Abstract
In a previous publication (Shaklai et al., 1977a) the present author developed a theory for evaluating proximity relations and surface densities sigma in biological membranes by measurements of excitation energy transfer from a donor attached to a specific site of a membrane protein and an acceptor attached to a specific carbon on a membrane lipid. It was assumed that the protein and lipid are randomly distributed in the plane of the membrane and that the donor and acceptor groups are confined to different planes in the membrane separated by a distance Rp. In this article several aspects of the theory presented in the previous paper are clarified, especially noting that the previous theoretical expressions for the time-dependent and steady state fluorescence intensities assumed that the labeled protein molecule is cylindrically symmetric with the symmetry axis perpendicular to the plane of the membrane and that the donor is positioned on the symmetry axis of the protein. This assumption is also implicitly or explicitly made in subsequent formulations by other investigators. In this article we generalize the theory to include the case where the donor is not on the symmetry axis of the labeled protein. Equations for calculating the time-dependent and steady state fluorescence intensities for this more general case are presented, and methods for applying these theoretical expressions to the analysis of steady state fluorescence intensity data and evaluation of proximity parameters are discussed. It is also shown in this article that the linear relation l/lo = 1 + Kq sigma previously derived for simple analysis of excitation transfer data for the condition rc/Ro 1 can be modified to apply to almost