Abstract
Time-resolved, terbium resonance x-ray diffraction experiments have provided the locations of three different high-affinity metal-binding/transport sites on the Ca2+ATPase enzyme in the profile structure of the sarcoplasmic reticulum (SR) membrane. By considering these results in conjunction with the known, moderate-resolution profile structure of the SR membrane (derived from nonresonance x-ray and neutron diffraction studies), it was determined that the three metal-binding sites are located at the "headpiece/stalk" junction in the Ca2+ATPase profile structure, in the "transbilayer" portion of the enzyme profile near the center of the membrane phospholipid bilayer, and at the intravesicular surface of the membrane profile. All three metal-binding sites so identified are simultaneously occupied in the unphosphorylated enzyme conformation. Phosphorylation of the ATPase causes a redistribution of metal density among the sites, resulting in a net movement of metal density toward the intravesicular side of the membrane, i.e., in the direction of calcium active transport. We propose that this redistribution of metal density is caused by changes in the relative binding affinities of the three sites, mediated by local structural changes at the sites resulting from the large-scale (i.e., long-range) changes in the profile structure of the Ca2+ATPase induced by phosphorylation, as reported in an accompanying paper. The implications of these results for the mechanism of calcium active transport by the SR Ca2+ATPase are discussed briefly.
Full text
PDF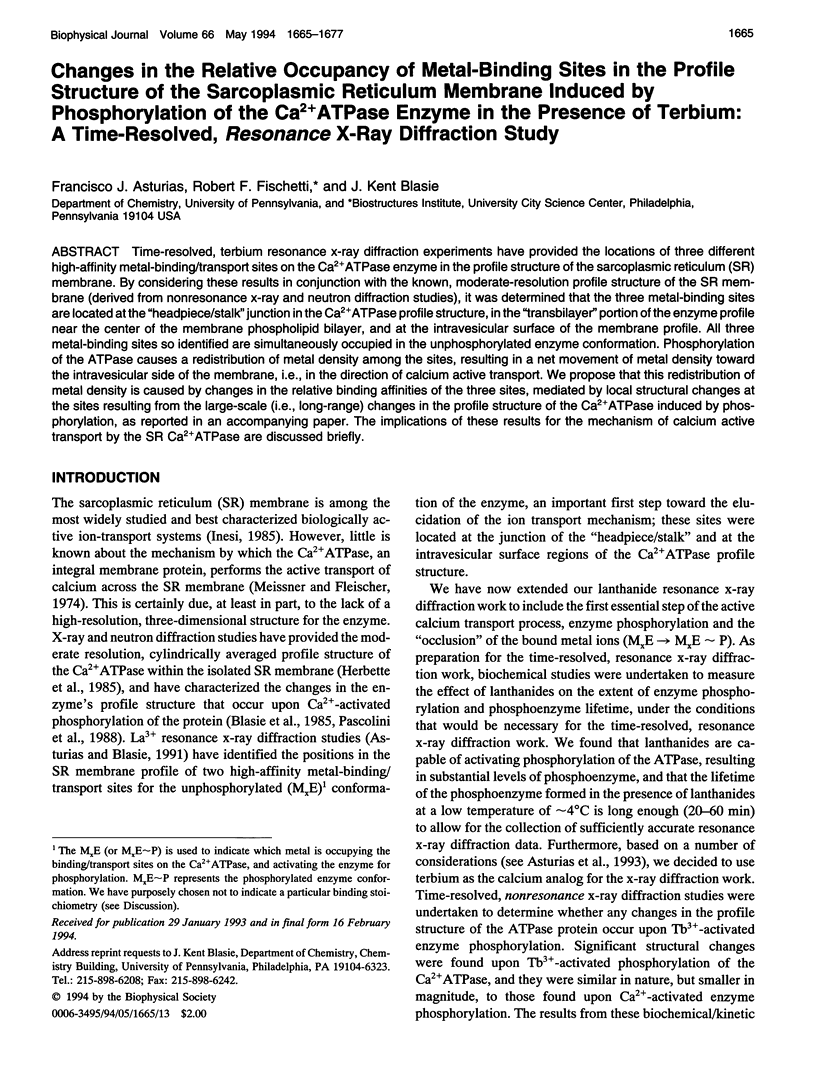
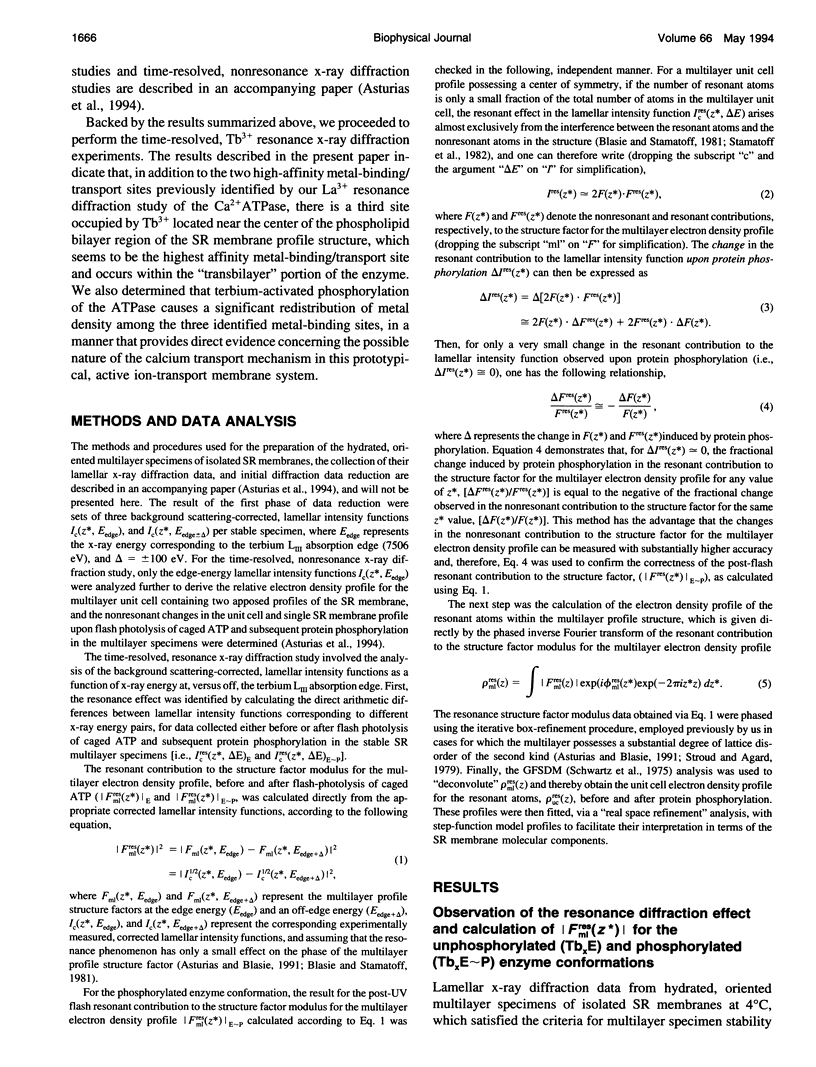
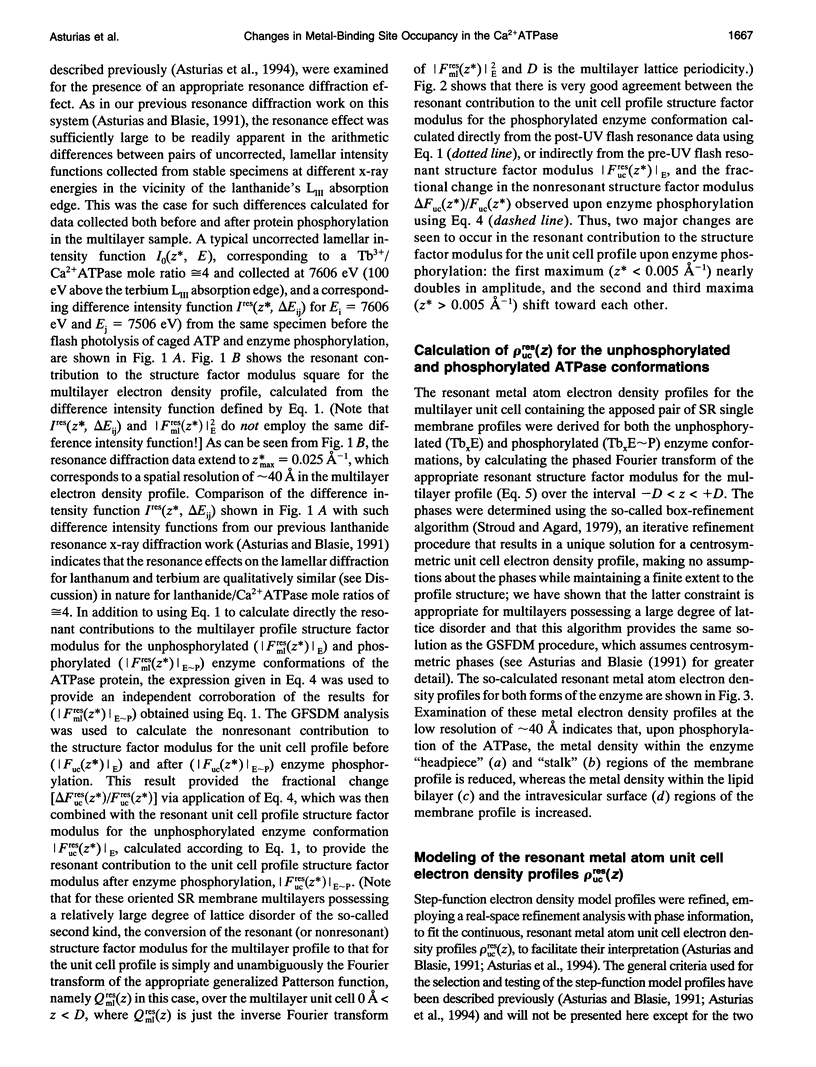
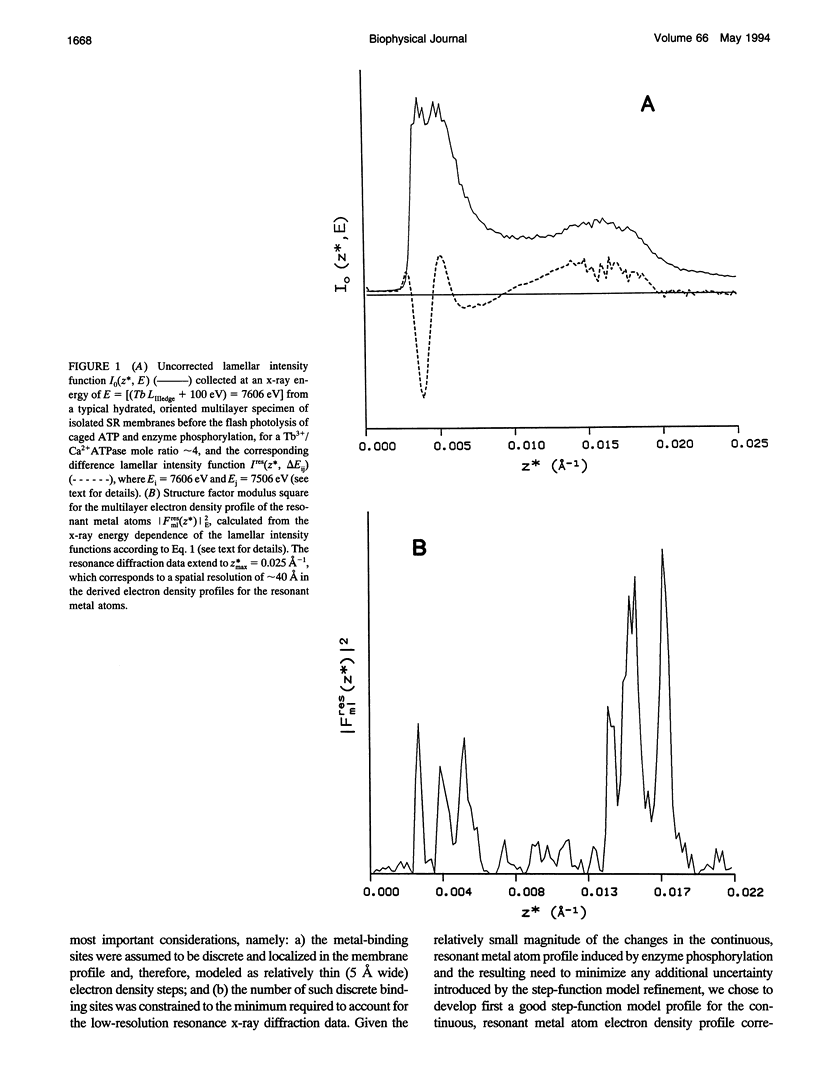
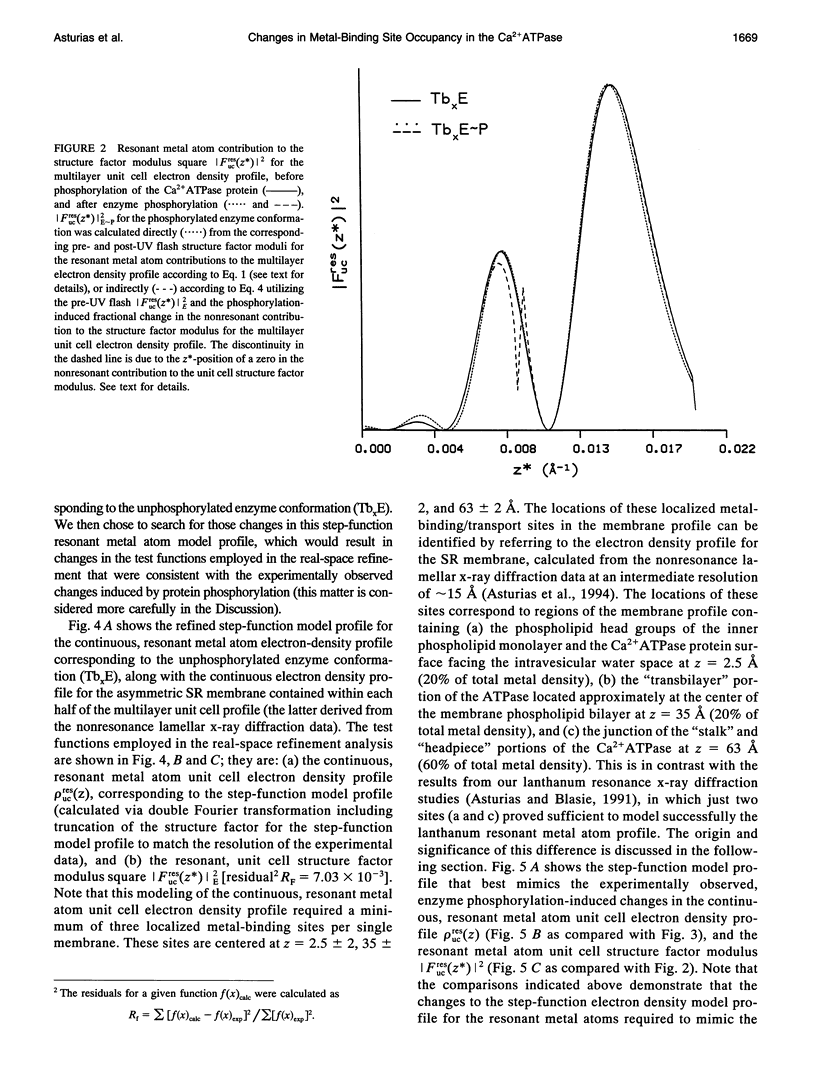
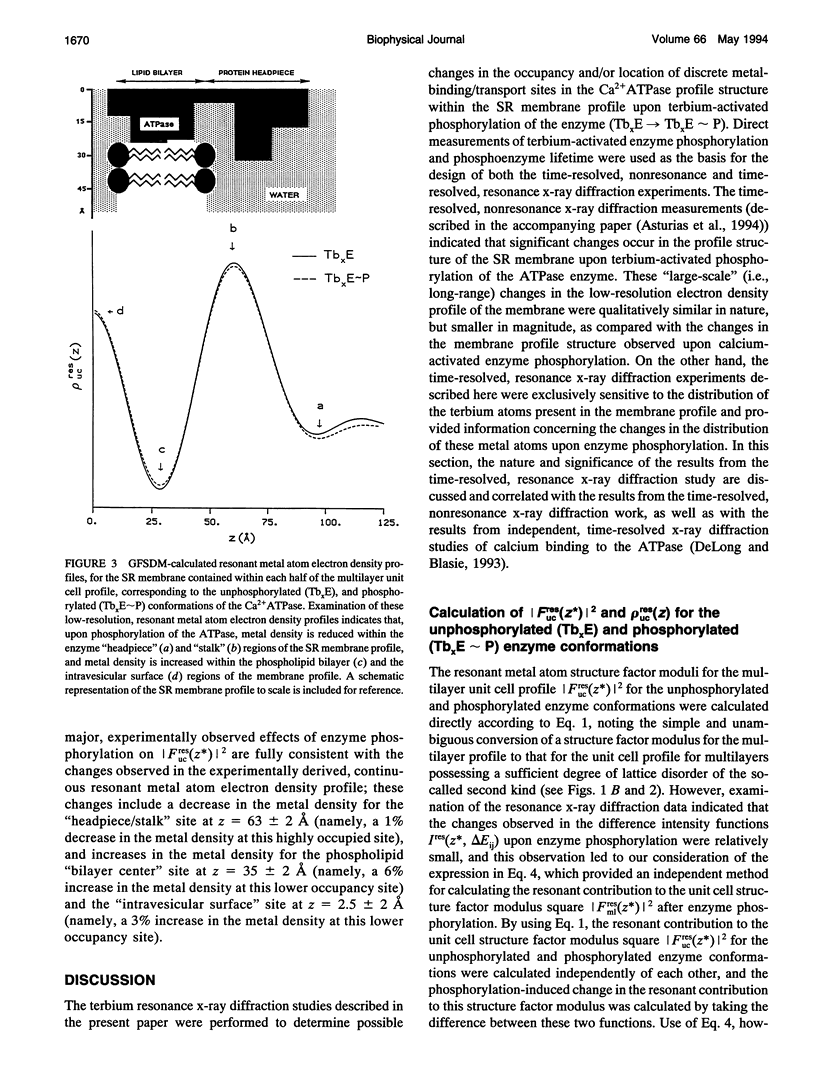
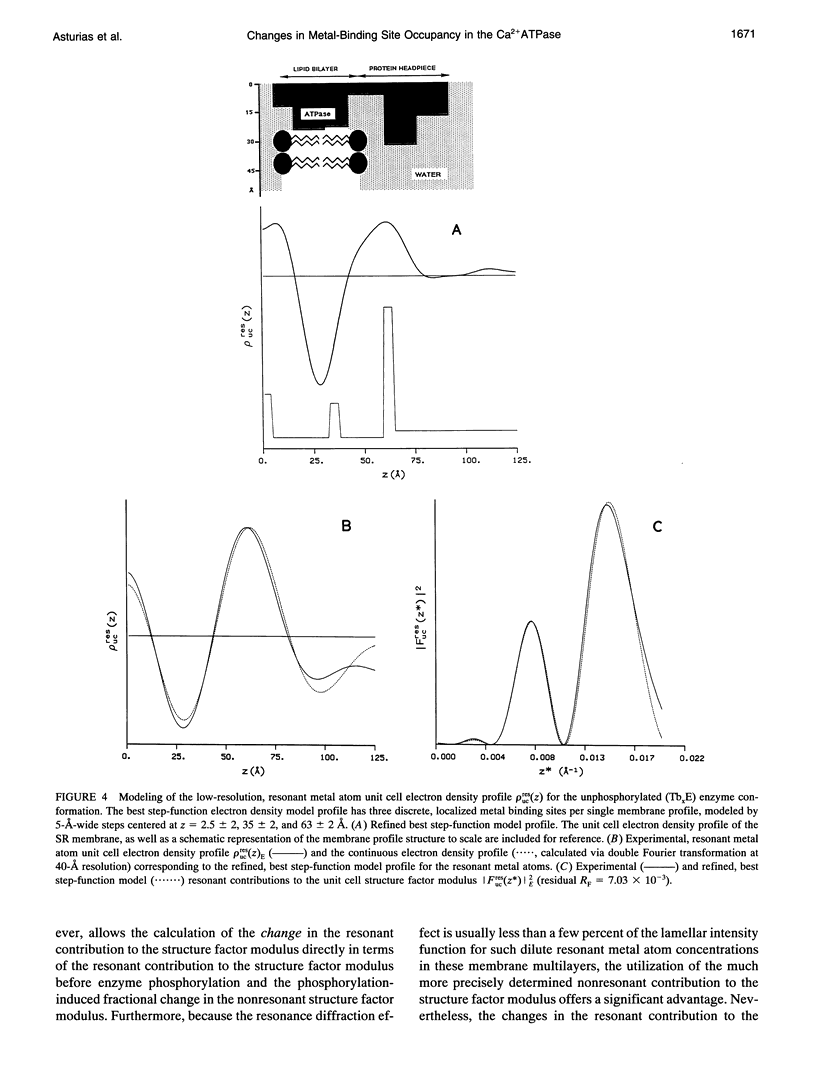
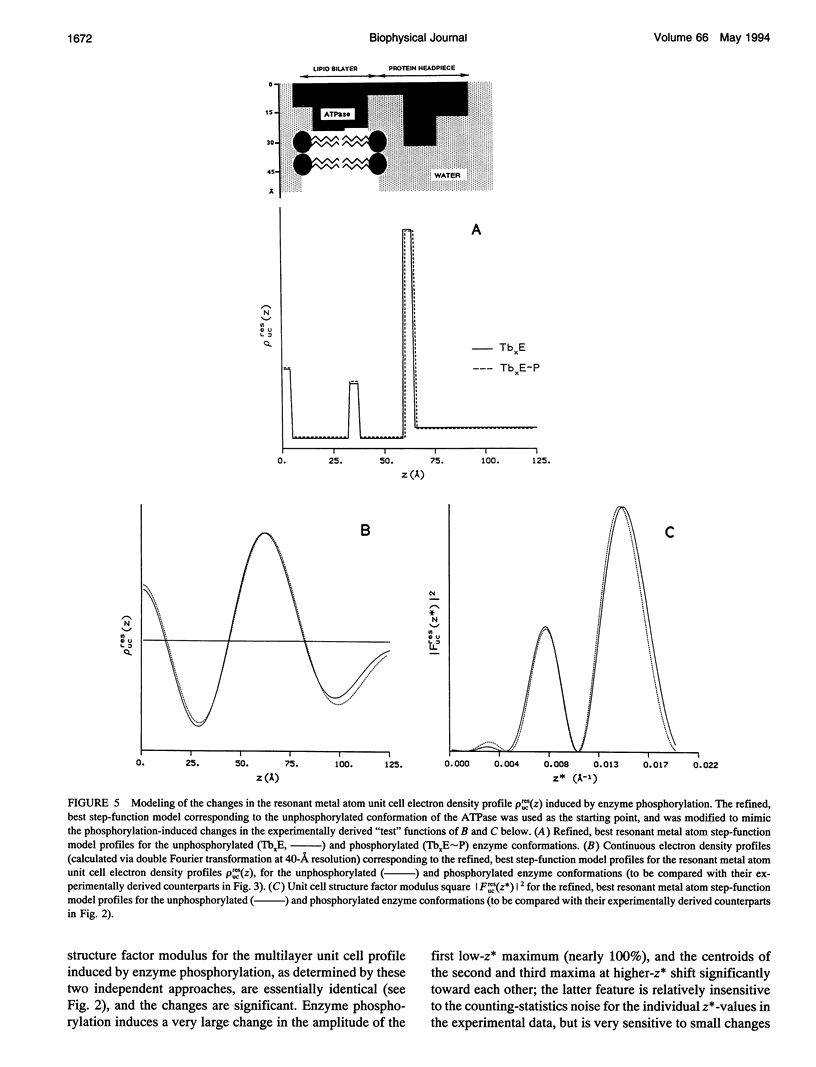
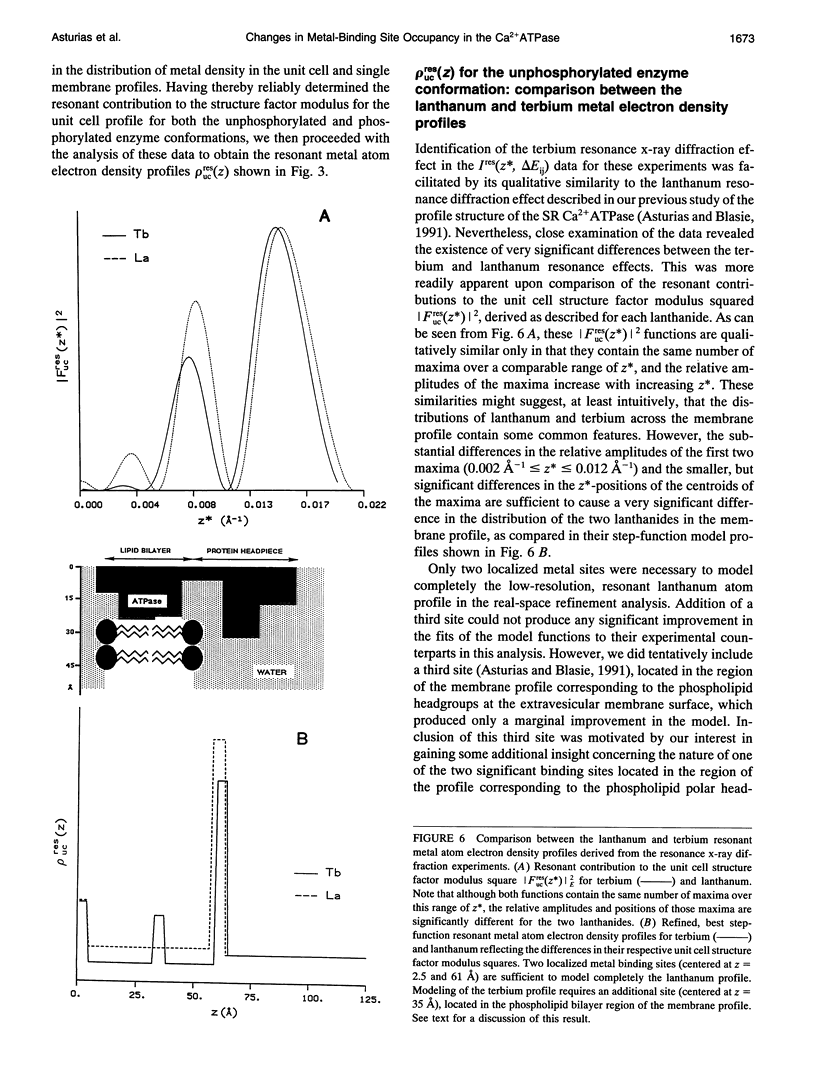
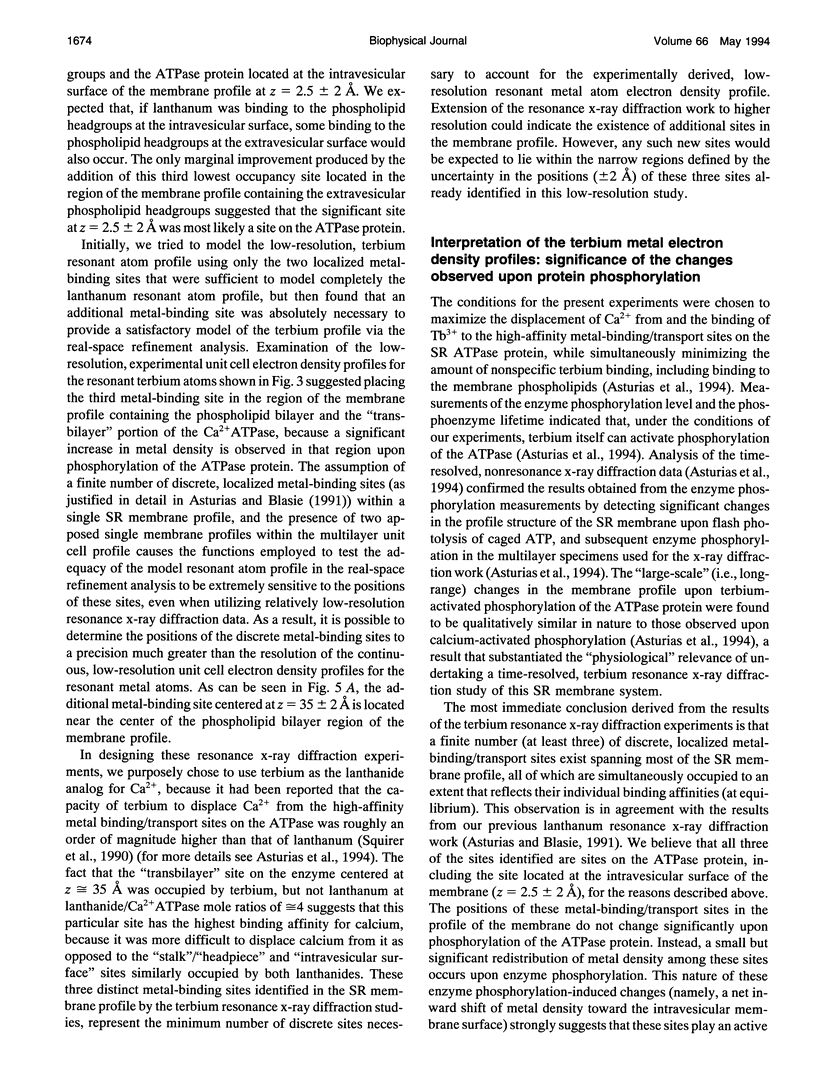
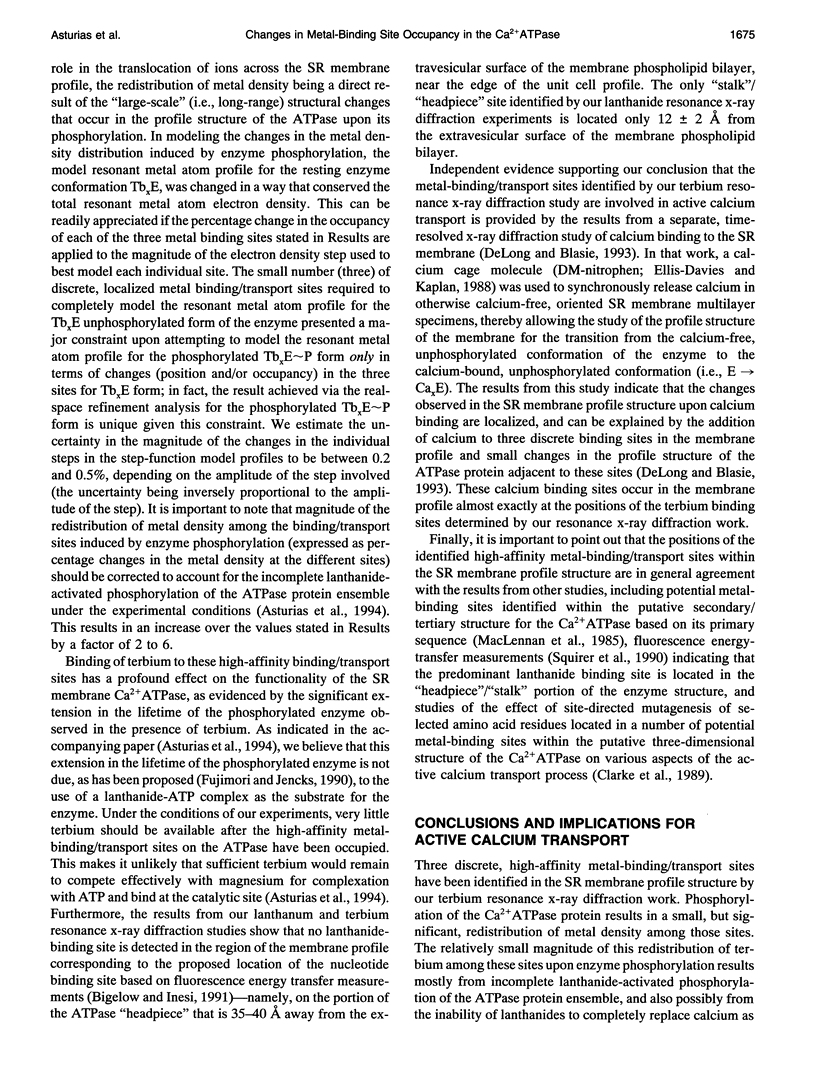
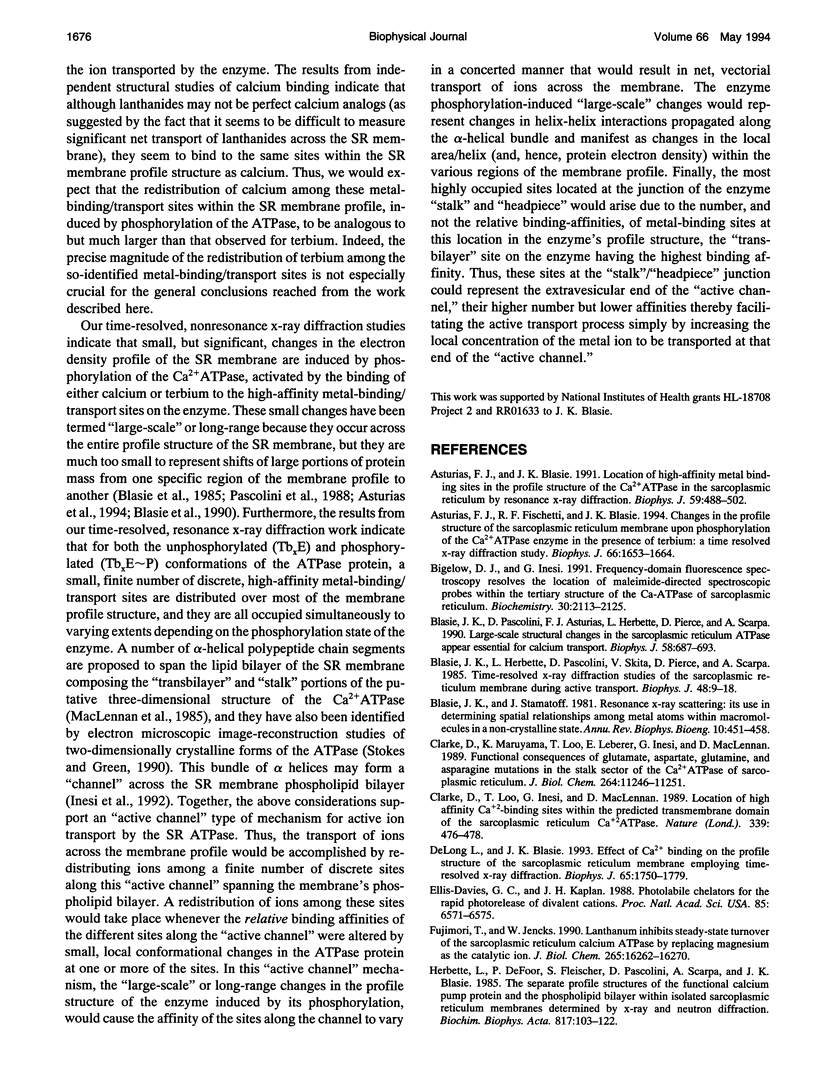
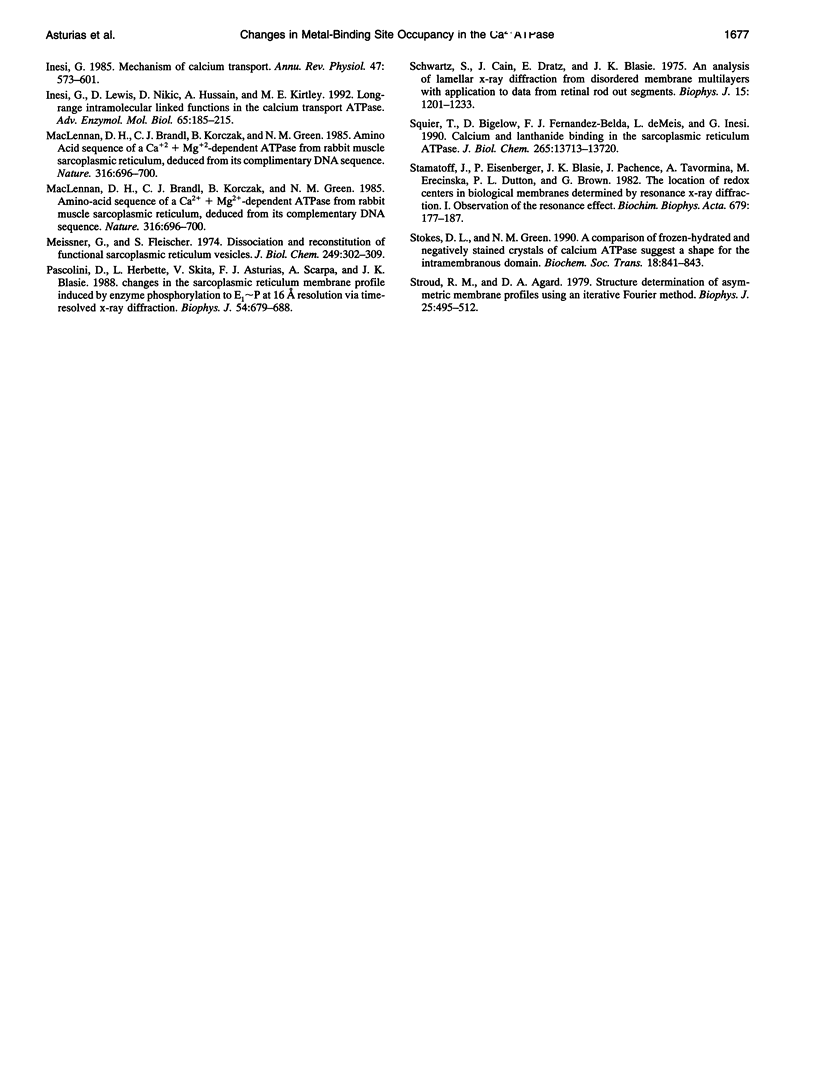
