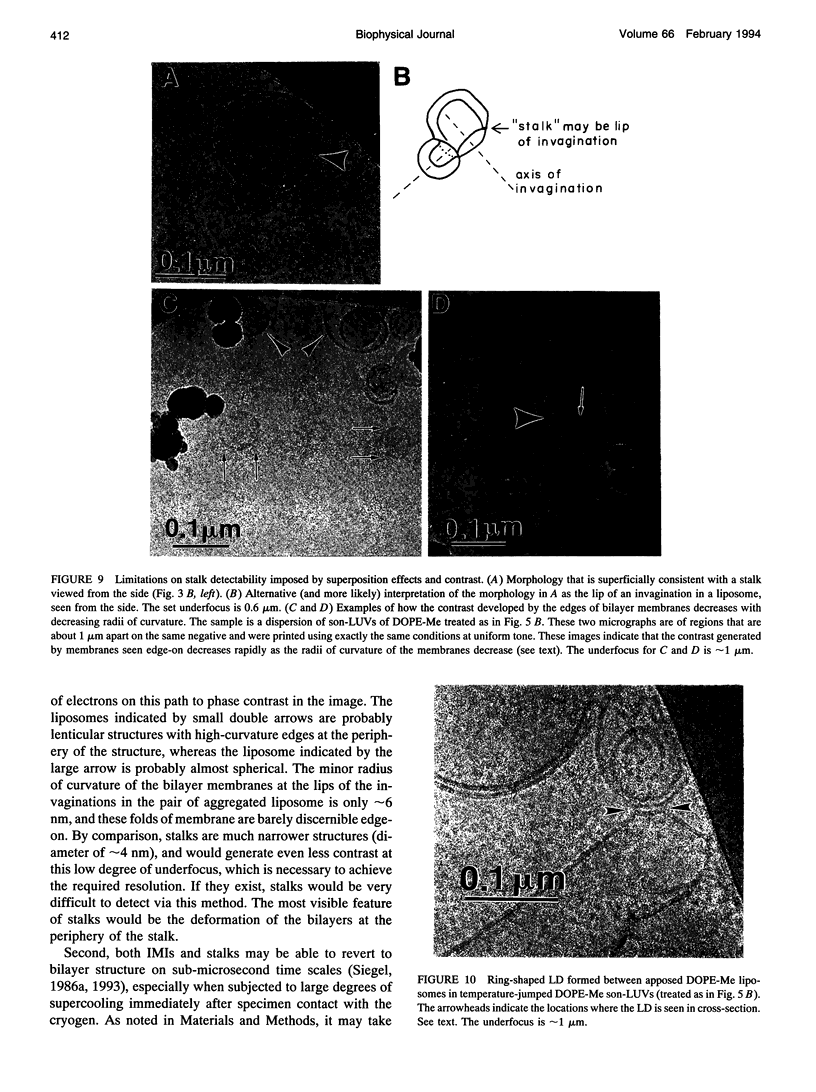
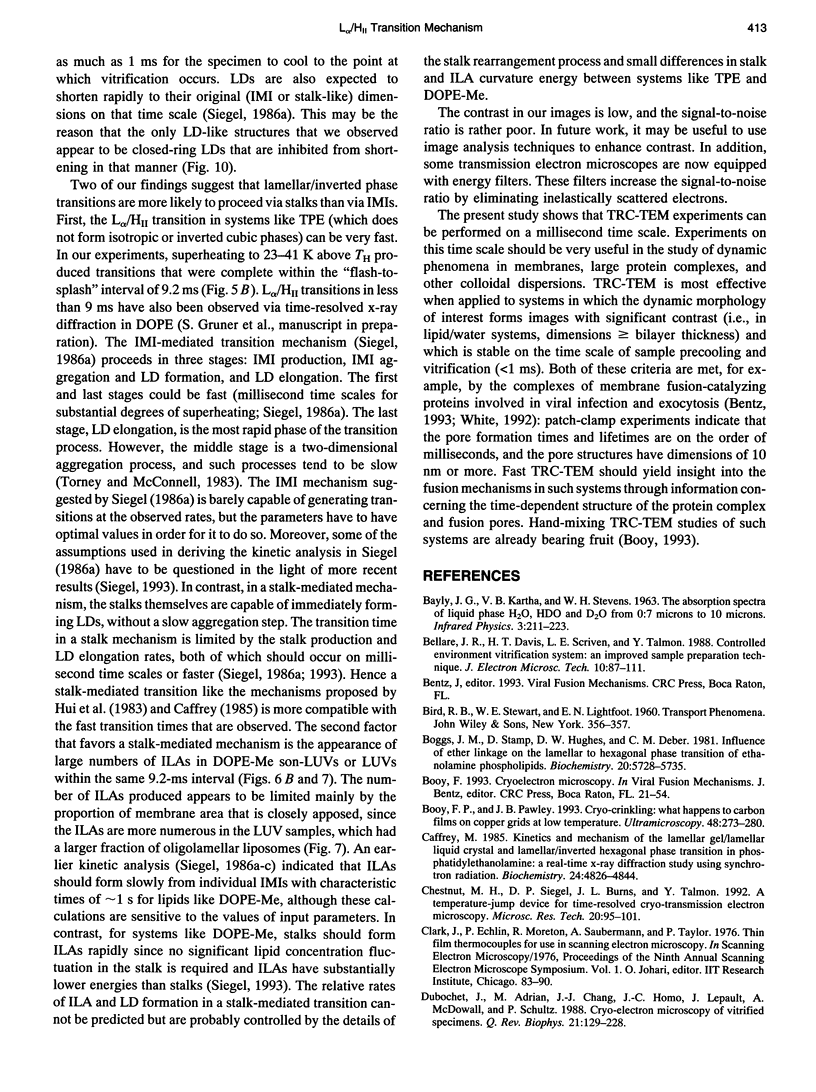
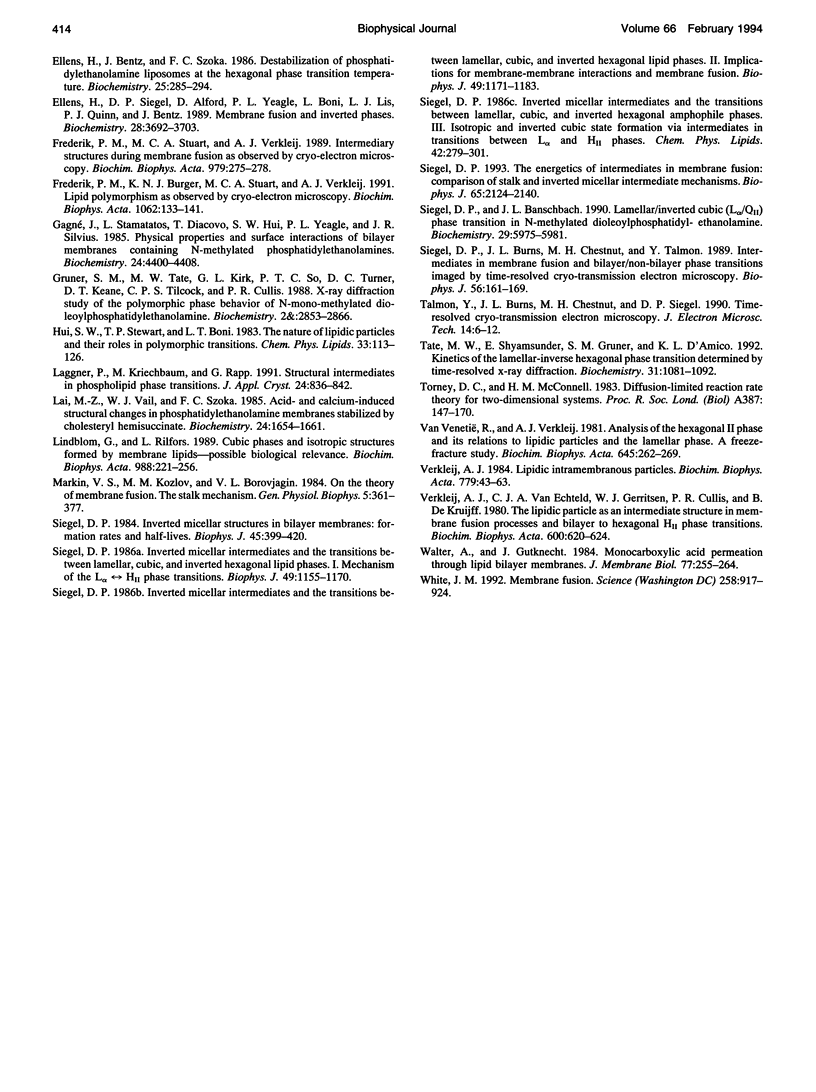
Images in this article
Selected References
These references are in PubMed. This may not be the complete list of references from this article.
