Abstract
Analysis of the far-ultraviolet solution and the oriented-film circular dichroic (CD) spectra of the purple membrane (PM) has indicated that the α-helical segments of its sole protein bacteriorhodopsin (bR) can undergo a significant tilting from the normal to the membrane plane during light-dependent hydroxylamine-mediated bleaching of the bR. However, this drastic change in tertiary structure is free of any observable secondary structural changes. This phenomenon can provide an excellent means for studying the relative contributions of forces responsible for the stability of this transmembrane protein within the membrane bilayer. Perturbation of the PM by varying degrees of papain digestion (resulting in changes in the bR ranging from only an elimination of the long COOH-terminal tail to the additional eliminations of the short NH2-terminal tail and a number of linkage amino acids between the helical segments of the bR) and by chemical cross-linking with dimethyl adipimidate (resulting primarily in the formation of intramolecular cross-links) resulted in a significant increase in this bleaching-induced tilting in all cases except the one in which only the COOH-tail was eliminated. The most severe perturbation (2-wk papain digestion) increased the net tilt angle per segment from 24 to 39° with no indication of any secondary structural changes. Although these perturbations drastically reduced the structural stability of the bR to bleaching, they caused virtually no observable changes in the intramolecular structure of the bR or the supramolecular structure of the PM based on analysis of extensive absorption, linear dichroic, and CD spectra. In addition, study of the bleaching rates for the perturbed PM samples indicated that a linear correlation exists between the calculated initial bleaching rates and the net tilt angles.
