Abstract
Temperature dependence of the isometric tension was examined in chemically skinned, glycerinated, rabbit Psoas, muscle fibers immersed in relaxing solution (pH approximately 7.1 at 20 degrees C, pCa approximately 8, ionic strength 200 mM); the average rate of heating/cooling was 0.5-1 degree C/s. The resting tension increased reversibly with temperature (5-42 degrees C); the tension increase was slight in warming to approximately 25 degrees C (a linear thermal contraction, -alpha, of approximately 0.1%/degree C) but became more pronounced above approximately 30 degrees C (similar behavior was seen in intact rat muscle fibers). The extra tension rise at the high temperatures was depressed in acidic pH and in the presence of 10 mM inorganic phosphate; it was absent in rigor fibers in which the tension decreased with heating (a linear thermal expansion, alpha, of approximately 4 x 10(-5)/degree C). Below approximately 20 degrees C, the tension response after a approximately 1% length increase (complete < 0.5 ms) consisted of a fast decay (approximately 150.s-1 at 20 degrees C) and a slow decay (approximately 10.s-1) of tension. The rate of fast decay increased with temperature (Q10 approximately 2.4); at 35-40 degrees C, it was approximately 800.s-1, and it was followed by a delayed tension rise (stretch-activation) at 30-40.s-1. The linear rise of passive tension in warming to approximately 25 degrees C may be due to increase of thermal stress in titin (connectin)-myosin composite filament, whereas the extra tension above approximately 30 degrees C may arise from cycling cross-bridges; based on previous findings from regulated actomyosin in solution (Fuchs, 1975), it is suggested that heating reversibly inactivates the troponin-tropomyosin