Selected References
These references are in PubMed. This may not be the complete list of references from this article.
- Asturias F. J., Blasie J. K. Location of high-affinity metal binding sites in the profile structure of the Ca+2-ATPase in the sarcoplasmic reticulum by resonance x-ray diffraction. Biophys J. 1991 Feb;59(2):488–502. doi: 10.1016/S0006-3495(91)82242-1. [DOI] [PMC free article] [PubMed] [Google Scholar]
- Asturias F. J., Fischetti R. F., Blasie J. K. Changes in the profile structure of the sarcoplasmic reticulum membrane induced by phosphorylation of the Ca2+ ATPase enzyme in the presence of terbium: a time-resolved x-ray diffraction study. Biophys J. 1994 May;66(5):1653–1664. doi: 10.1016/S0006-3495(94)80957-9. [DOI] [PMC free article] [PubMed] [Google Scholar]
- Bigelow D. J., Inesi G. Frequency-domain fluorescence spectroscopy resolves the location of maleimide-directed spectroscopic probes within the tertiary structure of the Ca-ATPase of sarcoplasmic reticulum. Biochemistry. 1991 Feb 26;30(8):2113–2125. doi: 10.1021/bi00222a016. [DOI] [PubMed] [Google Scholar]
- Blasie J. K., Herbette L. G., Pascolini D., Skita V., Pierce D. H., Scarpa A. Time-resolved x-ray diffraction studies of the sarcoplasmic reticulum membrane during active transport. Biophys J. 1985 Jul;48(1):9–18. doi: 10.1016/S0006-3495(85)83756-5. [DOI] [PMC free article] [PubMed] [Google Scholar]
- Blasie J. K., Pascolini D., Asturias F., Herbette L. G., Pierce D., Scarpa A. Large-scale structural changes in the sarcoplasmic reticulum ATPase appear essential for calcium transport. Biophys J. 1990 Sep;58(3):687–693. doi: 10.1016/S0006-3495(90)82411-5. [DOI] [PMC free article] [PubMed] [Google Scholar]
- Blasie J. K., Stamatoff J. Resonance X-ray scattering: its use in determining spatial relationships among metal atoms within macromolecules in a non-crystalline state. Annu Rev Biophys Bioeng. 1981;10:451–458. doi: 10.1146/annurev.bb.10.060181.002315. [DOI] [PubMed] [Google Scholar]
