Abstract
The kinetics of excitation energy transfer and electron transfer processes within the membrane of Heliobacillus mobilis were investigated using femtosecond transient absorption difference spectroscopy at room temperature. The kinetics in the 725- to 865-nm region, upon excitation at 590 and 670 nm, were fit using global analysis. The fits returned three kinetic components with lifetimes of 1-2 ps and 27-30 ps, and a component that does not decay within several nanoseconds. The 1- to 2-ps component is attributed to excitation equilibration to form a thermally relaxed excited state. The 27- to 30-ps phase corresponds to the decay of the relaxed excited state to form a charge-separated state. The intrinsic energy and electron transfer rates were estimated using the experimental results and theoretical models for excitation migration and trapping dynamics. Taking into account the number of antenna pigments and their spectral distribution, an upper limit of 1.2 ps for the intrinsic time constant for charge separation in the reaction center is calculated. This upper limit corresponds with the trapping-limited case for excitation migration and trapping. Reduction of the primary electron acceptor A0 was observed in the 640 to 700 nm region using excitation at 780 nm. An instantaneous absorbance increase followed by a decay of about 30 ps was observed over a broad wavelength region due to the excited state absorption and decay of BChl g molecules in the antenna. In addition, a narrow bleaching band centered at 670 nm grows in with an