Abstract
We describe a model that relates the maximum shortening velocity of a muscle fiber, Vm, to the kinetics of the dissociation of a myosin head from actin. At Vm, the positive work exerted by cross-bridges attached in the powerstroke must be balanced by cross-bridges that have been carried by movement of the filaments into a region where they exert a negative force. This balance allows one to relate Vm and the rate of cross-bridge detachment. Studies of actomyosin kinetics suggest that at high substrate, detachment should be limited by a slow protein isomerization (approximately 50 s-1) that precedes ADP release. This rate is too slow to be easily accommodated in existing models. However, a slow rate for cross-bridge dissociation, similar to that of the isomerization, is predicted if previous models are modified to include rapid detachment of cross-bridges that have been carried so far into the negative force region that their free energy exceeds that of the detached state. The model also explains another aspect of muscle contraction: at high shortening velocities, the observed rate of ATP hydrolysis is low, because a cross-bridge can interact with multiple actin binding sites before releasing the hydrolysis products and binding another ATP.
Full text
PDF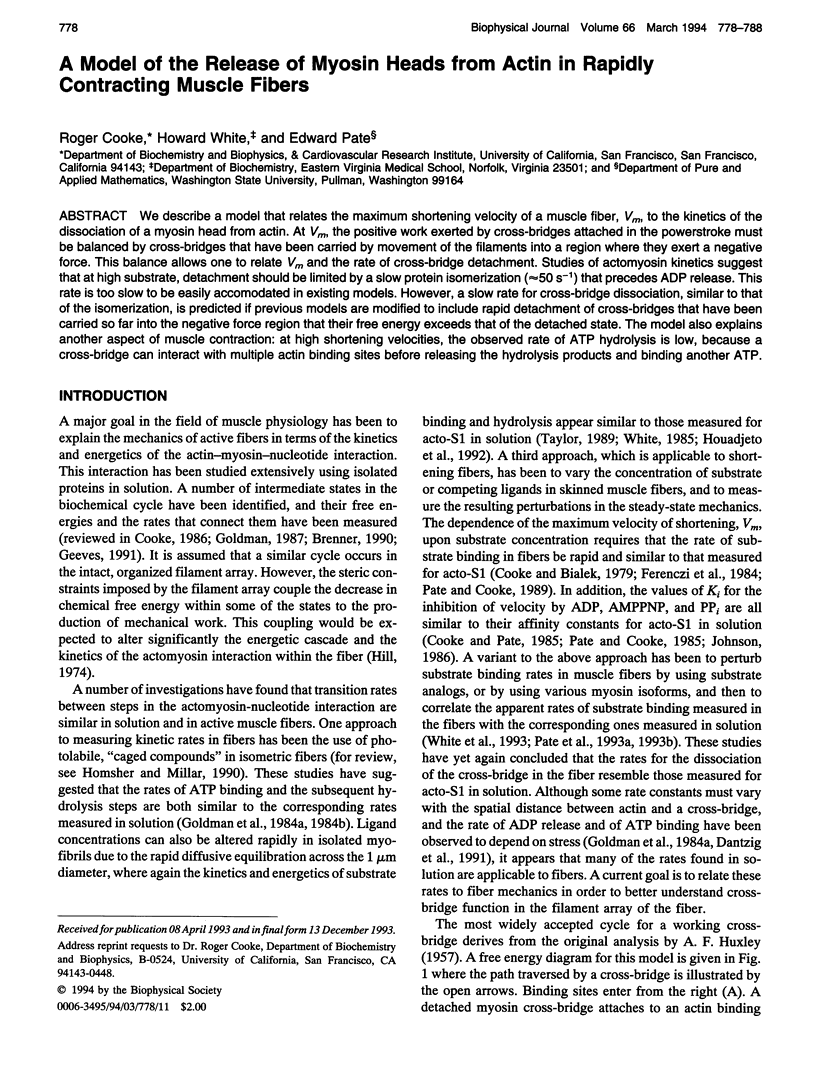
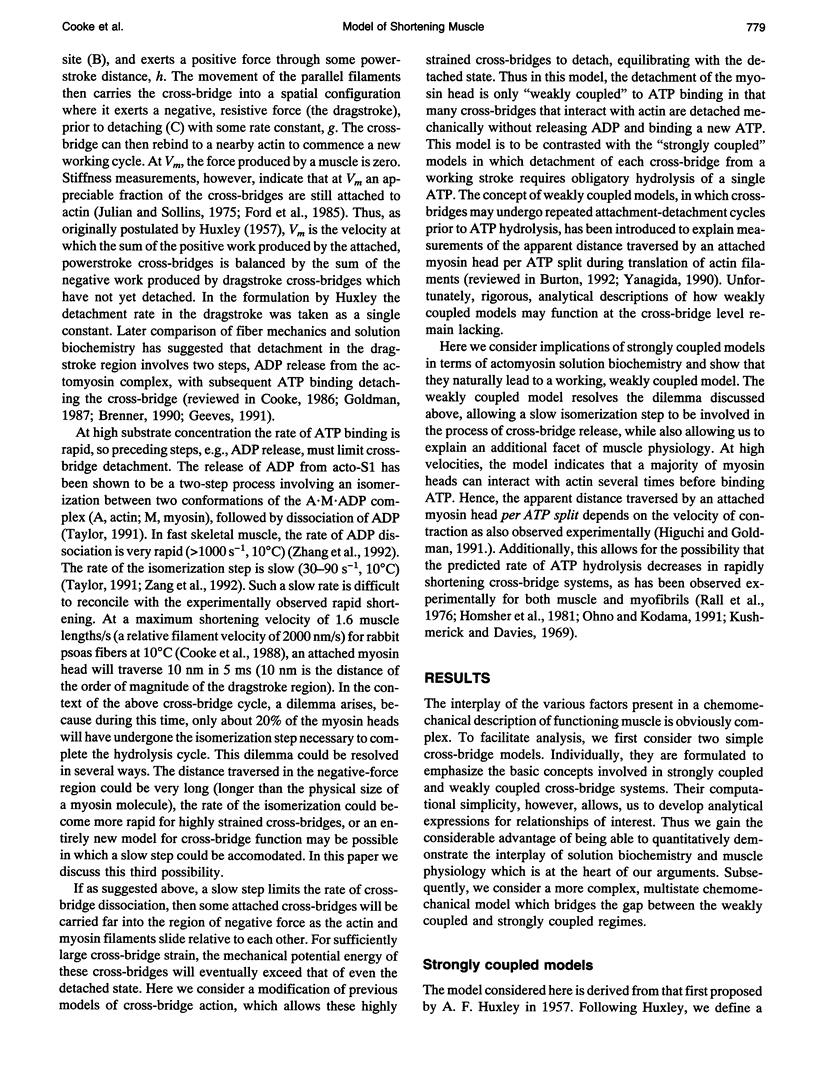
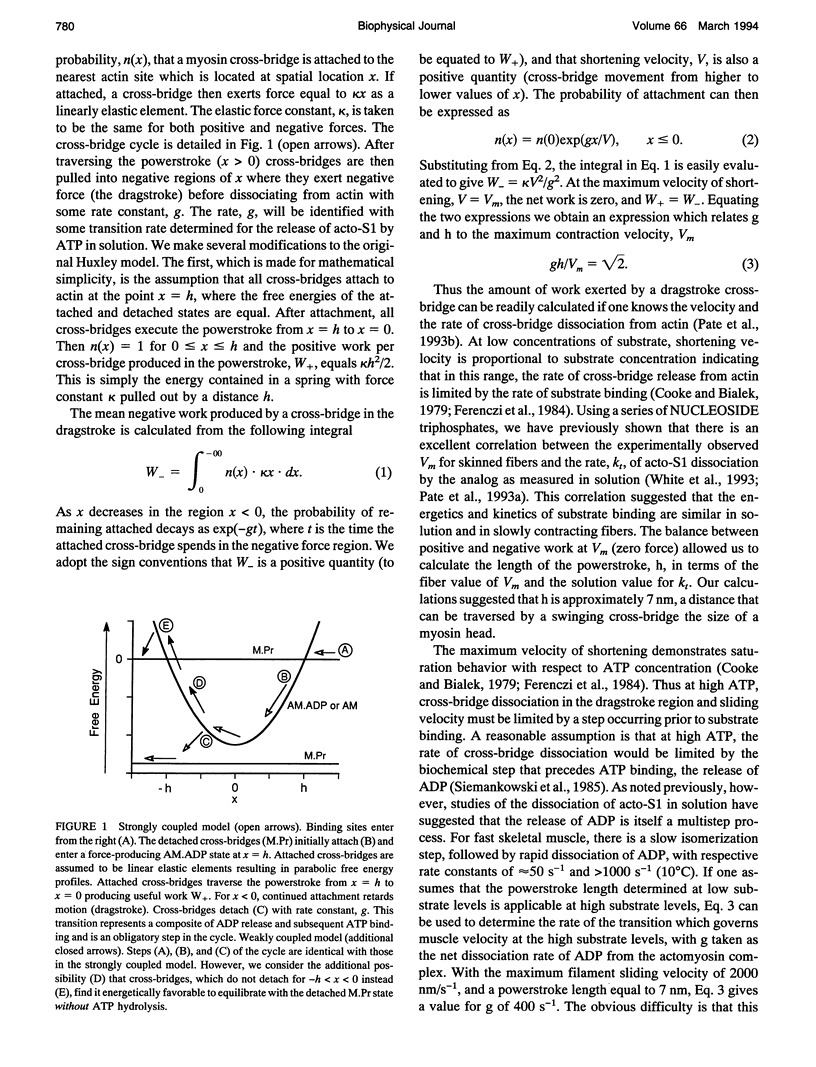
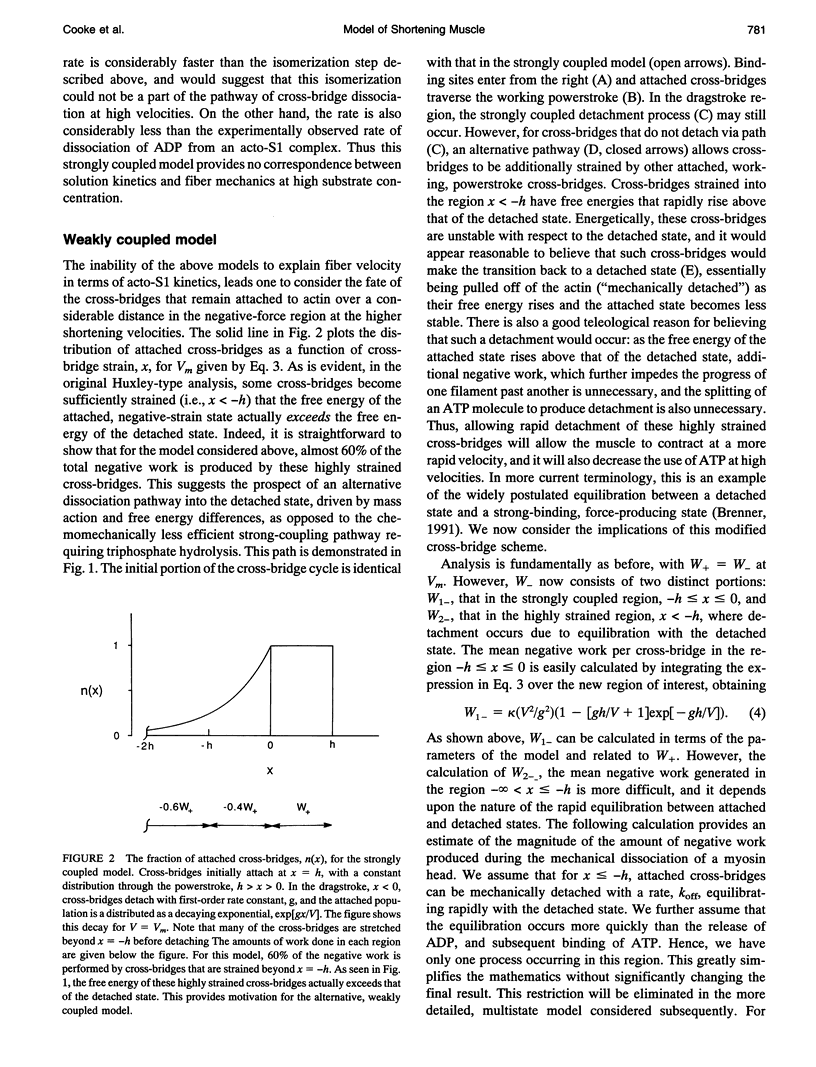
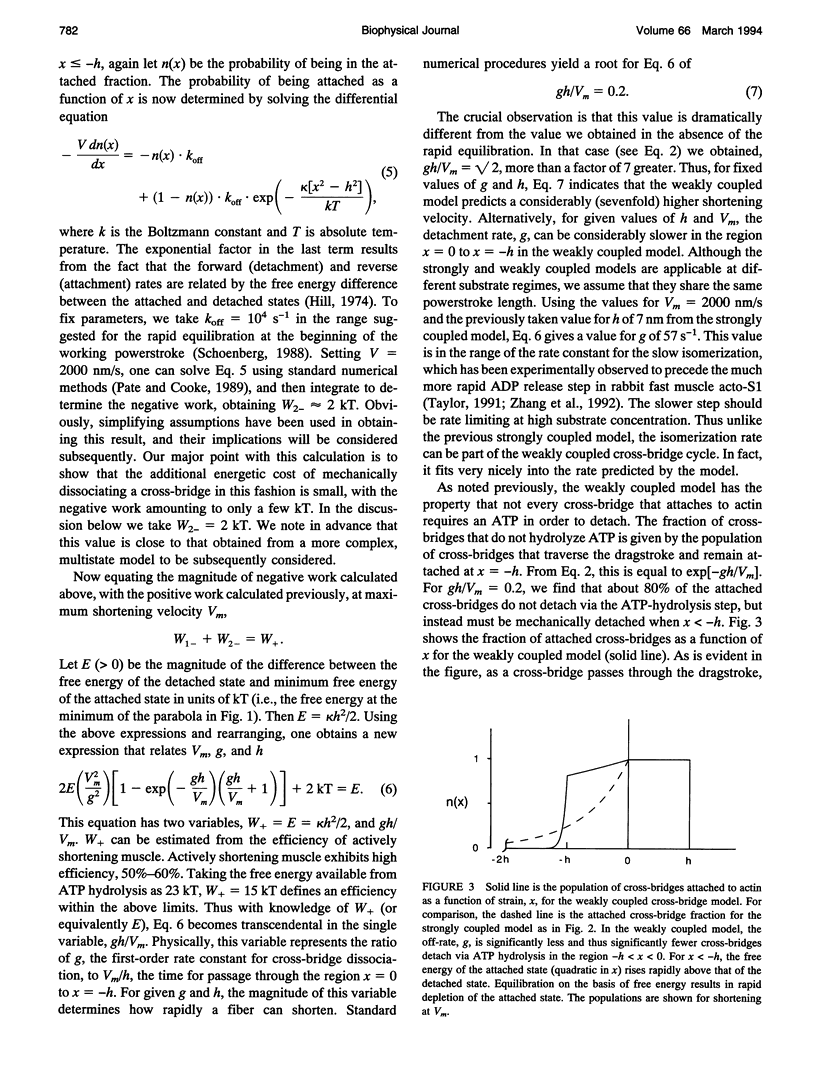
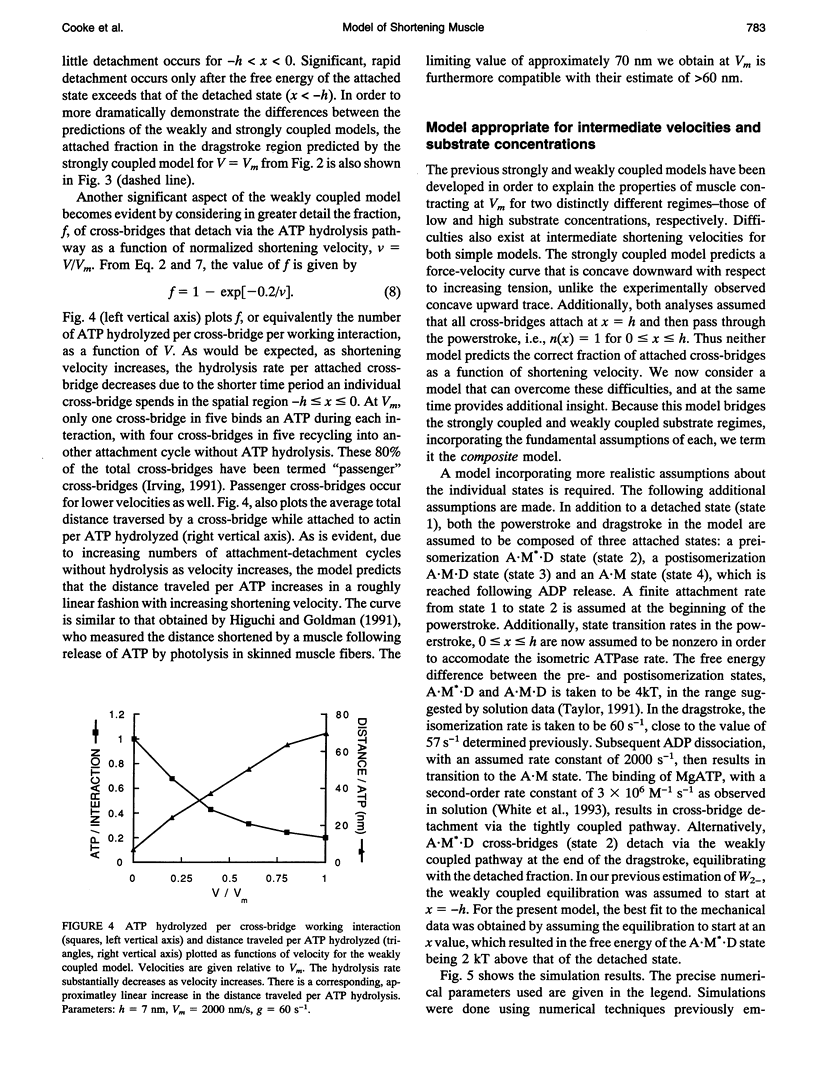