- Clarke D. M., Loo T. W., Inesi G., MacLennan D. H. Location of high affinity Ca2+-binding sites within the predicted transmembrane domain of the sarcoplasmic reticulum Ca2+-ATPase. Nature. 1989 Jun 8;339(6224):476–478. doi: 10.1038/339476a0. [DOI] [PubMed] [Google Scholar]
- Clarke D. M., Maruyama K., Loo T. W., Leberer E., Inesi G., MacLennan D. H. Functional consequences of glutamate, aspartate, glutamine, and asparagine mutations in the stalk sector of the Ca2+-ATPase of sarcoplasmic reticulum. J Biol Chem. 1989 Jul 5;264(19):11246–11251. [PubMed] [Google Scholar]
- DeLong L. J., Blasie J. K. Effect of Ca2+ binding on the profile structure of the sarcoplasmic reticulum membrane using time-resolved x-ray diffraction. Biophys J. 1993 Jun;64(6):1750–1759. doi: 10.1016/S0006-3495(93)81546-7. [DOI] [PMC free article] [PubMed] [Google Scholar]
- Fujimori T., Jencks W. P. Lanthanum inhibits steady-state turnover of the sarcoplasmic reticulum calcium ATPase by replacing magnesium as the catalytic ion. J Biol Chem. 1990 Sep 25;265(27):16262–16270. [PubMed] [Google Scholar]