apparent time constant of about 1.0 ps, superimposed on the 30-ps absorbance increase due to excited state absorption. Measurements on a longer time scale showed that besides the 670 nm pigment a BChl g molecule absorbing near 785 nm may be involved in the primary charge separation, and that this pigment may be in equilibrium with the 670 nm pigment. The bleaching bands at 670 nm and 785nm recovered with a time constant of about 600 ps, due to forward electron transport to a secondary electron acceptor. Energy and electron transfer properties of H. mobilis membranes are compared with Photosystem 1, to which the heliobacteria bear an evolutionary relationship.
Full text
PDF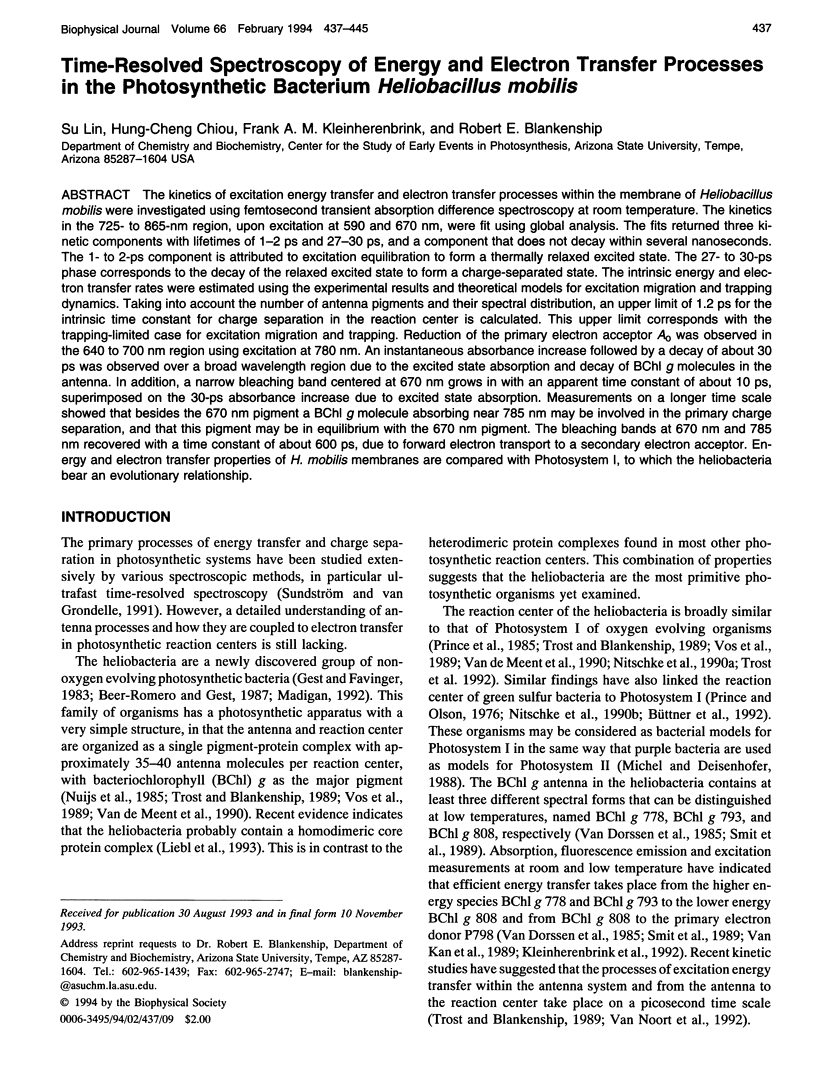
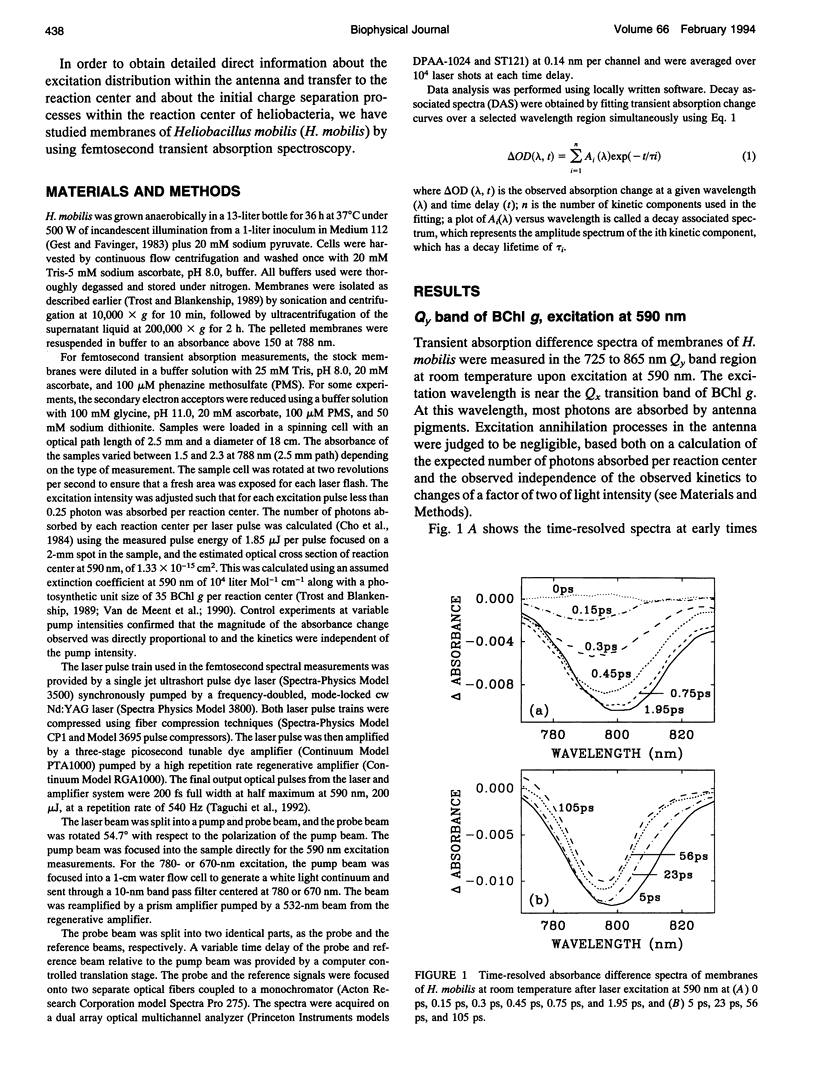