control mechanism and leads to Ca-independent thin filament activation at high temperatures. Additionally, we propose that the heating-induced increase of endo-sarcomeric stress within titin-myosin composite filament makes the cross-bridge mechanism stretch-sensitive at high temperatures.
Full text
PDF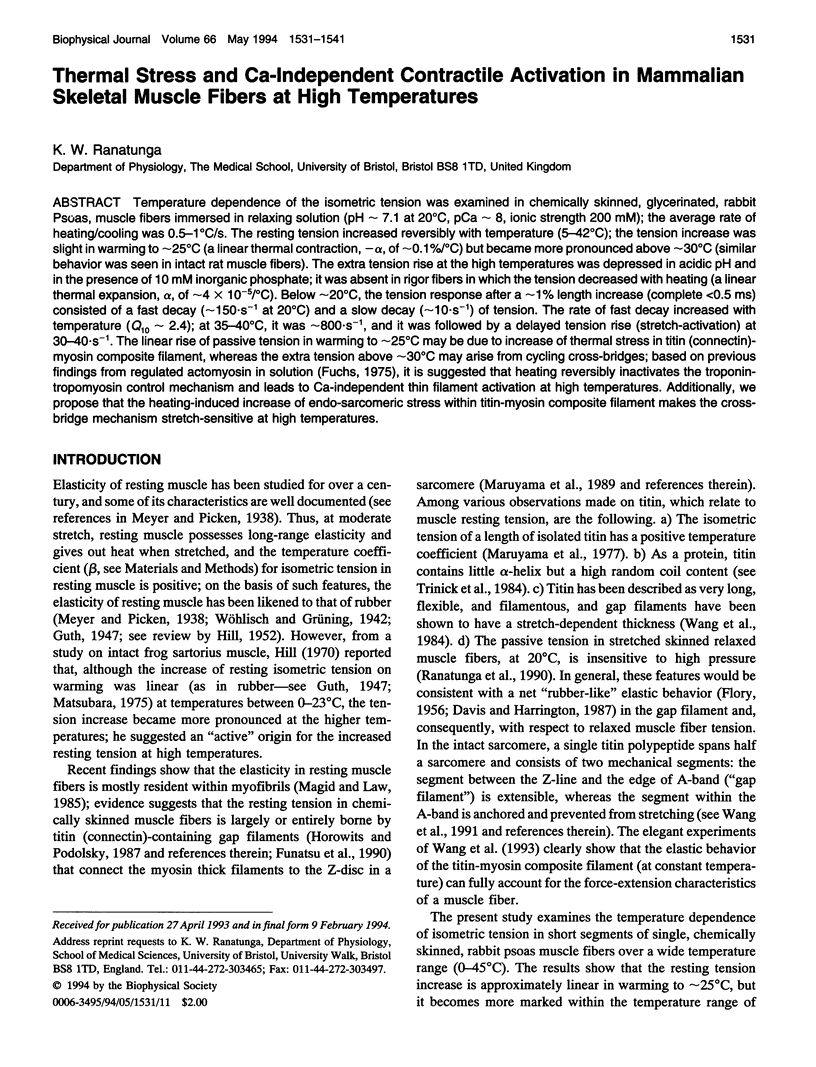
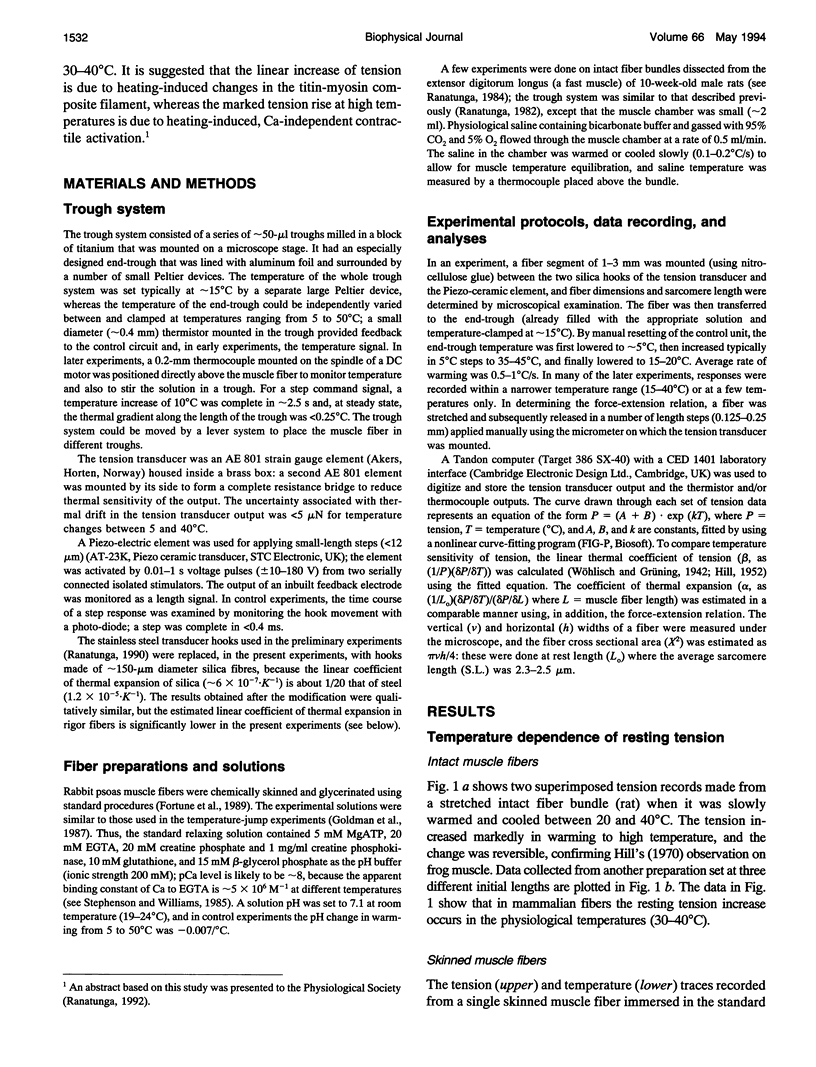
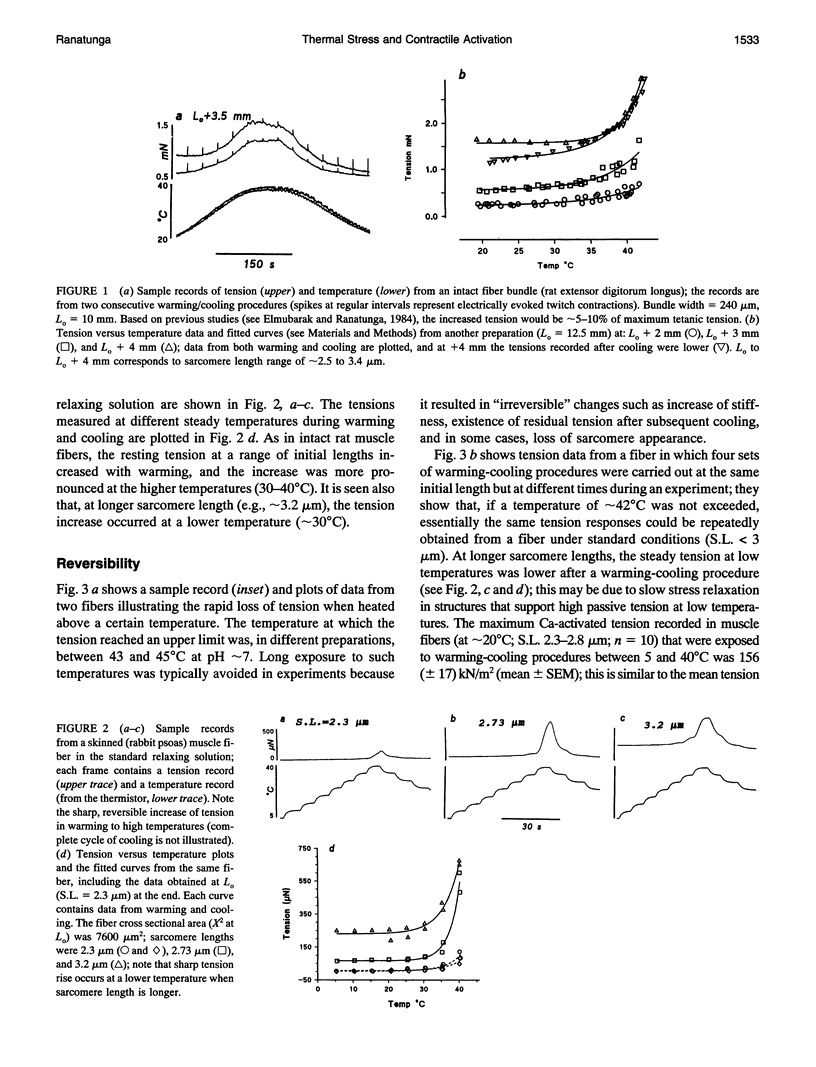
