Abstract
1. The ionic mechanisms involved in block of conduction of action potentials following high frequency stimulation were studied in a branching axon of the lobster Panulirus penicillatus. 2. A 2-3 mM increase in extracellular K concentration (normal concentration 12 mM) produced block of conduction into both daughter branches. 3. While conduction block induced by high frequency stimulation occurs first into the large daughter branch and only later into the smaller one, propagation into both branches is blocked simultaneously by increased extracellular K concentration. 4. Increasing extracellular K by 2-3 mM resulted in membrane depolarization, reduction in membrane resistance and reduced excitability. The latter two effects were larger than expected from the small depolarization. It appears that increase of extracellular K has direct effects on membrane excitability. 5. It is suggested that block of conduction after high frequency stimulation results from accumulation of K in the extracellular space. However, in order to account for differential conduction block in the two branches one must assume differential buildup of extracellular K concentration around the two branches during high frequency stimulation. 6. Ultrastructural studies using La and horseradish peroxidase as extracellular markers show that the space around the two branches is similar and is open to the extracellular space. Therefore differences in periaxonal volume cannot account for differential buildup of K around the two branches. 7. It is demonstrated that the lobster axon has a Na+/K+ electrogenic pump. After blocking this pump with ouabain, stimulation at high frequency resulted in a conduction block in the two branches almost at the same time. 8. Injection of Ca2+ intracellularly into the thick branch prevents or delays the appearance of conduction block after high frequency stimulation. 9. A mechanism based on these findings is suggested to explain the differential conduction block seen after high frequency stimulation in a branching axon with almost ideal impedance matching.
Full text
PDF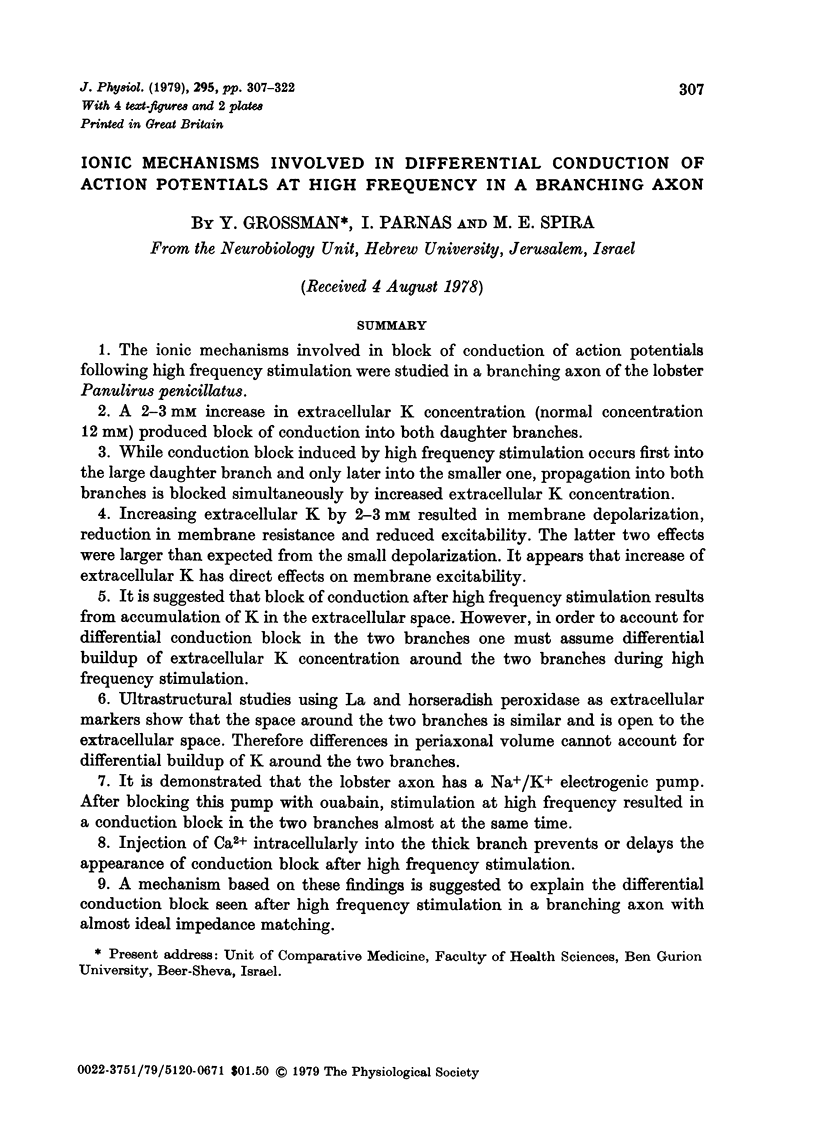
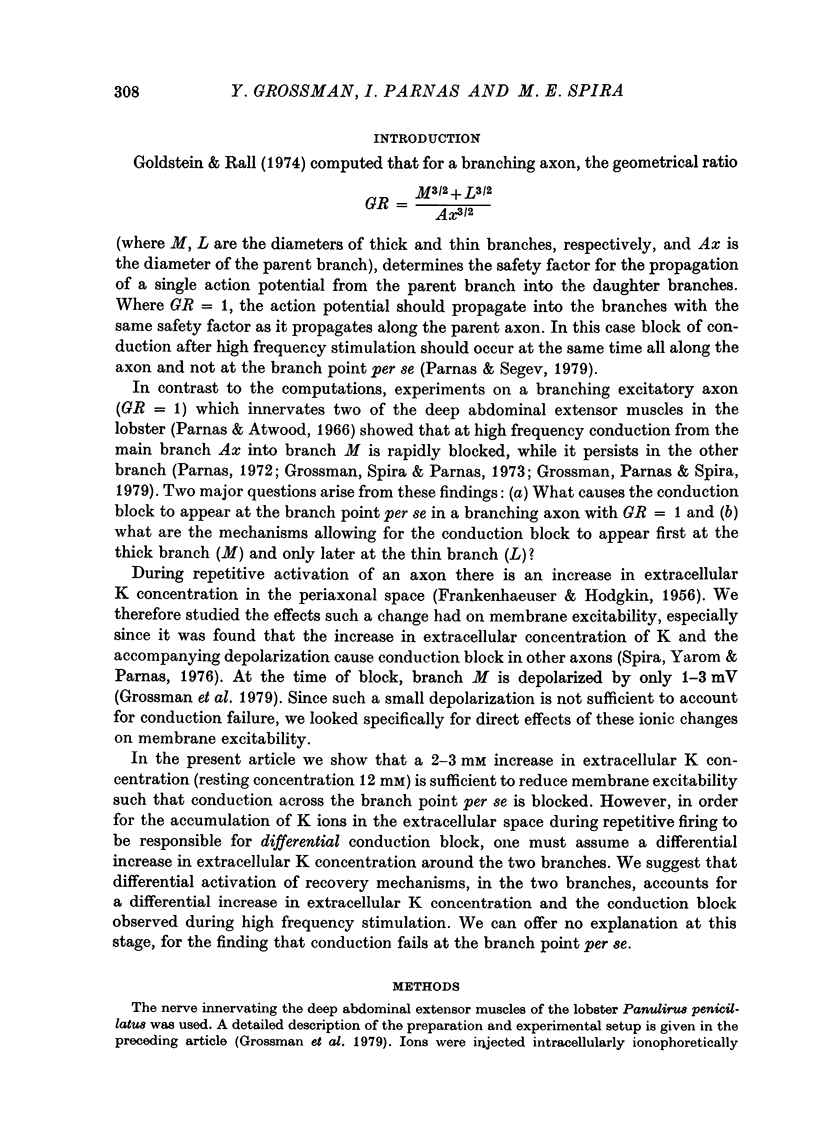
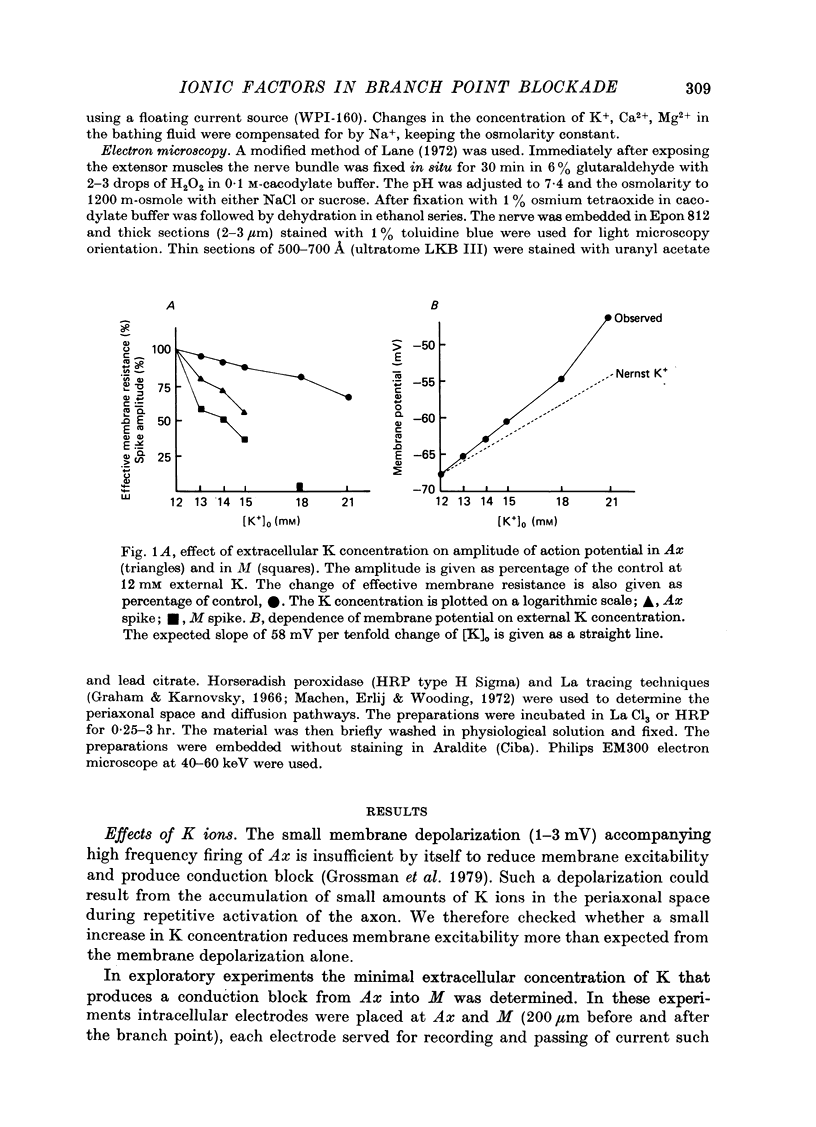
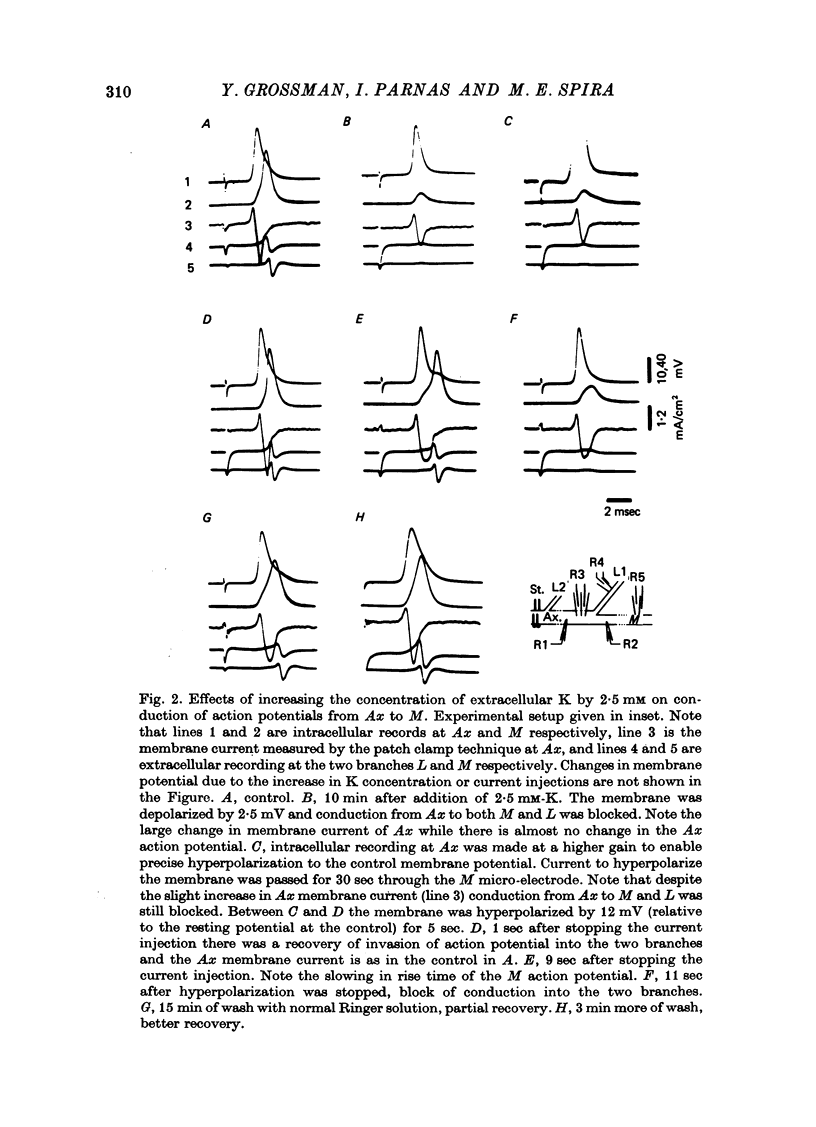
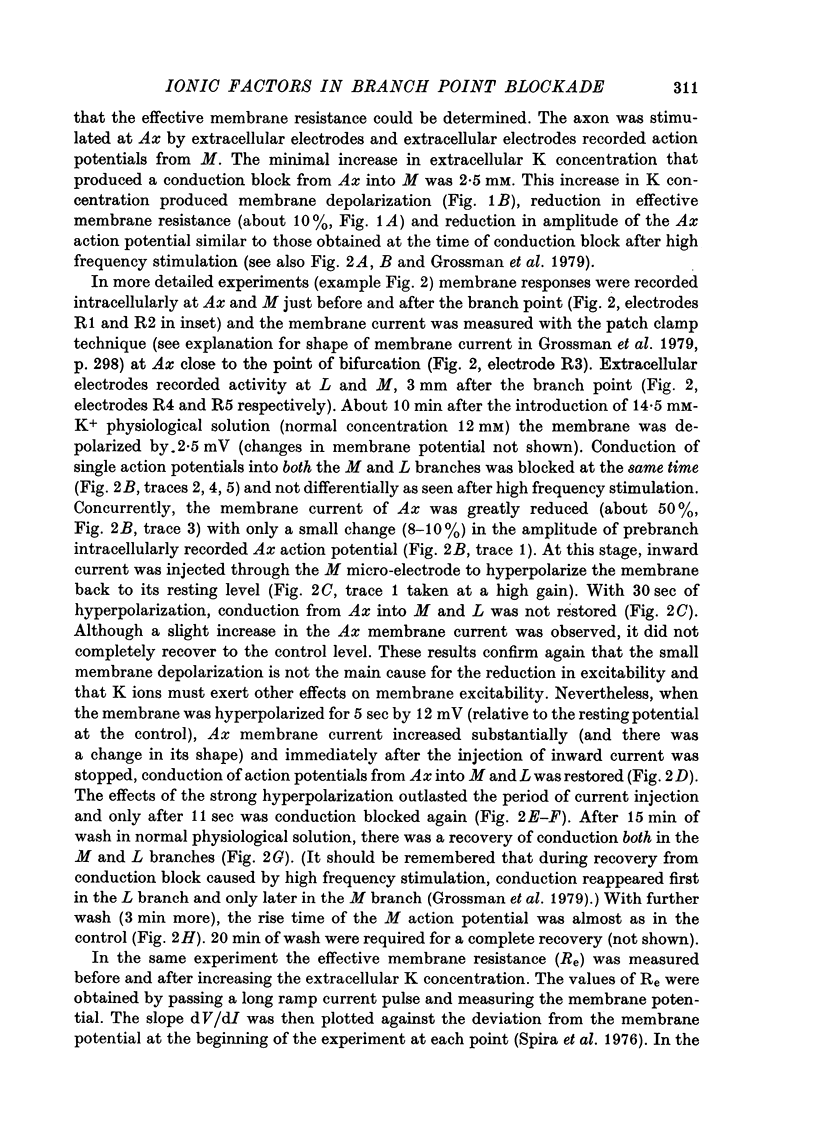
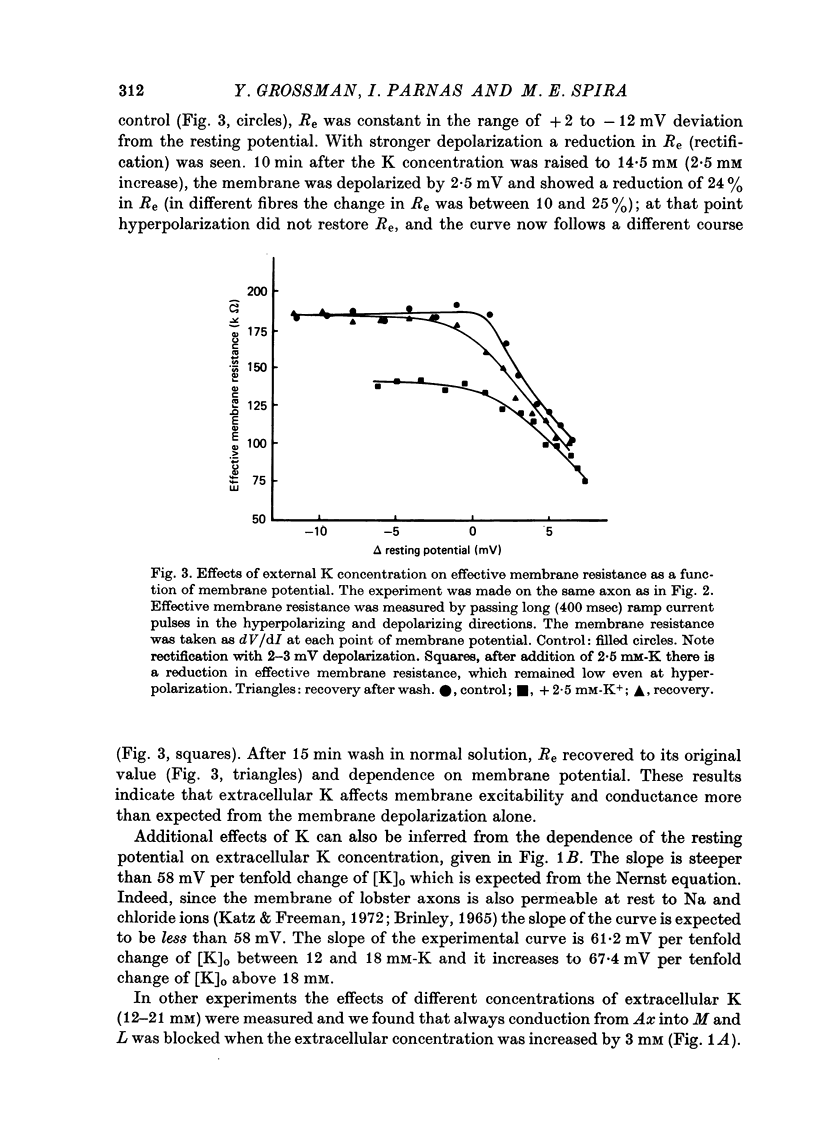
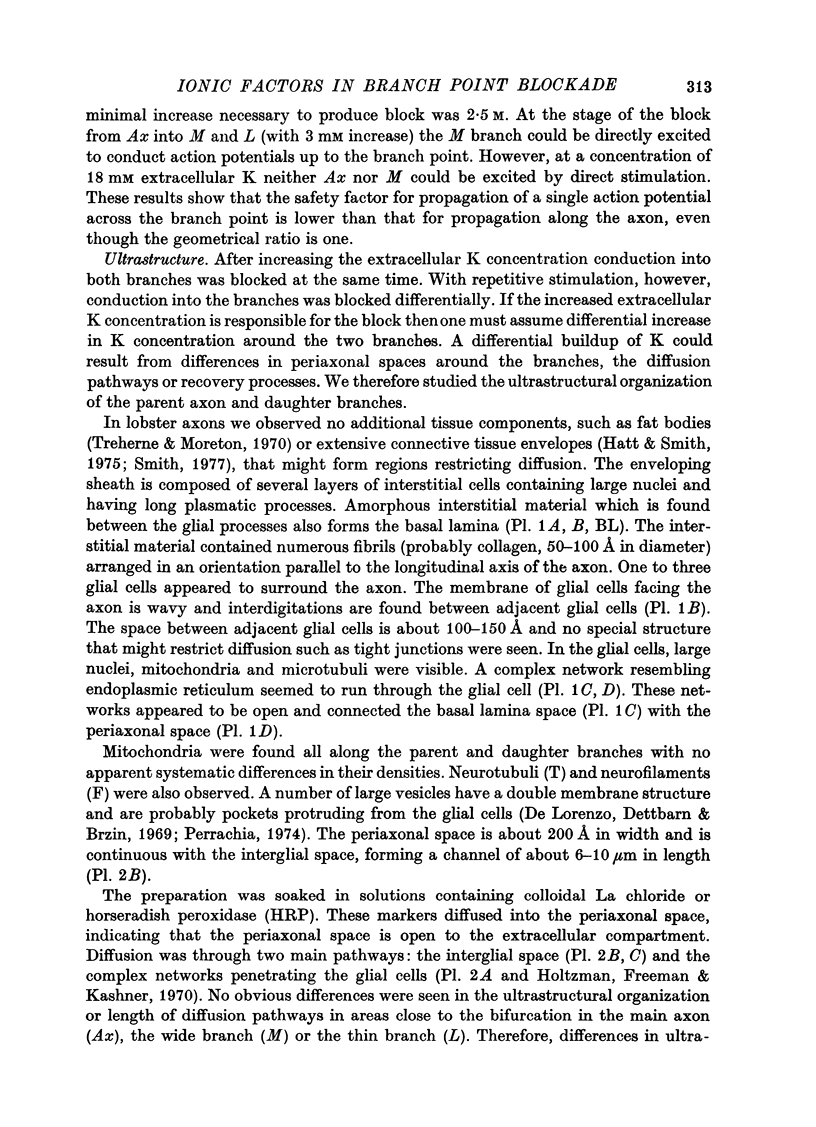
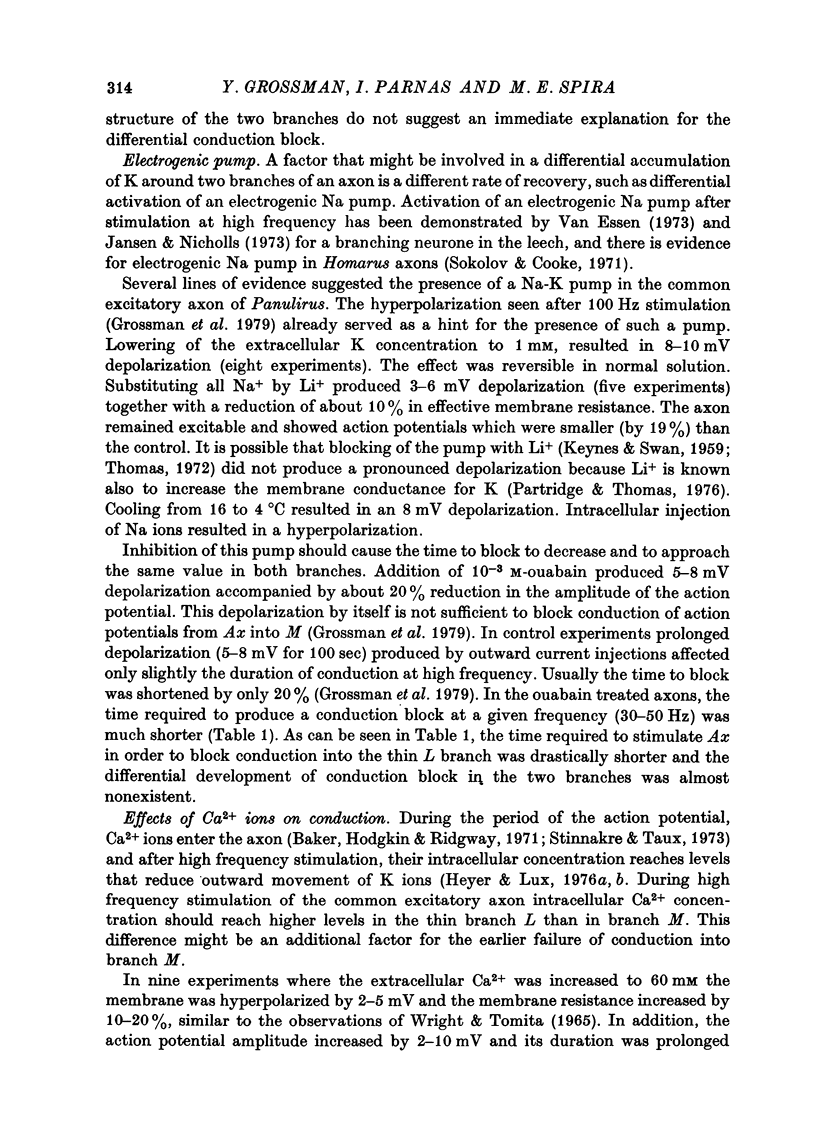
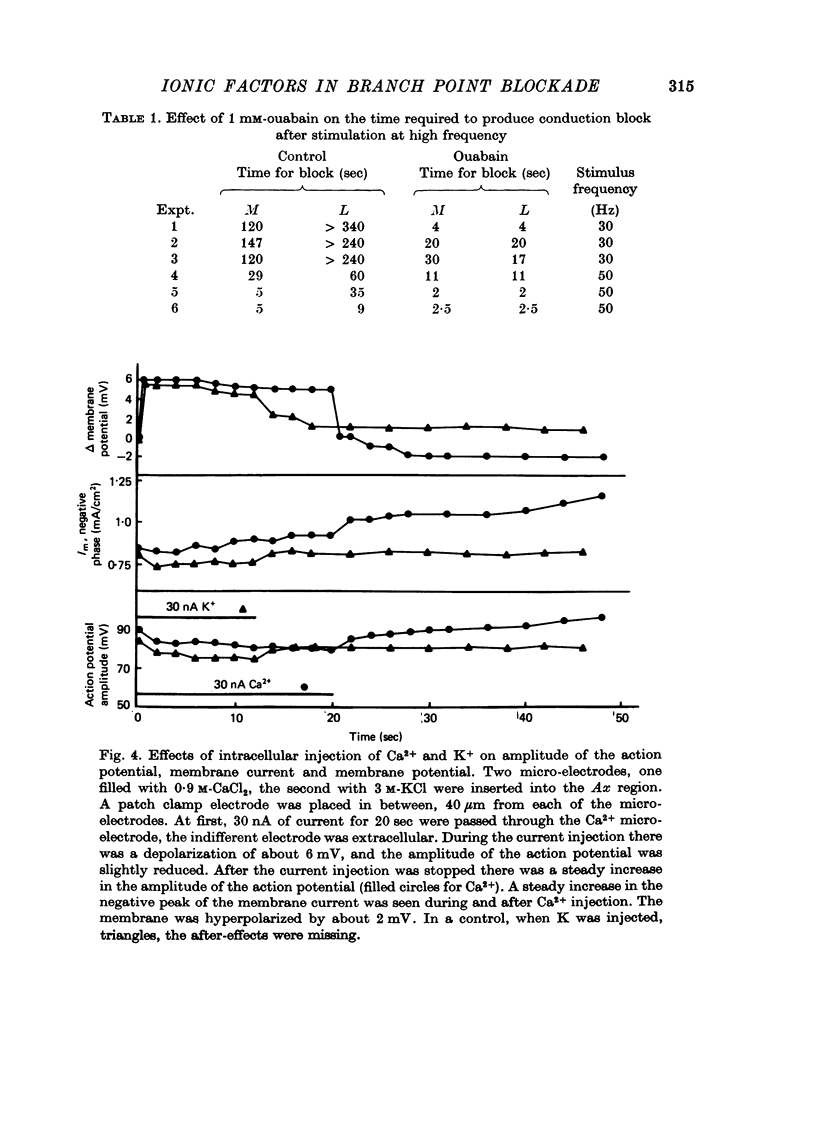
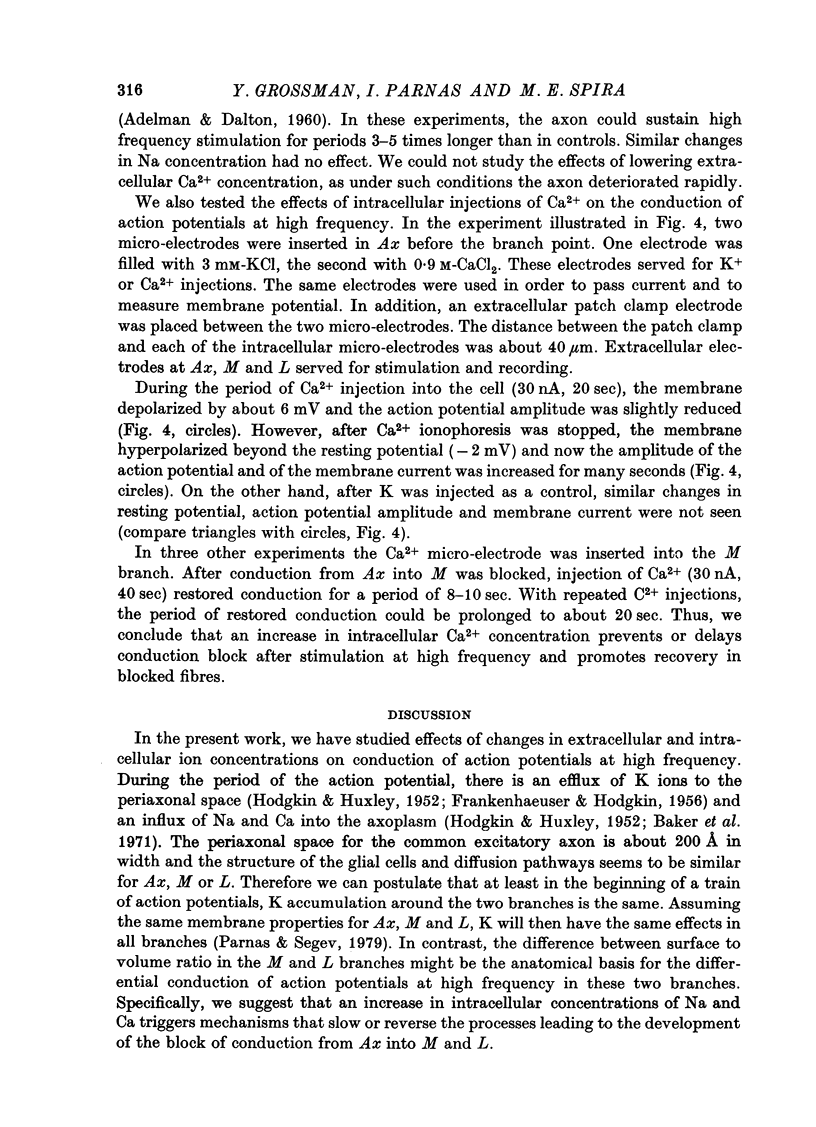
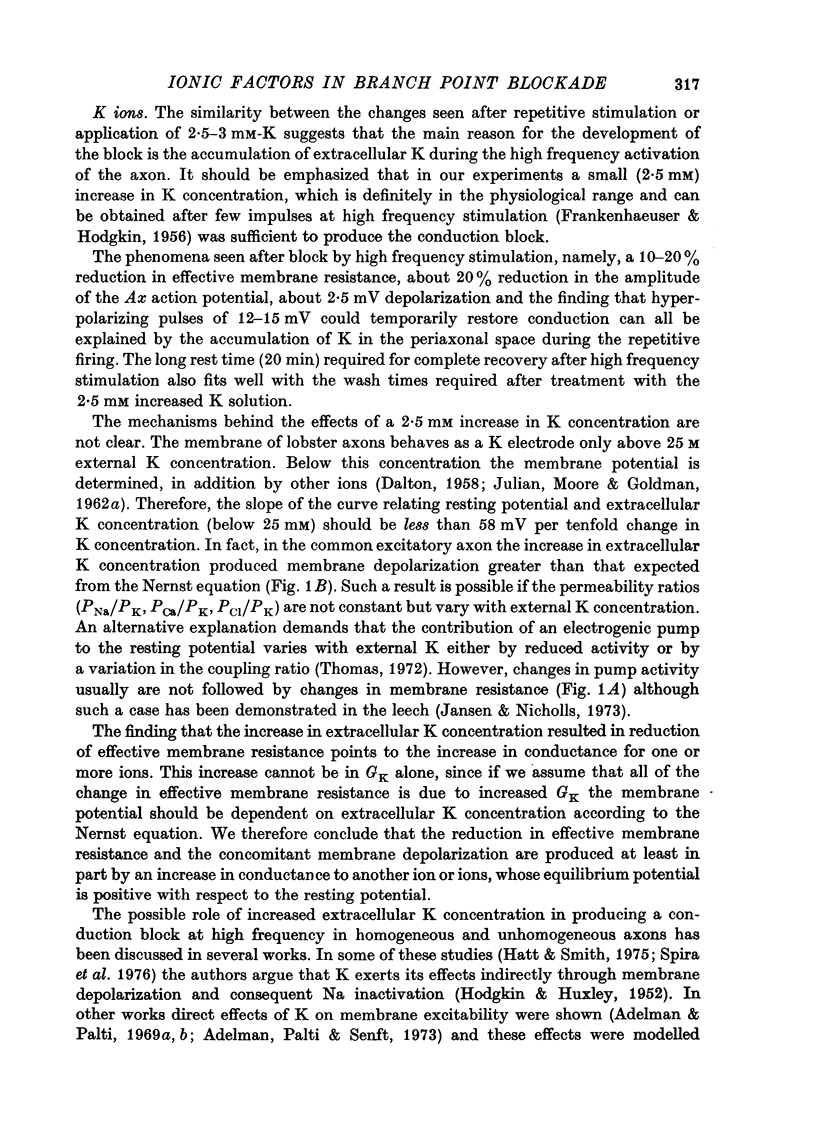
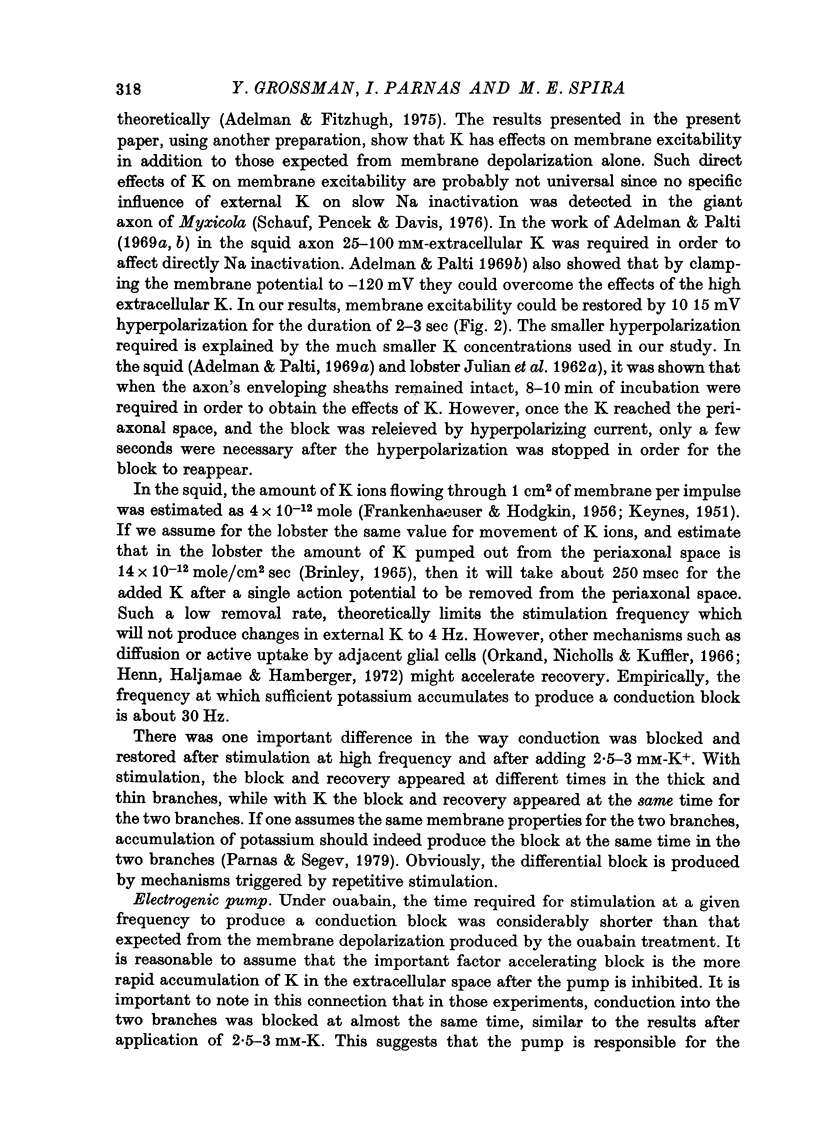
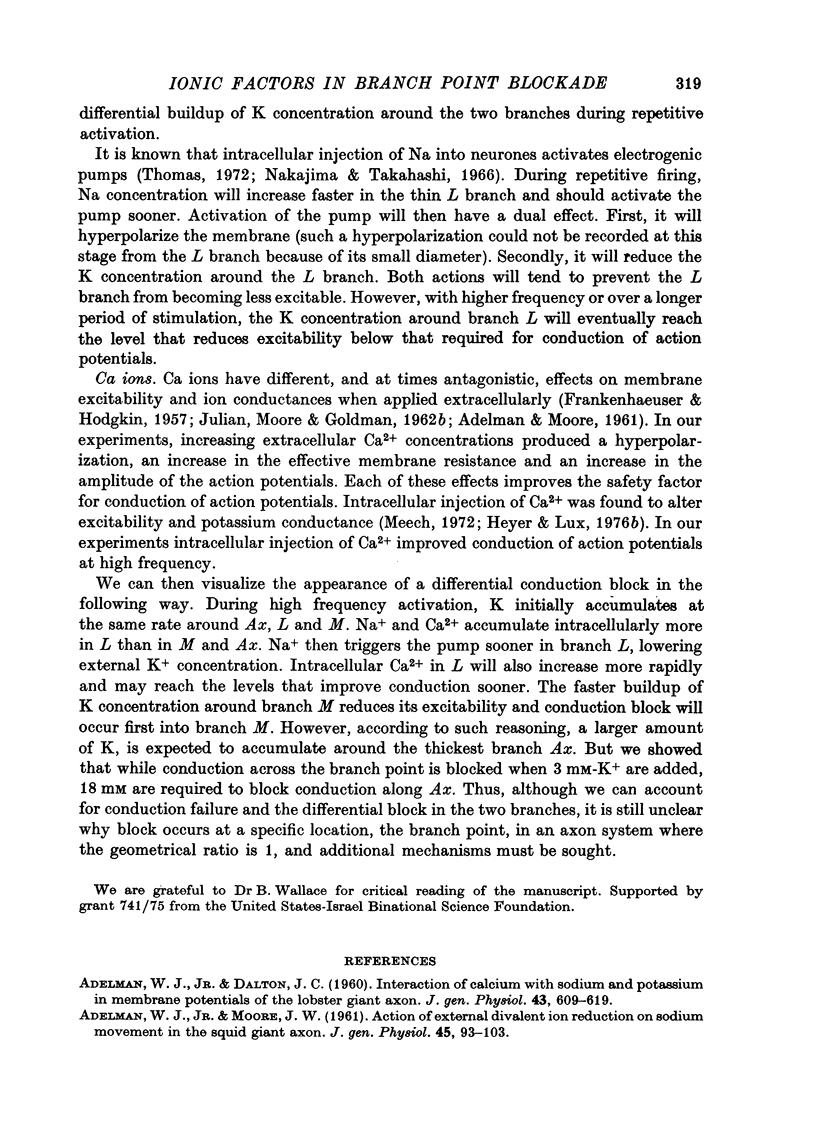
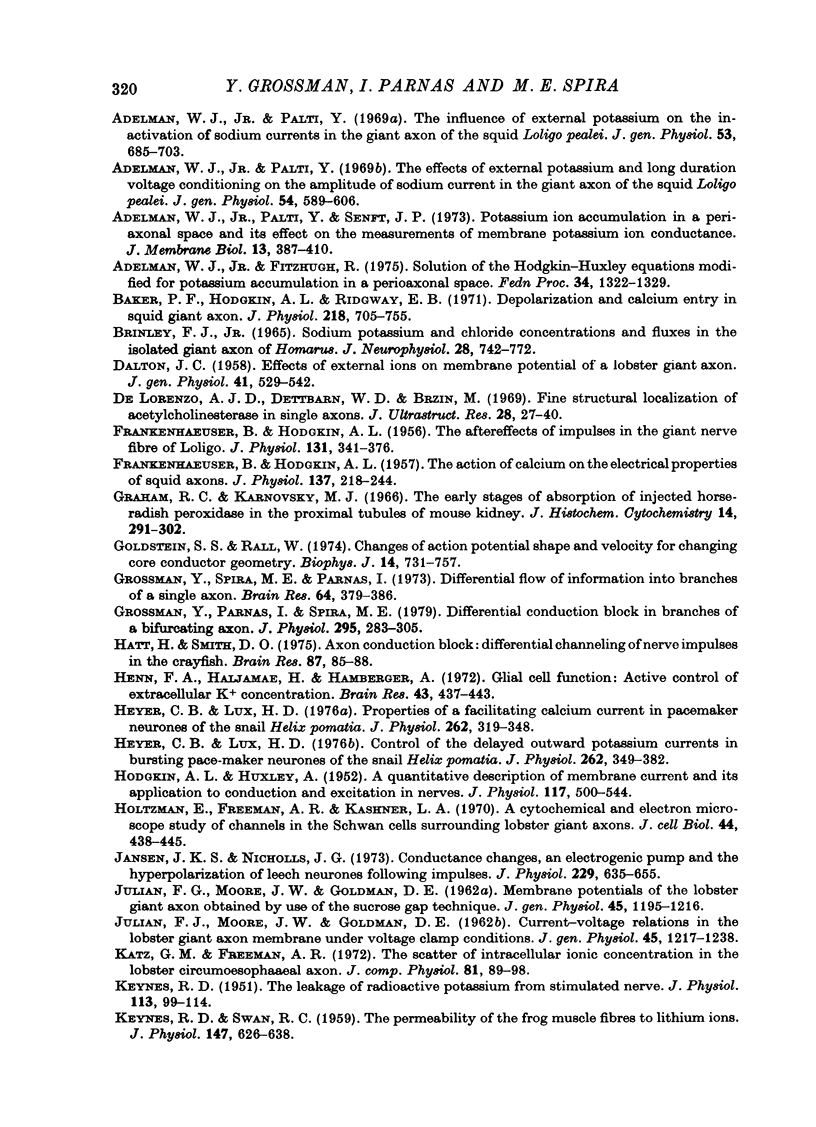
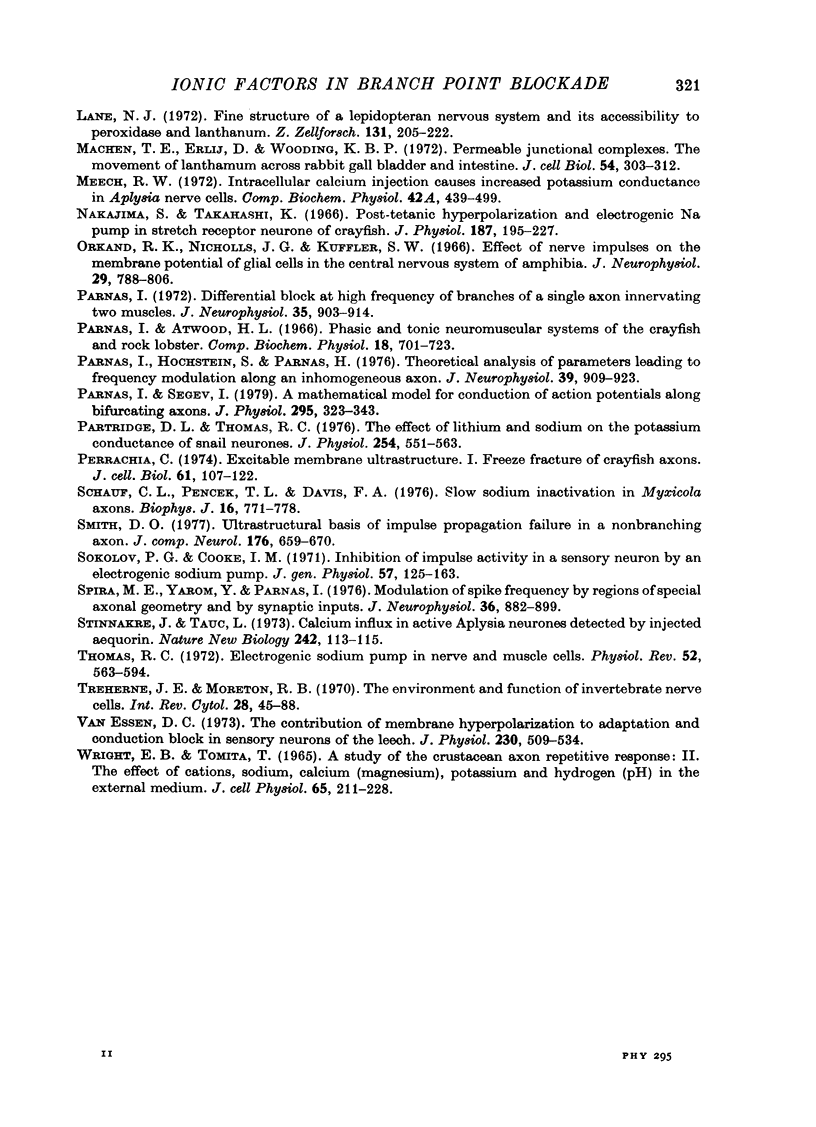
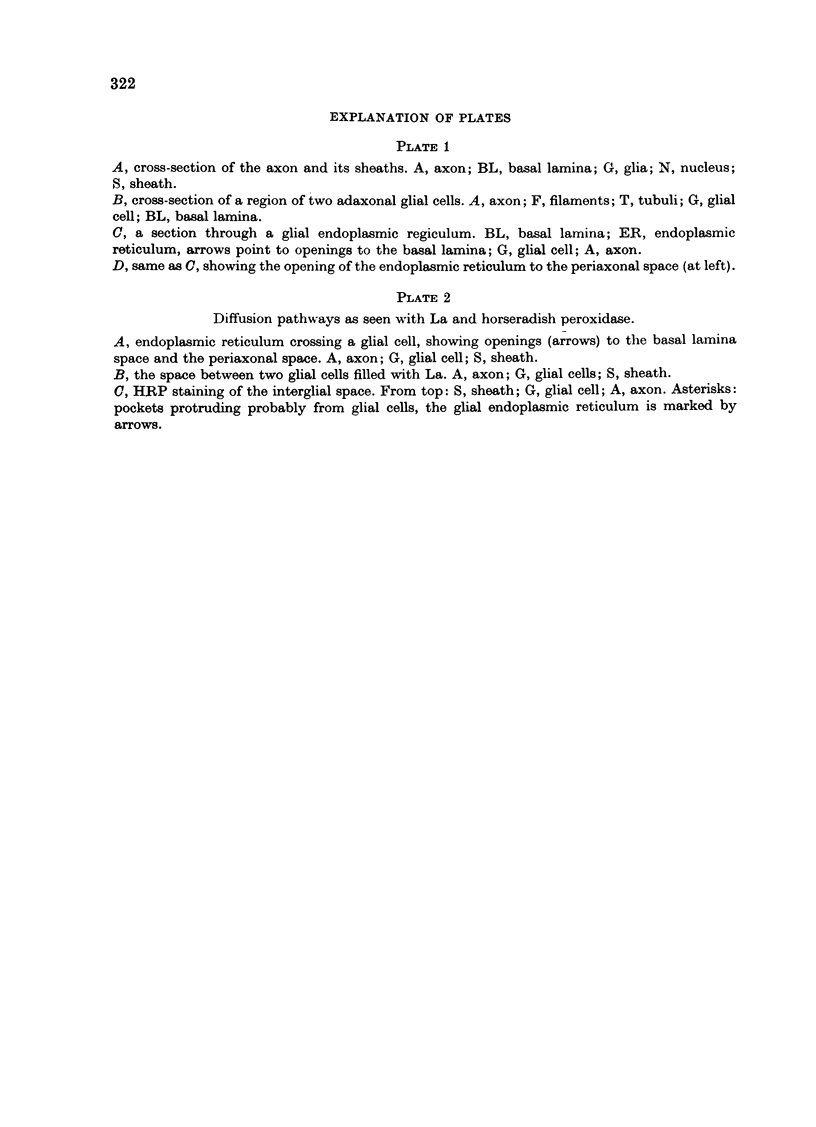
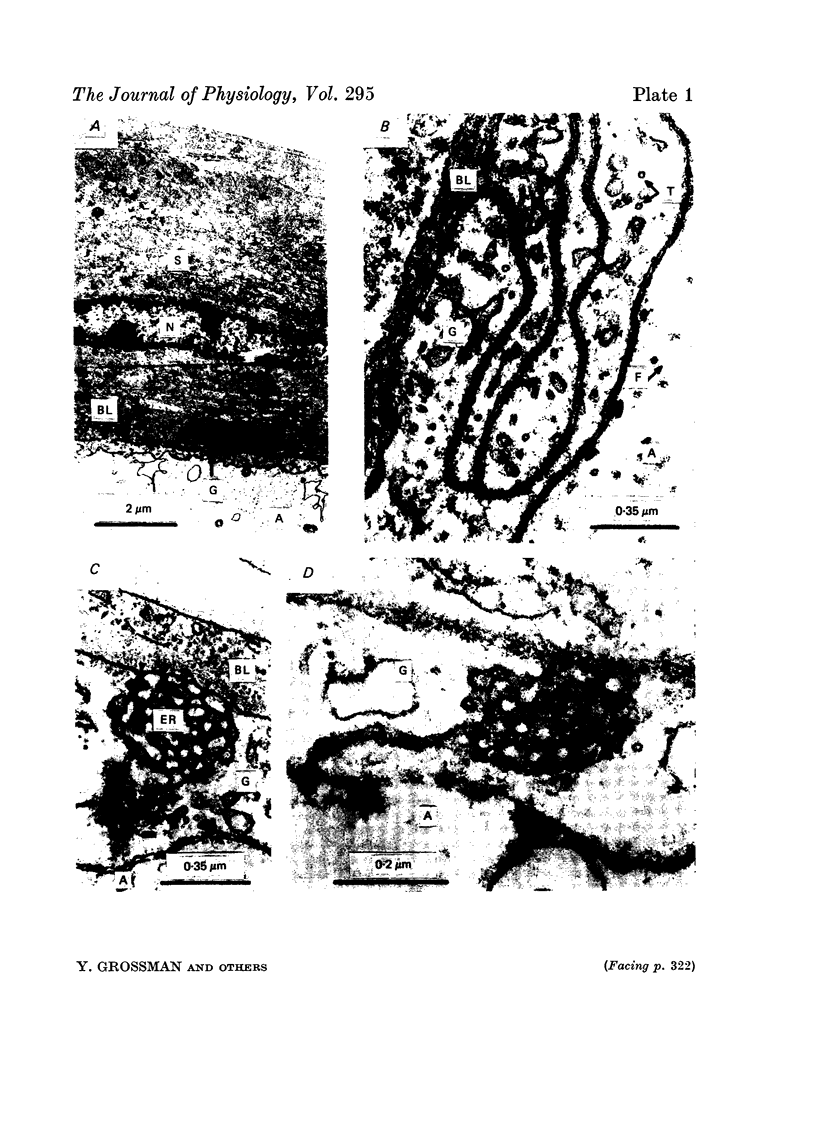
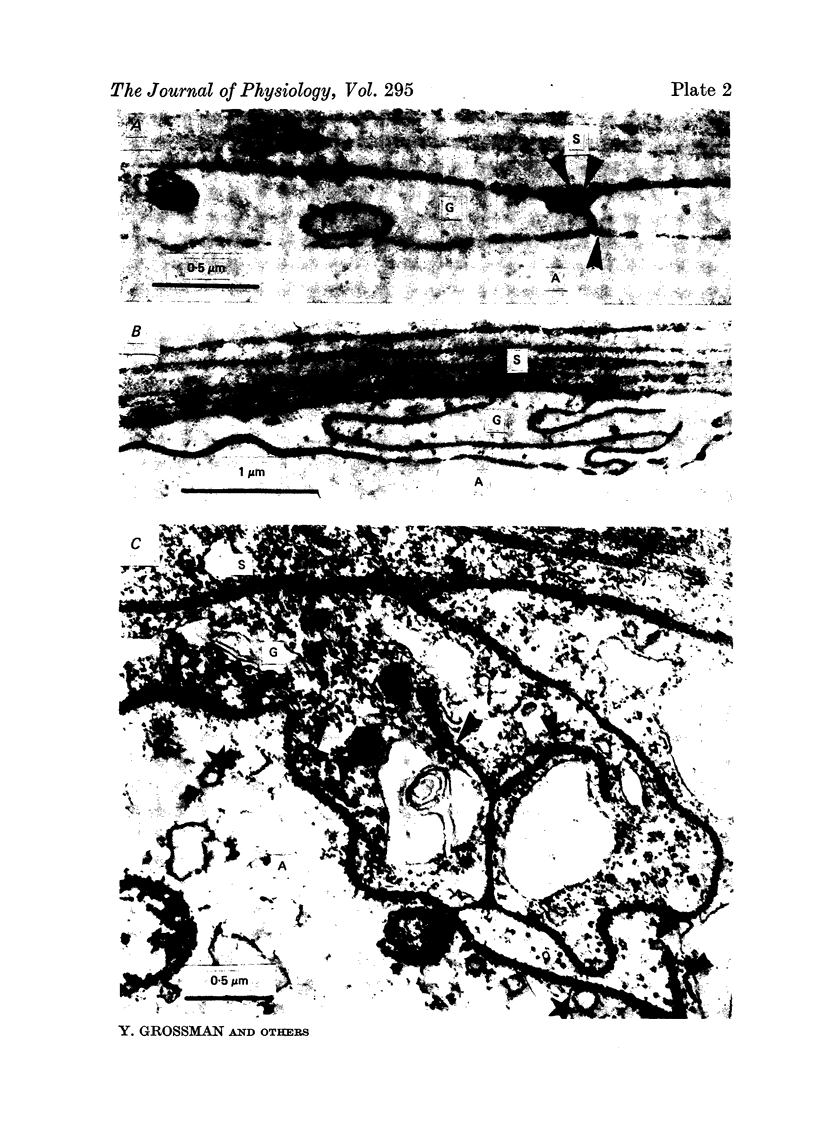
Images in this article
Selected References
These references are in PubMed. This may not be the complete list of references from this article.
- ADELMAN W. J., Jr, DALTON J. C. Interactions of calcium with sodium and potassium in membrane potentials of the lobster giant axon. J Gen Physiol. 1960 Jan;43:609–619. doi: 10.1085/jgp.43.3.609. [DOI] [PMC free article] [PubMed] [Google Scholar]
