Abstract
1. The influx of [3H]gamma-aminobutyric acid ([3H]GABA) into isolated rat superior cervical ganglia has been measured by radioassay, supplemented by autoradiography. Ganglia were incubated in oxygenated Krebs solution at 25 degrees C, containing 10 microM-amino-oxyacetic acid. Under these conditions more than 95% of accumulated tritium was unmetabolized [3H]GABA. 2. Ganglionic radioactivity increased linearly with incubation time, to yield an intracellular fluid/extracellular fluid concentration ratio (Ci/Co) of about 200 after 6 hr in 0.5 microM-external [3H]GABA. 3. Uptake showed saturation with an apparent transport constant (KT) of 6.8 microM and maximum influx velocity (Jmaxi) of 7 mumole 1. cell fluid-1- min-1. 4. The influx rate at Co = 0.5 microM was unaltered by raising intracellular GABA from 0.2 to 1 mM. 5. Influx velocity increased with temperature (5--35 degrees C) in a monotonic manner with an apparent activation energy of 14 kcal mole-1. 6. Concentrative uptake was depressed by reducing external [Na+] with ouabain, by raising [K+]o above 20 mM, or by removing external Cl-. Uptake was not particularly sensitive to Ca2+ or Mg2+ ions. 7. Utake of [3H]GABA (0.5 microM) was inhibited by beta-guanidinopropionic acid (apparent KI, 