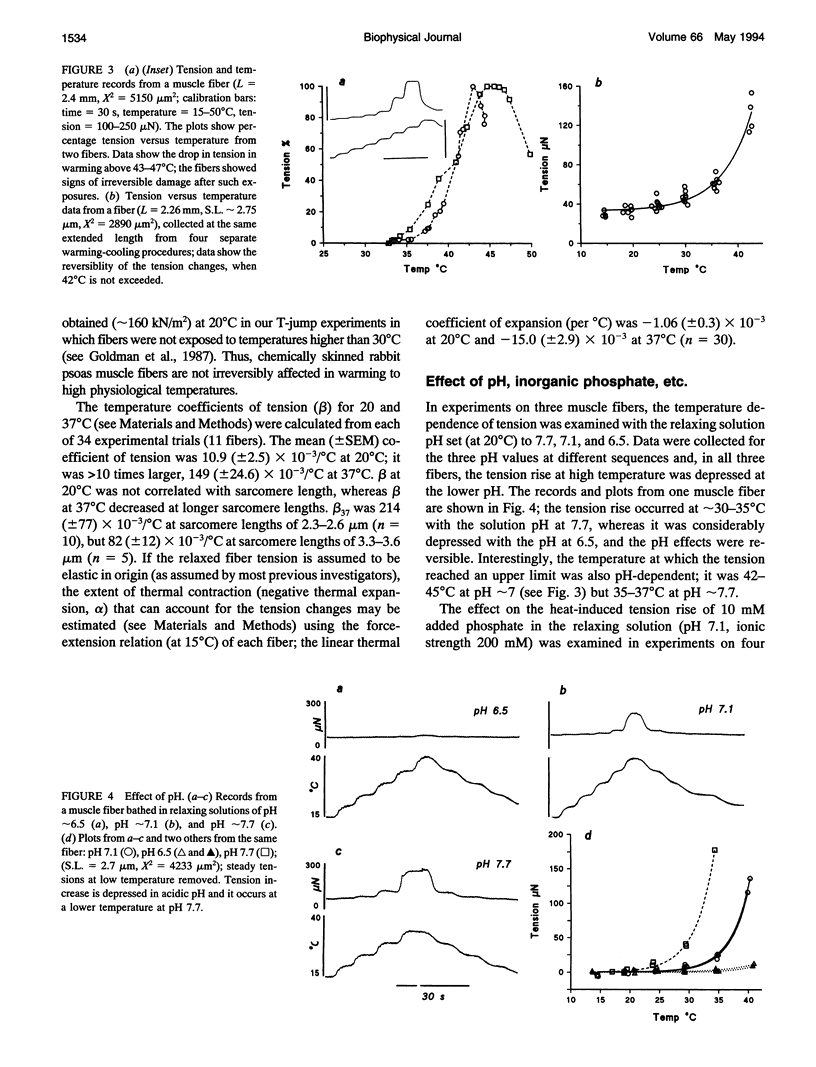
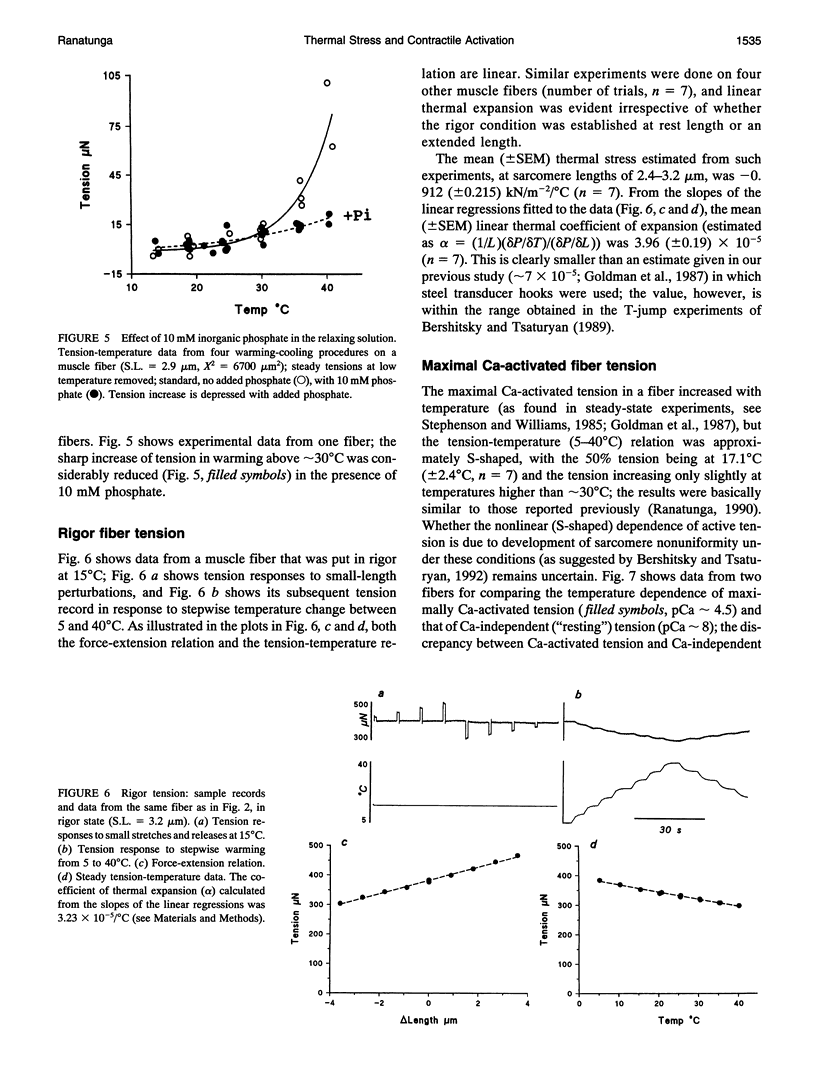
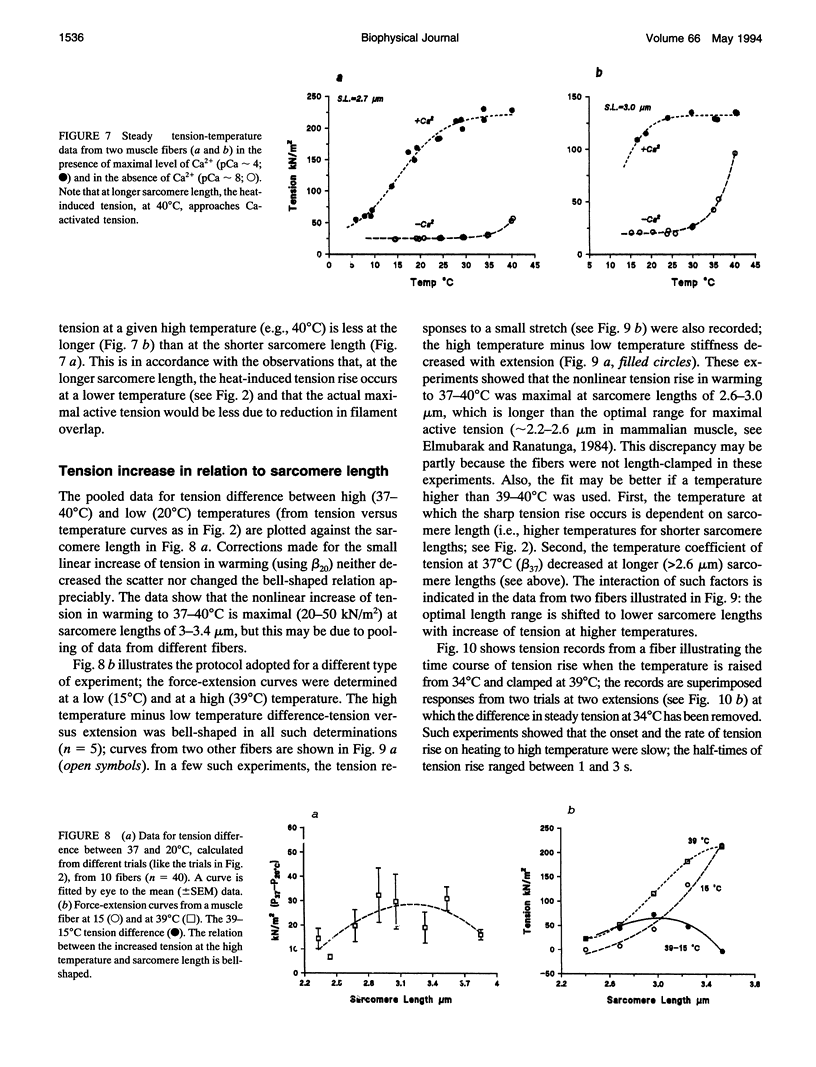
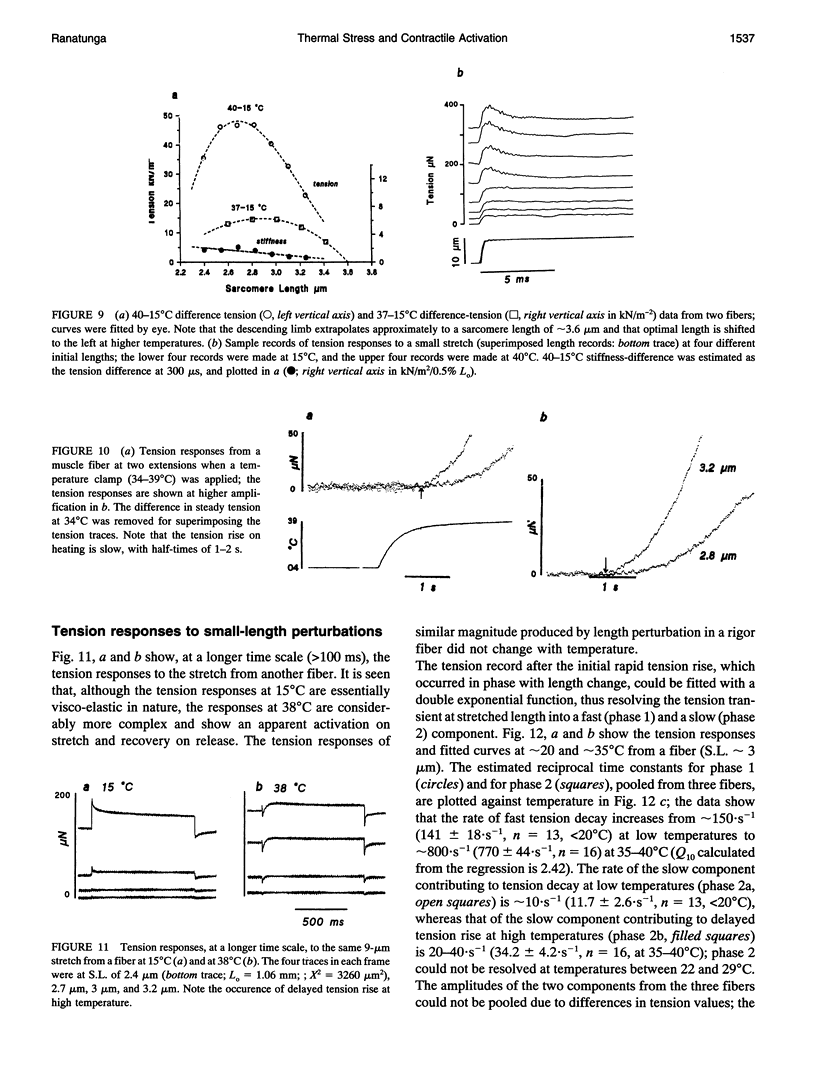
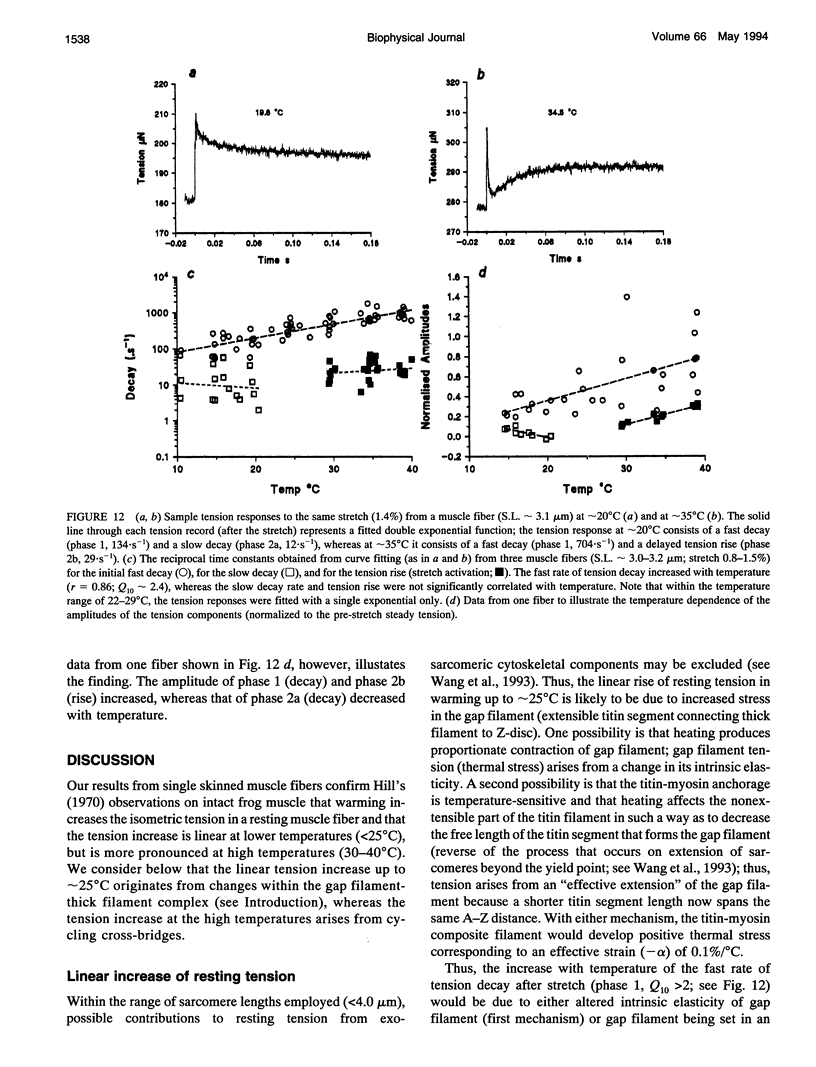
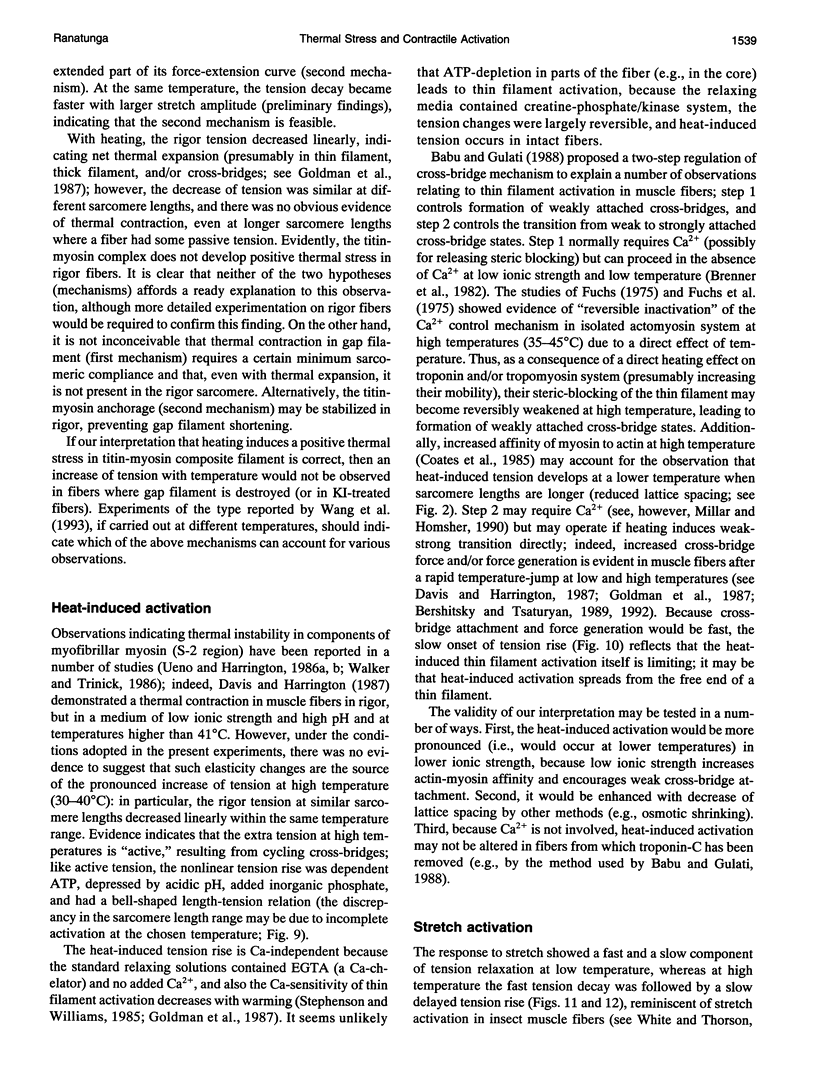
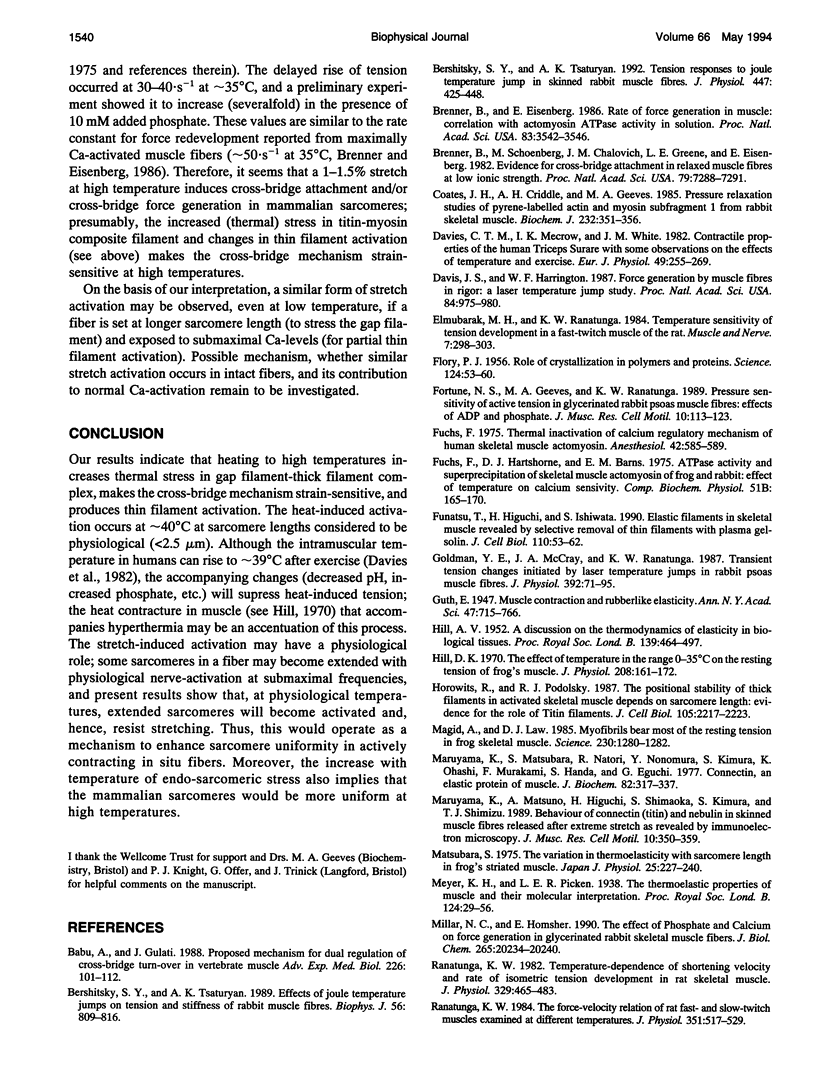
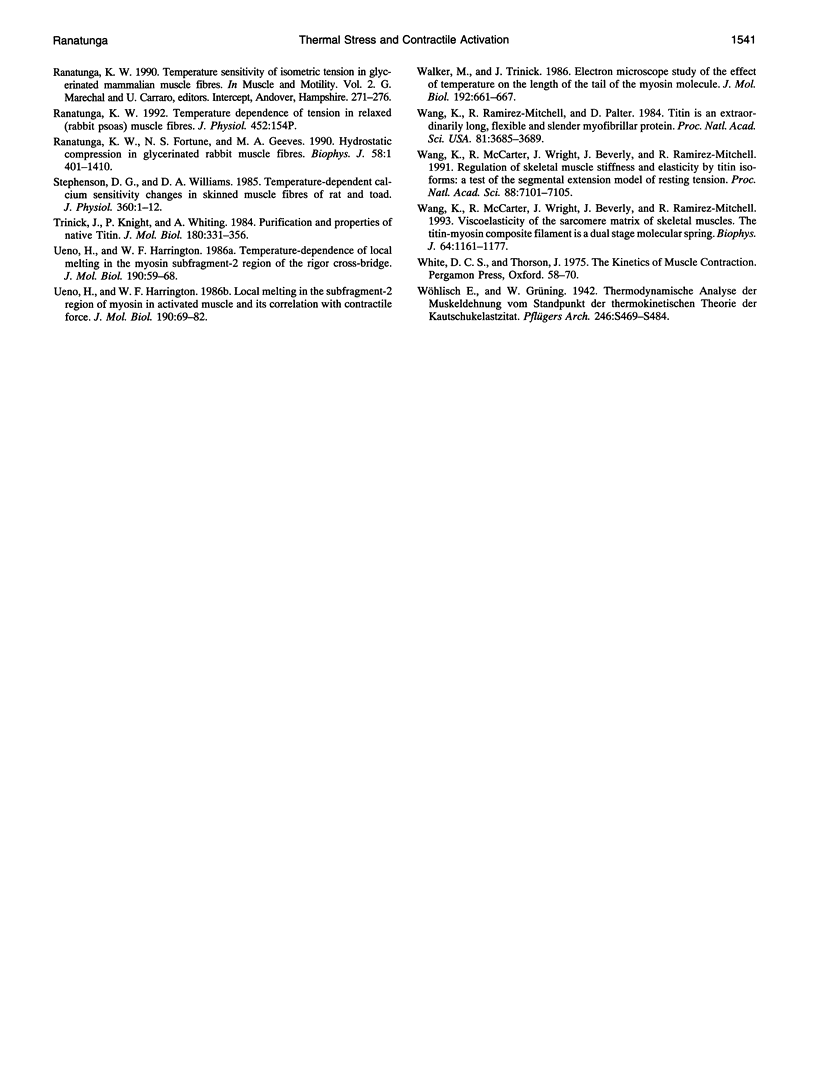
Selected References
These references are in PubMed. This may not be the complete list of references from this article.