- Herbette L., DeFoor P., Fleischer S., Pascolini D., Scarpa A., Blasie J. K. The separate profile structures of the functional calcium pump protein and the phospholipid bilayer within isolated sarcoplasmic reticulum membranes determined by X-ray and neutron diffraction. Biochim Biophys Acta. 1985 Jul 11;817(1):103–122. doi: 10.1016/0005-2736(85)90073-2. [DOI] [PubMed] [Google Scholar]
- Inesi G., Lewis D., Nikic D., Hussain A., Kirtley M. E. Long-range intramolecular linked functions in the calcium transport ATPase. Adv Enzymol Relat Areas Mol Biol. 1992;65:185–215. doi: 10.1002/9780470123119.ch5. [DOI] [PubMed] [Google Scholar]
- Inesi G. Mechanism of calcium transport. Annu Rev Physiol. 1985;47:573–601. doi: 10.1146/annurev.ph.47.030185.003041. [DOI] [PubMed] [Google Scholar]
- Kaplan J. H., Ellis-Davies G. C. Photolabile chelators for the rapid photorelease of divalent cations. Proc Natl Acad Sci U S A. 1988 Sep;85(17):6571–6575. doi: 10.1073/pnas.85.17.6571. [DOI] [PMC free article] [PubMed] [Google Scholar]