- ADELMAN W. J., Jr, MOORE J. W. Action of external divalent ion reduction on sodium movement in the squid giant axon. J Gen Physiol. 1961 Sep;45:93–103. doi: 10.1085/jgp.45.1.93. [DOI] [PMC free article] [PubMed] [Google Scholar]
- Adelman W. J., Jr, Fitzhugh R. Solutions of the Hodgkin-Huxley equations modified for potassium accumulation in a periaxonal space. Fed Proc. 1975 Apr;34(5):1322–1329. [PubMed] [Google Scholar]
- Adelman W. J., Jr, Palti Y., Senft J. P. Potassium ion accumulation in a periaxonal space and its effect on the measurement of membrane potassium ion conductance. J Membr Biol. 1973 Nov 8;13(4):387–410. doi: 10.1007/BF01868237. [DOI] [PubMed] [Google Scholar]
- Adelman W. J., Jr, Palti Y. The effects of external potassium and long duration voltage conditioning on the amplitude of sodium currents in the giant axon of the squid, Loligo pealei. J Gen Physiol. 1969 Nov;54(5):589–606. doi: 10.1085/jgp.54.5.589. [DOI] [PMC free article] [PubMed] [Google Scholar]
- Adelman W. J., Jr, Palti Y. The influence of external potassium on the inactivation of sodium currents in the giant axon of the squid, Loligo pealei. J Gen Physiol. 1969 Jun;53(6):685–703. doi: 10.1085/jgp.53.6.685. [DOI] [PMC free article] [PubMed] [Google Scholar]
- BRINLEY F. J., Jr SODIUM, POTASSIUM, AND CHLORIDE CONCENTRATIONS AND FLUXES IN THE ISOLATED GIANT AXON OF HOMARUS. J Neurophysiol. 1965 Jul;28:742–772. doi: 10.1152/jn.1965.28.4.742. [DOI] [PubMed] [Google Scholar]
- Baker P. F., Hodgkin A. L., Ridgway E. B. Depolarization and calcium entry in squid giant axons. J Physiol. 1971 Nov;218(3):709–755. doi: 10.1113/jphysiol.1971.sp009641. [DOI] [PMC free article] [PubMed] [Google Scholar]