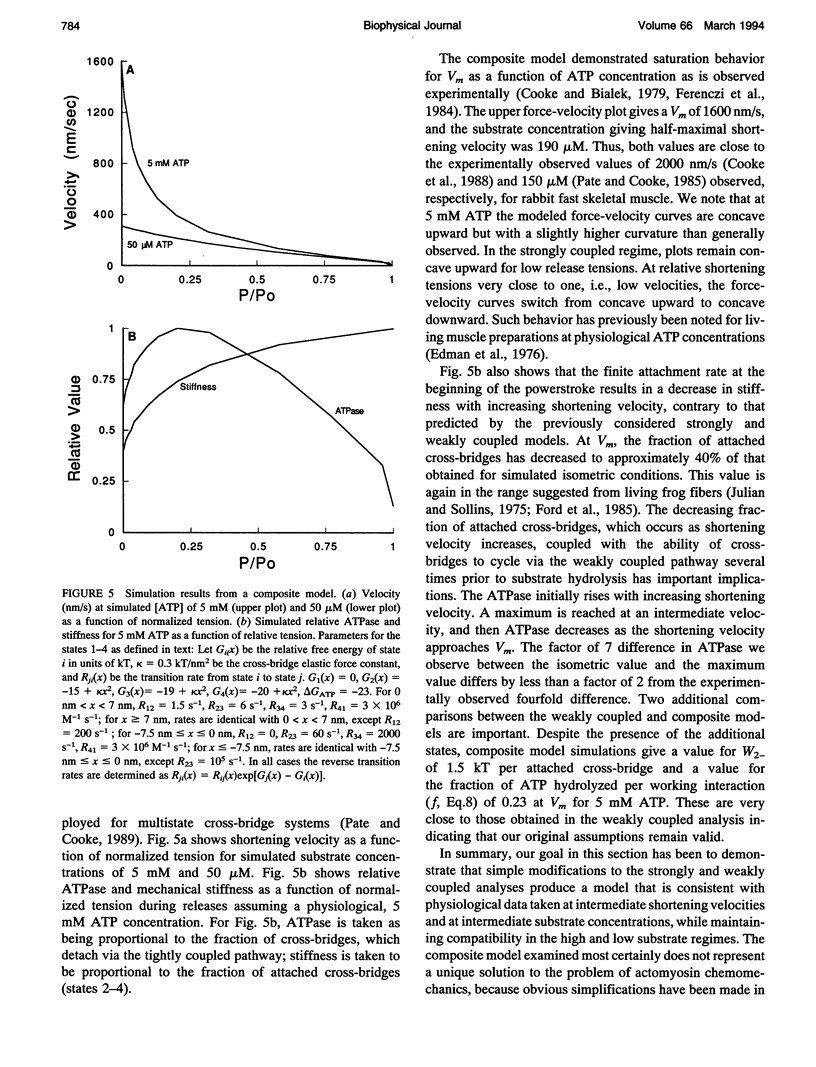
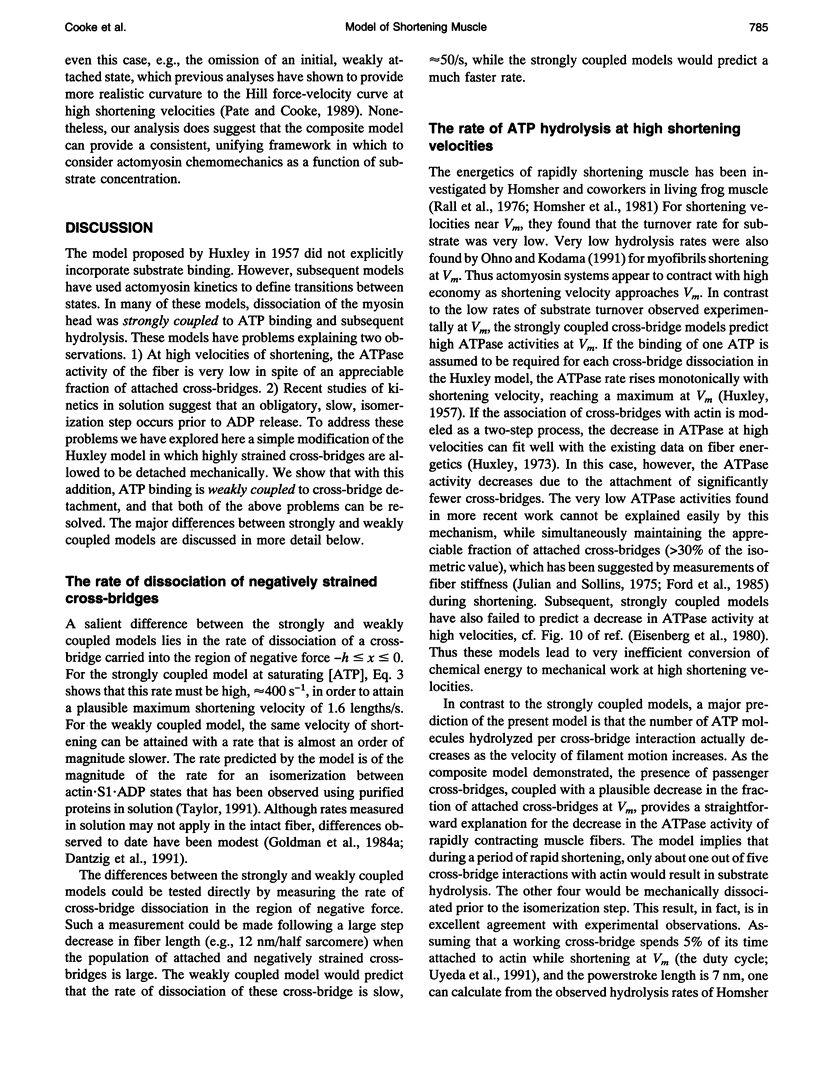
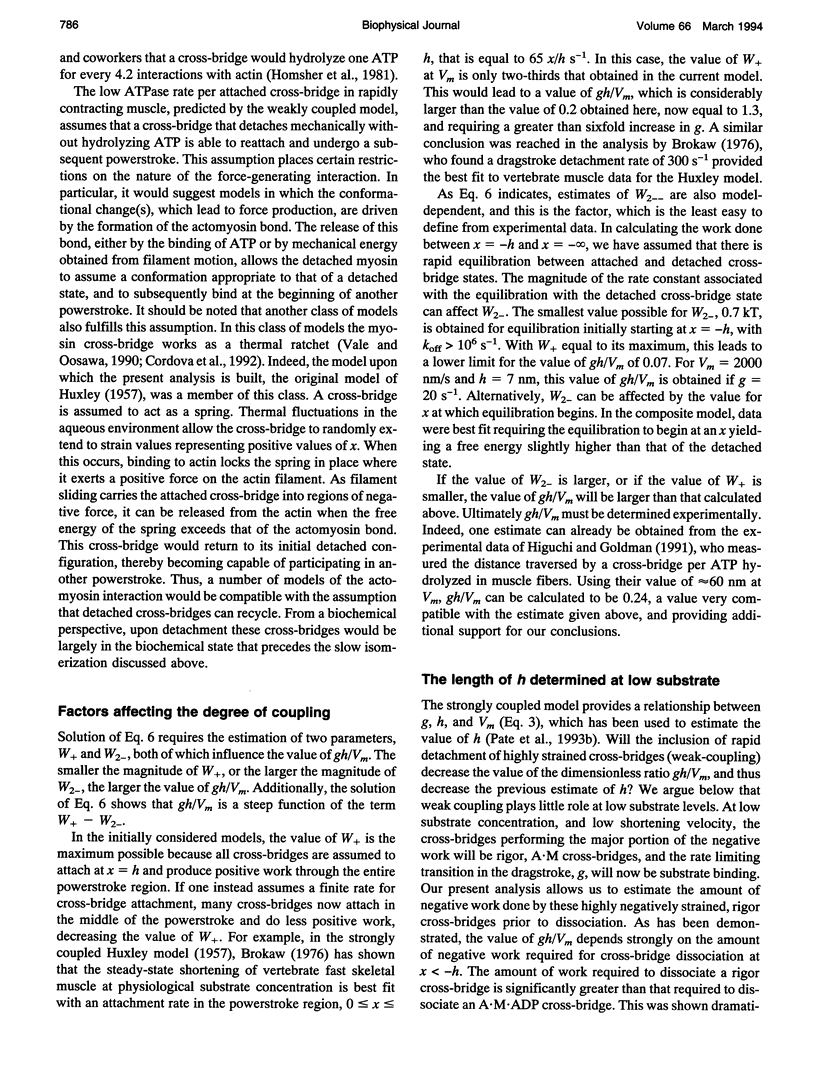
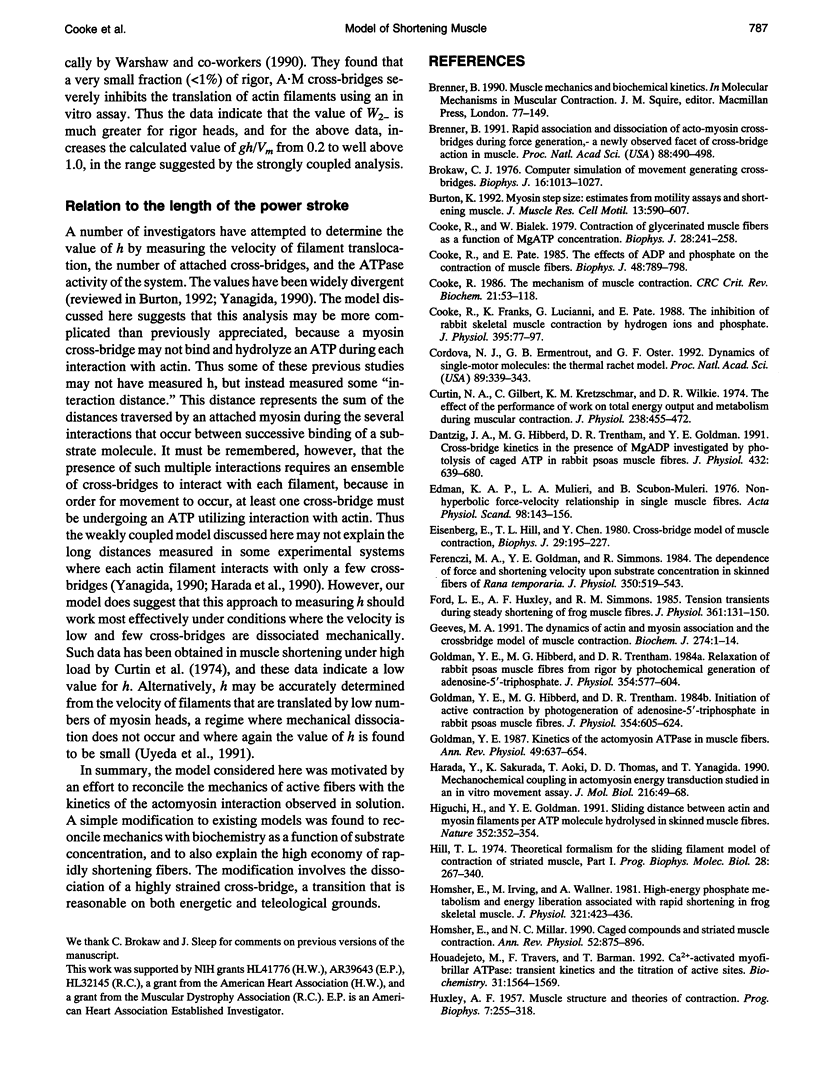
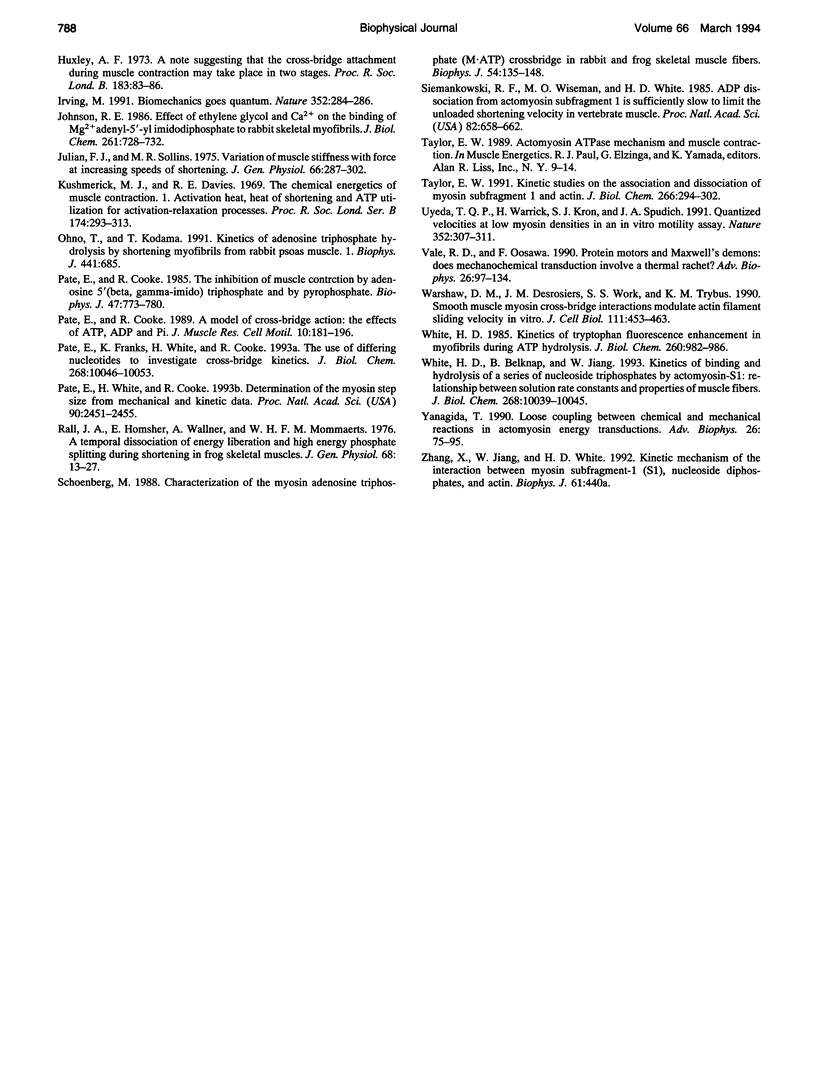
Selected References
These references are in PubMed. This may not be the complete list of references from this article.