28 microM), beta-alanine (KI, 55 microM), gamma-amino-beta-hydroxybutyric acid (KI, 220 microM), beta-amino-n-butyric acid (KI, 708 microM), 3-aminopropanesulphonic acid (KI, 832 microM) and taurine (KI greater than 1 mM). Uptake was not depressed by 1 mM-glycine, alpha-alanine, leucine, serine, methionine or alpha-amino-iso-butyric acid. 8. Radioactively labelled methionine, leucine, glycine, serine, beta-alanine and taurine (concentrations less than or equal to 5 microM) were also taken up by ganglia. Of these, only uptake of beta-alanine and taurine were significantly depressed by 1 mM-GABA. 9. Autoradiographs confirmed that [3H]GABA and [3H] beta-alanine were taken up predominantly into extraneuronal sites (presumed to be neuroglial cells). Methionine, leucine, glycine and serine showed preferential accumulation in neurones. Neuronal uptake of leucine was not prevented by inhibiting protein synthesis. 10. Calculations of net fluxes from unidirectional tracer fluxes suggest that the sympathetic glial cells are capable of promoting net uptake of GABA at external concentrations above 1 microM.
Full text
PDF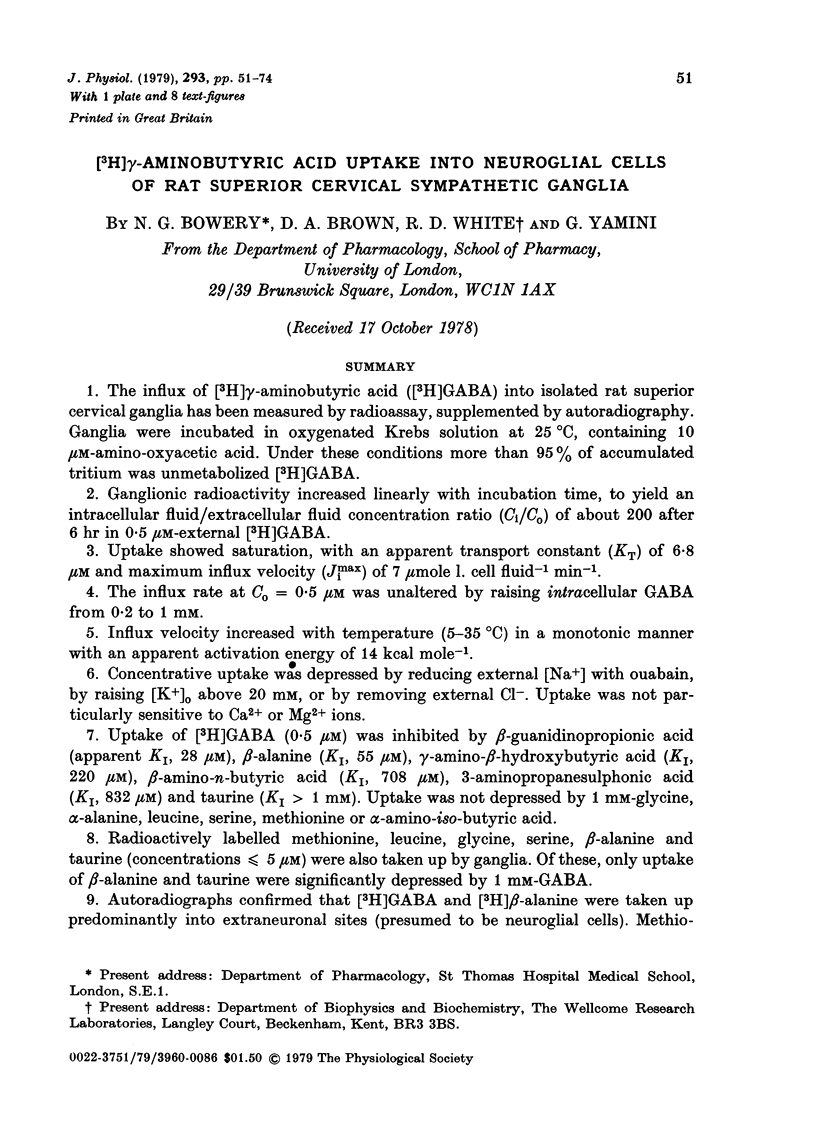
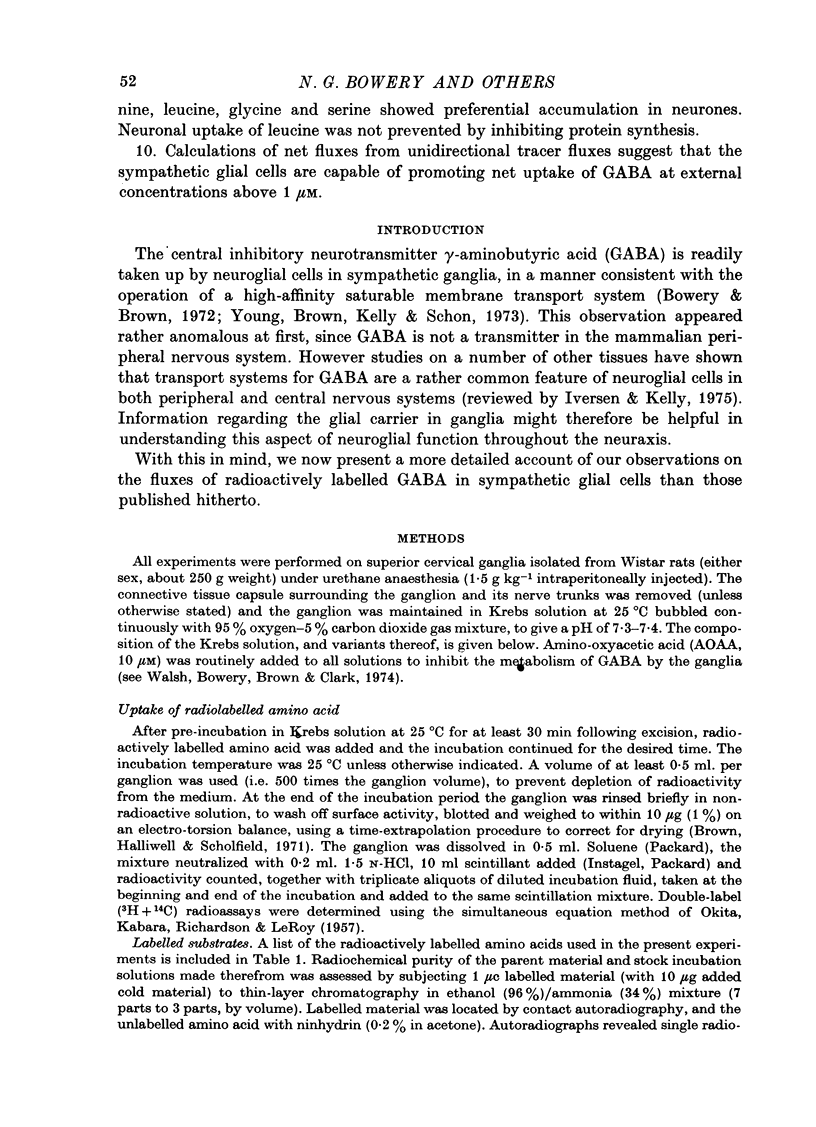
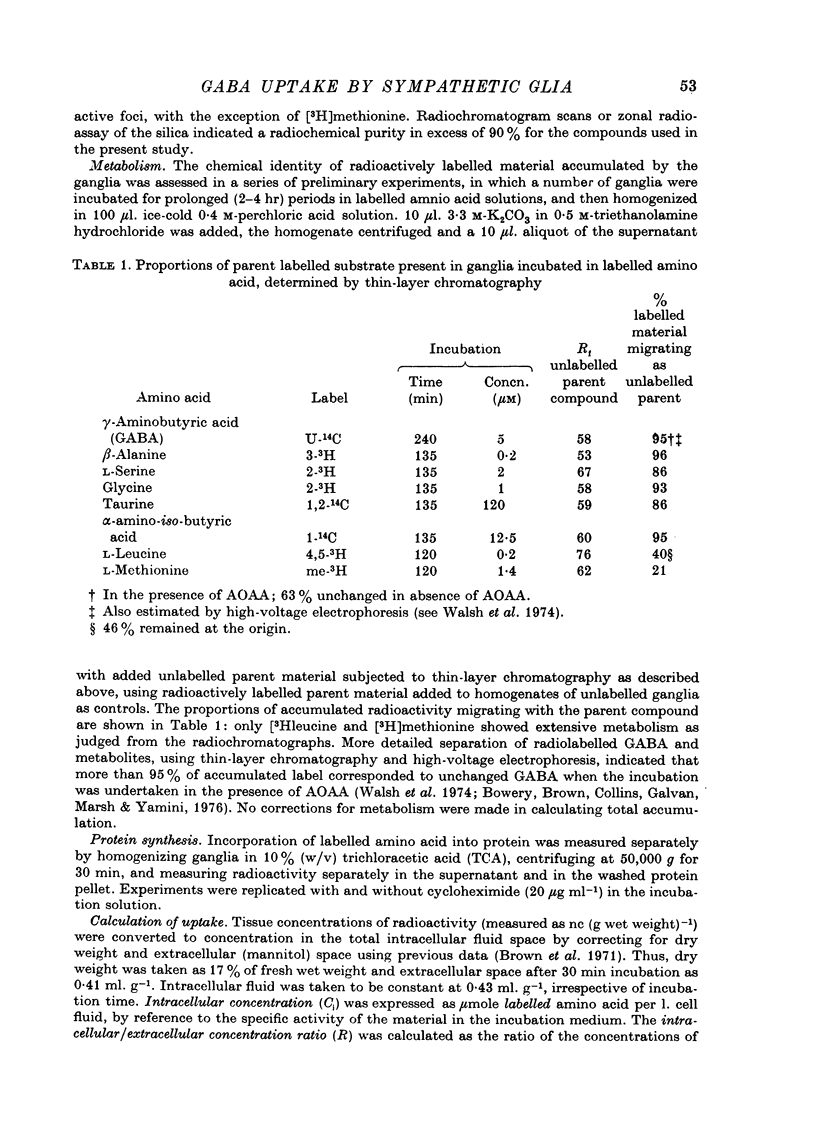