- DALTON J. C. Effects of external ions on membrane potentials of a lobster giant axon. J Gen Physiol. 1958 Jan 20;41(3):529–542. doi: 10.1085/jgp.41.3.529. [DOI] [PMC free article] [PubMed] [Google Scholar]
- Darin de Lorenzo A. J., Dettbarn W. D., Brzin M. Fine structural localization of acetylcholinesterase in single axons. J Ultrastruct Res. 1969 Jul;28(1):27–40. doi: 10.1016/s0022-5320(69)90004-5. [DOI] [PubMed] [Google Scholar]
- FRANKENHAEUSER B., HODGKIN A. L. The action of calcium on the electrical properties of squid axons. J Physiol. 1957 Jul 11;137(2):218–244. doi: 10.1113/jphysiol.1957.sp005808. [DOI] [PMC free article] [PubMed] [Google Scholar]
- FRANKENHAEUSER B., HODGKIN A. L. The after-effects of impulses in the giant nerve fibres of Loligo. J Physiol. 1956 Feb 28;131(2):341–376. doi: 10.1113/jphysiol.1956.sp005467. [DOI] [PMC free article] [PubMed] [Google Scholar]
- Goldstein S. S., Rall W. Changes of action potential shape and velocity for changing core conductor geometry. Biophys J. 1974 Oct;14(10):731–757. doi: 10.1016/S0006-3495(74)85947-3. [DOI] [PMC free article] [PubMed] [Google Scholar]
- Graham R. C., Jr, Karnovsky M. J. The early stages of absorption of injected horseradish peroxidase in the proximal tubules of mouse kidney: ultrastructural cytochemistry by a new technique. J Histochem Cytochem. 1966 Apr;14(4):291–302. doi: 10.1177/14.4.291. [DOI] [PubMed] [Google Scholar]
- Grossman Y., Parnas I., Spira M. E. Differential conduction block in branches of a bifurcating axon. J Physiol. 1979 Oct;295:283–305. doi: 10.1113/jphysiol.1979.sp012969. [DOI] [PMC free article] [PubMed] [Google Scholar]