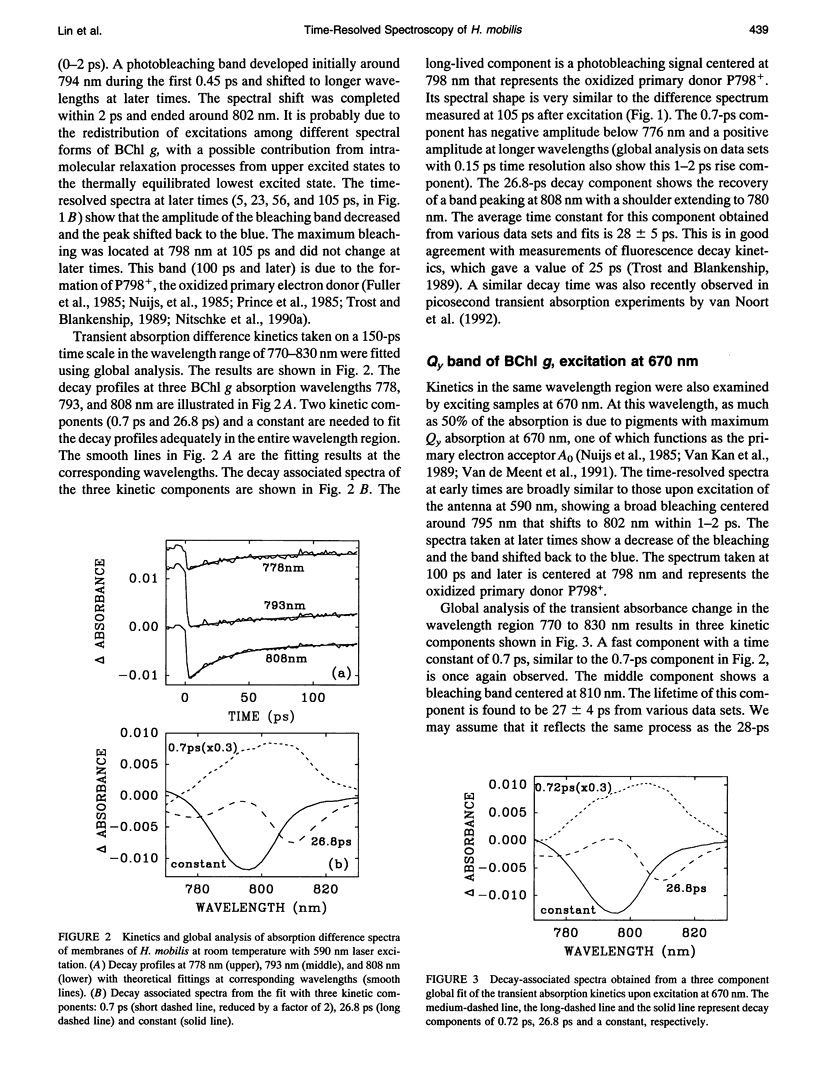
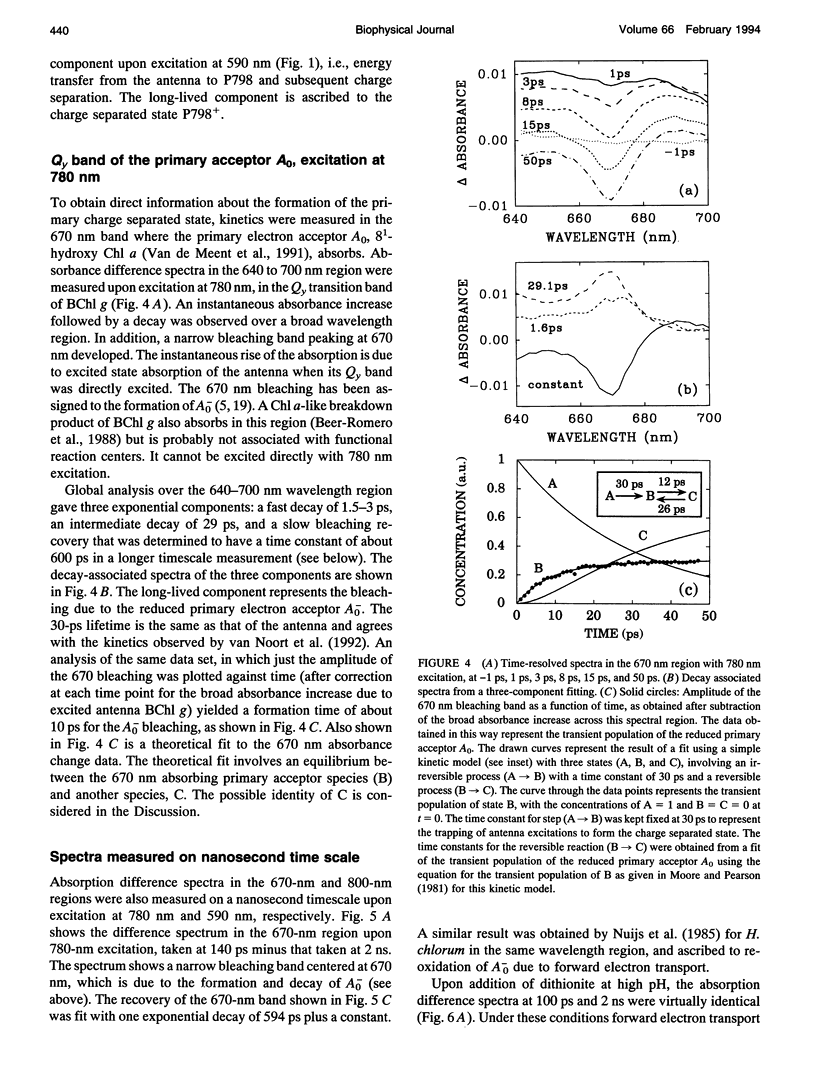
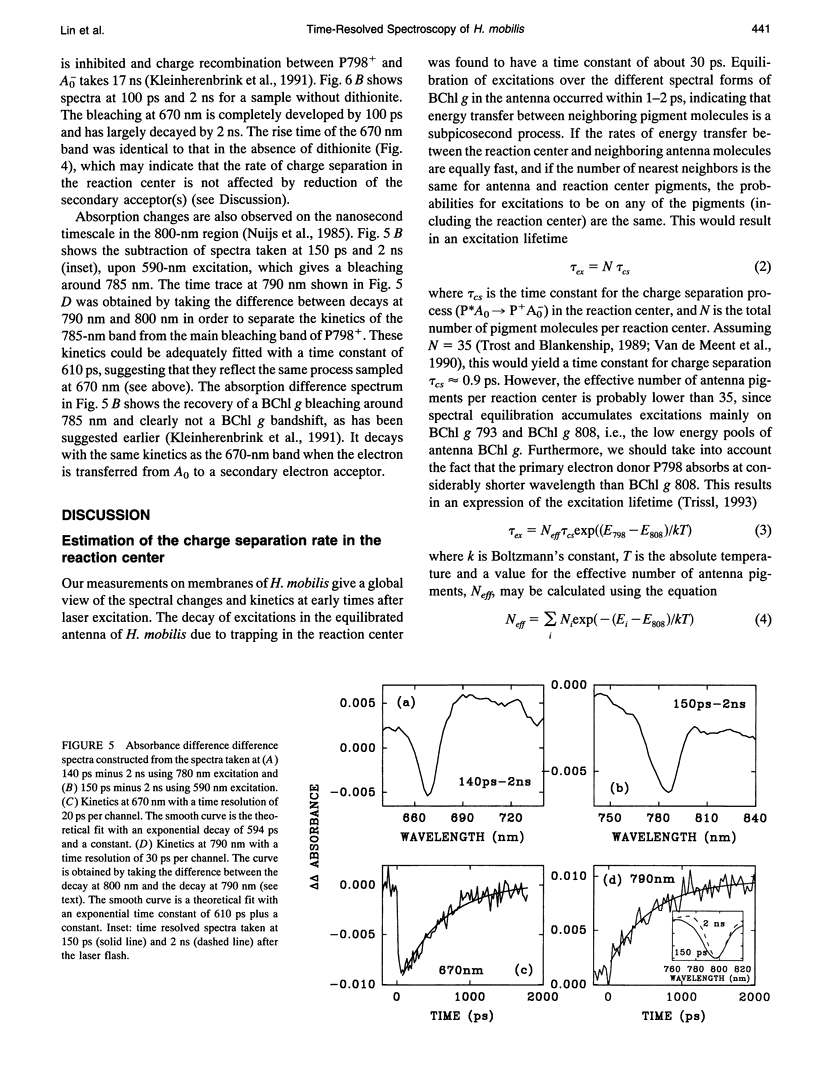
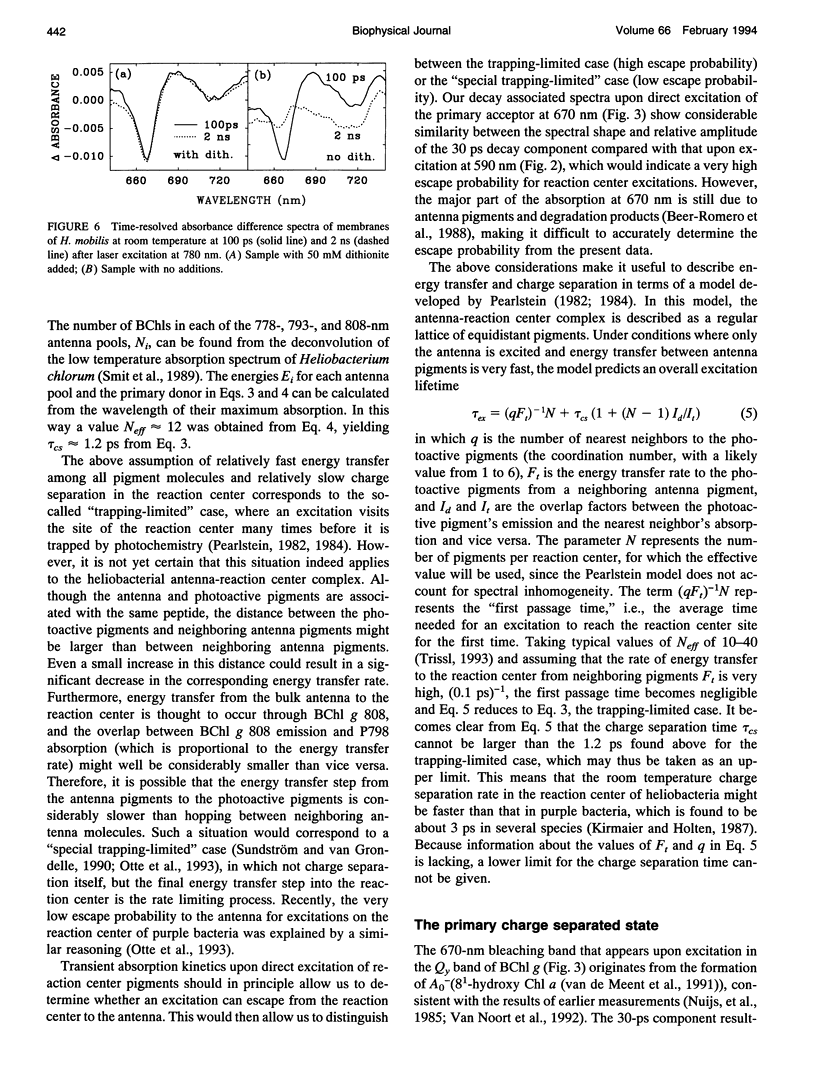
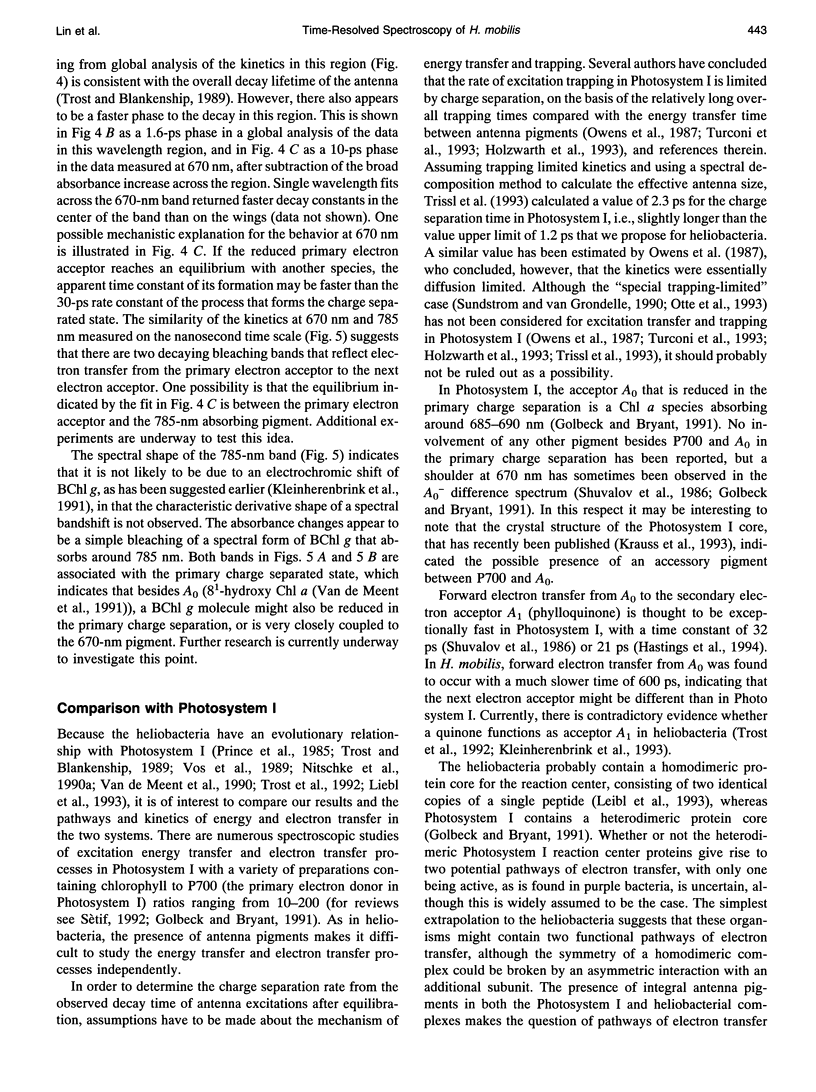
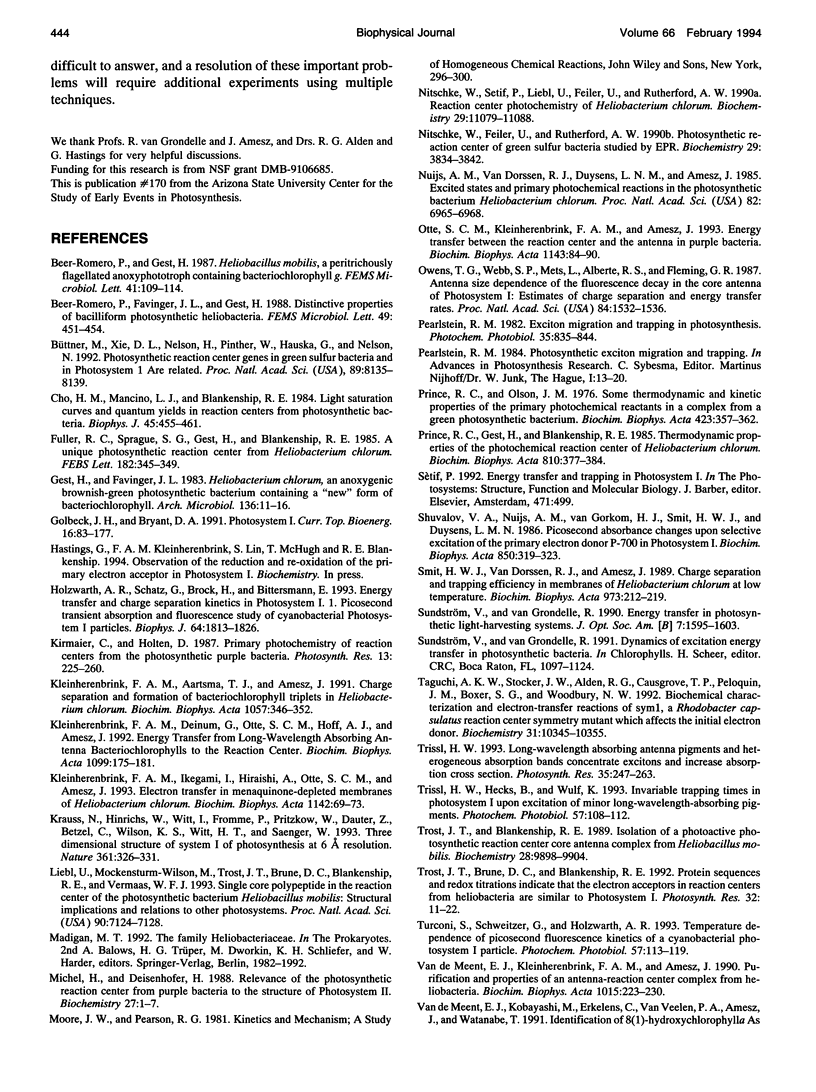
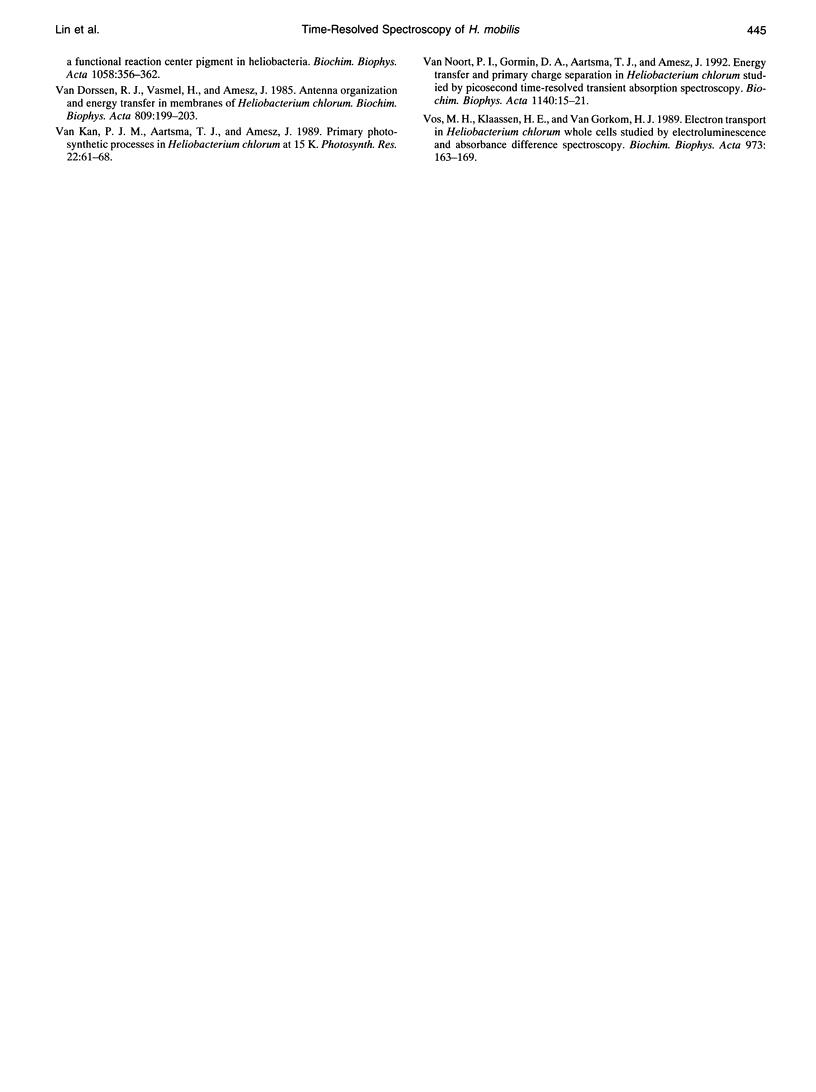
Selected References
These references are in PubMed. This may not be the complete list of references from this article.