- MacLennan D. H., Brandl C. J., Korczak B., Green N. M. Amino-acid sequence of a Ca2+ + Mg2+-dependent ATPase from rabbit muscle sarcoplasmic reticulum, deduced from its complementary DNA sequence. Nature. 1985 Aug 22;316(6030):696–700. doi: 10.1038/316696a0. [DOI] [PubMed] [Google Scholar]
- Meissner G., Fleischer S. Dissociation and reconstitution of functional sarcoplasmic reticulum vesicles. J Biol Chem. 1974 Jan 10;249(1):302–309. [PubMed] [Google Scholar]
- Pascolini D., Herbette L. G., Skita V., Asturias F., Scarpa A., Blasie J. K. Changes in the sarcoplasmic reticulum membrane profile induced by enzyme phosphorylation to E1 approximately P at 16 A resolution via time-resolved x-ray diffraction. Biophys J. 1988 Oct;54(4):679–687. doi: 10.1016/S0006-3495(88)83003-0. [DOI] [PMC free article] [PubMed] [Google Scholar]
- Schwartz S., Cain J. E., Dratz E. A., Blasie J. K. An analysis of lamellar x-ray diffraction from disordered membrane multilayers with application to data from retinal rod outer segments. Biophys J. 1975 Dec;15(12):1201–1233. doi: 10.1016/S0006-3495(75)85895-4. [DOI] [PMC free article] [PubMed] [Google Scholar]