- Grossman Y., Spira M. E., Parnas I. Differential flow of information into branches of a single axon. Brain Res. 1973 Dec 21;64:379–386. doi: 10.1016/0006-8993(73)90191-1. [DOI] [PubMed] [Google Scholar]
- HODGKIN A. L., HUXLEY A. F. A quantitative description of membrane current and its application to conduction and excitation in nerve. J Physiol. 1952 Aug;117(4):500–544. doi: 10.1113/jphysiol.1952.sp004764. [DOI] [PMC free article] [PubMed] [Google Scholar]
- Henn F. A., Haljamäe H., Hamberger A. Glial cell function: active control of extracellular K + concentration. Brain Res. 1972 Aug 25;43(2):437–443. doi: 10.1016/0006-8993(72)90399-x. [DOI] [PubMed] [Google Scholar]
- Heyer C. B., Lux H. D. Control of the delayed outward potassium currents in bursting pace-maker neurones of the snail, Helix pomatia. J Physiol. 1976 Nov;262(2):349–382. doi: 10.1113/jphysiol.1976.sp011599. [DOI] [PMC free article] [PubMed] [Google Scholar]
- Heyer C. B., Lux H. D. Properties of a facilitating calcium current in pace-maker neurones of the snail, Helix pomatia. J Physiol. 1976 Nov;262(2):319–348. doi: 10.1113/jphysiol.1976.sp011598. [DOI] [PMC free article] [PubMed] [Google Scholar]
- Holtzman E., Freeman A. R., Kashner L. A. A cytochemical and electron microscope study of channels in the Schwann cells surrounding lobster giant axons. J Cell Biol. 1970 Feb;44(2):438–445. doi: 10.1083/jcb.44.2.438. [DOI] [PMC free article] [PubMed] [Google Scholar]
- JULIAN F. J., MOORE J. W., GOLDMAN D. E. Current-voltage relations in the lobster giant axon membrane under voltage clamp conditions. J Gen Physiol. 1962 Jul;45:1217–1238. doi: 10.1085/jgp.45.6.1217. [DOI] [PMC free article] [PubMed] [Google Scholar]