- Bellare J. R., Davis H. T., Scriven L. E., Talmon Y. Controlled environment vitrification system: an improved sample preparation technique. J Electron Microsc Tech. 1988 Sep;10(1):87–111. doi: 10.1002/jemt.1060100111. [DOI] [PubMed] [Google Scholar]
- Boggs J. M., Stamp D., Hughes D. W., Deber C. M. Influence of ether linkage on the lamellar to hexagonal phase transition of ethanolamine phospholipids. Biochemistry. 1981 Sep 29;20(20):5728–5735. doi: 10.1021/bi00523a015. [DOI] [PubMed] [Google Scholar]
- Booy F. P., Pawley J. B. Cryo-crinkling: what happens to carbon films on copper grids at low temperature. Ultramicroscopy. 1993 Mar;48(3):273–280. doi: 10.1016/0304-3991(93)90101-3. [DOI] [PubMed] [Google Scholar]
- Caffrey M. Kinetics and mechanism of the lamellar gel/lamellar liquid-crystal and lamellar/inverted hexagonal phase transition in phosphatidylethanolamine: a real-time X-ray diffraction study using synchrotron radiation. Biochemistry. 1985 Aug 27;24(18):4826–4844. doi: 10.1021/bi00339a017. [DOI] [PubMed] [Google Scholar]
- Chestnut M. H., Siegel D. P., Burns J. L., Talmon Y. A temperature-jump device for time-resolved cryo-transmission electron microscopy. Microsc Res Tech. 1992 Jan 1;20(1):95–101. doi: 10.1002/jemt.1070200110. [DOI] [PubMed] [Google Scholar]
- Dubochet J., Adrian M., Chang J. J., Homo J. C., Lepault J., McDowall A. W., Schultz P. Cryo-electron microscopy of vitrified specimens. Q Rev Biophys. 1988 May;21(2):129–228. doi: 10.1017/s0033583500004297. [DOI] [PubMed] [Google Scholar]
- Ellens H., Bentz J., Szoka F. C. Destabilization of phosphatidylethanolamine liposomes at the hexagonal phase transition temperature. Biochemistry. 1986 Jan 28;25(2):285–294. doi: 10.1021/bi00350a001. [DOI] [PubMed] [Google Scholar]
- Ellens H., Siegel D. P., Alford D., Yeagle P. L., Boni L., Lis L. J., Quinn P. J., Bentz J. Membrane fusion and inverted phases. Biochemistry. 1989 May 2;28(9):3692–3703. doi: 10.1021/bi00435a011. [DOI] [PubMed] [Google Scholar]
- Frederik P. M., Burger K. N., Stuart M. C., Verkleij A. J. Lipid polymorphism as observed by cryo-electron microscopy. Biochim Biophys Acta. 1991 Feb 25;1062(2):133–141. doi: 10.1016/0005-2736(91)90384-k. [DOI] [PubMed] [Google Scholar]
- Frederik P. M., Stuart M. C., Verkleij A. J. Intermediary structures during membrane fusion as observed by cryo-electron microscopy. Biochim Biophys Acta. 1989 Feb 27;979(2):275–278. doi: 10.1016/0005-2736(89)90445-8. [DOI] [PubMed] [Google Scholar]
- Gagné J., Stamatatos L., Diacovo T., Hui S. W., Yeagle P. L., Silvius J. R. Physical properties and surface interactions of bilayer membranes containing N-methylated phosphatidylethanolamines. Biochemistry. 1985 Jul 30;24(16):4400–4408. doi: 10.1021/bi00337a022. [DOI] [PubMed] [Google Scholar]
- Gruner S. M., Tate M. W., Kirk G. L., So P. T., Turner D. C., Keane D. T., Tilcock C. P., Cullis P. R. X-ray diffraction study of the polymorphic behavior of N-methylated dioleoylphosphatidylethanolamine. Biochemistry. 1988 Apr 19;27(8):2853–2866. doi: 10.1021/bi00408a029. [DOI] [PubMed] [Google Scholar]
- Hui S. W., Stewart T. P., Boni L. T. The nature of lipidic particles and their roles in polymorphic transitions. Chem Phys Lipids. 1983 Aug;33(2):113–126. doi: 10.1016/0009-3084(83)90015-4. [DOI] [PubMed] [Google Scholar]
- Lai M. Z., Vail W. J., Szoka F. C. Acid- and calcium-induced structural changes in phosphatidylethanolamine membranes stabilized by cholesteryl hemisuccinate. Biochemistry. 1985 Mar 26;24(7):1654–1661. doi: 10.1021/bi00328a013. [DOI] [PubMed] [Google Scholar]