- Brokaw C. J. Computer simulation of movement-generating cross-bridges. Biophys J. 1976 Sep;16(9):1013–1027. doi: 10.1016/S0006-3495(76)85752-9. [DOI] [PMC free article] [PubMed] [Google Scholar]
- Burton K. Myosin step size: estimates from motility assays and shortening muscle. J Muscle Res Cell Motil. 1992 Dec;13(6):590–607. doi: 10.1007/BF01738249. [DOI] [PubMed] [Google Scholar]
- Cooke R., Bialek W. Contraction of glycerinated muscle fibers as a function of the ATP concentration. Biophys J. 1979 Nov;28(2):241–258. doi: 10.1016/S0006-3495(79)85174-7. [DOI] [PMC free article] [PubMed] [Google Scholar]
- Cooke R., Franks K., Luciani G. B., Pate E. The inhibition of rabbit skeletal muscle contraction by hydrogen ions and phosphate. J Physiol. 1988 Jan;395:77–97. doi: 10.1113/jphysiol.1988.sp016909. [DOI] [PMC free article] [PubMed] [Google Scholar]
- Cooke R., Pate E. The effects of ADP and phosphate on the contraction of muscle fibers. Biophys J. 1985 Nov;48(5):789–798. doi: 10.1016/S0006-3495(85)83837-6. [DOI] [PMC free article] [PubMed] [Google Scholar]
- Cooke R. The mechanism of muscle contraction. CRC Crit Rev Biochem. 1986;21(1):53–118. doi: 10.3109/10409238609113609. [DOI] [PubMed] [Google Scholar]
- Curtin N. A., Gilbert C., Kretzschmar K. M., Wilkie D. R. The effect of the performance of work on total energy output and metabolism during muscular contraction. J Physiol. 1974 May;238(3):455–472. doi: 10.1113/jphysiol.1974.sp010537. [DOI] [PMC free article] [PubMed] [Google Scholar]
- Córdova N. J., Ermentrout B., Oster G. F. Dynamics of single-motor molecules: the thermal ratchet model. Proc Natl Acad Sci U S A. 1992 Jan 1;89(1):339–343. doi: 10.1073/pnas.89.1.339. [DOI] [PMC free article] [PubMed] [Google Scholar]
- Dantzig J. A., Hibberd M. G., Trentham D. R., Goldman Y. E. Cross-bridge kinetics in the presence of MgADP investigated by photolysis of caged ATP in rabbit psoas muscle fibres. J Physiol. 1991 Jan;432:639–680. doi: 10.1113/jphysiol.1991.sp018405. [DOI] [PMC free article] [PubMed] [Google Scholar]
- Edman K. A., Mulieri L. A., Scubon-Mulieri B. Non-hyperbolic force-velocity relationship in single muscle fibres. Acta Physiol Scand. 1976 Oct;98(2):143–156. doi: 10.1111/j.1748-1716.1976.tb00234.x. [DOI] [PubMed] [Google Scholar]
- Eisenberg E., Hill T. L., Chen Y. Cross-bridge model of muscle contraction. Quantitative analysis. Biophys J. 1980 Feb;29(2):195–227. doi: 10.1016/S0006-3495(80)85126-5. [DOI] [PMC free article] [PubMed] [Google Scholar]
- Ferenczi M. A., Goldman Y. E., Simmons R. M. The dependence of force and shortening velocity on substrate concentration in skinned muscle fibres from Rana temporaria. J Physiol. 1984 May;350:519–543. doi: 10.1113/jphysiol.1984.sp015216. [DOI] [PMC free article] [PubMed] [Google Scholar]
- Ford L. E., Huxley A. F., Simmons R. M. Tension transients during steady shortening of frog muscle fibres. J Physiol. 1985 Apr;361:131–150. doi: 10.1113/jphysiol.1985.sp015637. [DOI] [PMC free article] [PubMed] [Google Scholar]
- Geeves M. A. The dynamics of actin and myosin association and the crossbridge model of muscle contraction. Biochem J. 1991 Feb 15;274(Pt 1):1–14. doi: 10.1042/bj2740001. [DOI] [PMC free article] [PubMed] [Google Scholar]
- Goldman Y. E., Hibberd M. G., Trentham D. R. Initiation of active contraction by photogeneration of adenosine-5'-triphosphate in rabbit psoas muscle fibres. J Physiol. 1984 Sep;354:605–624. doi: 10.1113/jphysiol.1984.sp015395. [DOI] [PMC free article] [PubMed] [Google Scholar]
- Goldman Y. E., Hibberd M. G., Trentham D. R. Relaxation of rabbit psoas muscle fibres from rigor by photochemical generation of adenosine-5'-triphosphate. J Physiol. 1984 Sep;354:577–604. doi: 10.1113/jphysiol.1984.sp015394. [DOI] [PMC free article] [PubMed] [Google Scholar]
- Goldman Y. E. Kinetics of the actomyosin ATPase in muscle fibers. Annu Rev Physiol. 1987;49:637–654. doi: 10.1146/annurev.ph.49.030187.003225. [DOI] [PubMed] [Google Scholar]
- HUXLEY A. F. Muscle structure and theories of contraction. Prog Biophys Biophys Chem. 1957;7:255–318. [PubMed] [Google Scholar]
- Harada Y., Sakurada K., Aoki T., Thomas D. D., Yanagida T. Mechanochemical coupling in actomyosin energy transduction studied by in vitro movement assay. J Mol Biol. 1990 Nov 5;216(1):49–68. doi: 10.1016/S0022-2836(05)80060-9. [DOI] [PubMed] [Google Scholar]
- Higuchi H., Goldman Y. E. Sliding distance between actin and myosin filaments per ATP molecule hydrolysed in skinned muscle fibres. Nature. 1991 Jul 25;352(6333):352–354. doi: 10.1038/352352a0. [DOI] [PubMed] [Google Scholar]
- Hill T. L. Theoretical formalism for the sliding filament model of contraction of striated muscle. Part I. Prog Biophys Mol Biol. 1974;28:267–340. doi: 10.1016/0079-6107(74)90020-0. [DOI] [PubMed] [Google Scholar]
- Homsher E., Irving M., Wallner A. High-energy phosphate metabolism and energy liberation associated with rapid shortening in frog skeletal muscle. J Physiol. 1981 Dec;321:423–436. doi: 10.1113/jphysiol.1981.sp013994. [DOI] [PMC free article] [PubMed] [Google Scholar]