- JULIAN F. J., MOORE J. W., GOLDMAN D. E. Membrane potentials of the lobster giant axon obtained by use of the sucrose-gap technique. J Gen Physiol. 1962 Jul;45:1195–1216. doi: 10.1085/jgp.45.6.1195. [DOI] [PMC free article] [PubMed] [Google Scholar]
- Jansen J. K., Nicholls J. G. Conductance changes, an electrogenic pump and the hyperpolarization of leech neurones following impulses. J Physiol. 1973 Mar;229(3):635–655. doi: 10.1113/jphysiol.1973.sp010158. [DOI] [PMC free article] [PubMed] [Google Scholar]
- KEYNES R. D., SWAN R. C. The permeability of frog muscle fibres to lithium ions. J Physiol. 1959 Oct;147:626–638. doi: 10.1113/jphysiol.1959.sp006265. [DOI] [PMC free article] [PubMed] [Google Scholar]
- KEYNES R. D. The leakage of radioactive potassium from stimulated nerve. J Physiol. 1951 Mar;113(1):99–114. doi: 10.1113/jphysiol.1951.sp004558. [DOI] [PMC free article] [PubMed] [Google Scholar]
- Lane N. J. Fine structure of a lepidopteram nervous system and its accessibility to peroxidase and lanthanum. Z Zellforsch Mikrosk Anat. 1972;131(2):205–222. doi: 10.1007/BF00306928. [DOI] [PubMed] [Google Scholar]
- Machen T. E., Erlij D., Wooding F. B. Permeable junctional complexes. The movement of lanthanum across rabbit gallbladder and intestine. J Cell Biol. 1972 Aug;54(2):302–312. doi: 10.1083/jcb.54.2.302. [DOI] [PMC free article] [PubMed] [Google Scholar]
- Meech R. W. Intracellular calcium injection causes increased potassium conductance in Aplysia nerve cells. Comp Biochem Physiol A Comp Physiol. 1972 Jun 1;42(2):493–499. doi: 10.1016/0300-9629(72)90128-4. [DOI] [PubMed] [Google Scholar]