- Markin V. S., Kozlov M. M., Borovjagin V. L. On the theory of membrane fusion. The stalk mechanism. Gen Physiol Biophys. 1984 Oct;3(5):361–377. [PubMed] [Google Scholar]
- Siegel D. P., Banschbach J. L. Lamellar/inverted cubic (L alpha/QII) phase transition in N-methylated dioleoylphosphatidylethanolamine. Biochemistry. 1990 Jun 26;29(25):5975–5981. doi: 10.1021/bi00477a014. [DOI] [PubMed] [Google Scholar]
- Siegel D. P., Burns J. L., Chestnut M. H., Talmon Y. Intermediates in membrane fusion and bilayer/nonbilayer phase transitions imaged by time-resolved cryo-transmission electron microscopy. Biophys J. 1989 Jul;56(1):161–169. doi: 10.1016/S0006-3495(89)82661-X. [DOI] [PMC free article] [PubMed] [Google Scholar]
- Siegel D. P. Energetics of intermediates in membrane fusion: comparison of stalk and inverted micellar intermediate mechanisms. Biophys J. 1993 Nov;65(5):2124–2140. doi: 10.1016/S0006-3495(93)81256-6. [DOI] [PMC free article] [PubMed] [Google Scholar]
- Siegel D. P. Inverted micellar intermediates and the transitions between lamellar, cubic, and inverted hexagonal amphiphile phases. III. Isotropic and inverted cubic state formation via intermediates in transitions between L alpha and HII phases. Chem Phys Lipids. 1986 Dec 31;42(4):279–301. doi: 10.1016/0009-3084(86)90087-3. [DOI] [PubMed] [Google Scholar]
- Siegel D. P. Inverted micellar intermediates and the transitions between lamellar, cubic, and inverted hexagonal lipid phases. I. Mechanism of the L alpha----HII phase transitions. Biophys J. 1986 Jun;49(6):1155–1170. doi: 10.1016/S0006-3495(86)83744-4. [DOI] [PMC free article] [PubMed] [Google Scholar]
- Siegel D. P. Inverted micellar intermediates and the transitions between lamellar, cubic, and inverted hexagonal lipid phases. II. Implications for membrane-membrane interactions and membrane fusion. Biophys J. 1986 Jun;49(6):1171–1183. doi: 10.1016/S0006-3495(86)83745-6. [DOI] [PMC free article] [PubMed] [Google Scholar]
- Siegel D. P. Inverted micellar structures in bilayer membranes. Formation rates and half-lives. Biophys J. 1984 Feb;45(2):399–420. doi: 10.1016/S0006-3495(84)84164-8. [DOI] [PMC free article] [PubMed] [Google Scholar]
- Talmon Y., Burns J. L., Chestnut M. H., Siegel D. P. Time-resolved cryotransmission electron microscopy. J Electron Microsc Tech. 1990 Jan;14(1):6–12. doi: 10.1002/jemt.1060140103. [DOI] [PubMed] [Google Scholar]
- Tate M. W., Shyamsunder E., Gruner S. M., D'Amico K. L. Kinetics of the lamellar-inverse hexagonal phase transition determined by time-resolved X-ray diffraction. Biochemistry. 1992 Feb 4;31(4):1081–1092. doi: 10.1021/bi00119a017. [DOI] [PubMed] [Google Scholar]
- Van Venetie R., Verkleij A. J. Analysis of the hexagonal II phase and its relations to lipidic particles and the lamellar phase. A freeze-fracture study. Biochim Biophys Acta. 1981 Jul 20;645(2):262–269. doi: 10.1016/0005-2736(81)90197-8. [DOI] [PubMed] [Google Scholar]
- Verkleij A. J. Lipidic intramembranous particles. Biochim Biophys Acta. 1984 Jan 27;779(1):43–63. doi: 10.1016/0304-4157(84)90003-0. [DOI] [PubMed] [Google Scholar]
- Verkleij A. J., van Echteld C. J., Gerritsen W. J., Cullis P. R., de Kruijff B. The lipidic particle as an intermediate structure in membrane fusion processes and bilayer to hexagonal HII transitions. Biochim Biophys Acta. 1980 Aug 14;600(3):620–624. doi: 10.1016/0005-2736(80)90465-4. [DOI] [PubMed] [Google Scholar]
- Walter A., Gutknecht J. Monocarboxylic acid permeation through lipid bilayer membranes. J Membr Biol. 1984;77(3):255–264. doi: 10.1007/BF01870573. [DOI] [PubMed] [Google Scholar]
- White J. M. Membrane fusion. Science. 1992 Nov 6;258(5084):917–924. doi: 10.1126/science.1439803. [DOI] [PubMed] [Google Scholar]