Abstract
When placed in rotating electric fields red blood cells show a typical electrorotation spectrum with antifield rotation in the lower and cofield rotation in the higher frequency range. Assuming a spherical cell geometry, however, dielectrical parameters were obtained that differ from those measured by independent methods. Dielectrophoresis and, in particular, electrorotation yielded lower membrane capacitance values than expected. Introduction of an ellipsoidal model with an axis ratio of 1:2 allowed a description that proved to be consistent with dielectrophoresis and electrorotation data. For control cells an internal conductivity of 0.535 S/m, a specific membrane capacitance of 0.82 x 10(-2) F/m2, and a specific conductance of 480 S/m2 were obtained. The first characteristic frequency (frequency of fastest antifield rotation) and the related rotation speed can be measured quite quickly by means of a compensation method. Thus it was possible to follow changes of dielectric properties on individual cells after nystatin application. Ionophore-membrane interaction caused cell shrinkage in parallel to a decrease of the first characteristic frequency and rotation speed. Analysis of data revealed a decrease of the internal conductivity that is not only caused by ion loss but also, to a large extent, by a strong increase of hindrance because of shrinkage. Ionophore-induced membrane permeabilities can be calculated from volume decrease as well as from electrorotational data. In no case can these permeabilities count for the high membrane-AC conductivity that is attributed to the band-3 anion exchanging protein. The membrane-AC conductance was found not to be decreased for cells in Donnan equilibrium, which had leaked out almost completely.
Full text
PDF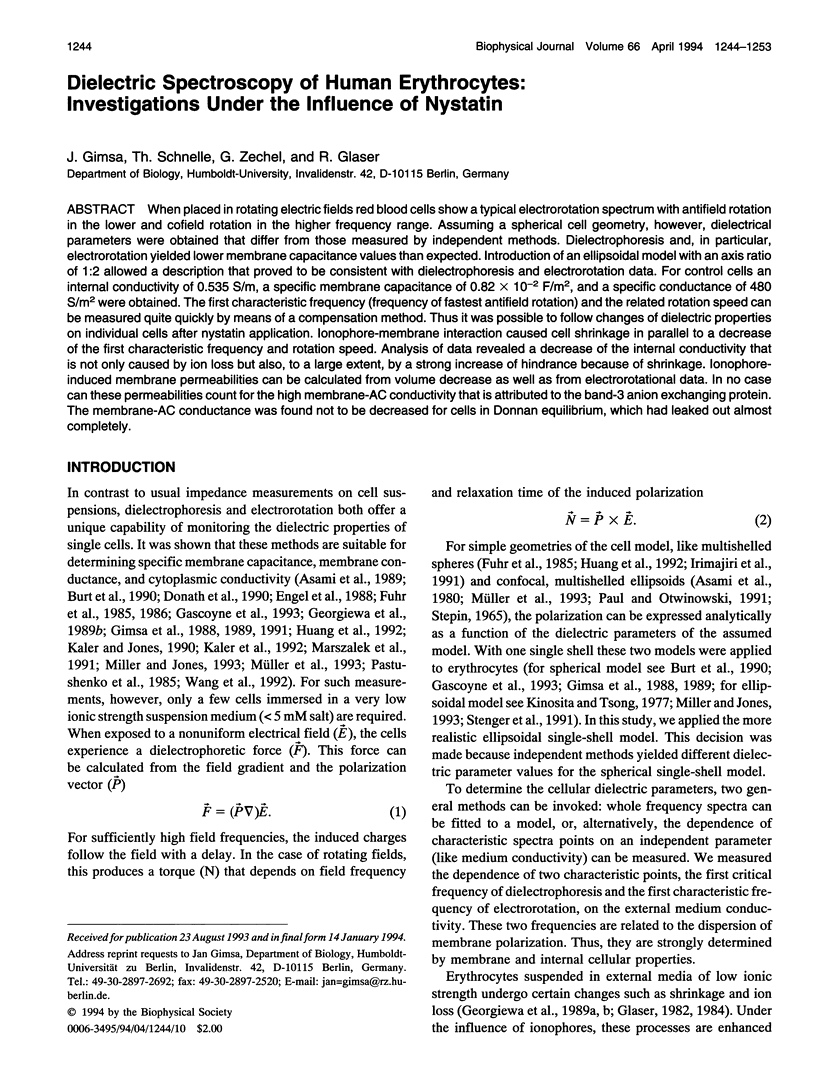