- Squier T. C., Bigelow D. J., Fernandez-Belda F. J., deMeis L., Inesi G. Calcium and lanthanide binding in the sarcoplasmic reticulum ATPase. J Biol Chem. 1990 Aug 15;265(23):13713–13720. [PubMed] [Google Scholar]
- Stamatoff J., Eisenberger P., Blasie J. K., Pachence J. M., Tavormina A., Erecinska M., Dutton P. L., Brown G. The location of redox centers in biological membranes determined by resonance x-ray diffraction. I. Observation of the resonance effect. Biochim Biophys Acta. 1982 Feb 17;679(2):177–187. doi: 10.1016/0005-2728(82)90289-4. [DOI] [PubMed] [Google Scholar]
- Stokes D. L., Green N. M. Comparison of frozen-hydrated and negatively stained crystals of Ca-ATPase suggests a shape for the intramembranous domain. Biochem Soc Trans. 1990 Oct;18(5):841–843. doi: 10.1042/bst0180841. [DOI] [PubMed] [Google Scholar]
- Stroud R. M., Agard D. A. Structure determination of asymmetric membrane profiles using an iterative Fourier method. Biophys J. 1979 Mar;25(3):495–512. doi: 10.1016/S0006-3495(79)85319-9. [DOI] [PMC free article] [PubMed] [Google Scholar]