all practical ranges of rc/Ro without much affecting its simplicity in the analysis of experimental data.
Full text
PDF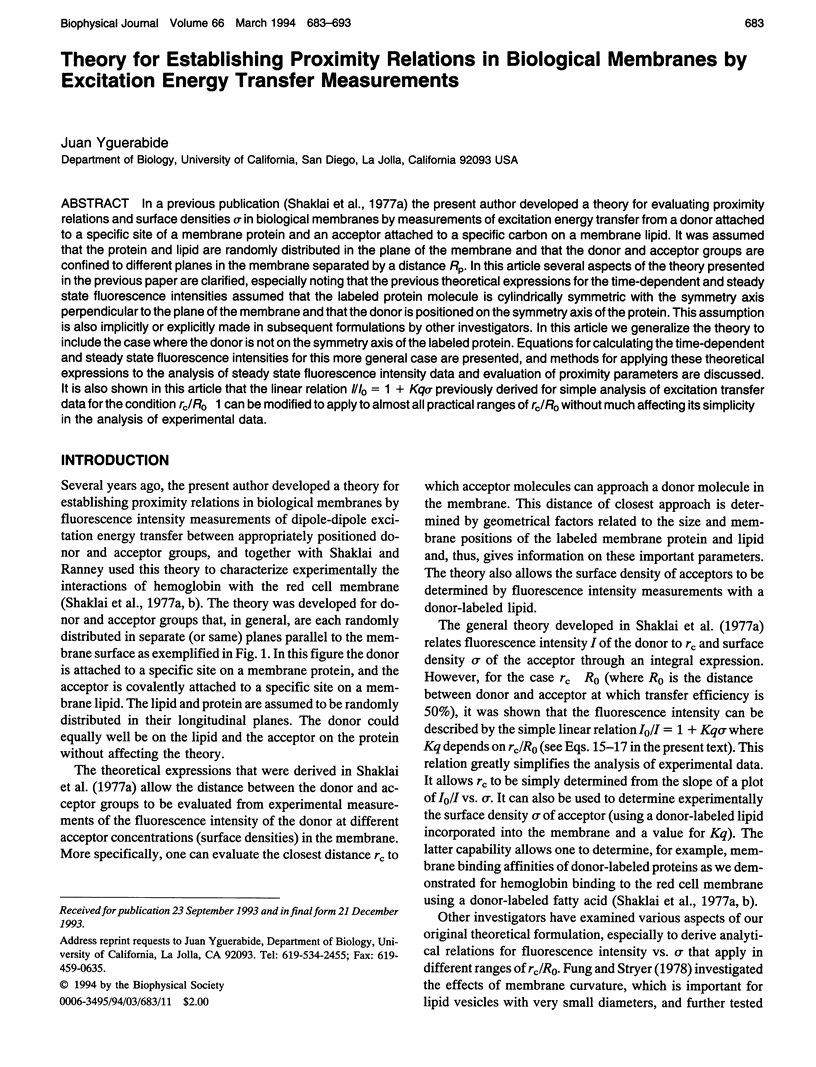
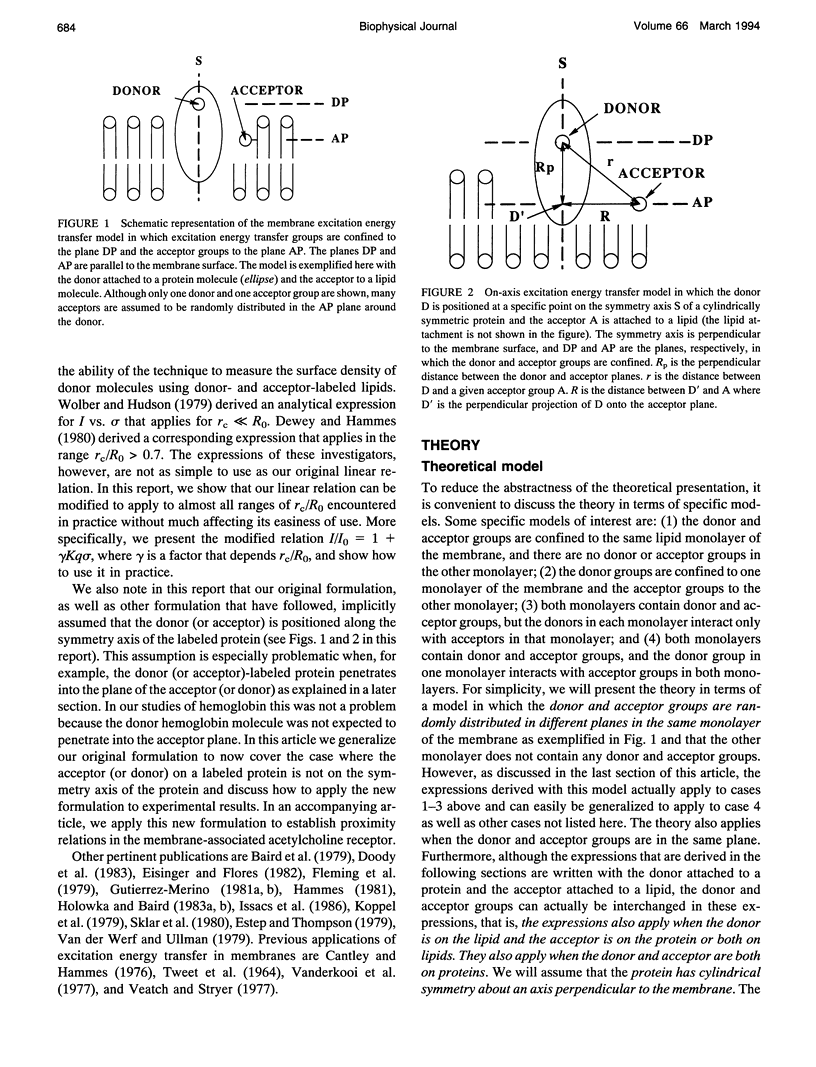
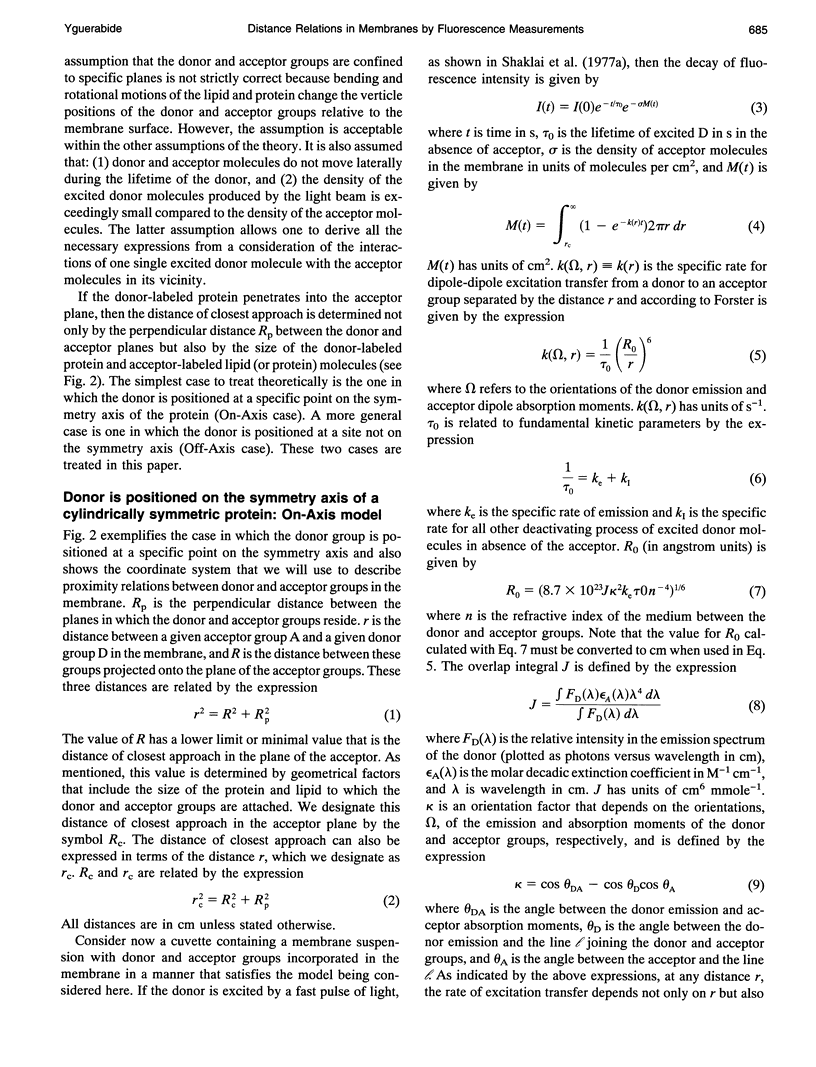
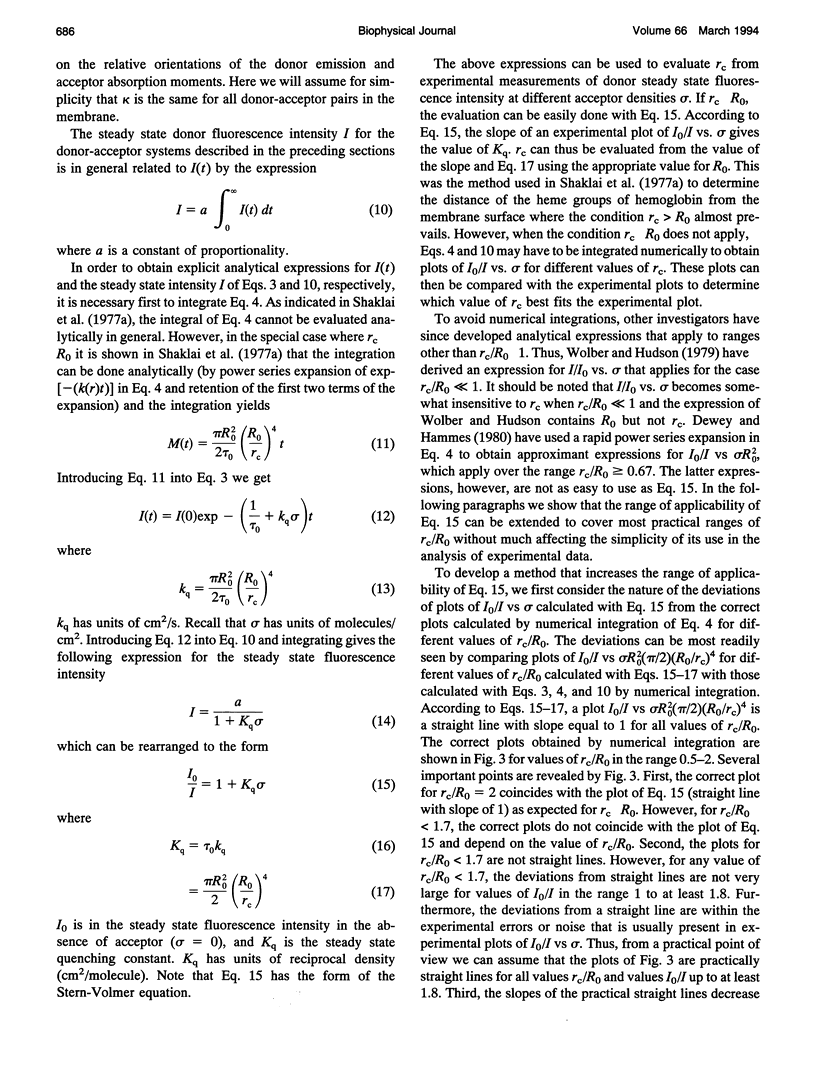
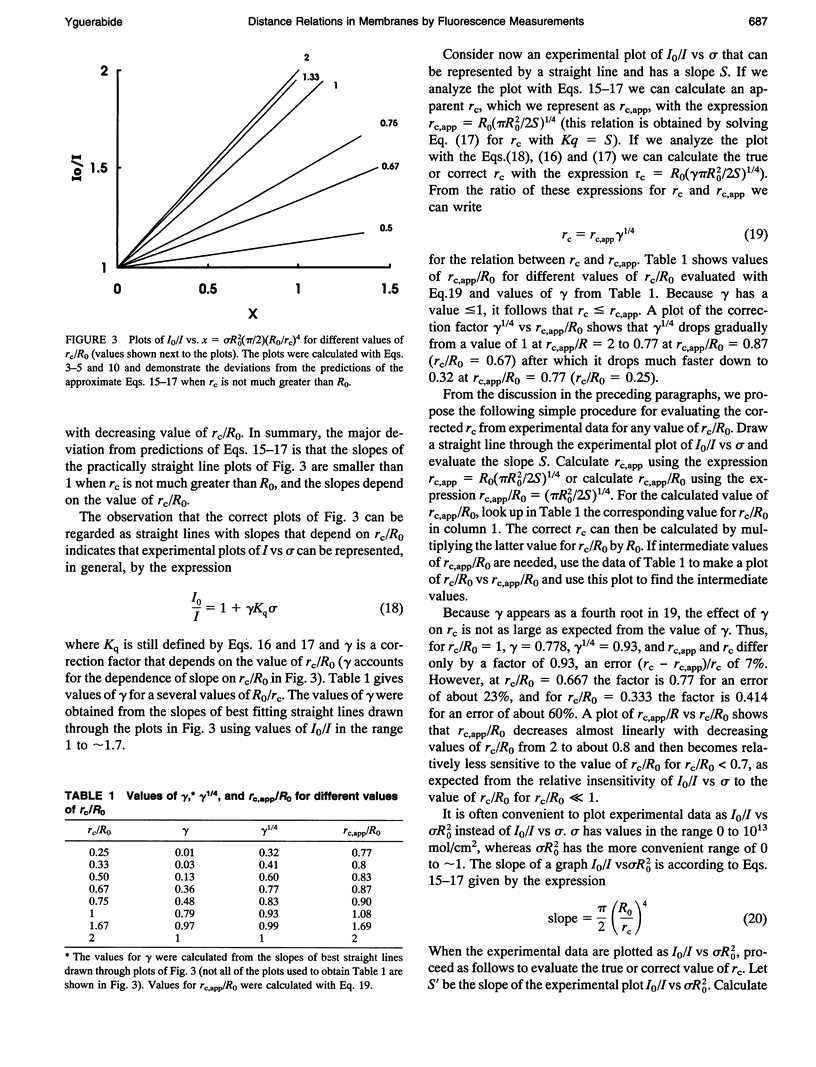