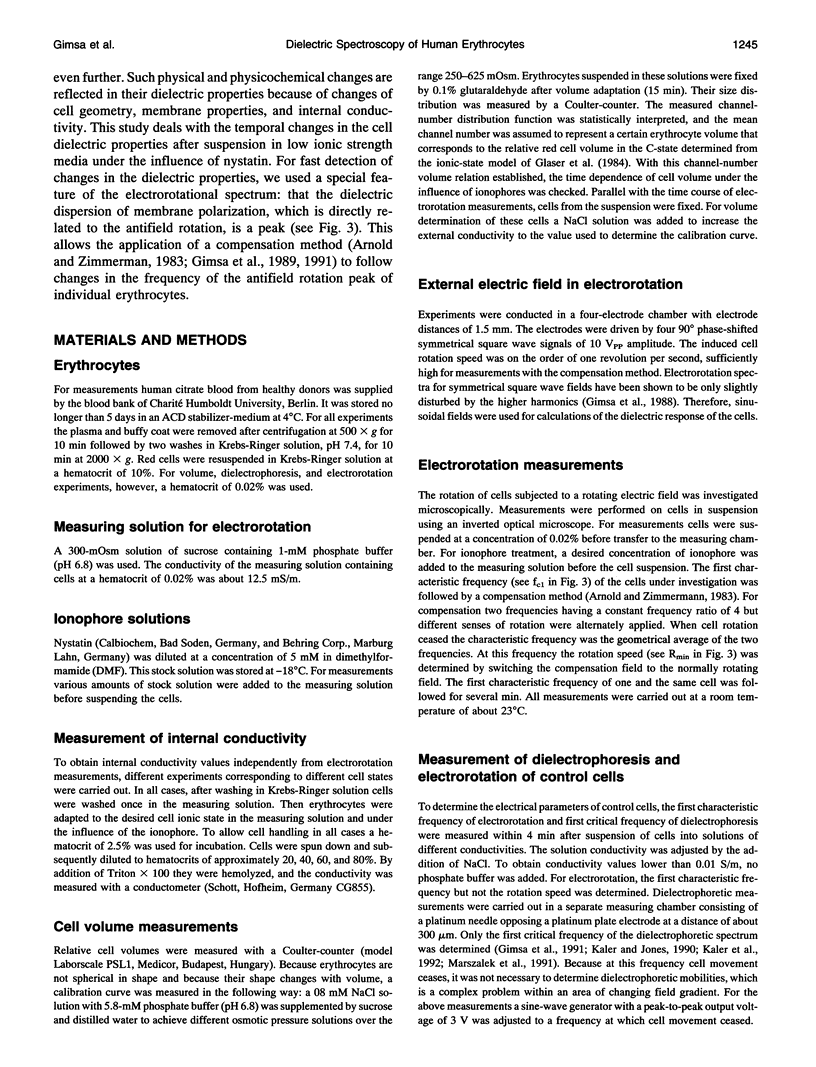
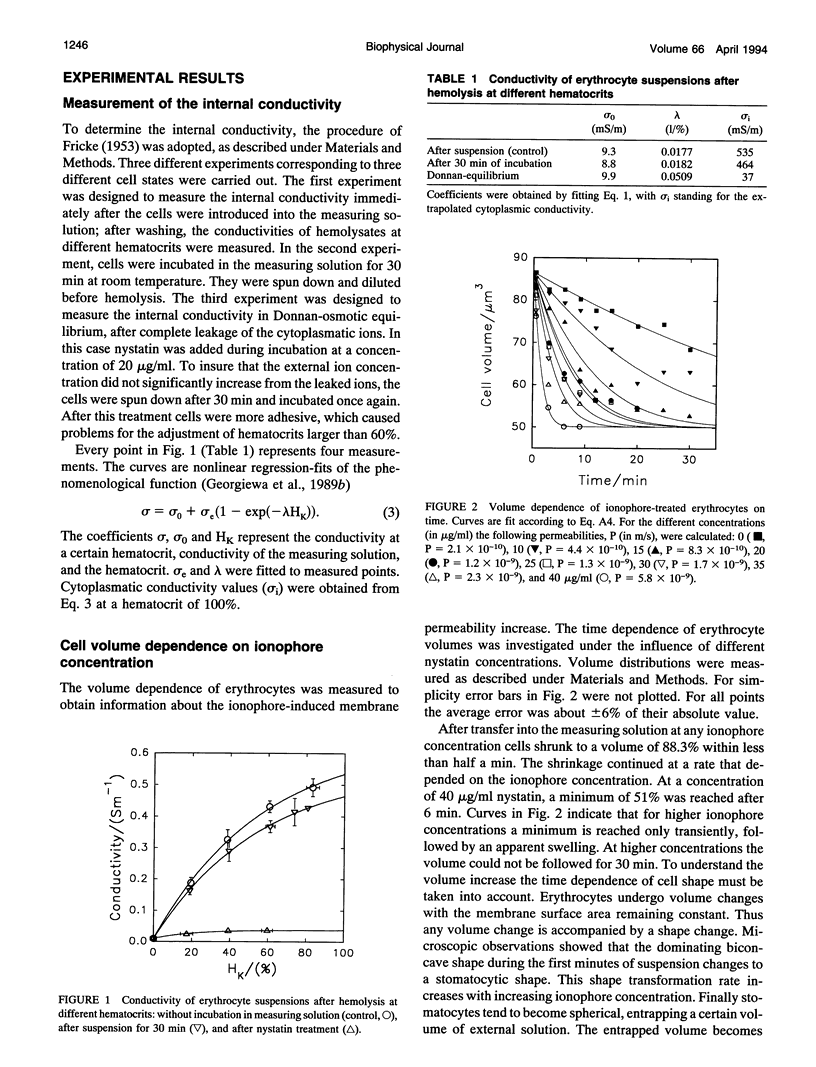
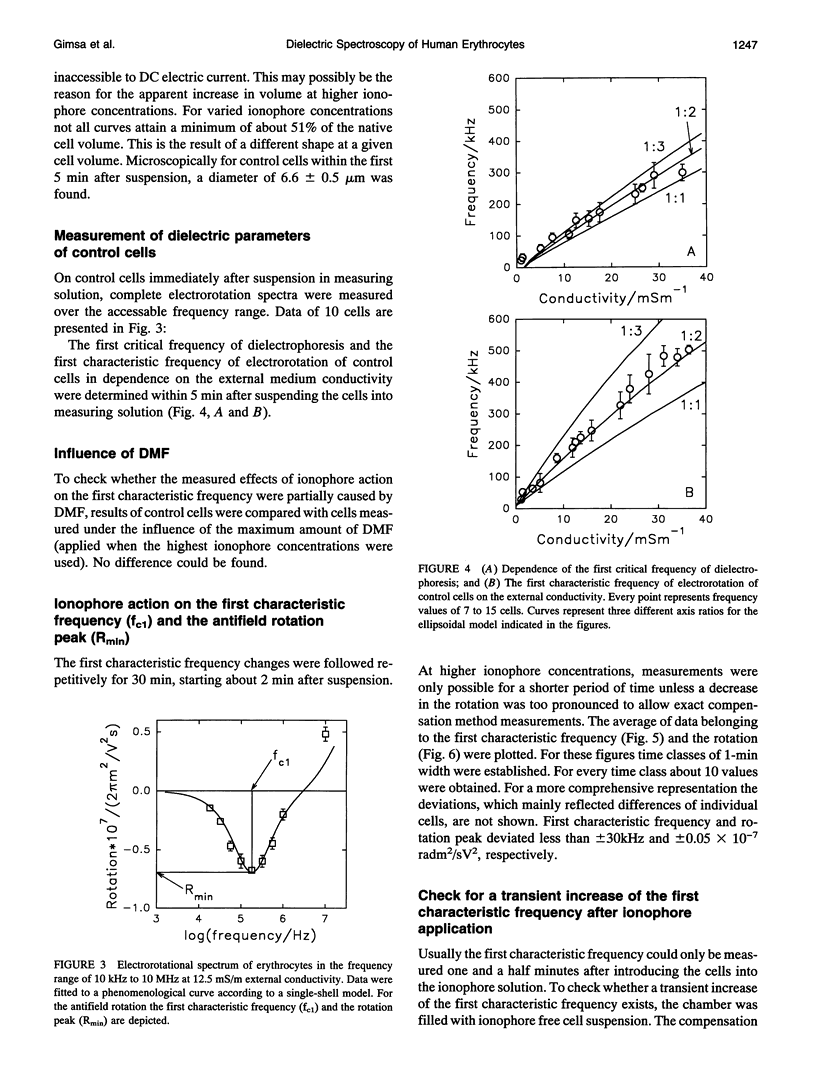
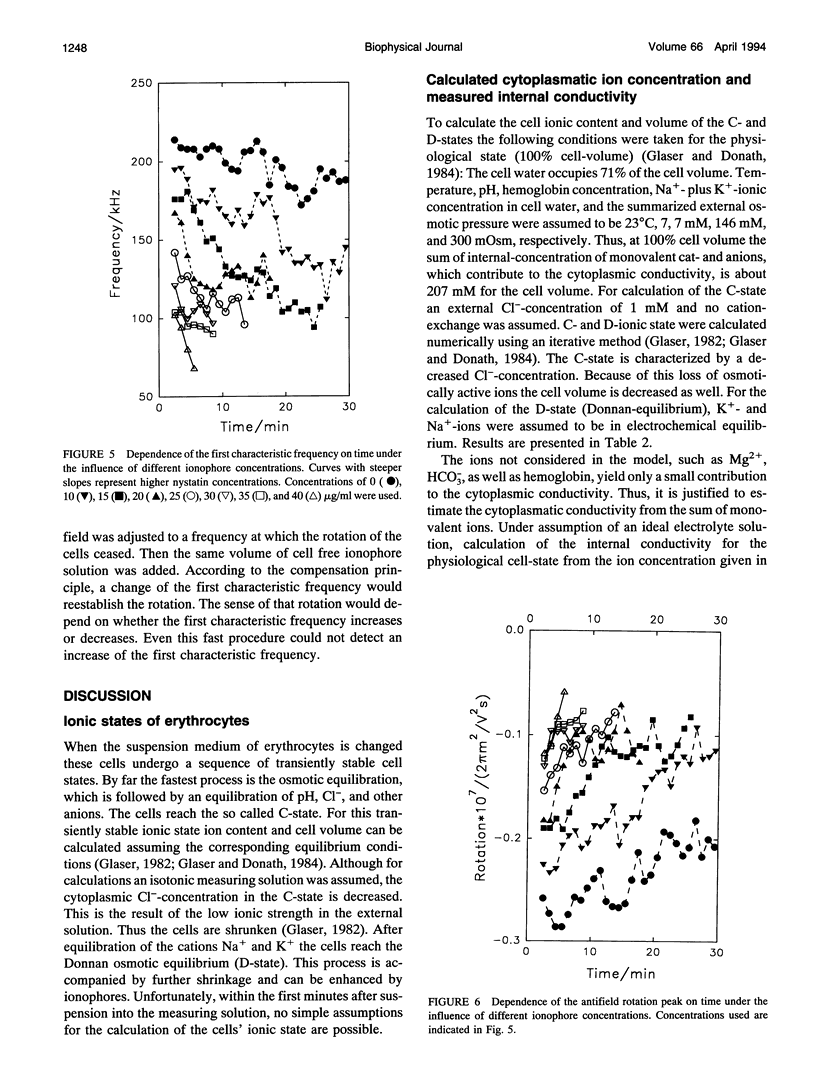
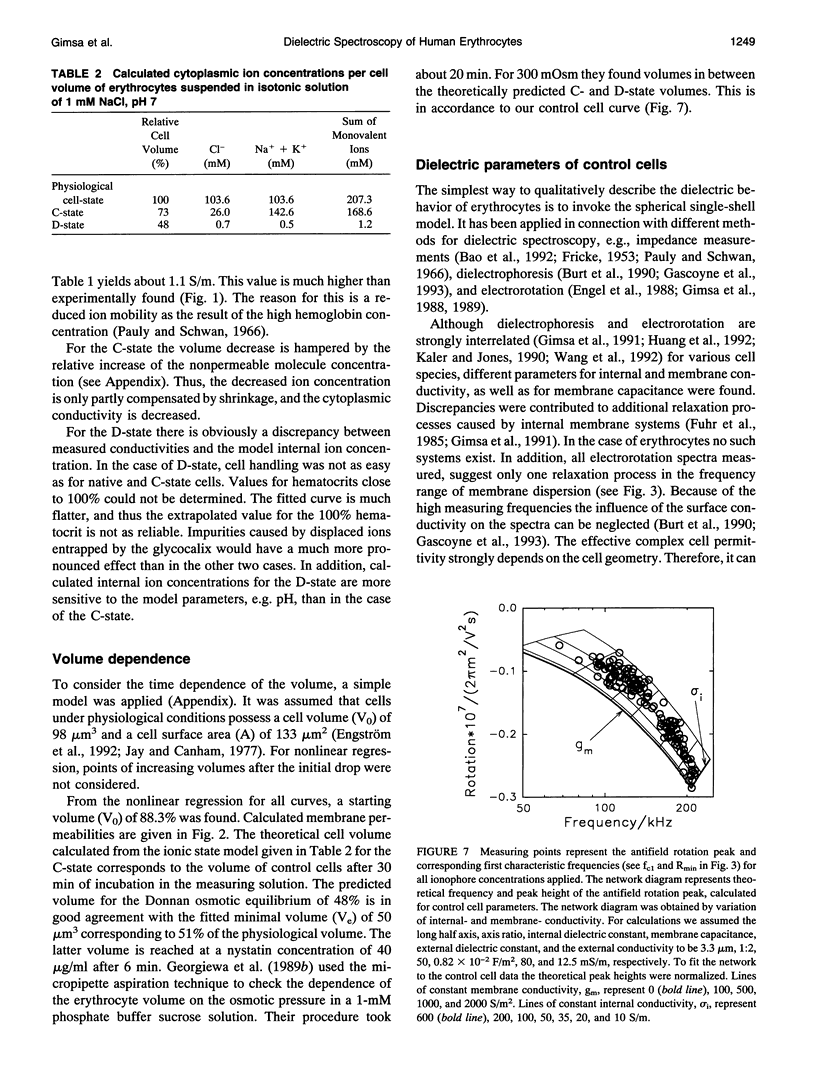
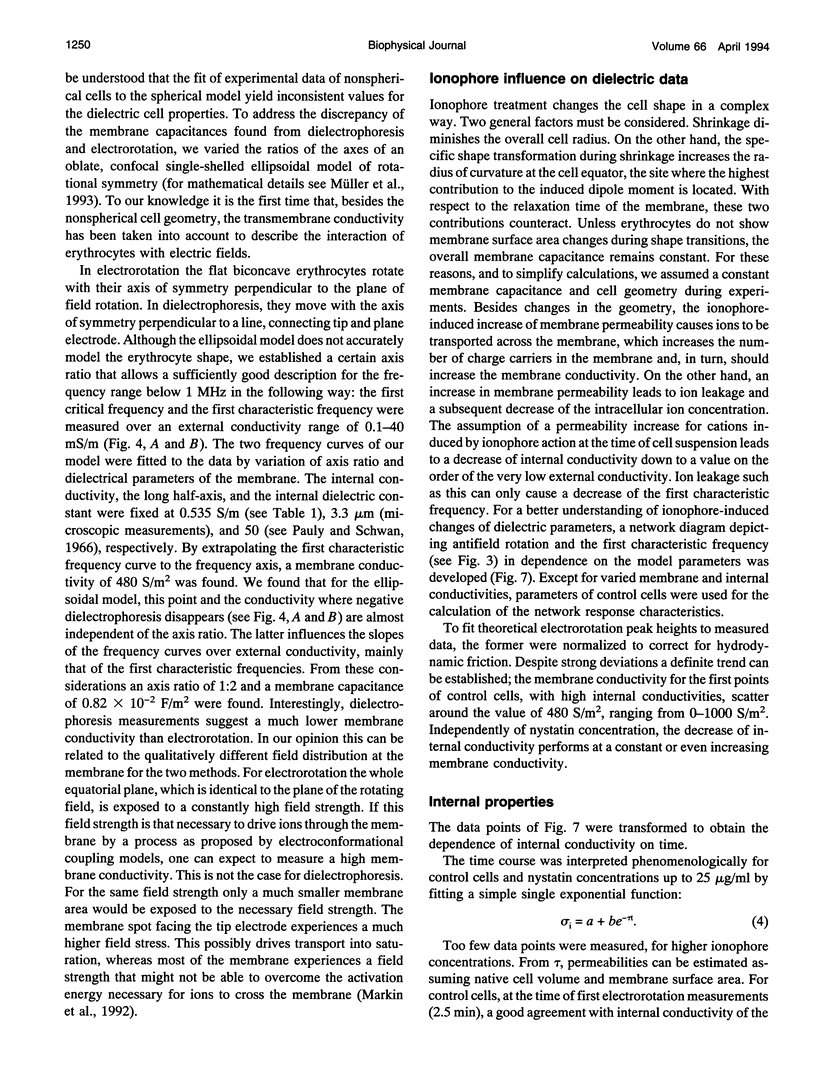
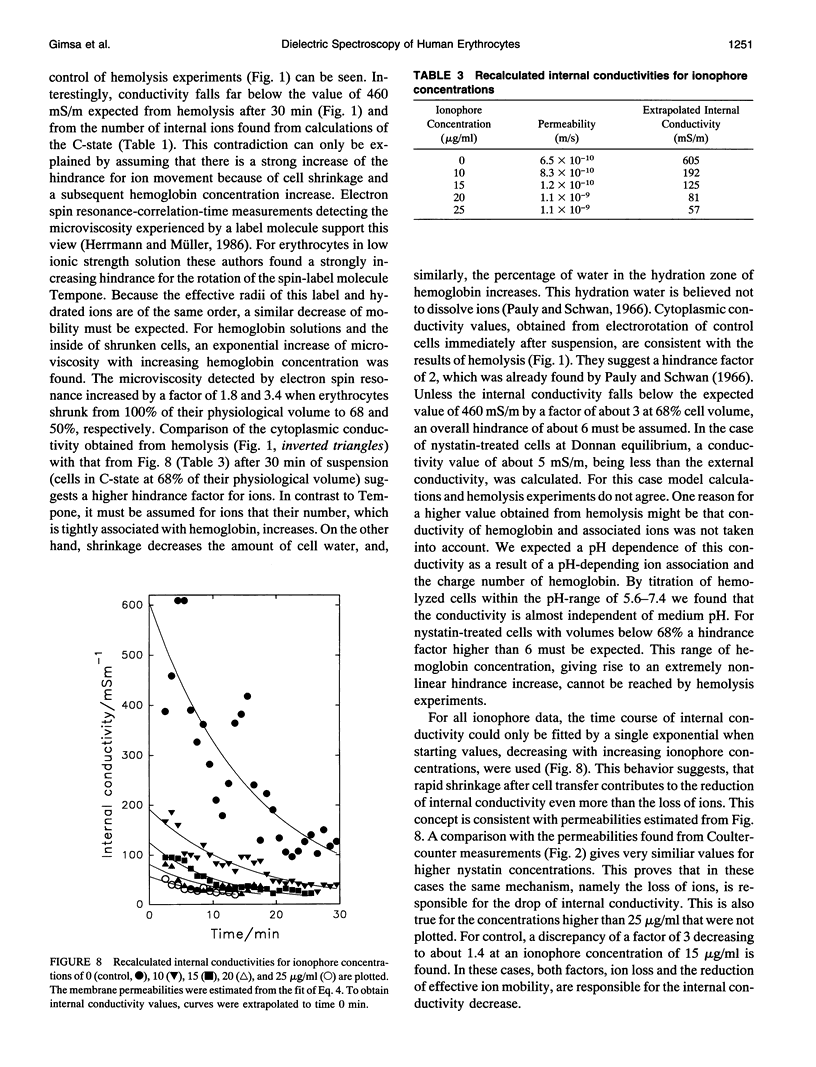
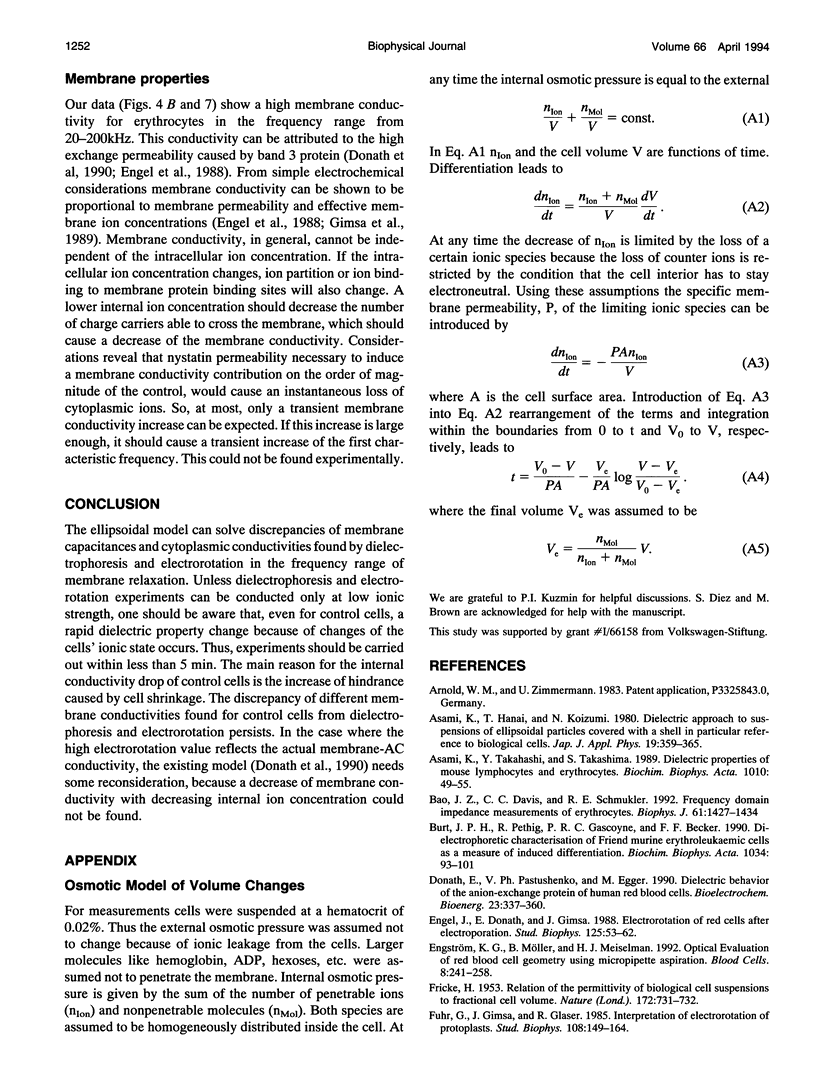
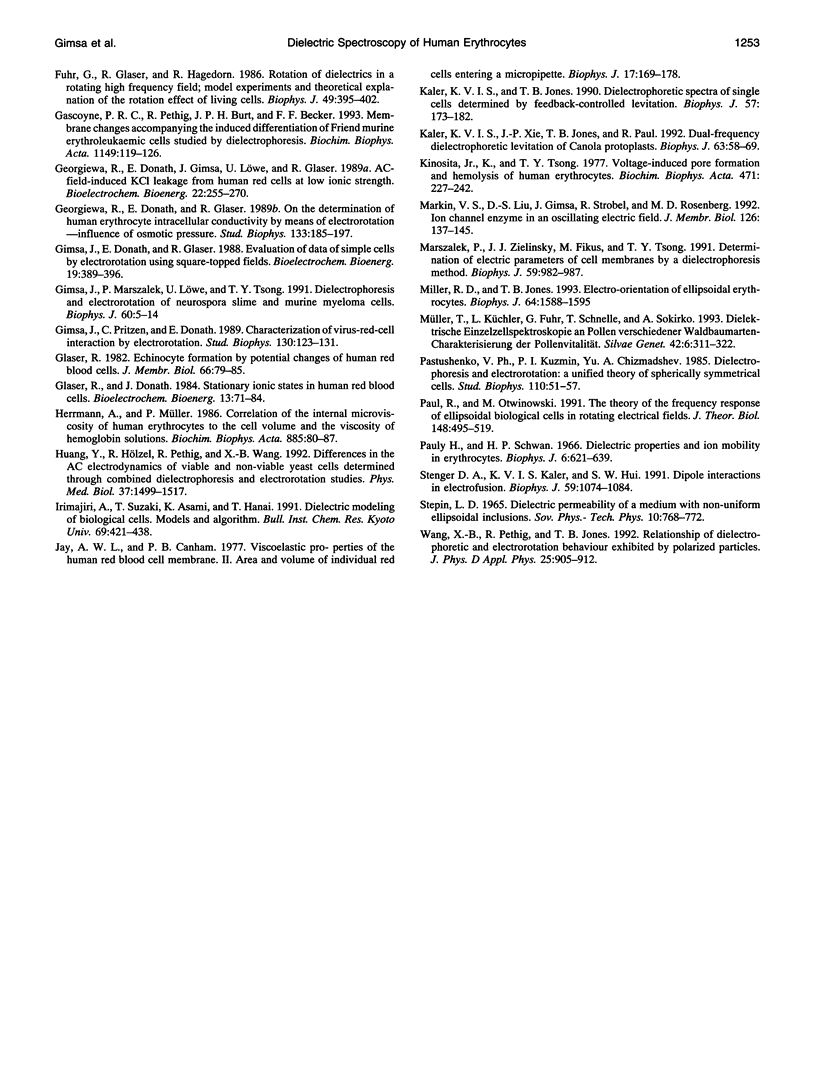
Selected References
These references are in PubMed. This may not be the complete list of references from this article.
- Asami K., Takahashi Y., Takashima S. Dielectric properties of mouse lymphocytes and erythrocytes. Biochim Biophys Acta. 1989 Jan 17;1010(1):49–55. doi: 10.1016/0167-4889(89)90183-3. [DOI] [PubMed] [Google Scholar]
- Bao J. Z., Davis C. C., Schmukler R. E. Frequency domain impedance measurements of erythrocytes. Constant phase angle impedance characteristics and a phase transition. Biophys J. 1992 May;61(5):1427–1434. doi: 10.1016/S0006-3495(92)81948-3. [DOI] [PMC free article] [PubMed] [Google Scholar]