Considering the forces generally assumed to account for the stability of transmembrane proteins in membranes, (a) intersegmental hydrogen bonding and electrostatic interactions, (b) electrostatic interactions between hydrophilic polypeptide segments extending outside the bilayer and the many charged lipid heads of the bilayer, and (c) hydrophobic interactions, it is clear that the results of the bleaching experiments eliminate all but perhaps the last as contributing significantly to the bR stability in the PM. Furthermore, they provide more compelling evidence than previously available that the bR is capable of undergoing relatively large retinyldiene-controlled tertiary structural changes and that the chromophoric retinal serves as the most important factor in the native bR structural stability. This dynamic view of the bR bears directly on models proposed for bR function, favoring those in which protein structural metastability, rather than rigidity, is an essential factor. The proteinquake or deformation wave model proposed by this laboratory falls into this category.
Full text
PDF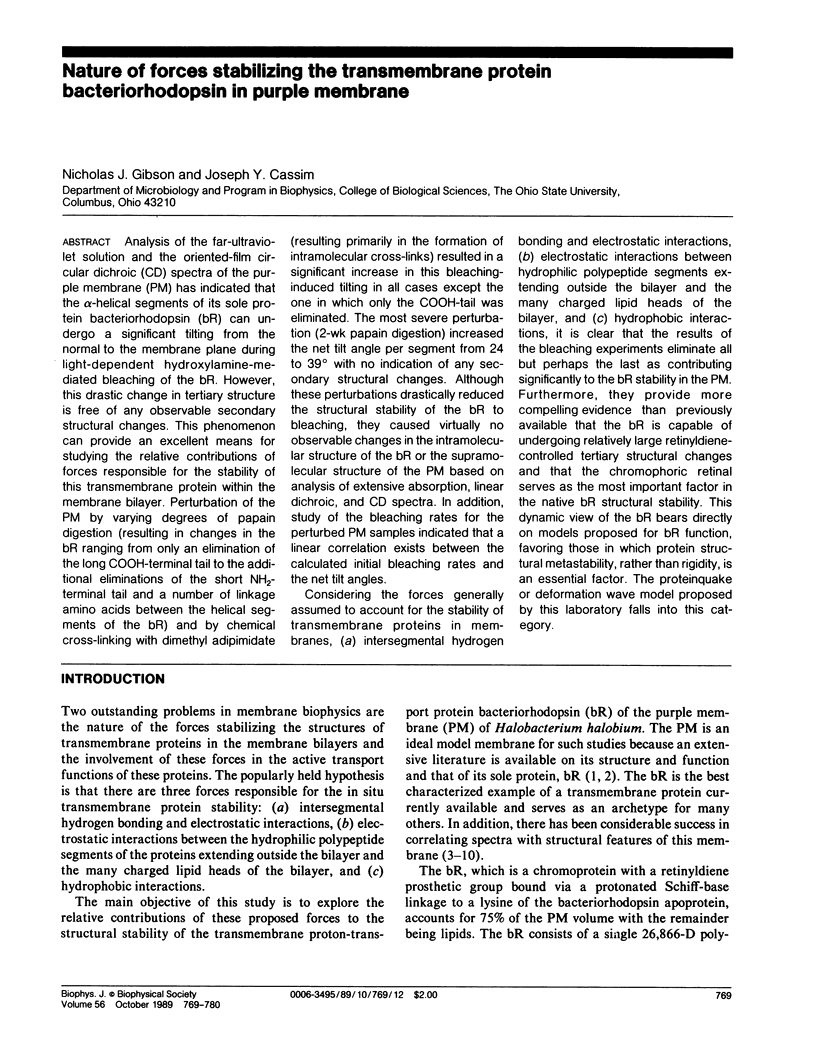
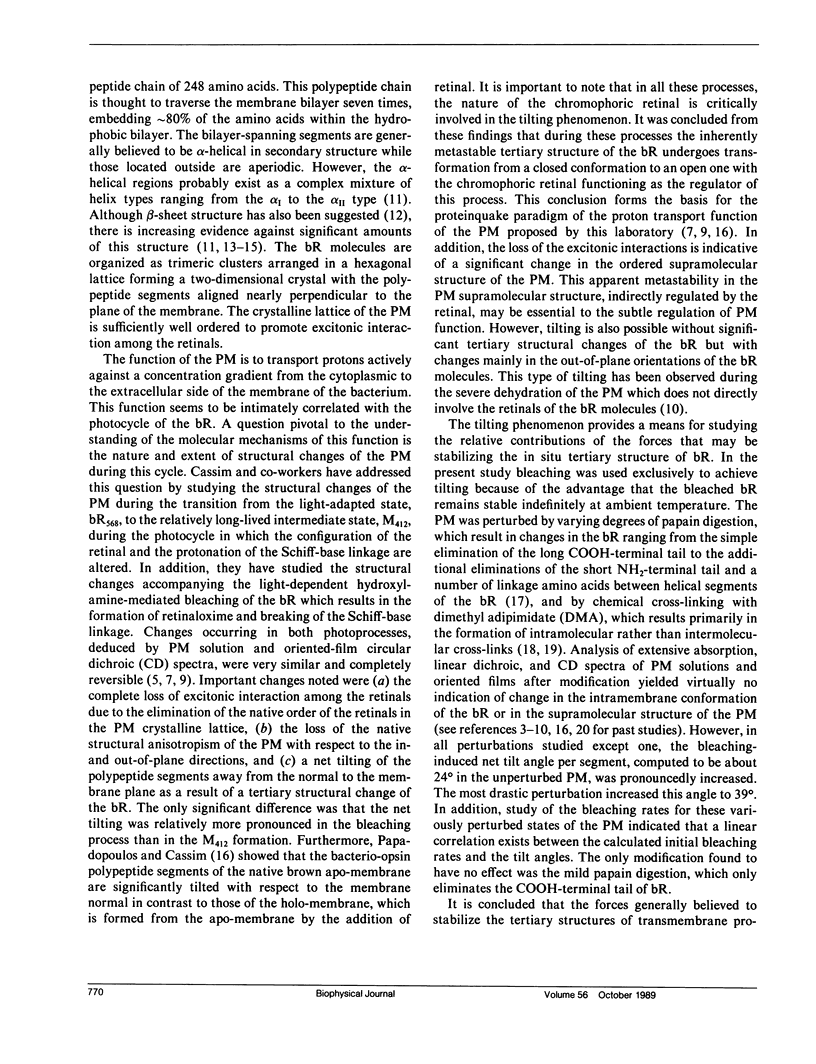
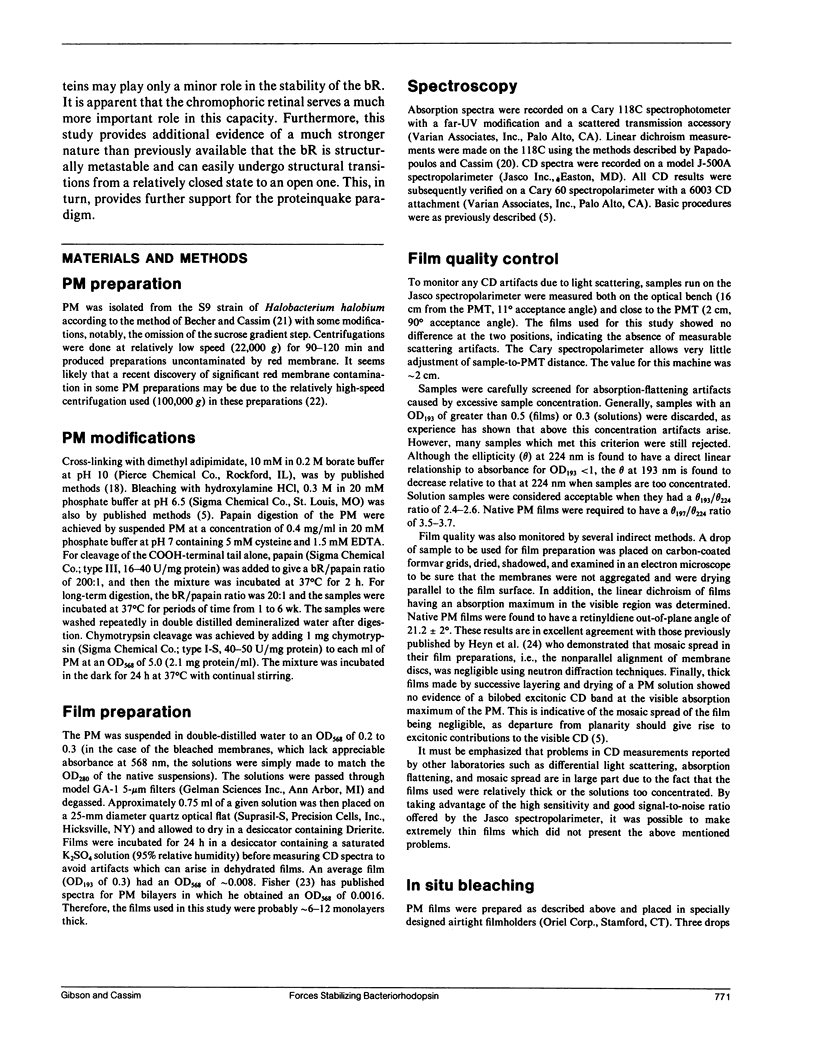
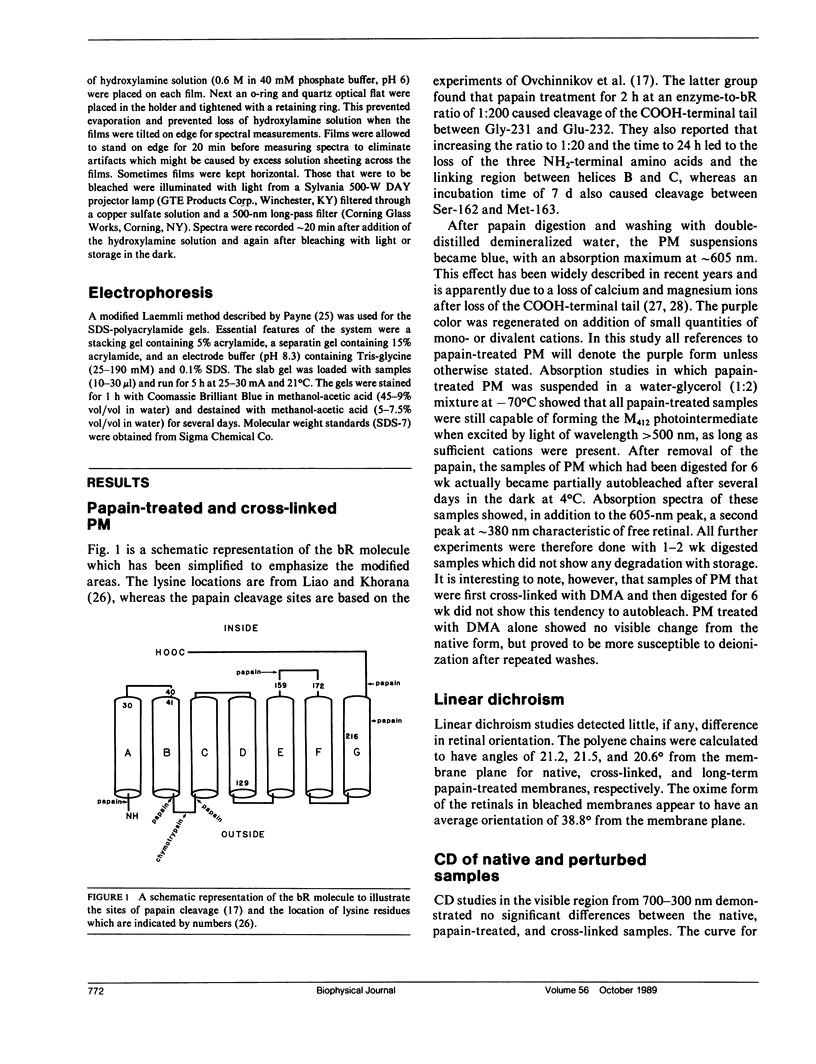
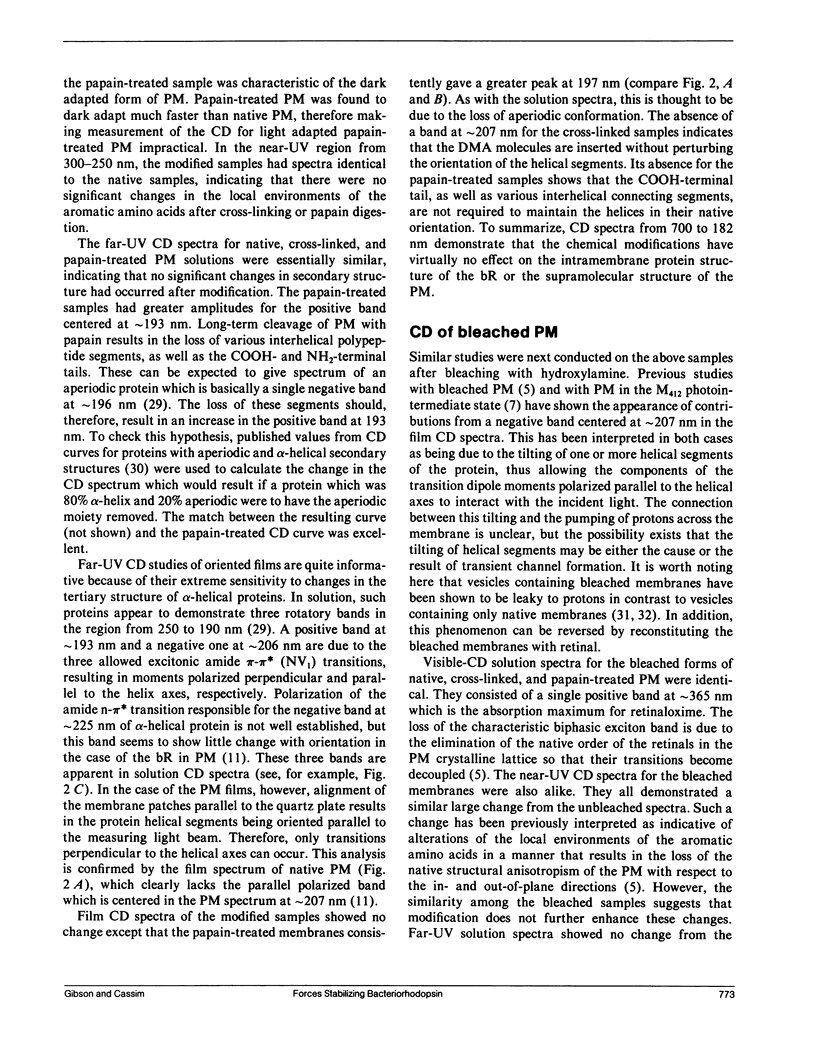
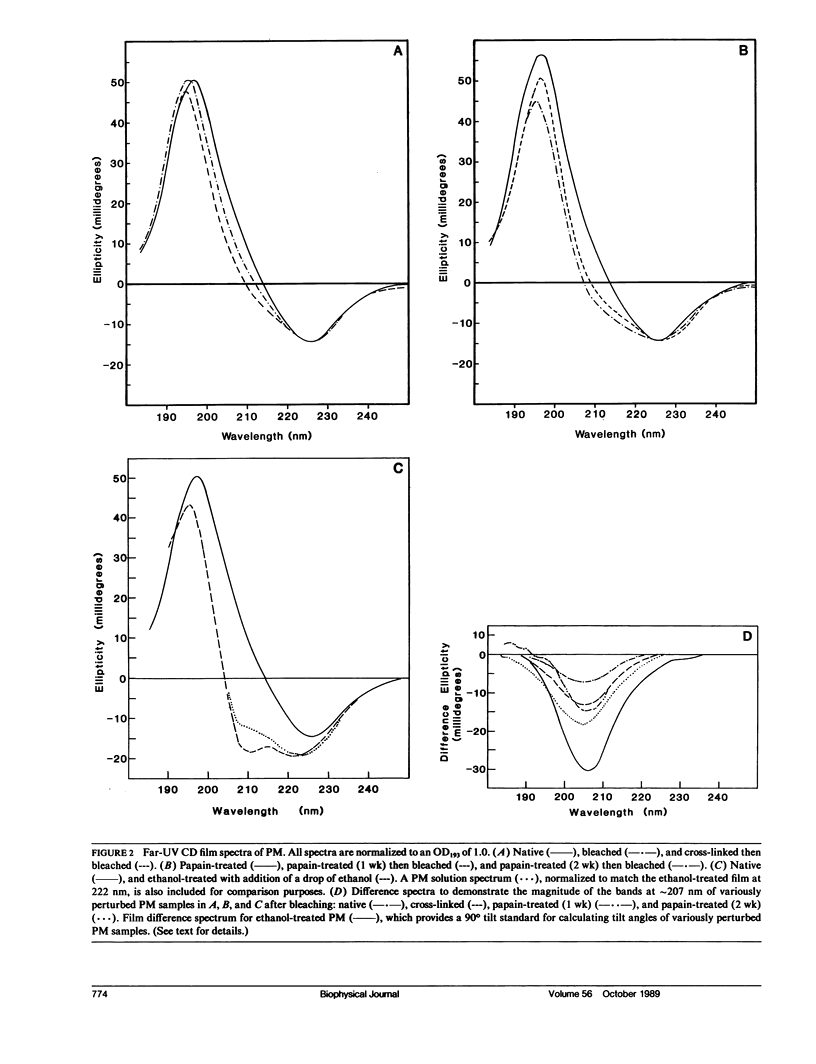
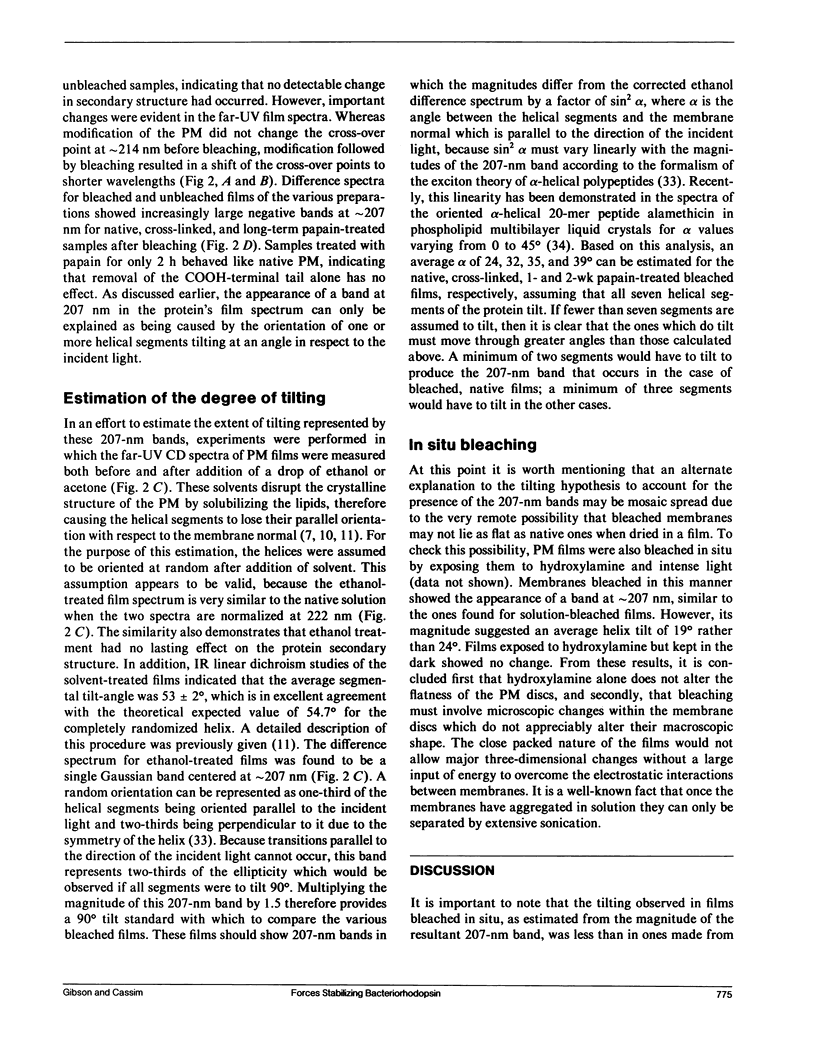
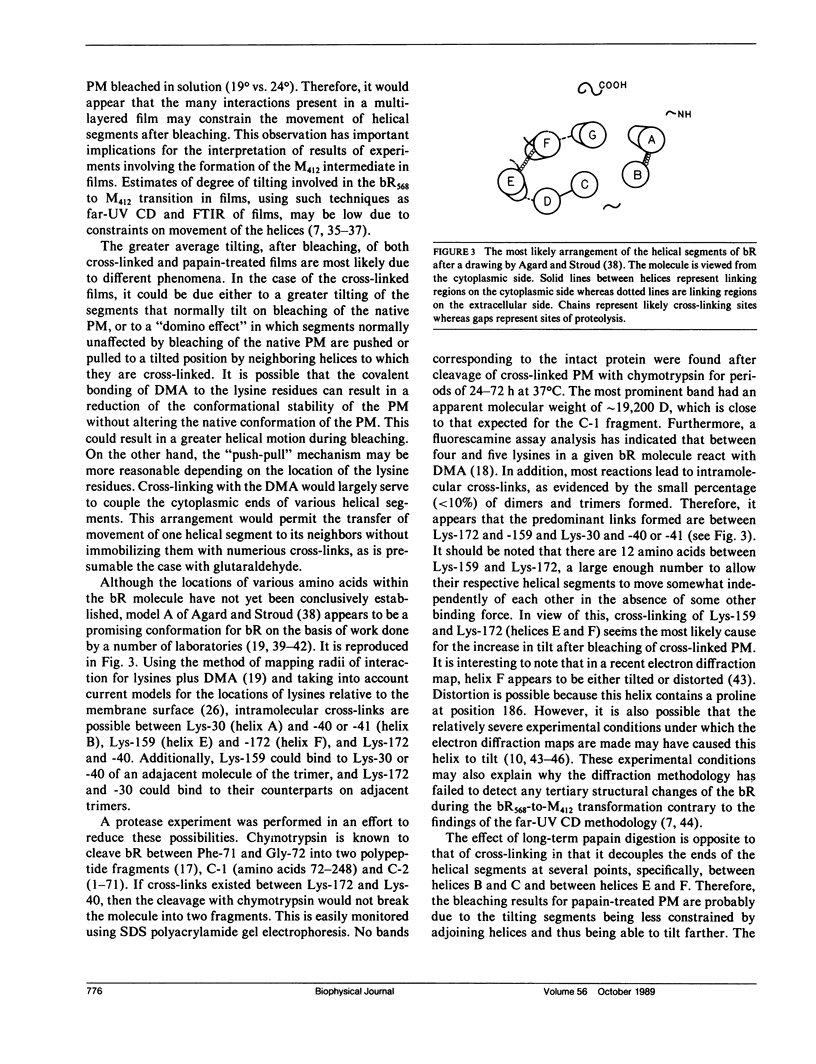
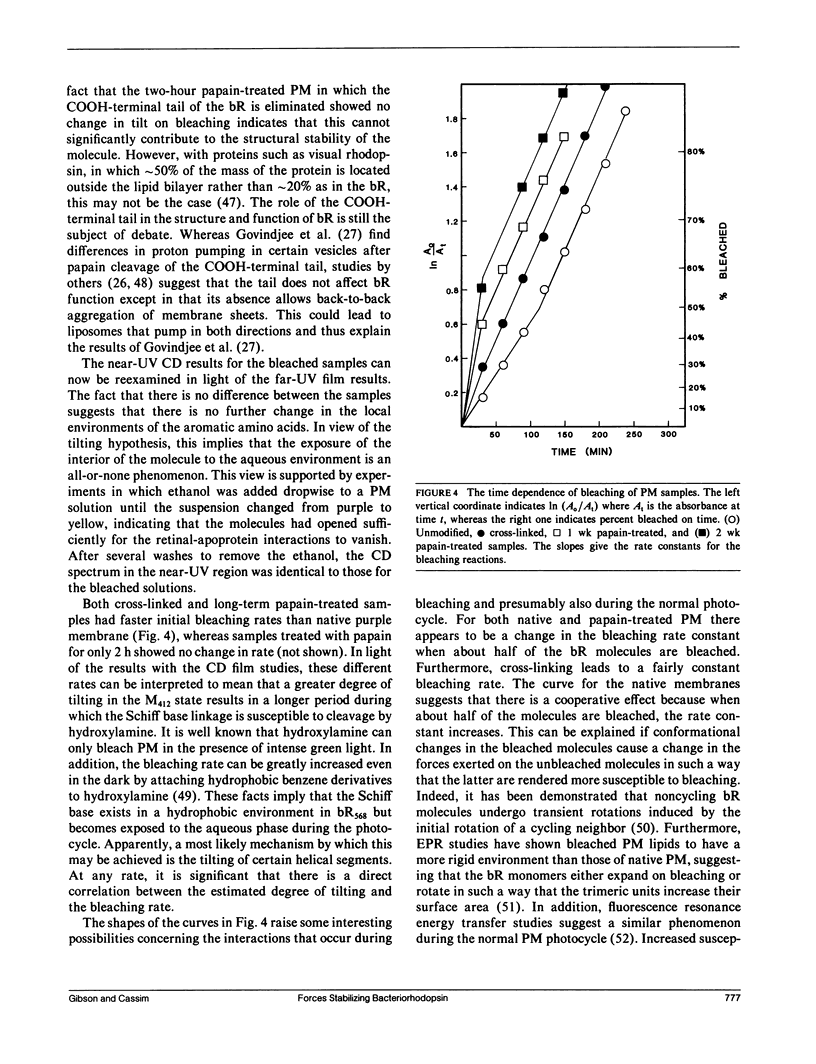
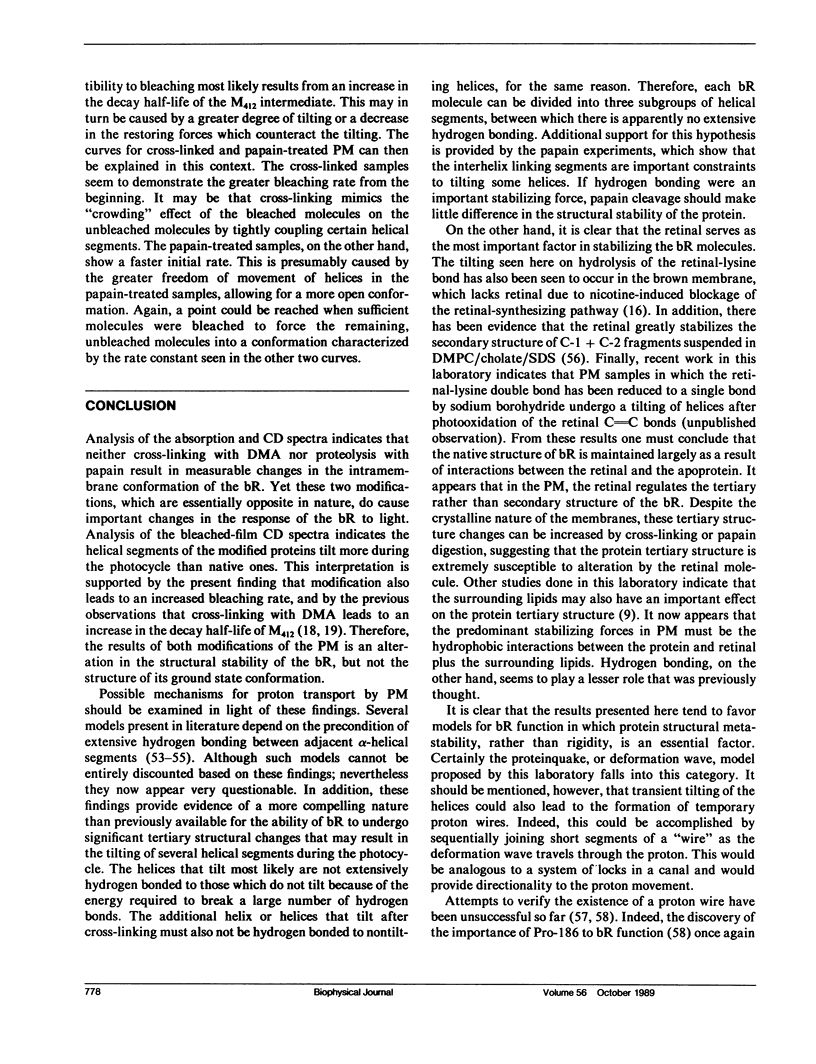
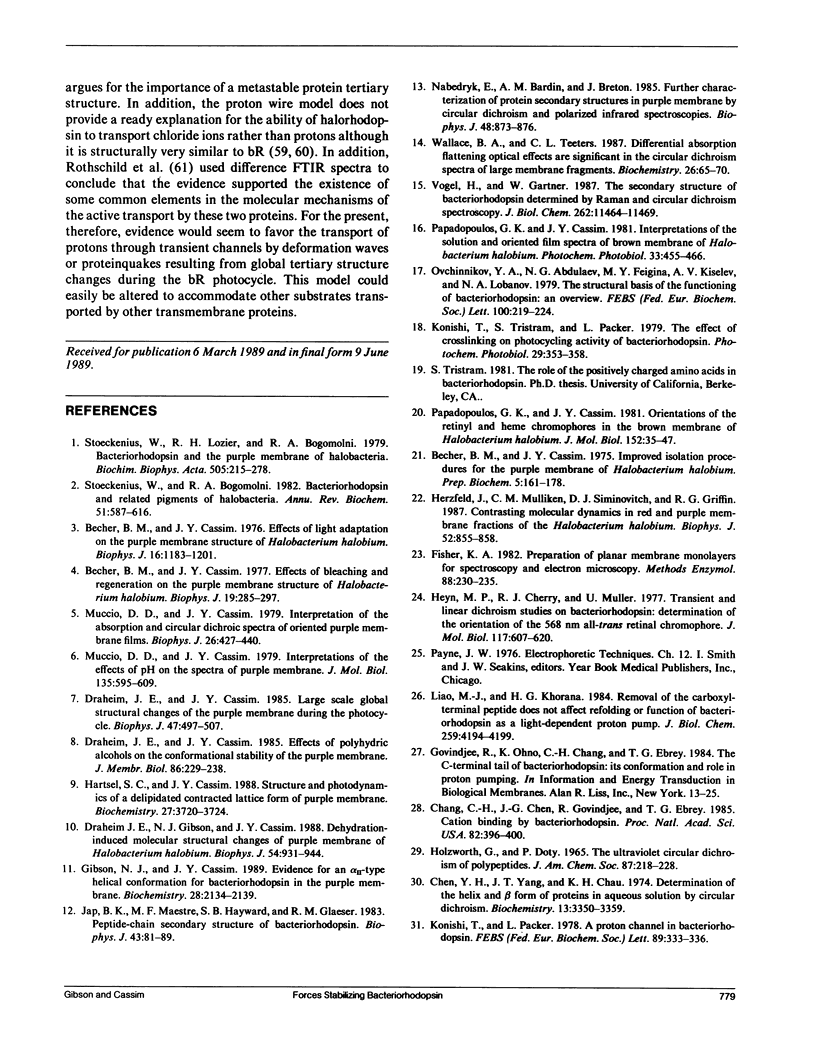
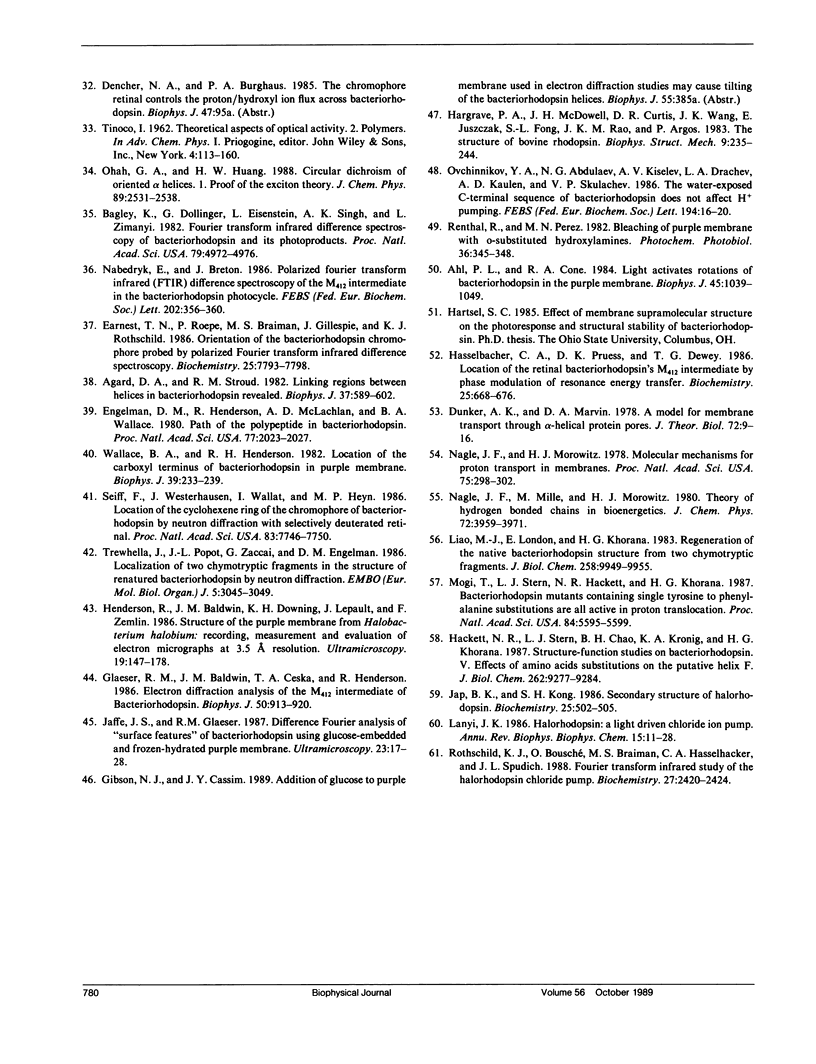
Selected References
These references are in PubMed. This may not be the complete list of references from this article.