- Orkand R. K., Nicholls J. G., Kuffler S. W. Effect of nerve impulses on the membrane potential of glial cells in the central nervous system of amphibia. J Neurophysiol. 1966 Jul;29(4):788–806. doi: 10.1152/jn.1966.29.4.788. [DOI] [PubMed] [Google Scholar]
- Parnas I., Atwood H. L. Phasic and tonic neuromuscular systems in the abdominal extensor muscles of the crayfish and rock lobster. Comp Biochem Physiol. 1966 Aug;18(4):701–723. doi: 10.1016/0010-406x(66)90206-4. [DOI] [PubMed] [Google Scholar]
- Parnas I. Differential block at high frequency of branches of a single axon innervating two muscles. J Neurophysiol. 1972 Nov;35(6):903–914. doi: 10.1152/jn.1972.35.6.903. [DOI] [PubMed] [Google Scholar]
- Parnas I., Hochstein S., Parnas H. Theoretical analysis of parameters leading to frequency modulation along an inhomogeneous axon. J Neurophysiol. 1976 Jul;39(4):909–923. doi: 10.1152/jn.1976.39.4.909. [DOI] [PubMed] [Google Scholar]
- Parnas I., Segev I. A mathematical model for conduction of action potentials along bifurcating axons. J Physiol. 1979 Oct;295:323–343. doi: 10.1113/jphysiol.1979.sp012971. [DOI] [PMC free article] [PubMed] [Google Scholar]
- Partridge L. D., Thomas R. C. The effects of lithium and sodium on the potassium conductance of snail neurones. J Physiol. 1976 Jan;254(3):551–563. doi: 10.1113/jphysiol.1976.sp011246. [DOI] [PMC free article] [PubMed] [Google Scholar]
- Schauf C. L., Pencek T. L., Davis F. A. Slow sodium inactivation in Myxicola axons. Evidence for a second inactive state. Biophys J. 1976 Jul;16(7):771–778. doi: 10.1016/S0006-3495(76)85727-X. [DOI] [PMC free article] [PubMed] [Google Scholar]