- Burt J. P., Pethig R., Gascoyne P. R., Becker F. F. Dielectrophoretic characterisation of Friend murine erythroleukaemic cells as a measure of induced differentiation. Biochim Biophys Acta. 1990 Apr 23;1034(1):93–101. doi: 10.1016/0304-4165(90)90158-s. [DOI] [PubMed] [Google Scholar]
- Engström K. G., Möller B., Meiselman H. J. Optical evaluation of red blood cell geometry using micropipette aspiration. Blood Cells. 1992;18(2):241–265. [PubMed] [Google Scholar]
- FRICKE H. Relation of the permitivity of biological cell suspensions to fractional cell volume. Nature. 1953 Oct 17;172(4381):731–732. doi: 10.1038/172731a0. [DOI] [PubMed] [Google Scholar]
- Fuhr G., Glaser R., Hagedorn R. Rotation of dielectrics in a rotating electric high-frequency field. Model experiments and theoretical explanation of the rotation effect of living cells. Biophys J. 1986 Feb;49(2):395–402. doi: 10.1016/S0006-3495(86)83649-9. [DOI] [PMC free article] [PubMed] [Google Scholar]
- Gascoyne P. R., Pethig R., Burt J. P., Becker F. F. Membrane changes accompanying the induced differentiation of Friend murine erythroleukemia cells studied by dielectrophoresis. Biochim Biophys Acta. 1993 Jun 18;1149(1):119–126. doi: 10.1016/0005-2736(93)90032-u. [DOI] [PubMed] [Google Scholar]
- Glaser R. Echinocyte formation induced by potential changes of human red blood cells. J Membr Biol. 1982;66(2):79–85. doi: 10.1007/BF01868484. [DOI] [PubMed] [Google Scholar]
- Herrmann A., Müller P. Correlation of the internal microviscosity of human erythrocytes to the cell volume and the viscosity of hemoglobin solutions. Biochim Biophys Acta. 1986 Jan 23;885(1):80–87. doi: 10.1016/0167-4889(86)90041-8. [DOI] [PubMed] [Google Scholar]