- Agard D. A., Stroud R. M. Linking regions between helices in bacteriorhodopsin revealed. Biophys J. 1982 Mar;37(3):589–602. [PMC free article] [PubMed] [Google Scholar]
- Ahl P. L., Cone R. A. Light activates rotations of bacteriorhodopsin in the purple membrane. Biophys J. 1984 Jun;45(6):1039–1049. doi: 10.1016/S0006-3495(84)84251-4. [DOI] [PMC free article] [PubMed] [Google Scholar]
- Bagley K., Dollinger G., Eisenstein L., Singh A. K., Zimányi L. Fourier transform infrared difference spectroscopy of bacteriorhodopsin and its photoproducts. Proc Natl Acad Sci U S A. 1982 Aug;79(16):4972–4976. doi: 10.1073/pnas.79.16.4972. [DOI] [PMC free article] [PubMed] [Google Scholar]
- Becher B. M., Cassim J. Y. Improved isolation procedures for the purple membrane of Halobacterium halobium. Prep Biochem. 1975;5(2):161–178. doi: 10.1080/00327487508061568. [DOI] [PubMed] [Google Scholar]
- Becher B., Cassim J. Y. Effects of bleaching and regeneration on the purple membrane structure of Halobaterium halobium. Biophys J. 1977 Sep;19(3):285–297. doi: 10.1016/s0006-3495(77)85588-4. [DOI] [PMC free article] [PubMed] [Google Scholar]
- Becher B., Cassim J. Y. Effects of light adaptation on the purple membrane structure of Halobacterium halobium. Biophys J. 1976 Oct;16(10):1183–1200. doi: 10.1016/S0006-3495(76)85767-0. [DOI] [PMC free article] [PubMed] [Google Scholar]
- Chang C. H., Chen J. G., Govindjee R., Ebrey T. Cation binding by bacteriorhodopsin. Proc Natl Acad Sci U S A. 1985 Jan;82(2):396–400. doi: 10.1073/pnas.82.2.396. [DOI] [PMC free article] [PubMed] [Google Scholar]
- Chen Y. H., Yang J. T., Chau K. H. Determination of the helix and beta form of proteins in aqueous solution by circular dichroism. Biochemistry. 1974 Jul 30;13(16):3350–3359. doi: 10.1021/bi00713a027. [DOI] [PubMed] [Google Scholar]
- Draheim J. E., Cassim J. Y. Large Scale Global Structural Changes of the Purple Membrane during the Photocycle. Biophys J. 1985 Apr;47(4):497–507. doi: 10.1016/S0006-3495(85)83943-6. [DOI] [PMC free article] [PubMed] [Google Scholar]
- Draheim J. E., Gibson N. J., Cassim J. Y. Dehydration-induced molecular structural changes of purple membrane of halobacterium halobium. Biophys J. 1988 Nov;54(5):931–944. doi: 10.1016/S0006-3495(88)83029-7. [DOI] [PMC free article] [PubMed] [Google Scholar]
- Dunker A. K., Marvin D. A. A model for membrane transport through alpha-helical protein pores. J Theor Biol. 1978 May 8;72(1):9–16. doi: 10.1016/0022-5193(78)90015-2. [DOI] [PubMed] [Google Scholar]
- Earnest T. N., Roepe P., Braiman M. S., Gillespie J., Rothschild K. J. Orientation of the bacteriorhodopsin chromophore probed by polarized Fourier transform infrared difference spectroscopy. Biochemistry. 1986 Dec 2;25(24):7793–7798. doi: 10.1021/bi00372a002. [DOI] [PubMed] [Google Scholar]
- Engelman D. M., Henderson R., McLachlan A. D., Wallace B. A. Path of the polypeptide in bacteriorhodopsin. Proc Natl Acad Sci U S A. 1980 Apr;77(4):2023–2027. doi: 10.1073/pnas.77.4.2023. [DOI] [PMC free article] [PubMed] [Google Scholar]
- Glaeser R. M., Baldwin J., Ceska T. A., Henderson R. Electron diffraction analysis of the M412 intermediate of bacteriorhodopsin. Biophys J. 1986 Nov;50(5):913–920. doi: 10.1016/S0006-3495(86)83532-9. [DOI] [PMC free article] [PubMed] [Google Scholar]
- Govindjee R., Ohno K., Chang C. H., Ebrey T. G. The C-terminal tail of bacteriorhodopsin--its conformation and role in proton pumping. Prog Clin Biol Res. 1984;164:13–25. [PubMed] [Google Scholar]
- HOLZWARTH G., DOTY P. THE ULTRAVIOLET CIRCULAR DICHROISM OF POLYPEPTIDES. J Am Chem Soc. 1965 Jan 20;87:218–228. doi: 10.1021/ja01080a015. [DOI] [PubMed] [Google Scholar]
- Hackett N. R., Stern L. J., Chao B. H., Kronis K. A., Khorana H. G. Structure-function studies on bacteriorhodopsin. V. Effects of amino acid substitutions in the putative helix F. J Biol Chem. 1987 Jul 5;262(19):9277–9284. [PubMed] [Google Scholar]
- Hargrave P. A., McDowell J. H., Curtis D. R., Wang J. K., Juszczak E., Fong S. L., Rao J. K., Argos P. The structure of bovine rhodopsin. Biophys Struct Mech. 1983;9(4):235–244. doi: 10.1007/BF00535659. [DOI] [PubMed] [Google Scholar]
- Herzfeld J., Mulliken C. M., Siminovitch D. J., Griffin R. G. Contrasting molecular dynamics in red and purple membrane fractions of the Halobacterium halobium. Biophys J. 1987 Nov;52(5):855–858. doi: 10.1016/S0006-3495(87)83278-2. [DOI] [PMC free article] [PubMed] [Google Scholar]
- Heyn M. P., Cherry R. J., Müller U. Transient and linear dichroism studies on bacteriorhodopsin: determination of the orientation of the 568 nm all-trans retinal chromophore. J Mol Biol. 1977 Dec 15;117(3):607–620. doi: 10.1016/0022-2836(77)90060-2. [DOI] [PubMed] [Google Scholar]
- Jaffe J. S., Glaeser R. M. Difference Fourier analysis of "surface features" of bacteriorhodopsin using glucose-embedded and frozen-hydrated purple membrane. Ultramicroscopy. 1987;23(1):17–28. doi: 10.1016/0304-3991(87)90223-3. [DOI] [PubMed] [Google Scholar]