- Babu A., Gulati J. Proposed mechanism for dual regulation of cross-bridge turn-over in vertebrate muscle. Adv Exp Med Biol. 1988;226:101–112. [PubMed] [Google Scholar]
- Bershitsky SYu, Tsaturyan A. K. Effect of joule temperature jump on tension and stiffness of skinned rabbit muscle fibers. Biophys J. 1989 Nov;56(5):809–816. doi: 10.1016/S0006-3495(89)82727-4. [DOI] [PMC free article] [PubMed] [Google Scholar]
- Brenner B., Eisenberg E. Rate of force generation in muscle: correlation with actomyosin ATPase activity in solution. Proc Natl Acad Sci U S A. 1986 May;83(10):3542–3546. doi: 10.1073/pnas.83.10.3542. [DOI] [PMC free article] [PubMed] [Google Scholar]
- Brenner B., Schoenberg M., Chalovich J. M., Greene L. E., Eisenberg E. Evidence for cross-bridge attachment in relaxed muscle at low ionic strength. Proc Natl Acad Sci U S A. 1982 Dec;79(23):7288–7291. doi: 10.1073/pnas.79.23.7288. [DOI] [PMC free article] [PubMed] [Google Scholar]
- Coates J. H., Criddle A. H., Geeves M. A. Pressure-relaxation studies of pyrene-labelled actin and myosin subfragment 1 from rabbit skeletal muscle. Evidence for two states of acto-subfragment 1. Biochem J. 1985 Dec 1;232(2):351–356. doi: 10.1042/bj2320351. [DOI] [PMC free article] [PubMed] [Google Scholar]
- Davies C. T., Mecrow I. K., White M. J. Contractile properties of the human triceps surae with some observations on the effects of temperature and exercise. Eur J Appl Physiol Occup Physiol. 1982;49(2):255–269. doi: 10.1007/BF02334074. [DOI] [PubMed] [Google Scholar]
- Davis J. S., Harrington W. F. Force generation by muscle fibers in rigor: a laser temperature-jump study. Proc Natl Acad Sci U S A. 1987 Feb;84(4):975–979. doi: 10.1073/pnas.84.4.975. [DOI] [PMC free article] [PubMed] [Google Scholar]
- Elmubarak M. H., Ranatunga K. W. Temperature sensitivity of tension development in a fast-twitch muscle of the rat. Muscle Nerve. 1984 May;7(4):298–303. doi: 10.1002/mus.880070408. [DOI] [PubMed] [Google Scholar]
- Flory P. J. Role of Crystallization in Polymers and Proteins. Science. 1956 Jul 13;124(3211):53–60. doi: 10.1126/science.124.3211.53. [DOI] [PubMed] [Google Scholar]
- Fortune N. S., Geeves M. A., Ranatunga K. W. Pressure sensitivity of active tension in glycerinated rabbit psoas muscle fibres: effects of ADP and phosphate. J Muscle Res Cell Motil. 1989 Apr;10(2):113–123. doi: 10.1007/BF01739967. [DOI] [PubMed] [Google Scholar]
- Fuchs F., Hartshorne D. J., Barns E. M. ATPase activity and superprecipitation of skeletal muscle actomyosin of frog and rabbit: effect of temperature on calcium sensitivity. Comp Biochem Physiol B. 1975 Jun 15;51(2):165–170. doi: 10.1016/0305-0491(75)90201-1. [DOI] [PubMed] [Google Scholar]
- Fuchs F. Thermal inactivation of the calcium regulatory mechanism of human skeletal muscle actomyosin: a possible contributing factor in the rigidity of malignant hyperthermia. Anesthesiology. 1975 May;42(5):584–589. doi: 10.1097/00000542-197505000-00014. [DOI] [PubMed] [Google Scholar]
- Funatsu T., Higuchi H., Ishiwata S. Elastic filaments in skeletal muscle revealed by selective removal of thin filaments with plasma gelsolin. J Cell Biol. 1990 Jan;110(1):53–62. doi: 10.1083/jcb.110.1.53. [DOI] [PMC free article] [PubMed] [Google Scholar]
- Goldman Y. E., McCray J. A., Ranatunga K. W. Transient tension changes initiated by laser temperature jumps in rabbit psoas muscle fibres. J Physiol. 1987 Nov;392:71–95. doi: 10.1113/jphysiol.1987.sp016770. [DOI] [PMC free article] [PubMed] [Google Scholar]
- HILL A. V. The thermodynamics of elasticity in resting striated muscle. Proc R Soc Lond B Biol Sci. 1952 Jul 10;139(897):464–passim. doi: 10.1098/rspb.1952.0024. [DOI] [PubMed] [Google Scholar]
- Horowits R., Podolsky R. J. The positional stability of thick filaments in activated skeletal muscle depends on sarcomere length: evidence for the role of titin filaments. J Cell Biol. 1987 Nov;105(5):2217–2223. doi: 10.1083/jcb.105.5.2217. [DOI] [PMC free article] [PubMed] [Google Scholar]