- Büttner M., Xie D. L., Nelson H., Pinther W., Hauska G., Nelson N. Photosynthetic reaction center genes in green sulfur bacteria and in photosystem 1 are related. Proc Natl Acad Sci U S A. 1992 Sep 1;89(17):8135–8139. doi: 10.1073/pnas.89.17.8135. [DOI] [PMC free article] [PubMed] [Google Scholar]
- Cho H. M., Mancino L. J., Blankenship R. E. Light saturation curves and quantum yields in reaction centers from photosynthetic bacteria. Biophys J. 1984 Feb;45(2):455–461. doi: 10.1016/S0006-3495(84)84168-5. [DOI] [PMC free article] [PubMed] [Google Scholar]
- Holzwarth A. R., Schatz G., Brock H., Bittersmann E. Energy transfer and charge separation kinetics in photosystem I: Part 1: Picosecond transient absorption and fluorescence study of cyanobacterial photosystem I particles. Biophys J. 1993 Jun;64(6):1813–1826. doi: 10.1016/S0006-3495(93)81552-2. [DOI] [PMC free article] [PubMed] [Google Scholar]
- Liebl U., Mockensturm-Wilson M., Trost J. T., Brune D. C., Blankenship R. E., Vermaas W. Single core polypeptide in the reaction center of the photosynthetic bacterium Heliobacillus mobilis: structural implications and relations to other photosystems. Proc Natl Acad Sci U S A. 1993 Aug 1;90(15):7124–7128. doi: 10.1073/pnas.90.15.7124. [DOI] [PMC free article] [PubMed] [Google Scholar]
- Michelson A. M., Blake C. C., Evans S. T., Orkin S. H. Structure of the human phosphoglycerate kinase gene and the intron-mediated evolution and dispersal of the nucleotide-binding domain. Proc Natl Acad Sci U S A. 1985 Oct;82(20):6965–6969. doi: 10.1073/pnas.82.20.6965. [DOI] [PMC free article] [PubMed] [Google Scholar]
- Nitschke W., Feiler U., Rutherford A. W. Photosynthetic reaction center of green sulfur bacteria studied by EPR. Biochemistry. 1990 Apr 24;29(16):3834–3842. doi: 10.1021/bi00468a005. [DOI] [PubMed] [Google Scholar]
- Nitschke W., Sétif P., Liebl U., Feiler U., Rutherford A. W. Reaction center photochemistry of Heliobacterium chlorum. Biochemistry. 1990 Dec 18;29(50):11079–11088. doi: 10.1021/bi00502a010. [DOI] [PubMed] [Google Scholar]
- Owens T. G., Webb S. P., Mets L., Alberte R. S., Fleming G. R. Antenna size dependence of fluorescence decay in the core antenna of photosystem I: estimates of charge separation and energy transfer rates. Proc Natl Acad Sci U S A. 1987 Mar;84(6):1532–1536. doi: 10.1073/pnas.84.6.1532. [DOI] [PMC free article] [PubMed] [Google Scholar]
- Prince R. C., Olson J. M. Some thermodynamic and kinetic properties of the primary photochemical reactants in a complex from a green photosynthetic bacterium. Biochim Biophys Acta. 1976 Feb 16;423(2):357–362. doi: 10.1016/0005-2728(76)90191-2. [DOI] [PubMed] [Google Scholar]
- Taguchi A. K., Stocker J. W., Alden R. G., Causgrove T. P., Peloquin J. M., Boxer S. G., Woodbury N. W. Biochemical characterization and electron-transfer reactions of sym1, a Rhodobacter capsulatus reaction center symmetry mutant which affects the initial electron donor. Biochemistry. 1992 Oct 27;31(42):10345–10355. doi: 10.1021/bi00157a024. [DOI] [PubMed] [Google Scholar]
- Trost J. T., Blankenship R. E. Isolation of a photoactive photosynthetic reaction center-core antenna complex from Heliobacillus mobilis. Biochemistry. 1989 Dec 26;28(26):9898–9904. doi: 10.1021/bi00452a003. [DOI] [PubMed] [Google Scholar]
- Trost J. T., Brune D. C., Blankenship R. E. Protein sequences and redox titrations indicate that the electron acceptors in reaction centers from heliobacteria are similar to Photosystem I. Photosynth Res. 1992;32:11–22. [PubMed] [Google Scholar]