- Jap B. K., Kong S. H. Secondary structure of halorhodopsin. Biochemistry. 1986 Jan 28;25(2):502–505. doi: 10.1021/bi00350a034. [DOI] [PubMed] [Google Scholar]
- Jap B. K., Maestre M. F., Hayward S. B., Glaeser R. M. Peptide-chain secondary structure of bacteriorhodopsin. Biophys J. 1983 Jul;43(1):81–89. doi: 10.1016/S0006-3495(83)84326-4. [DOI] [PMC free article] [PubMed] [Google Scholar]
- Konishi T., Packer L. A proton channel in bacteriorhodopsin. FEBS Lett. 1978 May 15;89(2):333–336. doi: 10.1016/0014-5793(78)80249-x. [DOI] [PubMed] [Google Scholar]
- Lanyi J. K. Halorhodopsin: a light-driven chloride ion pump. Annu Rev Biophys Biophys Chem. 1986;15:11–28. doi: 10.1146/annurev.bb.15.060186.000303. [DOI] [PubMed] [Google Scholar]
- Liao M. J., Khorana H. G. Removal of the carboxyl-terminal peptide does not affect refolding or function of bacteriorhodopsin as a light-dependent proton pump. J Biol Chem. 1984 Apr 10;259(7):4194–4199. [PubMed] [Google Scholar]
- Liao M. J., London E., Khorana H. G. Regeneration of the native bacteriorhodopsin structure from two chymotryptic fragments. J Biol Chem. 1983 Aug 25;258(16):9949–9955. [PubMed] [Google Scholar]
- Mogi T., Stern L. J., Hackett N. R., Khorana H. G. Bacteriorhodopsin mutants containing single tyrosine to phenylalanine substitutions are all active in proton translocation. Proc Natl Acad Sci U S A. 1987 Aug;84(16):5595–5599. doi: 10.1073/pnas.84.16.5595. [DOI] [PMC free article] [PubMed] [Google Scholar]
- Muccio D. D., Cassim J. Y. Interpretation of the absorption and circular dichroic spectra of oriented purple membrane films. Biophys J. 1979 Jun;26(3):427–440. doi: 10.1016/S0006-3495(79)85263-7. [DOI] [PMC free article] [PubMed] [Google Scholar]
- Muccio D. D., Cassim J. Y. Interpretations of the effects of pH on the spectra of purple membrane. J Mol Biol. 1979 Dec 15;135(3):595–609. doi: 10.1016/0022-2836(79)90166-9. [DOI] [PubMed] [Google Scholar]
- Nabedryk E., Bardin A. M., Breton J. Further characterization of protein secondary structures in purple membrane by circular dichroism and polarized infrared spectroscopies. Biophys J. 1985 Dec;48(6):873–876. doi: 10.1016/S0006-3495(85)83848-0. [DOI] [PMC free article] [PubMed] [Google Scholar]
- Nagle J. F., Morowitz H. J. Molecular mechanisms for proton transport in membranes. Proc Natl Acad Sci U S A. 1978 Jan;75(1):298–302. doi: 10.1073/pnas.75.1.298. [DOI] [PMC free article] [PubMed] [Google Scholar]
- Ovchinnikov Y. A., Abdulaev N. G., Feigina M. Y., Kiselev A. V., Lobanov N. A. The structural basis of the functioning of bacteriorhodopsin: an overview. FEBS Lett. 1979 Apr 15;100(2):219–224. doi: 10.1016/0014-5793(79)80338-5. [DOI] [PubMed] [Google Scholar]
- Papadopoulos G. K., Cassim J. Y. Orientations of the retinyl and the heme chromophores in the brown membrane of Halobacterium halobium. J Mol Biol. 1981 Oct 15;152(1):35–47. doi: 10.1016/0022-2836(81)90094-2. [DOI] [PubMed] [Google Scholar]
- Rothschild K. J., Bousché O., Braiman M. S., Hasselbacher C. A., Spudich J. L. Fourier transform infrared study of the halorhodopsin chloride pump. Biochemistry. 1988 Apr 5;27(7):2420–2424. doi: 10.1021/bi00407a026. [DOI] [PubMed] [Google Scholar]
- Seiff F., Westerhausen J., Wallat I., Heyn M. P. Location of the cyclohexene ring of the chromophore of bacteriorhodopsin by neutron diffraction with selectively deuterated retinal. Proc Natl Acad Sci U S A. 1986 Oct;83(20):7746–7750. doi: 10.1073/pnas.83.20.7746. [DOI] [PMC free article] [PubMed] [Google Scholar]
- Stoeckenius W., Bogomolni R. A. Bacteriorhodopsin and related pigments of halobacteria. Annu Rev Biochem. 1982;51:587–616. doi: 10.1146/annurev.bi.51.070182.003103. [DOI] [PubMed] [Google Scholar]
- Stoeckenius W., Lozier R. H., Bogomolni R. A. Bacteriorhodopsin and the purple membrane of halobacteria. Biochim Biophys Acta. 1979 Mar 14;505(3-4):215–278. doi: 10.1016/0304-4173(79)90006-5. [DOI] [PubMed] [Google Scholar]
- Trewhella J., Popot J. L., Zaccaï G., Engelman D. M. Localization of two chymotryptic fragments in the structure of renatured bacteriorhodopsin by neutron diffraction. EMBO J. 1986 Nov;5(11):3045–3049. doi: 10.1002/j.1460-2075.1986.tb04604.x. [DOI] [PMC free article] [PubMed] [Google Scholar]
- Vogel H., Gärtner W. The secondary structure of bacteriorhodopsin determined by Raman and circular dichroism spectroscopy. J Biol Chem. 1987 Aug 25;262(24):11464–11469. [PubMed] [Google Scholar]
- Wallace B. A., Henderson R. Location of the carboxyl terminus of bacteriorhodopsin in purple membrane. Biophys J. 1982 Sep;39(3):233–239. doi: 10.1016/S0006-3495(82)84513-X. [DOI] [PMC free article] [PubMed] [Google Scholar]
- Wallace B. A., Teeters C. L. Differential absorption flattening optical effects are significant in the circular dichroism spectra of large membrane fragments. Biochemistry. 1987 Jan 13;26(1):65–70. doi: 10.1021/bi00375a010. [DOI] [PubMed] [Google Scholar]