- Homsher E., Millar N. C. Caged compounds and striated muscle contraction. Annu Rev Physiol. 1990;52:875–896. doi: 10.1146/annurev.ph.52.030190.004303. [DOI] [PubMed] [Google Scholar]
- Houadjeto M., Travers F., Barman T. Ca(2+)-activated myofibrillar ATPase: transient kinetics and the titration of its active sites. Biochemistry. 1992 Feb 11;31(5):1564–1569. doi: 10.1021/bi00120a038. [DOI] [PubMed] [Google Scholar]
- Huxley A. F. A note suggesting that the cross-bridge attachment during muscle contraction may take place in two stages. Proc R Soc Lond B Biol Sci. 1973 Feb 27;183(1070):83–86. doi: 10.1098/rspb.1973.0006. [DOI] [PubMed] [Google Scholar]
- Irving M. Motor proteins. Biomechanics goes quantum. Nature. 1991 Jul 25;352(6333):284–286. doi: 10.1038/352284a0. [DOI] [PubMed] [Google Scholar]
- Johnson R. E. Effect of ethylene glycol and Ca2+ on the binding of Mg2+ x adenyl-5'-yl imidodiphosphate to rabbit skeletal myofibrils. J Biol Chem. 1986 Jan 15;261(2):728–732. [PubMed] [Google Scholar]
- Julian F. J., Sollins M. R. Variation of muscle stiffness with force at increasing speeds of shortening. J Gen Physiol. 1975 Sep;66(3):287–302. doi: 10.1085/jgp.66.3.287. [DOI] [PMC free article] [PubMed] [Google Scholar]
- Kushmerick M. J., Larson R. E., Davies R. E. The chemical energetics of muscle contraction. I. Activation heat, heat of shortening and ATP utilization for activation-relaxation processes. Proc R Soc Lond B Biol Sci. 1969 Dec 23;174(1036):293–313. doi: 10.1098/rspb.1969.0095. [DOI] [PubMed] [Google Scholar]
- Ohno T., Kodama T. Kinetics of adenosine triphosphate hydrolysis by shortening myofibrils from rabbit psoas muscle. J Physiol. 1991 Sep;441:685–702. doi: 10.1113/jphysiol.1991.sp018773. [DOI] [PMC free article] [PubMed] [Google Scholar]
- Pate E., Cooke R. A model of crossbridge action: the effects of ATP, ADP and Pi. J Muscle Res Cell Motil. 1989 Jun;10(3):181–196. doi: 10.1007/BF01739809. [DOI] [PubMed] [Google Scholar]
- Pate E., Cooke R. The inhibition of muscle contraction by adenosine 5' (beta, gamma-imido) triphosphate and by pyrophosphate. Biophys J. 1985 Jun;47(6):773–780. doi: 10.1016/S0006-3495(85)83980-1. [DOI] [PMC free article] [PubMed] [Google Scholar]
- Pate E., Franks-Skiba K., White H., Cooke R. The use of differing nucleotides to investigate cross-bridge kinetics. J Biol Chem. 1993 May 15;268(14):10046–10053. [PubMed] [Google Scholar]
- Pate E., White H., Cooke R. Determination of the myosin step size from mechanical and kinetic data. Proc Natl Acad Sci U S A. 1993 Mar 15;90(6):2451–2455. doi: 10.1073/pnas.90.6.2451. [DOI] [PMC free article] [PubMed] [Google Scholar]
- Rall J. A., Homsher E., Wallner A., Mommaerts W. F. A temporal dissociation of energy liberation and high energy phosphate splitting during shortening in frog skeletal muscles. J Gen Physiol. 1976 Jul;68(1):13–27. doi: 10.1085/jgp.68.1.13. [DOI] [PMC free article] [PubMed] [Google Scholar]
- Schoenberg M. Characterization of the myosin adenosine triphosphate (M.ATP) crossbridge in rabbit and frog skeletal muscle fibers. Biophys J. 1988 Jul;54(1):135–148. doi: 10.1016/S0006-3495(88)82938-2. [DOI] [PMC free article] [PubMed] [Google Scholar]
- Siemankowski R. F., Wiseman M. O., White H. D. ADP dissociation from actomyosin subfragment 1 is sufficiently slow to limit the unloaded shortening velocity in vertebrate muscle. Proc Natl Acad Sci U S A. 1985 Feb;82(3):658–662. doi: 10.1073/pnas.82.3.658. [DOI] [PMC free article] [PubMed] [Google Scholar]
- Taylor E. W. Kinetic studies on the association and dissociation of myosin subfragment 1 and actin. J Biol Chem. 1991 Jan 5;266(1):294–302. [PubMed] [Google Scholar]
- Uyeda T. Q., Warrick H. M., Kron S. J., Spudich J. A. Quantized velocities at low myosin densities in an in vitro motility assay. Nature. 1991 Jul 25;352(6333):307–311. doi: 10.1038/352307a0. [DOI] [PubMed] [Google Scholar]
- Vale R. D., Oosawa F. Protein motors and Maxwell's demons: does mechanochemical transduction involve a thermal ratchet? Adv Biophys. 1990;26:97–134. doi: 10.1016/0065-227x(90)90009-i. [DOI] [PubMed] [Google Scholar]
- Warshaw D. M., Desrosiers J. M., Work S. S., Trybus K. M. Smooth muscle myosin cross-bridge interactions modulate actin filament sliding velocity in vitro. J Cell Biol. 1990 Aug;111(2):453–463. doi: 10.1083/jcb.111.2.453. [DOI] [PMC free article] [PubMed] [Google Scholar]
- White H. D., Belknap B., Jiang W. Kinetics of binding and hydrolysis of a series of nucleoside triphosphates by actomyosin-S1. Relationship between solution rate constants and properties of muscle fibers. J Biol Chem. 1993 May 15;268(14):10039–10045. [PubMed] [Google Scholar]
- White H. D. Kinetics of tryptophan fluorescence enhancement in myofibrils during ATP hydrolysis. J Biol Chem. 1985 Jan 25;260(2):982–986. [PubMed] [Google Scholar]
- Yanagida T. Loose coupling between chemical and mechanical reactions in actomyosin energy transduction. Adv Biophys. 1990;26:75–95. doi: 10.1016/0065-227x(90)90008-h. [DOI] [PubMed] [Google Scholar]