- Magid A., Law D. J. Myofibrils bear most of the resting tension in frog skeletal muscle. Science. 1985 Dec 13;230(4731):1280–1282. doi: 10.1126/science.4071053. [DOI] [PubMed] [Google Scholar]
- Maruyama K., Matsubara S., Natori R., Nonomura Y., Kimura S. Connectin, an elastic protein of muscle. Characterization and Function. J Biochem. 1977 Aug;82(2):317–337. [PubMed] [Google Scholar]
- Maruyama K., Matsuno A., Higuchi H., Shimaoka S., Kimura S., Shimizu T. Behaviour of connectin (titin) and nebulin in skinned muscle fibres released after extreme stretch as revealed by immunoelectron microscopy. J Muscle Res Cell Motil. 1989 Oct;10(5):350–359. doi: 10.1007/BF01758431. [DOI] [PubMed] [Google Scholar]
- Matsubara S. The variation in thermoelasticity with sarcomere length in frog's striated muscle. Jpn J Physiol. 1975;25(2):227–240. doi: 10.2170/jjphysiol.25.227. [DOI] [PubMed] [Google Scholar]
- Millar N. C., Homsher E. The effect of phosphate and calcium on force generation in glycerinated rabbit skeletal muscle fibers. A steady-state and transient kinetic study. J Biol Chem. 1990 Nov 25;265(33):20234–20240. [PubMed] [Google Scholar]
- Ranatunga K. W., Fortune N. S., Geeves M. A. Hydrostatic compression in glycerinated rabbit muscle fibers. Biophys J. 1990 Dec;58(6):1401–1410. doi: 10.1016/S0006-3495(90)82486-3. [DOI] [PMC free article] [PubMed] [Google Scholar]
- Ranatunga K. W. Temperature-dependence of shortening velocity and rate of isometric tension development in rat skeletal muscle. J Physiol. 1982 Aug;329:465–483. doi: 10.1113/jphysiol.1982.sp014314. [DOI] [PMC free article] [PubMed] [Google Scholar]
- Ranatunga K. W. The force-velocity relation of rat fast- and slow-twitch muscles examined at different temperatures. J Physiol. 1984 Jun;351:517–529. doi: 10.1113/jphysiol.1984.sp015260. [DOI] [PMC free article] [PubMed] [Google Scholar]
- Stephenson D. G., Williams D. A. Temperature-dependent calcium sensitivity changes in skinned muscle fibres of rat and toad. J Physiol. 1985 Mar;360:1–12. doi: 10.1113/jphysiol.1985.sp015600. [DOI] [PMC free article] [PubMed] [Google Scholar]
- Trinick J., Knight P., Whiting A. Purification and properties of native titin. J Mol Biol. 1984 Dec 5;180(2):331–356. doi: 10.1016/s0022-2836(84)80007-8. [DOI] [PubMed] [Google Scholar]
- Ueno H., Harrington W. F. Local melting in the subfragment-2 region of myosin in activated muscle and its correlation with contractile force. J Mol Biol. 1986 Jul 5;190(1):69–82. doi: 10.1016/0022-2836(86)90076-8. [DOI] [PubMed] [Google Scholar]
- Ueno H., Harrington W. F. Temperature-dependence of local melting in the myosin subfragment-2 region of the rigor cross-bridge. J Mol Biol. 1986 Jul 5;190(1):59–68. doi: 10.1016/0022-2836(86)90075-6. [DOI] [PubMed] [Google Scholar]
- Walker M., Trinick J. Electron microscope study of the effect of temperature on the length of the tail of the myosin molecule. J Mol Biol. 1986 Dec 5;192(3):661–667. doi: 10.1016/0022-2836(86)90283-4. [DOI] [PubMed] [Google Scholar]
- Wang K., McCarter R., Wright J., Beverly J., Ramirez-Mitchell R. Regulation of skeletal muscle stiffness and elasticity by titin isoforms: a test of the segmental extension model of resting tension. Proc Natl Acad Sci U S A. 1991 Aug 15;88(16):7101–7105. doi: 10.1073/pnas.88.16.7101. [DOI] [PMC free article] [PubMed] [Google Scholar]
- Wang K., McCarter R., Wright J., Beverly J., Ramirez-Mitchell R. Viscoelasticity of the sarcomere matrix of skeletal muscles. The titin-myosin composite filament is a dual-stage molecular spring. Biophys J. 1993 Apr;64(4):1161–1177. doi: 10.1016/S0006-3495(93)81482-6. [DOI] [PMC free article] [PubMed] [Google Scholar]
- Wang K., Ramirez-Mitchell R., Palter D. Titin is an extraordinarily long, flexible, and slender myofibrillar protein. Proc Natl Acad Sci U S A. 1984 Jun;81(12):3685–3689. doi: 10.1073/pnas.81.12.3685. [DOI] [PMC free article] [PubMed] [Google Scholar]