- Smith D. O. Ultrastructural basis of impulse propagation failure in a nonbranching axon. J Comp Neurol. 1977 Dec 15;176(4):659–669. doi: 10.1002/cne.901760413. [DOI] [PubMed] [Google Scholar]
- Sokolove P. G., Cooke I. M. Inhibition of impulse activity in a sensory neuron by an electrogenic pump. J Gen Physiol. 1971 Feb;57(2):125–163. doi: 10.1085/jgp.57.2.125. [DOI] [PMC free article] [PubMed] [Google Scholar]
- Stinnakre J., Tauc L. Calcium influx in active Aplysia neurones detected by injected aequorin. Nat New Biol. 1973 Mar 28;242(117):113–115. doi: 10.1038/newbio242113b0. [DOI] [PubMed] [Google Scholar]
- Thomas R. C. Electrogenic sodium pump in nerve and muscle cells. Physiol Rev. 1972 Jul;52(3):563–594. doi: 10.1152/physrev.1972.52.3.563. [DOI] [PubMed] [Google Scholar]
- Treherne J. E., Moreton R. B. The environment and function of invertebrate nerve cells. Int Rev Cytol. 1970;28:45–88. doi: 10.1016/s0074-7696(08)62540-1. [DOI] [PubMed] [Google Scholar]
- Van Essen D. C. The contribution of membrane hyperpolarization to adaptation and conduction block in sensory neurones of the leech. J Physiol. 1973 May;230(3):509–534. doi: 10.1113/jphysiol.1973.sp010201. [DOI] [PMC free article] [PubMed] [Google Scholar]
- Wright E. B., Tomita T. A study of the crustacean axon repetitive response. II. The effect of cations, sodium, calcium (magnesium), potassium and hydrogen (pH) in the external medium. J Cell Physiol. 1965 Apr;65(2):211–228. doi: 10.1002/jcp.1030650208. [DOI] [PubMed] [Google Scholar]