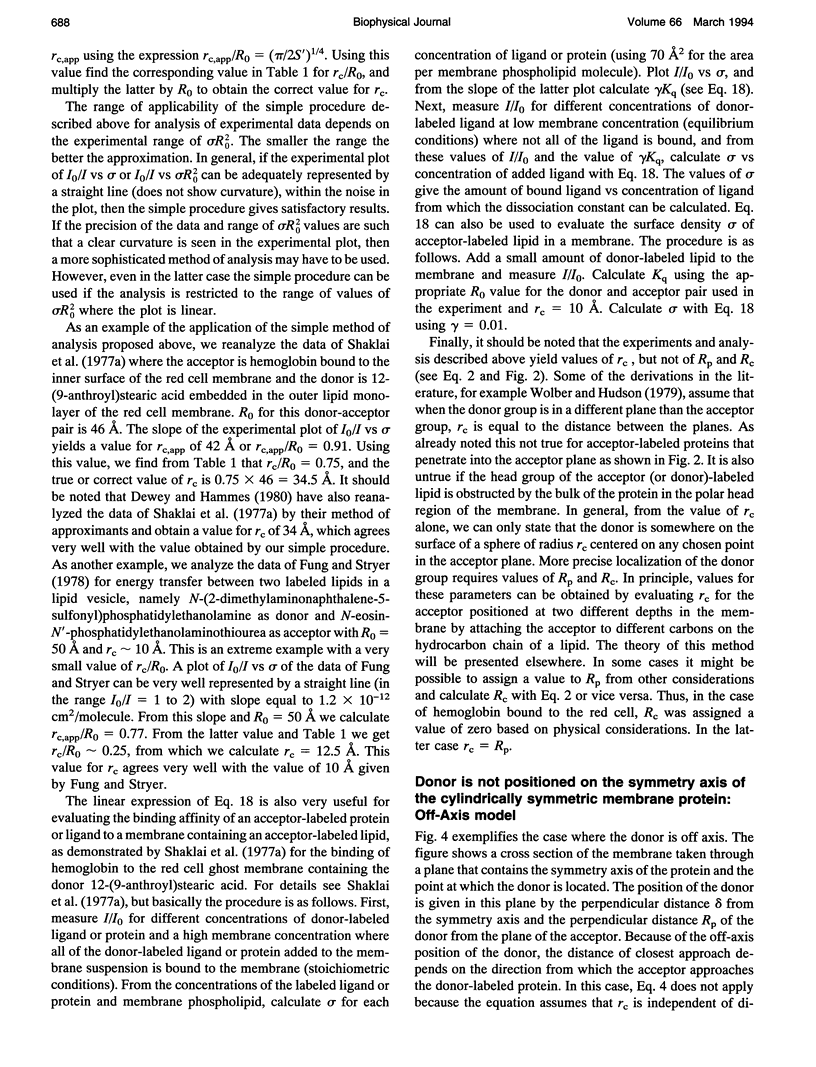
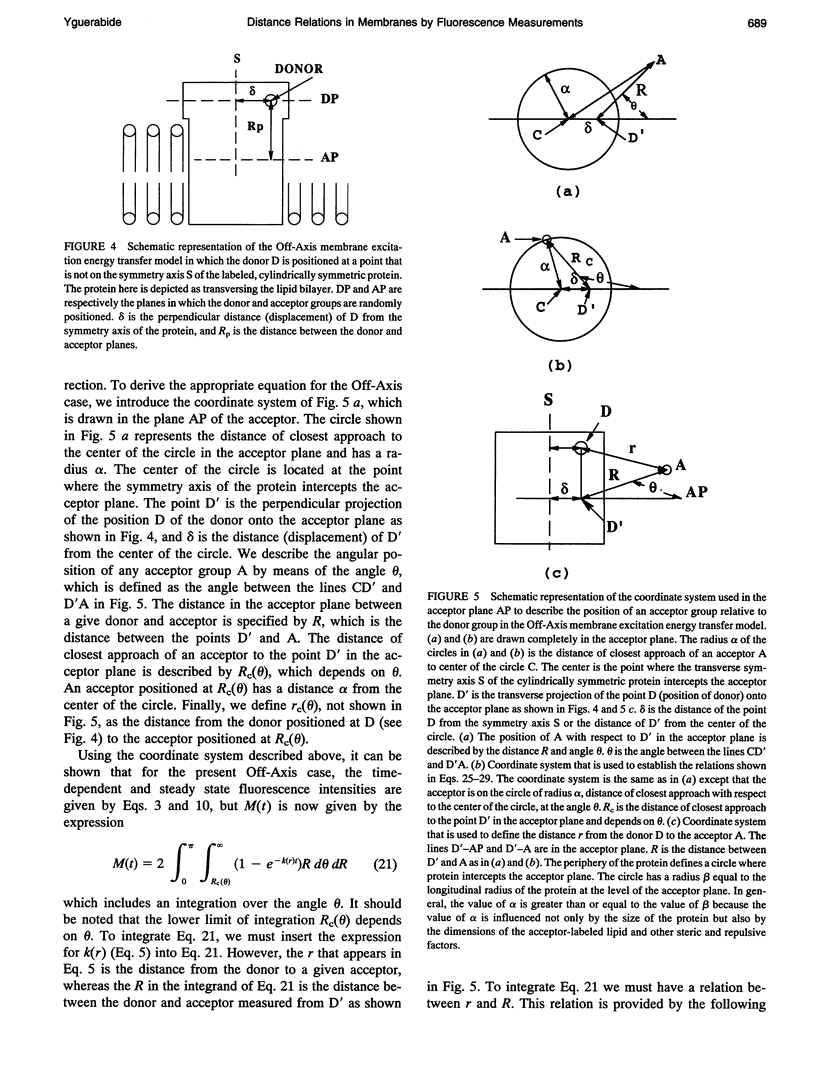
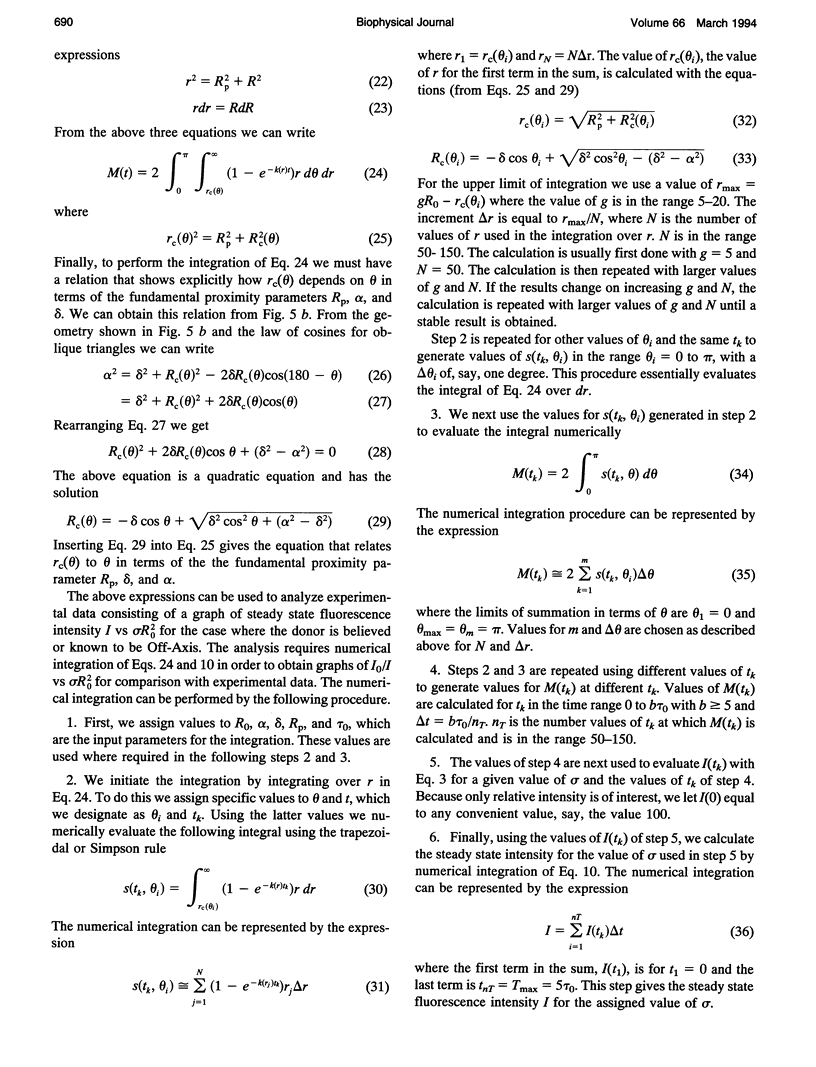
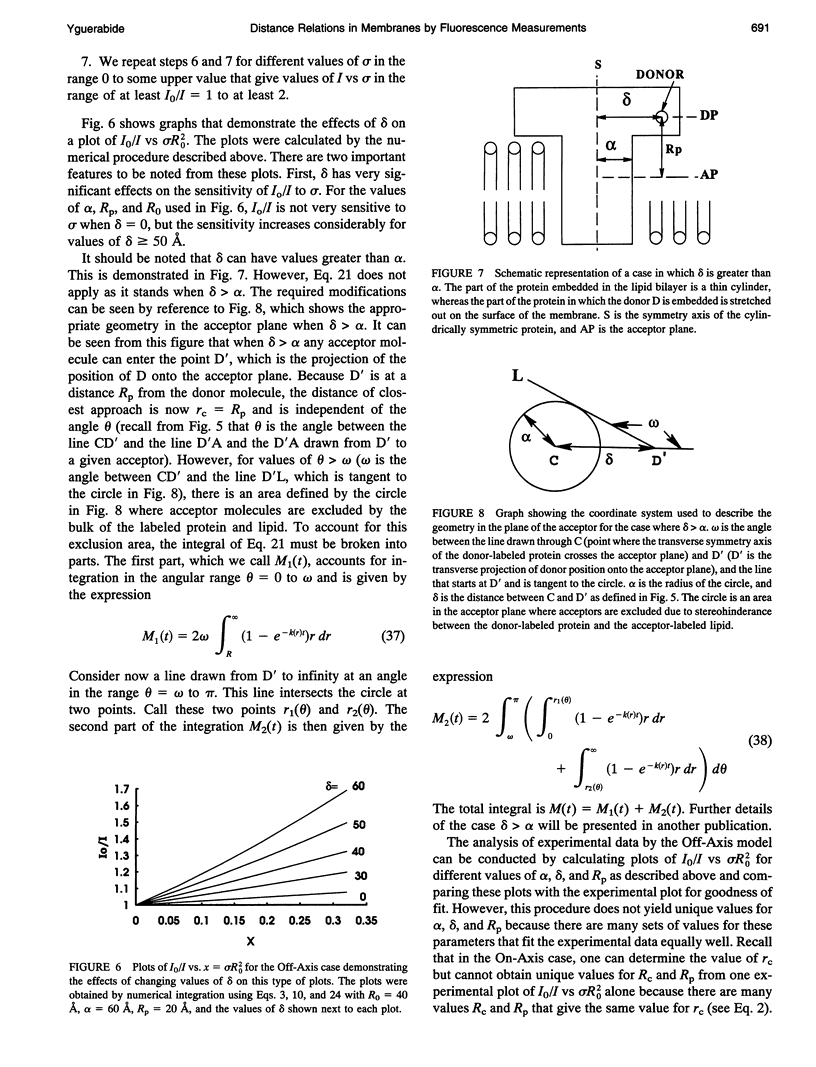
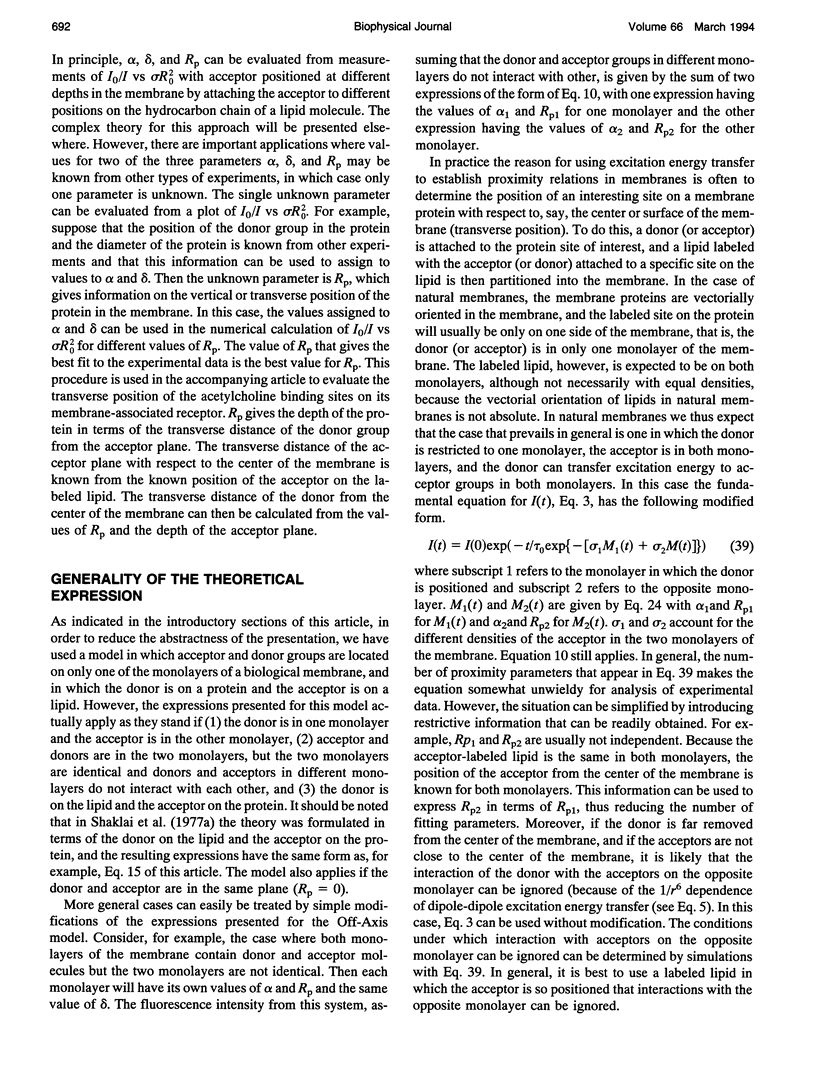
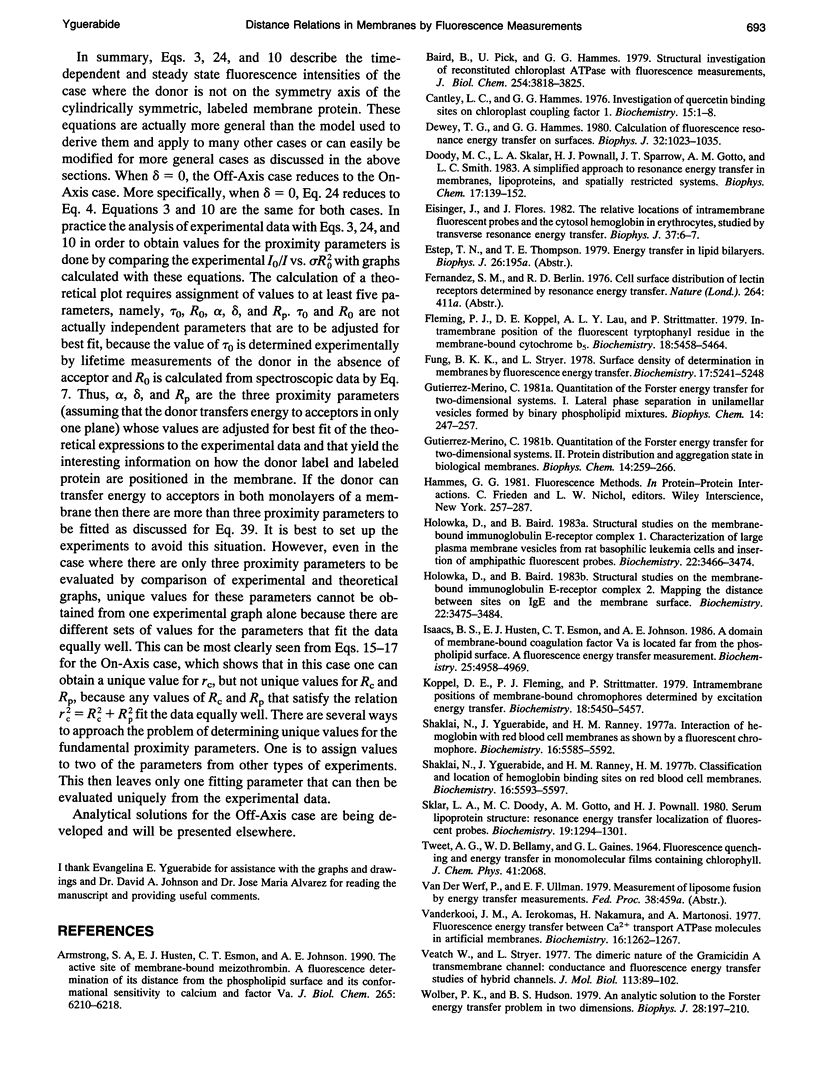
Selected References
These references are in PubMed. This may not be the complete list of references from this article.