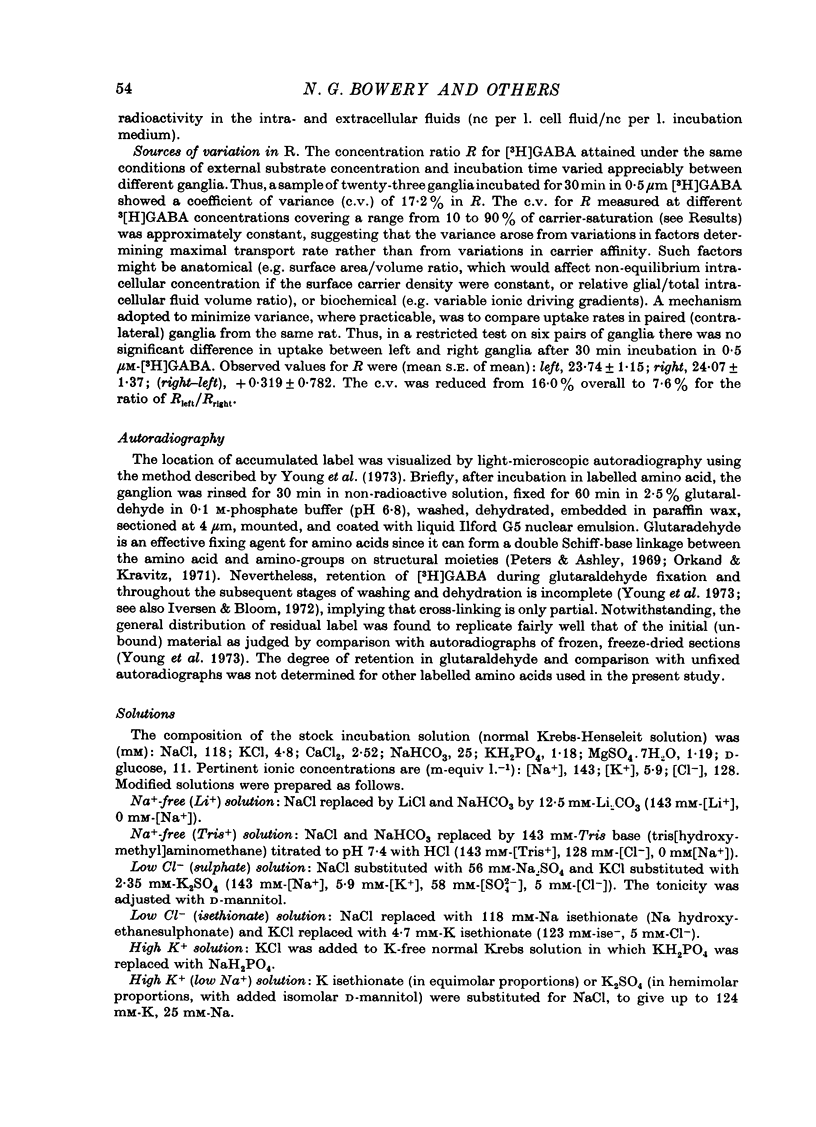
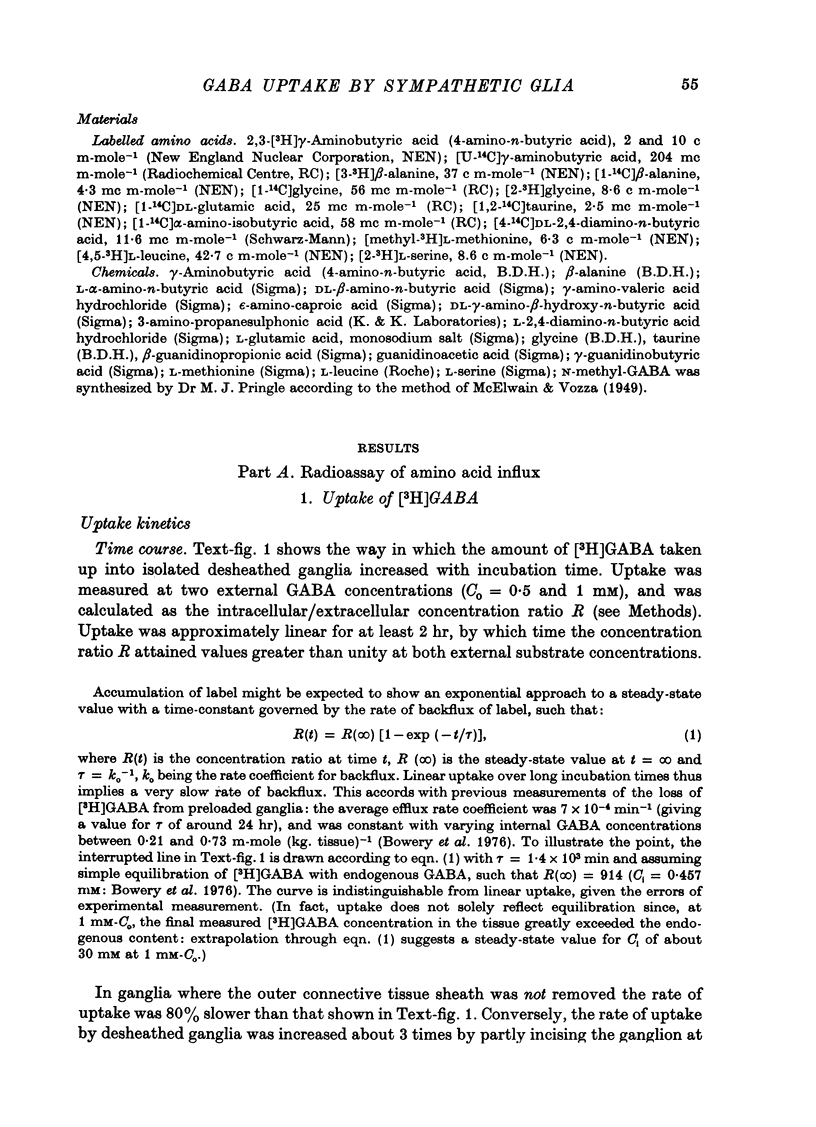
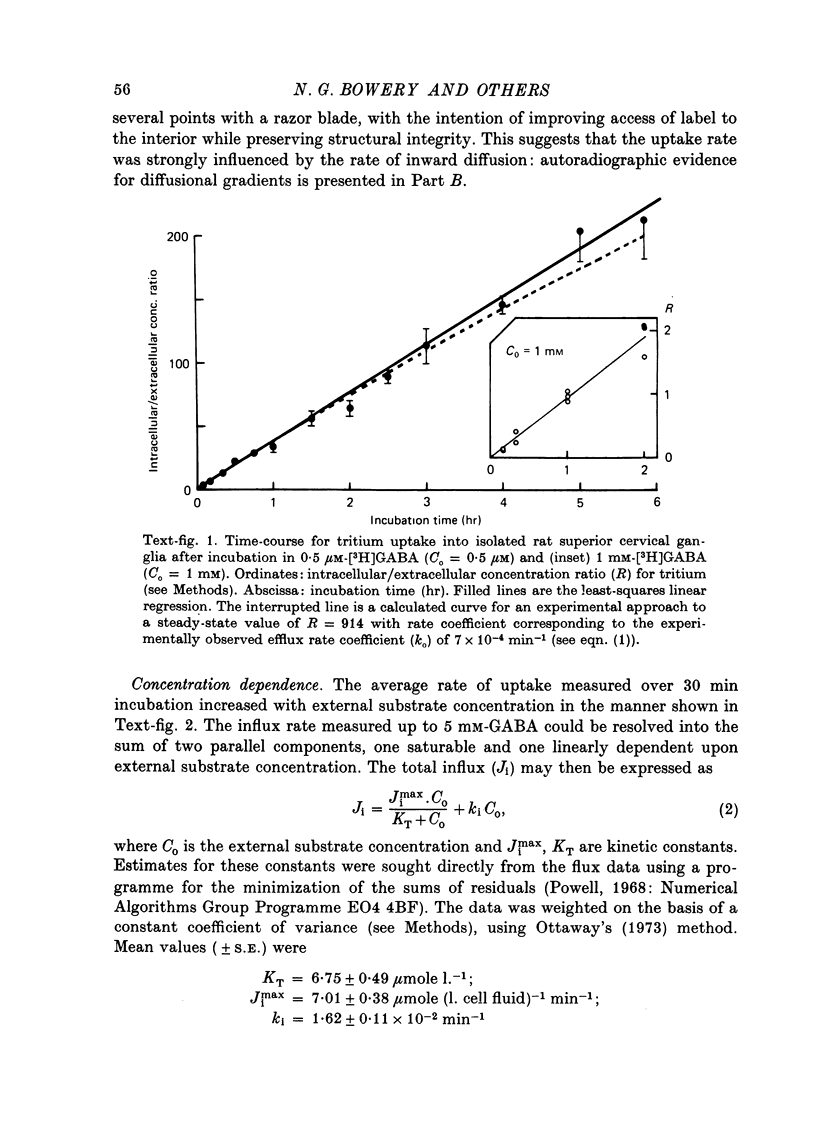
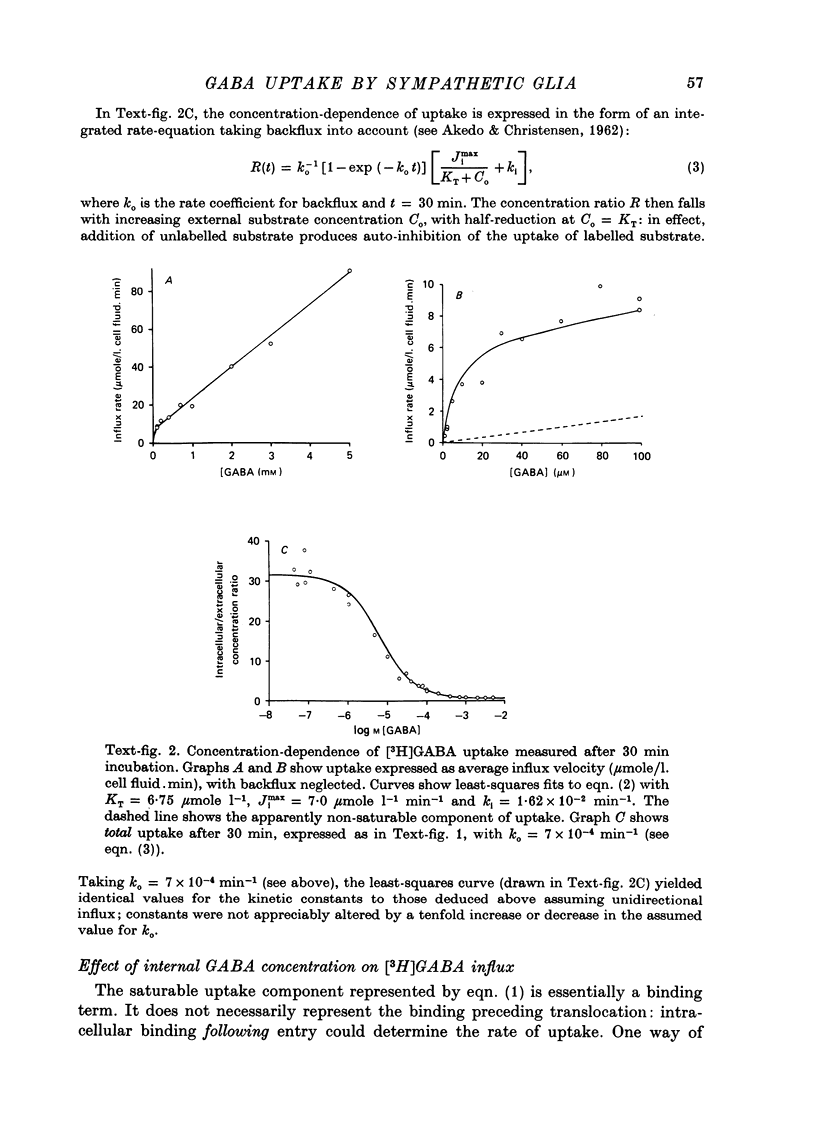
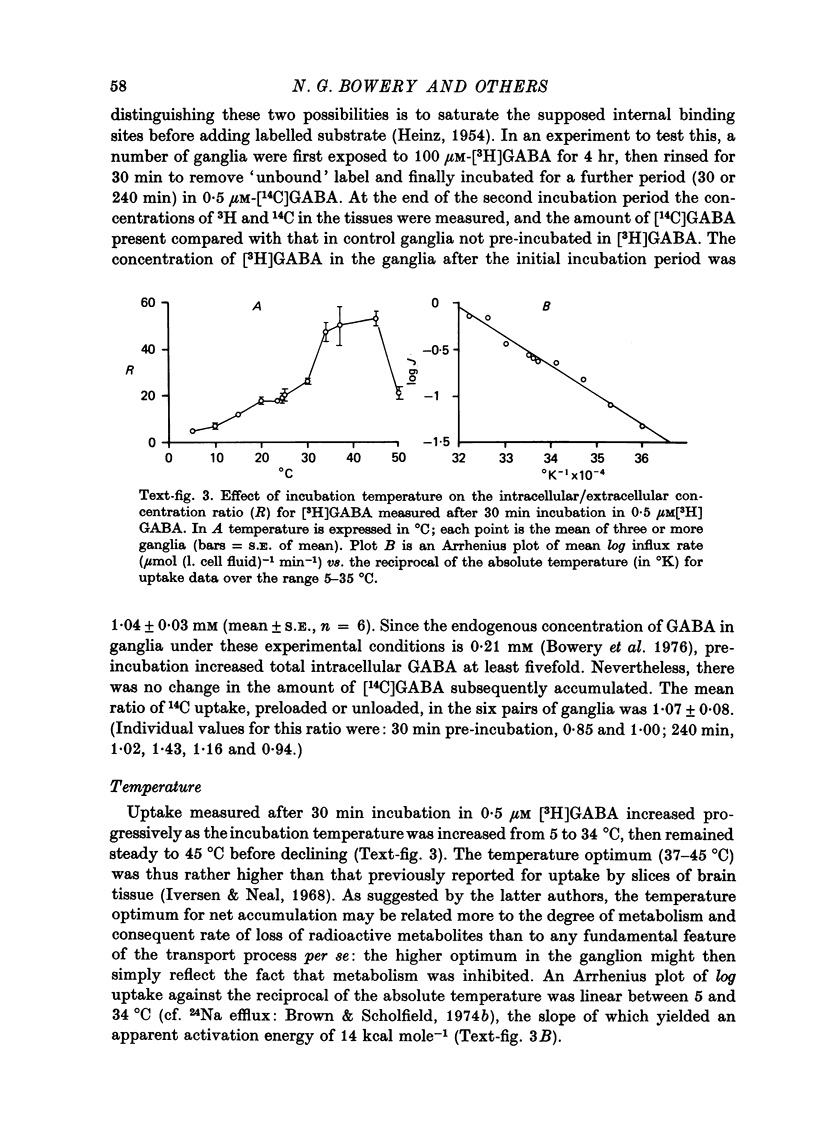
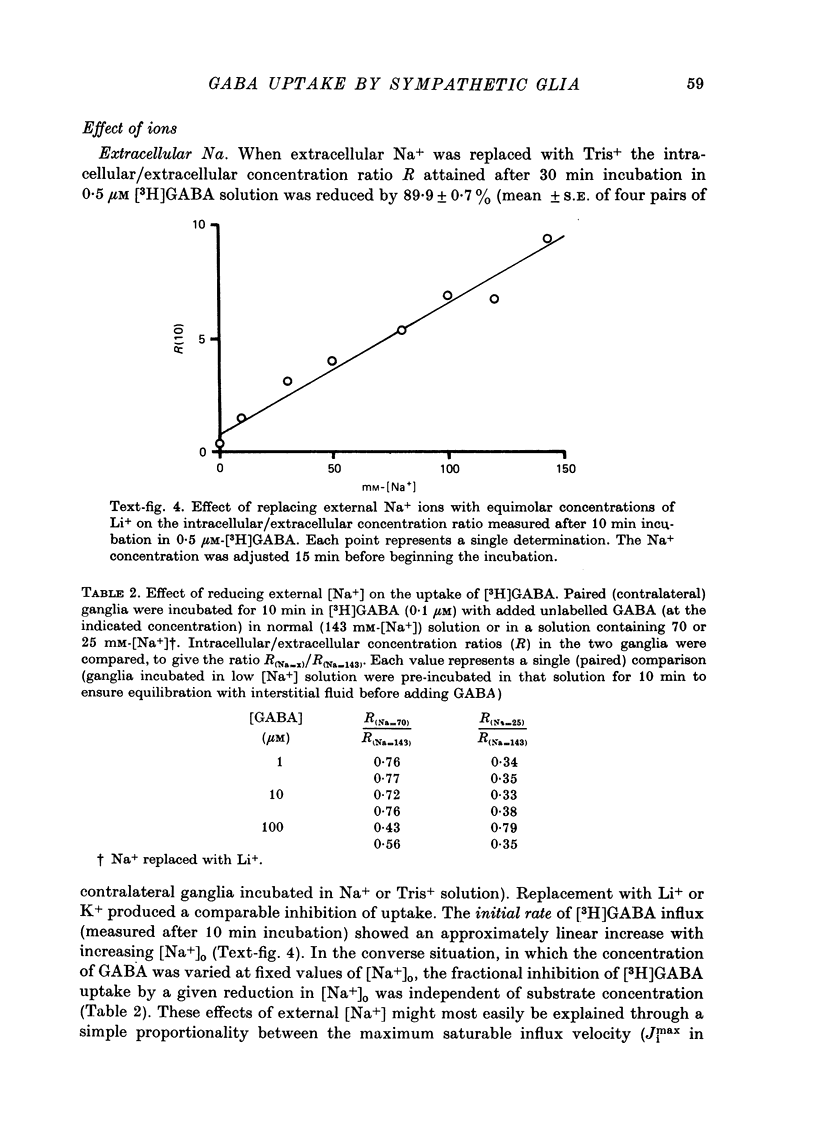
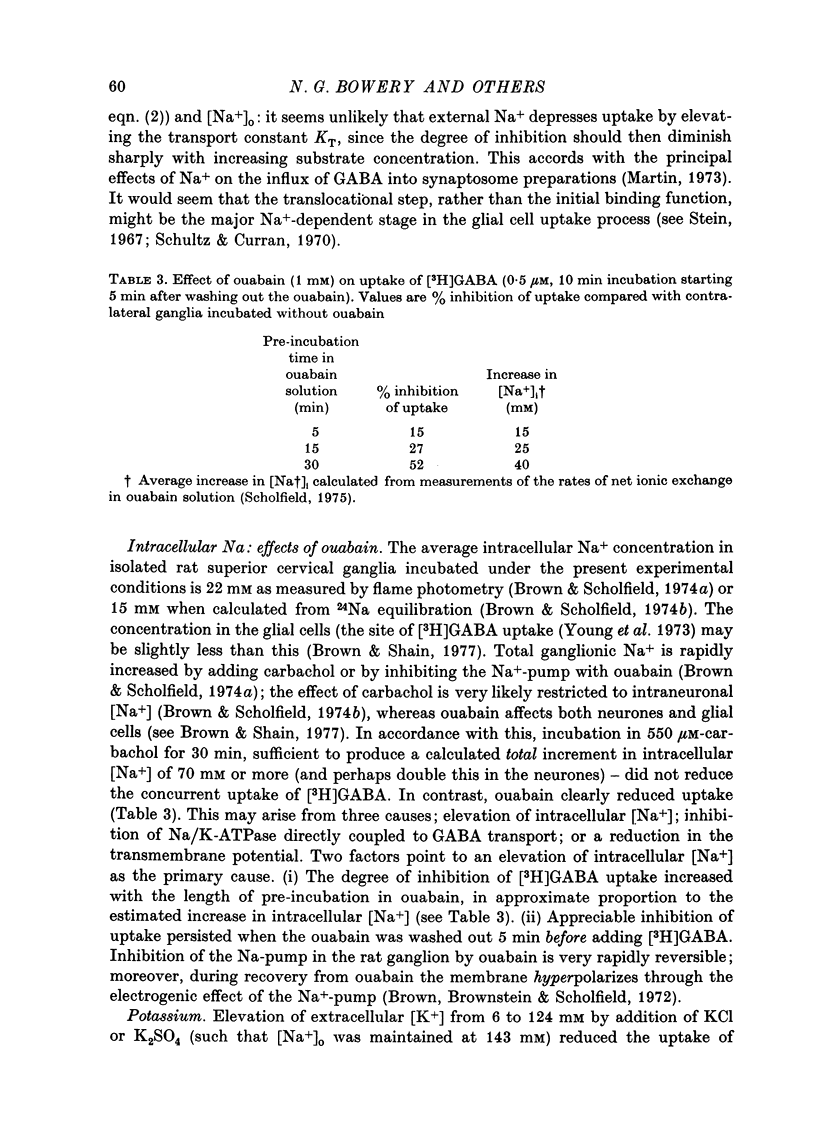
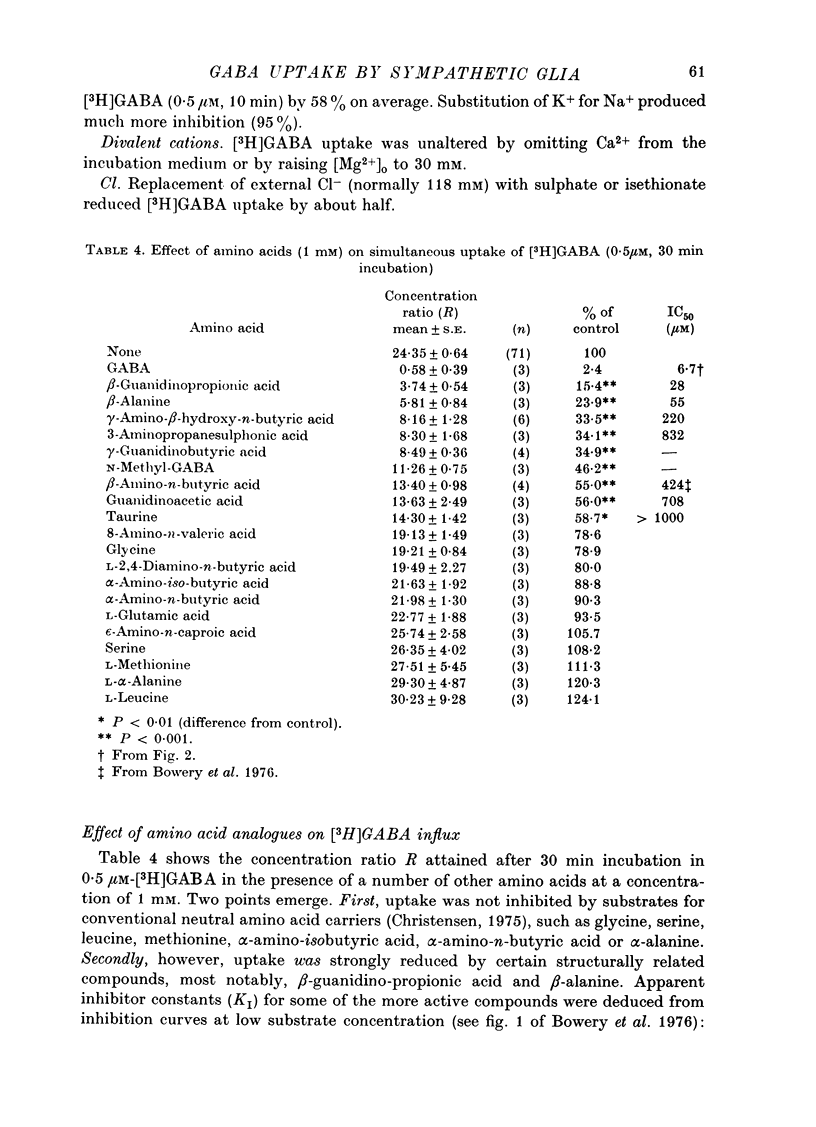
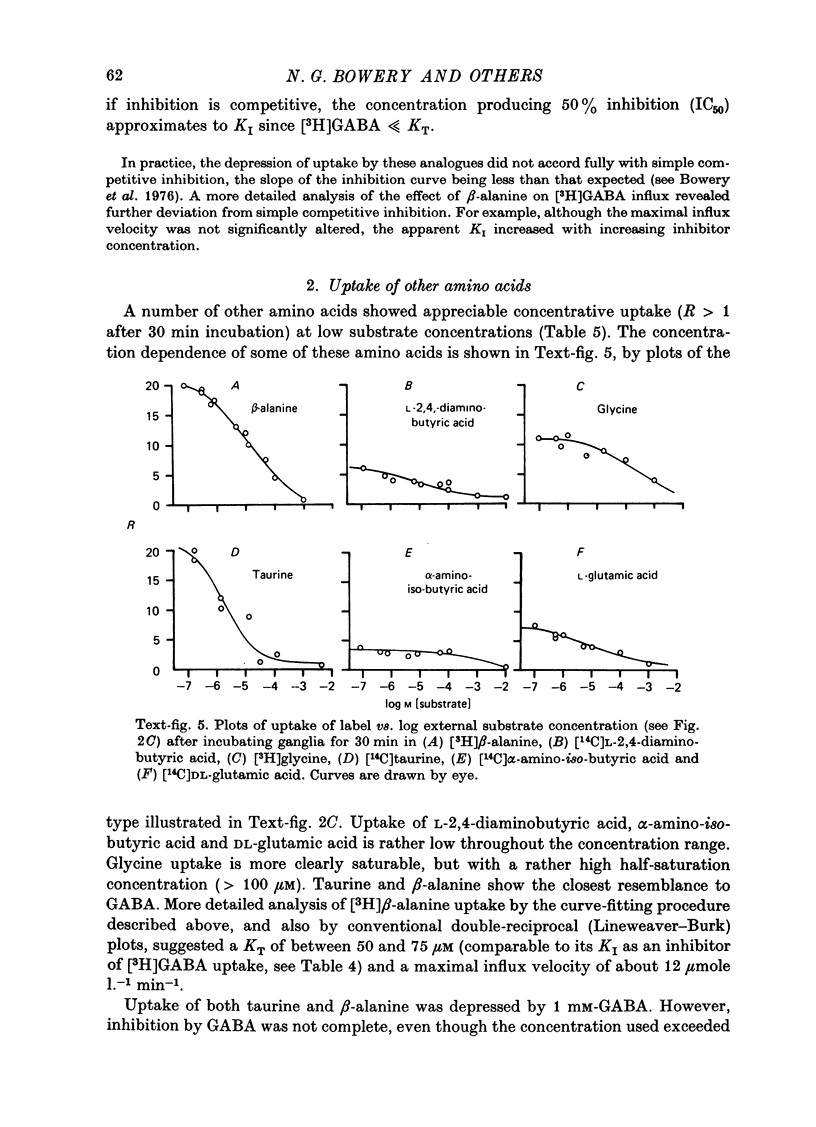
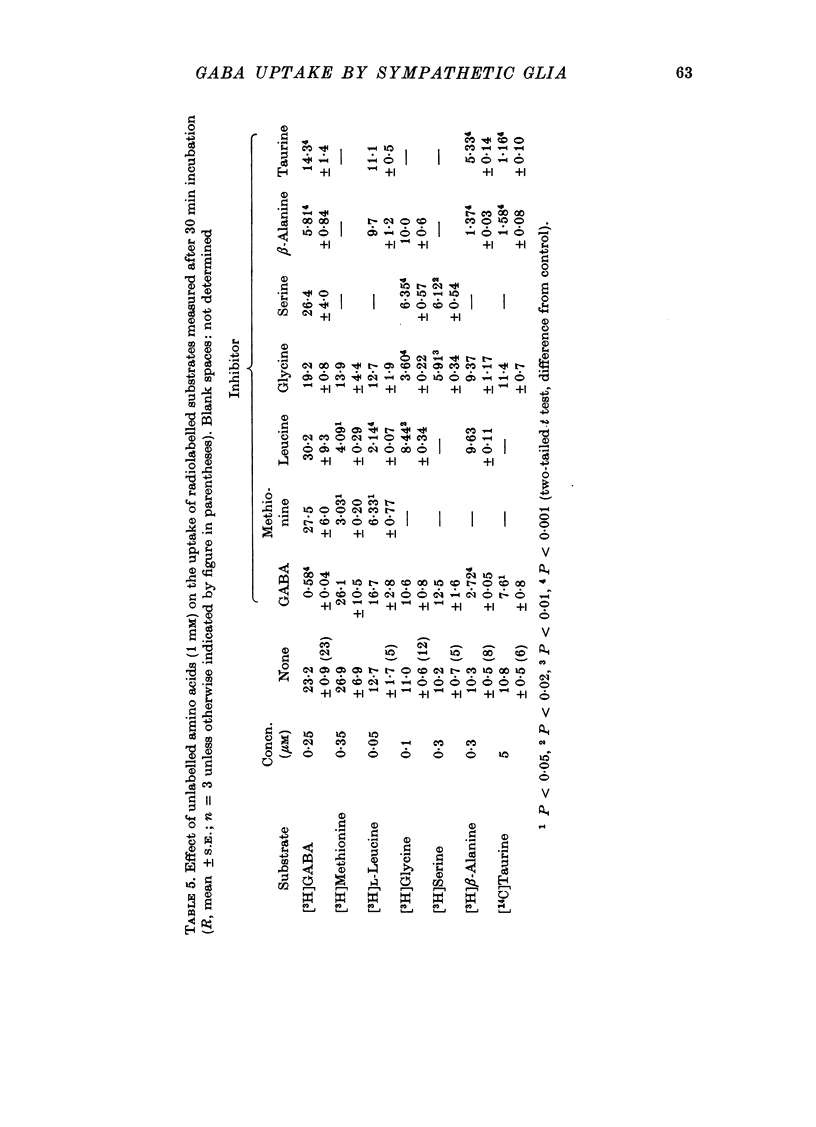
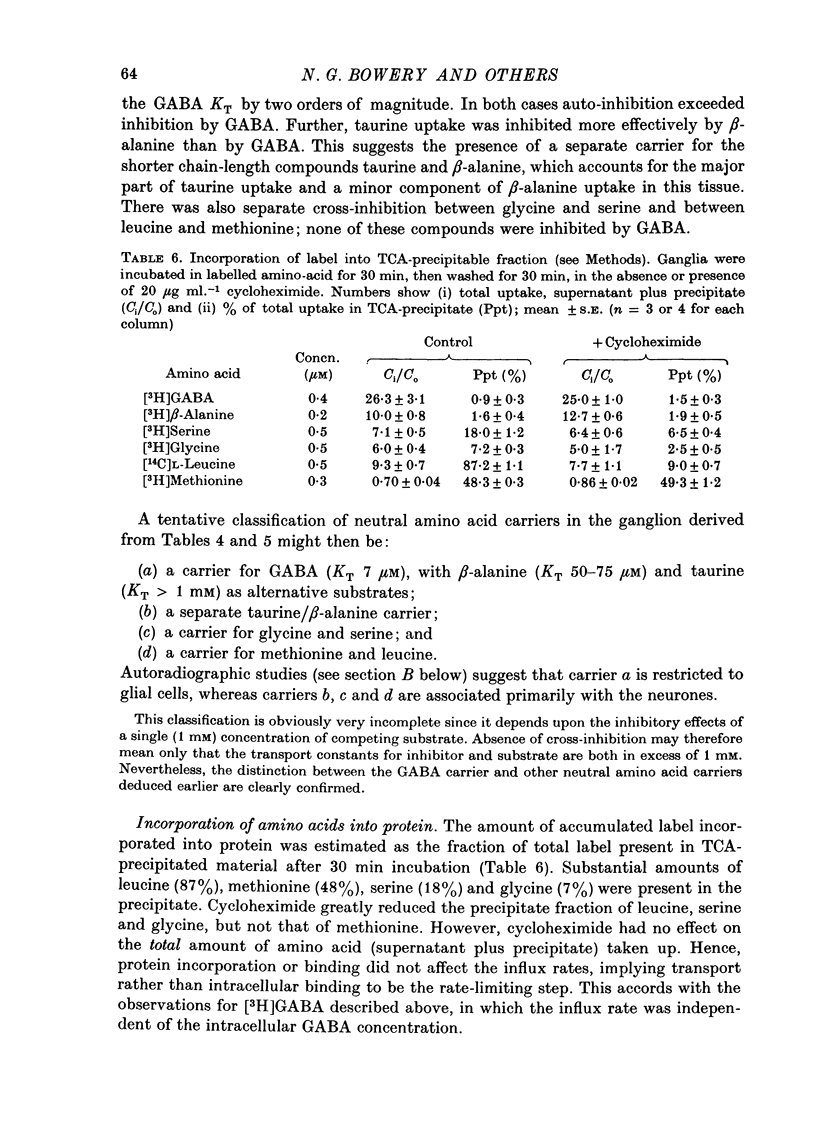