- Huang Y., Hölzel R., Pethig R., Wang X. B. Differences in the AC electrodynamics of viable and non-viable yeast cells determined through combined dielectrophoresis and electrorotation studies. Phys Med Biol. 1992 Jul;37(7):1499–1517. doi: 10.1088/0031-9155/37/7/003. [DOI] [PubMed] [Google Scholar]
- Jay A. W., Canham P. B. Viscoelastic properties of the human red blood cell membrane. II. Area and volume of individual red cells entering a micropipette. Biophys J. 1977 Feb;17(2):169–178. doi: 10.1016/S0006-3495(77)85634-8. [DOI] [PMC free article] [PubMed] [Google Scholar]
- Kaler K. V., Jones T. B. Dielectrophoretic spectra of single cells determined by feedback-controlled levitation. Biophys J. 1990 Feb;57(2):173–182. doi: 10.1016/S0006-3495(90)82520-0. [DOI] [PMC free article] [PubMed] [Google Scholar]
- Kaler K. V., Xie J. P., Jones T. B., Paul R. Dual-frequency dielectrophoretic levitation of Canola protoplasts. Biophys J. 1992 Jul;63(1):58–69. doi: 10.1016/S0006-3495(92)81586-2. [DOI] [PMC free article] [PubMed] [Google Scholar]
- Kinosita K., Jr, Tsong T. Y. Voltage-induced pore formation and hemolysis of human erythrocytes. Biochim Biophys Acta. 1977 Dec 1;471(2):227–242. doi: 10.1016/0005-2736(77)90252-8. [DOI] [PubMed] [Google Scholar]
- Markin V. S., Liu D., Gimsa J., Strobel R., Rosenberg M. D., Tsong T. Y. Ion channel enzyme in an oscillating electric field. J Membr Biol. 1992 Mar;126(2):137–145. doi: 10.1007/BF00231912. [DOI] [PubMed] [Google Scholar]
- Marszalek P., Zielinsky J. J., Fikus M., Tsong T. Y. Determination of electric parameters of cell membranes by a dielectrophoresis method. Biophys J. 1991 May;59(5):982–987. doi: 10.1016/S0006-3495(91)82312-8. [DOI] [PMC free article] [PubMed] [Google Scholar]
- Miller R. D., Jones T. B. Electro-orientation of ellipsoidal erythrocytes. Theory and experiment. Biophys J. 1993 May;64(5):1588–1595. doi: 10.1016/S0006-3495(93)81529-7. [DOI] [PMC free article] [PubMed] [Google Scholar]
- Paul R., Otwinowski M. The theory of the frequency response of ellipsoidal biological cells in rotating electrical fields. J Theor Biol. 1991 Feb 21;148(4):495–519. doi: 10.1016/s0022-5193(05)80233-4. [DOI] [PubMed] [Google Scholar]
- Pauly H., Schwan H. P. Dielectric properties and ion mobility in erythrocytes. Biophys J. 1966 Sep;6(5):621–639. doi: 10.1016/S0006-3495(66)86682-1. [DOI] [PMC free article] [PubMed] [Google Scholar]
- Stenger D. A., Kaler K. V., Hui S. W. Dipole interactions in electrofusion. Contributions of membrane potential and effective dipole interaction pressures. Biophys J. 1991 May;59(5):1074–1084. doi: 10.1016/S0006-3495(91)82322-0. [DOI] [PMC free article] [PubMed] [Google Scholar]