- Armstrong S. A., Husten E. J., Esmon C. T., Johnson A. E. The active site of membrane-bound meizothrombin. A fluorescence determination of its distance from the phospholipid surface and its conformational sensitivity to calcium and factor Va. J Biol Chem. 1990 Apr 15;265(11):6210–6218. [PubMed] [Google Scholar]
- Baird B. A., Pick U., Hammes G. G. Structural investigation of reconstituted chloroplast ATPase with fluorescence measurements. J Biol Chem. 1979 May 25;254(10):3818–3825. [PubMed] [Google Scholar]
- Cantley L. C., Jr, Hammes G. G. Investigation of quercetin binding sites on chloroplast coupling factor 1. Biochemistry. 1976 Jan 13;15(1):1–8. doi: 10.1021/bi00646a001. [DOI] [PubMed] [Google Scholar]
- Dewey T. G., Hammes G. G. Calculation on fluorescence resonance energy transfer on surfaces. Biophys J. 1980 Dec;32(3):1023–1035. doi: 10.1016/S0006-3495(80)85033-8. [DOI] [PMC free article] [PubMed] [Google Scholar]
- Doody M. C., Sklar L. A., Pownall H. J., Sparrow J. T., Gotto A. M., Jr, Smith L. C. A simplified approach to resonance energy transfer in membranes, lipoproteins and spatially restricted systems. Biophys Chem. 1983 Mar;17(2):139–152. doi: 10.1016/0301-4622(83)80008-8. [DOI] [PubMed] [Google Scholar]
- Eisinger J., Flores J. The relative locations of intramembrane fluorescent probes and of the cytosol hemoglobin in erythrocytes, studied by transverse resonance energy transfer. Biophys J. 1982 Jan;37(1):6–7. doi: 10.1016/S0006-3495(82)84571-2. [DOI] [PMC free article] [PubMed] [Google Scholar]
- Fleming P. J., Koppel D. E., Lau A. L., Strittmatter P. Intramembrane position of the fluorescent tryptophanyl residue in membrane-bound cytochrome b5. Biochemistry. 1979 Nov 27;18(24):5458–5464. doi: 10.1021/bi00591a031. [DOI] [PubMed] [Google Scholar]
- Fung B. K., Stryer L. Surface density determination in membranes by fluorescence energy transfer. Biochemistry. 1978 Nov 28;17(24):5241–5248. doi: 10.1021/bi00617a025. [DOI] [PubMed] [Google Scholar]
- Gutierrez-Merino C. Quantitation of the Förster energy transfer for two-dimensional systems. I. Lateral phase separation in unilamellar vesicles formed by binary phospholipid mixtures. Biophys Chem. 1981 Nov;14(3):247–257. doi: 10.1016/0301-4622(81)85025-9. [DOI] [PubMed] [Google Scholar]
- Gutierrez-Merino C. Quantitation of the Förster energy transfer for two-dimensional systems. II. Protein distribution and aggregation state in biological membranes. Biophys Chem. 1981 Nov;14(3):259–266. doi: 10.1016/0301-4622(81)85026-0. [DOI] [PubMed] [Google Scholar]
- Holowka D., Baird B. Structural studies on the membrane-bound immunoglobulin E-receptor complex. 1. Characterization of large plasma membrane vesicles from rat basophilic leukemia cells and insertion of amphipathic fluorescent probes. Biochemistry. 1983 Jul 5;22(14):3466–3474. doi: 10.1021/bi00283a025. [DOI] [PubMed] [Google Scholar]
- Isaacs B. S., Husten E. J., Esmon C. T., Johnson A. E. A domain of membrane-bound blood coagulation factor Va is located far from the phospholipid surface. A fluorescence energy transfer measurement. Biochemistry. 1986 Aug 26;25(17):4958–4969. doi: 10.1021/bi00365a036. [DOI] [PubMed] [Google Scholar]
- Koppel D. E., Fleming P. J., Strittmatter P. Intramembrane positions of membrane-bound chromophores determined by excitation energy transfer. Biochemistry. 1979 Nov 27;18(24):5450–5457. doi: 10.1021/bi00591a030. [DOI] [PubMed] [Google Scholar]
- Shaklai N., Yguerabide J., Ranney H. M. Classification and localization of hemoglobin binding sites on the red blood cell membrane. Biochemistry. 1977 Dec 13;16(25):5593–5597. doi: 10.1021/bi00644a032. [DOI] [PubMed] [Google Scholar]
- Shaklai N., Yguerabide J., Ranney H. M. Interaction of hemoglobin with red blood cell membranes as shown by a fluorescent chromophore. Biochemistry. 1977 Dec 13;16(25):5585–5592. doi: 10.1021/bi00644a031. [DOI] [PubMed] [Google Scholar]
- Sklar L. A., Doody M. C., Gotto A. M., Jr, Pownall H. J. Serum lipoprotein structure: resonance energy transfer localization of fluorescent lipid probes. Biochemistry. 1980 Apr 1;19(7):1294–1301. doi: 10.1021/bi00548a005. [DOI] [PubMed] [Google Scholar]
- Vanderkooi J. M., Ierokomas A., Nakamura H., Martonosi A. Fluorescence energy transfer between Ca2+ transport ATPase molecules in artificial membranes. Biochemistry. 1977 Apr 5;16(7):1262–1267. doi: 10.1021/bi00626a003. [DOI] [PubMed] [Google Scholar]
- Veatch W., Stryer L. The dimeric nature of the gramicidin A transmembrane channel: conductance and fluorescence energy transfer studies of hybrid channels. J Mol Biol. 1977 Jun 15;113(1):89–102. doi: 10.1016/0022-2836(77)90042-0. [DOI] [PubMed] [Google Scholar]
- Wolber P. K., Hudson B. S. An analytic solution to the Förster energy transfer problem in two dimensions. Biophys J. 1979 Nov;28(2):197–210. doi: 10.1016/S0006-3495(79)85171-1. [DOI] [PMC free article] [PubMed] [Google Scholar]