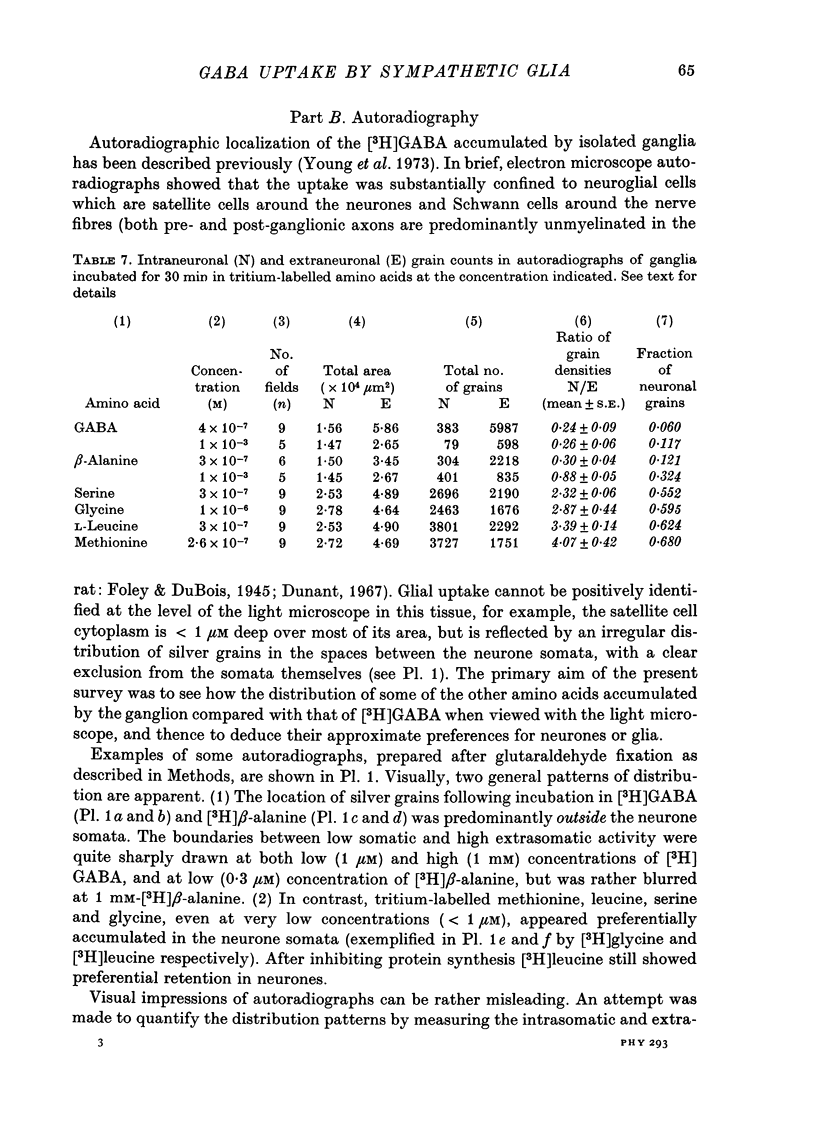
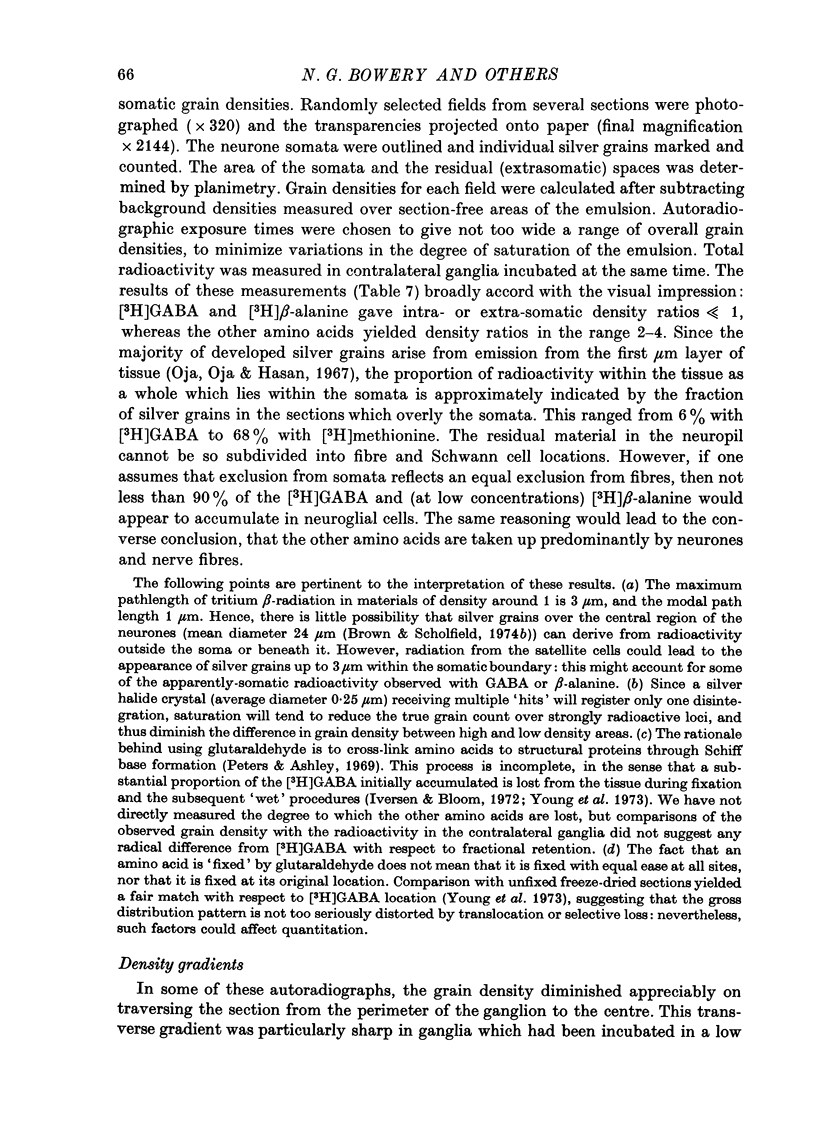
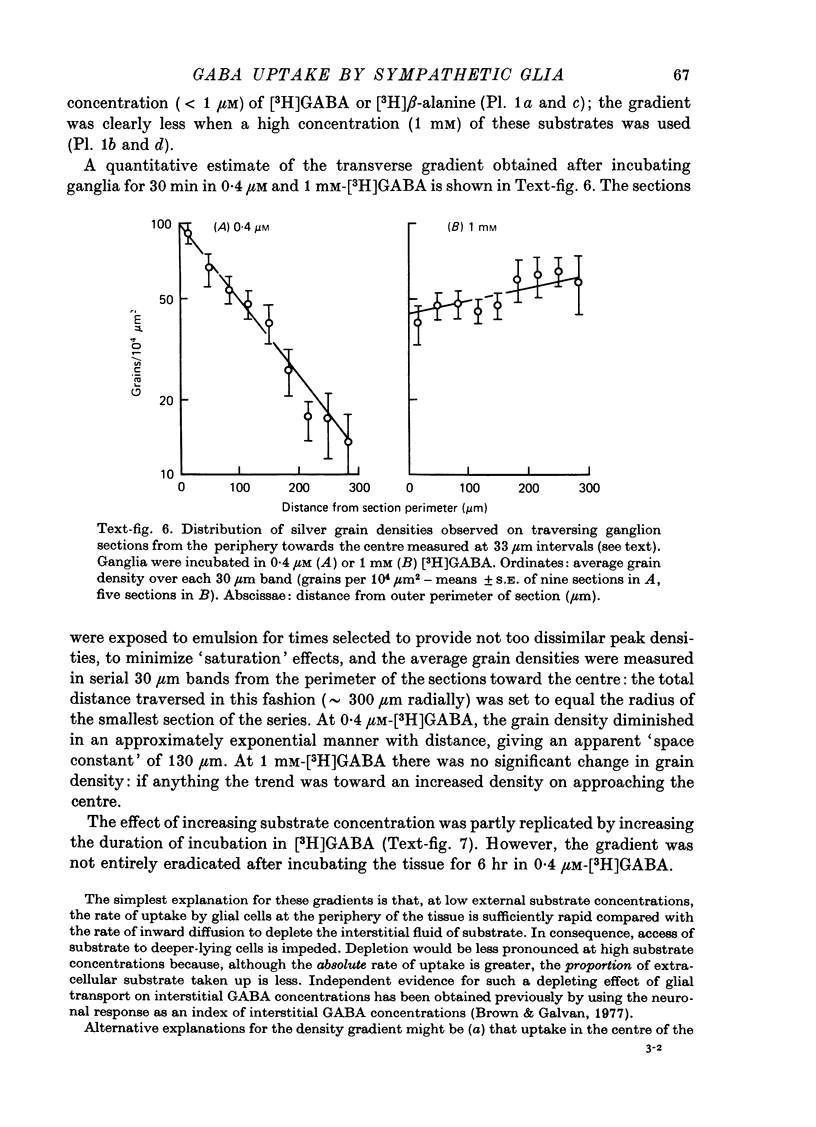
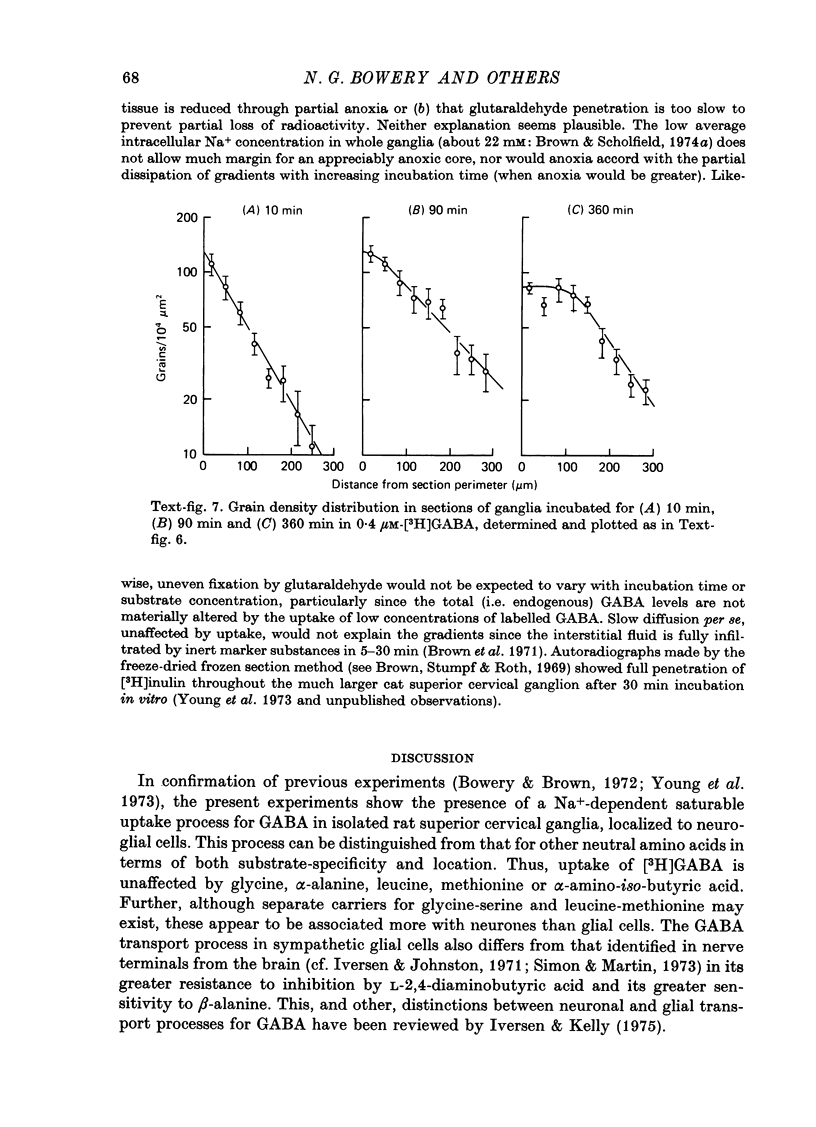
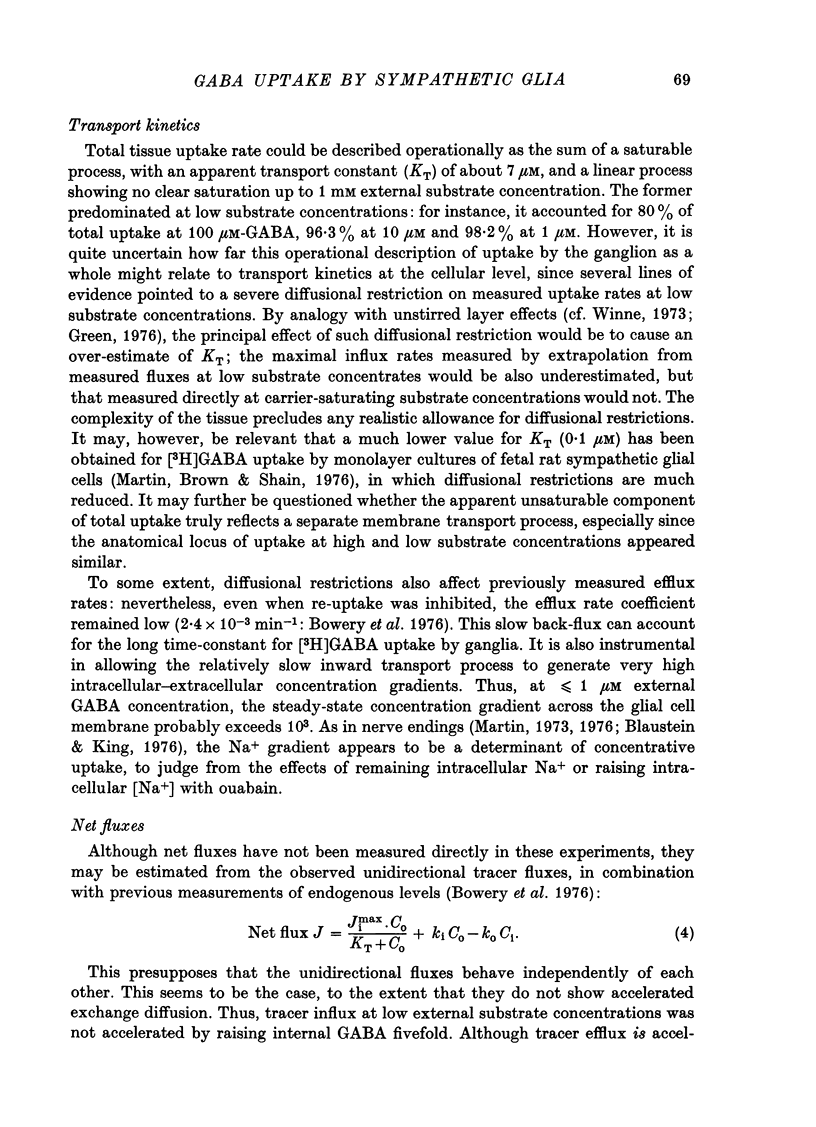
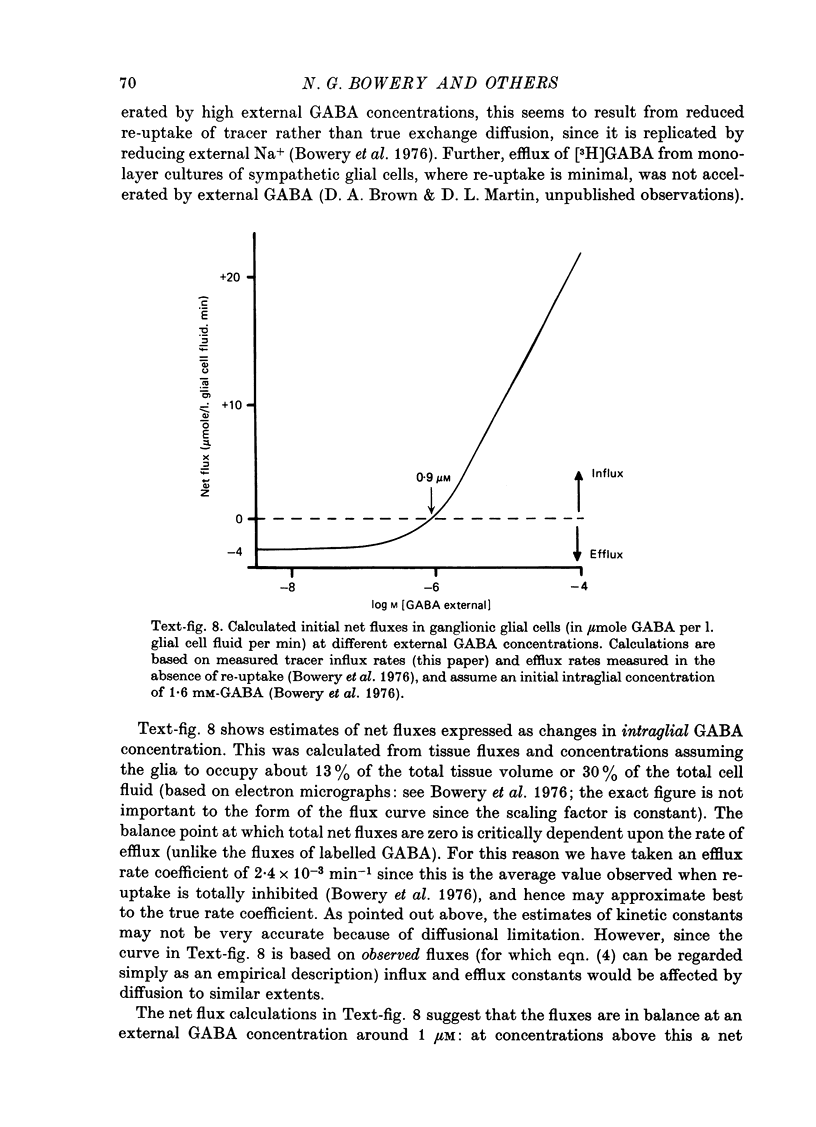
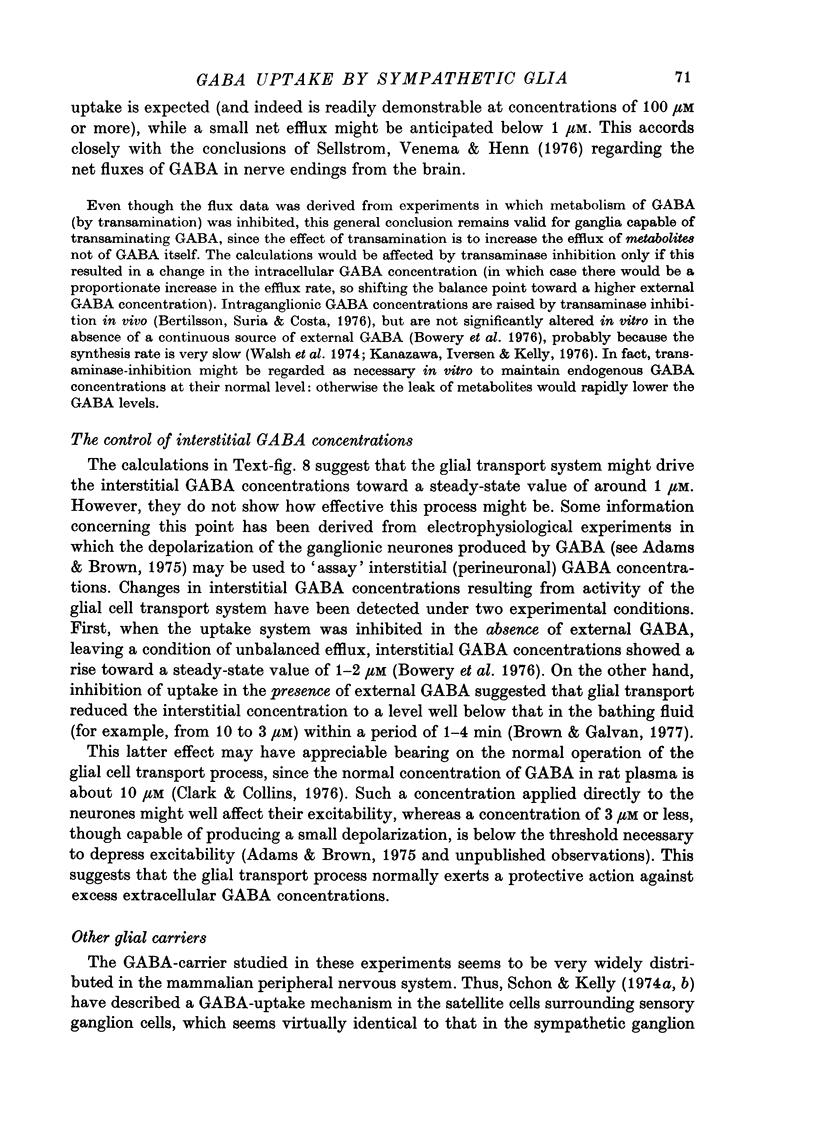
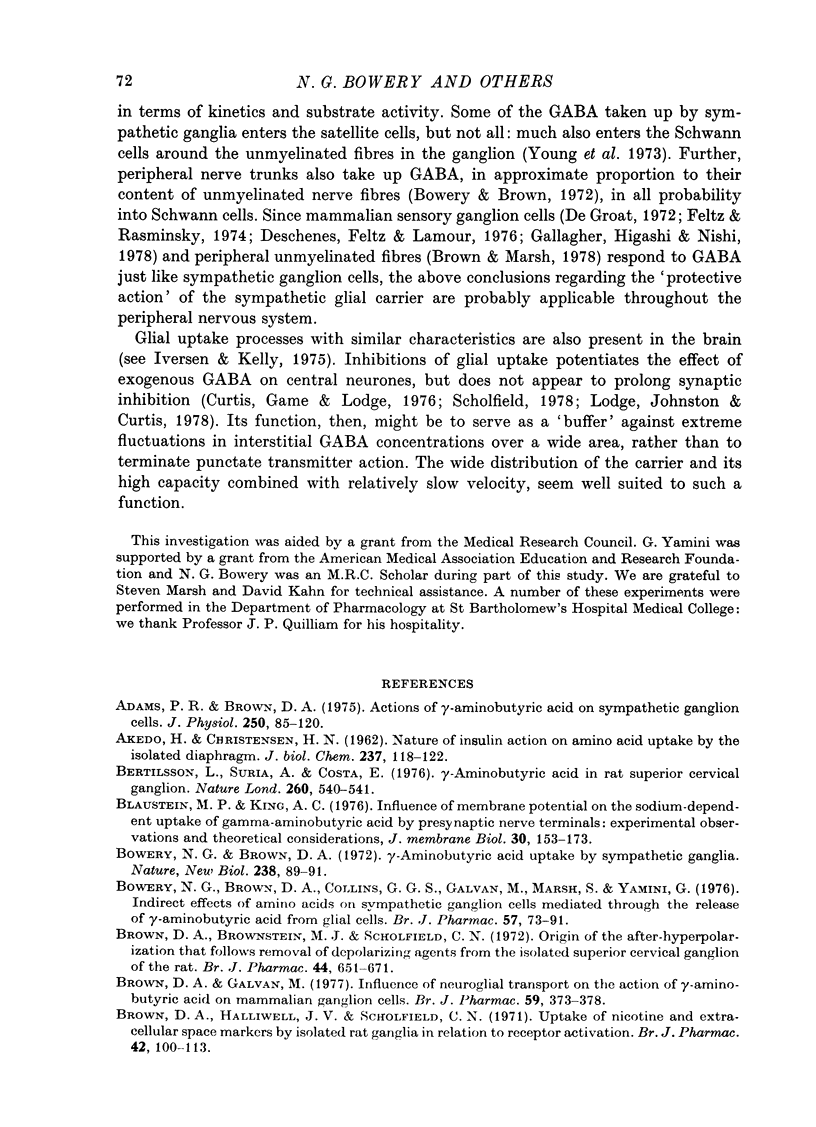
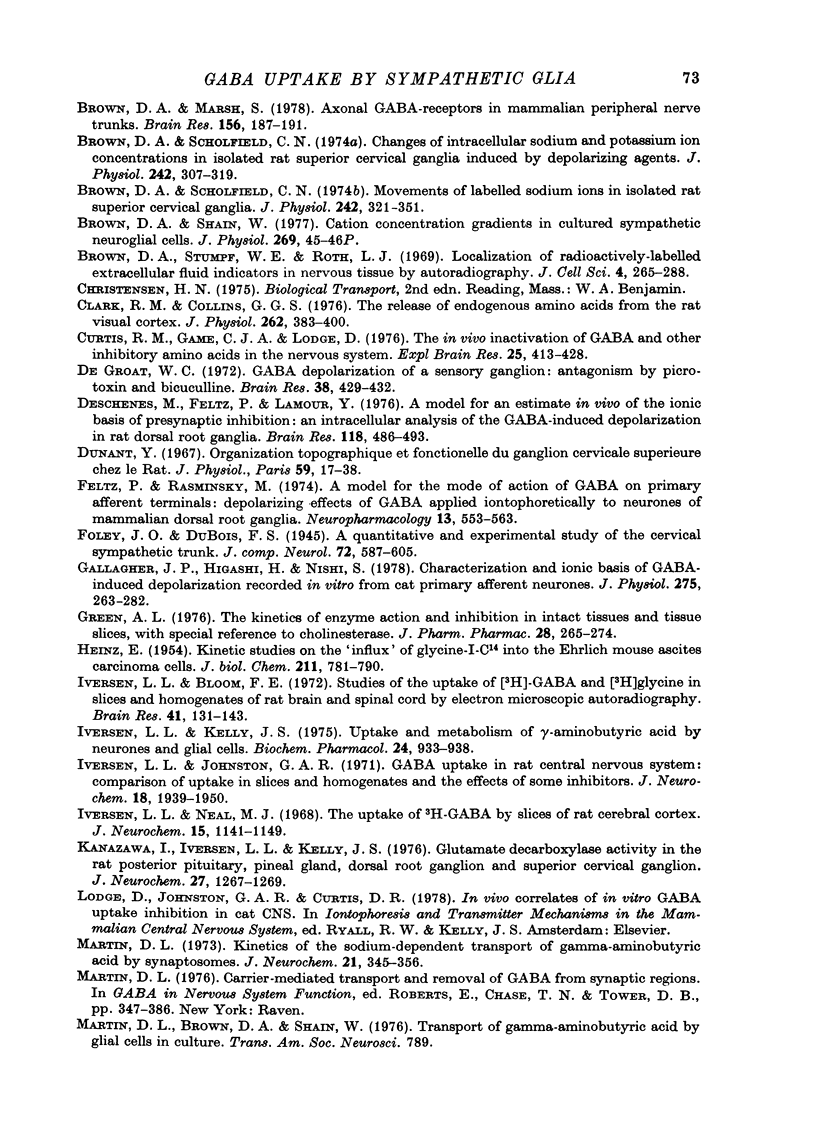
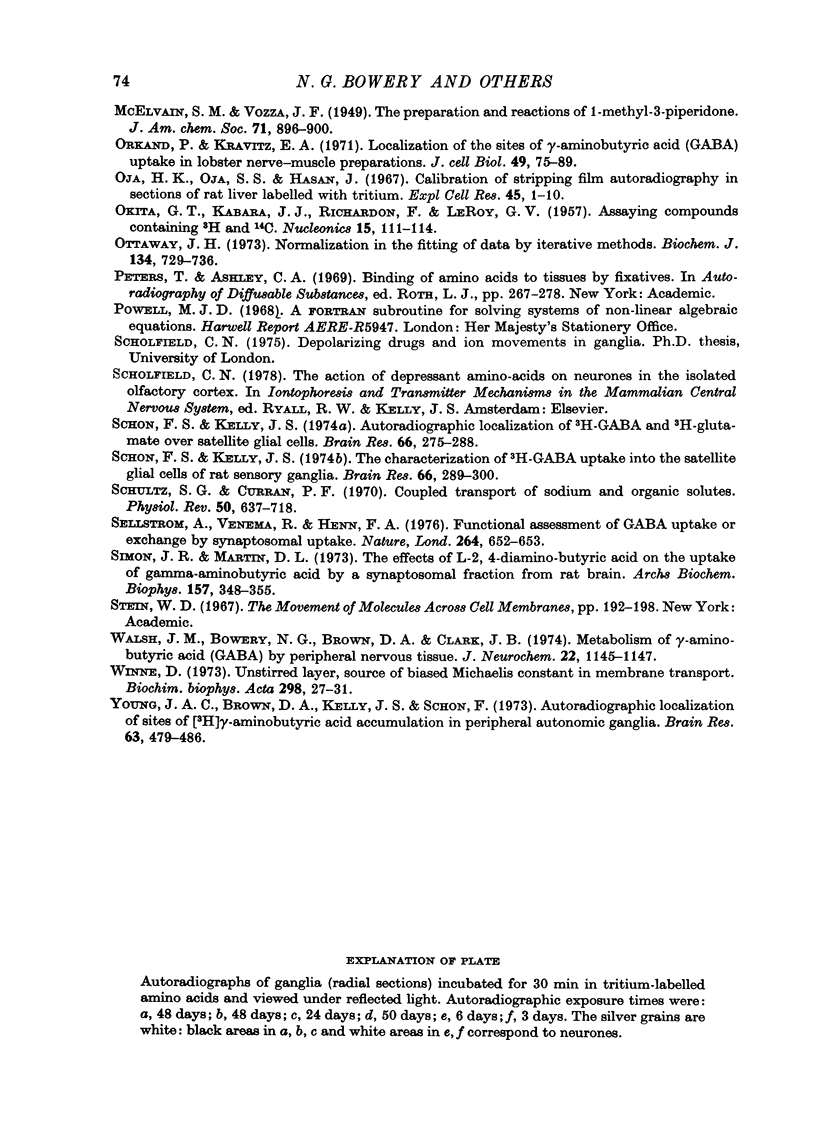
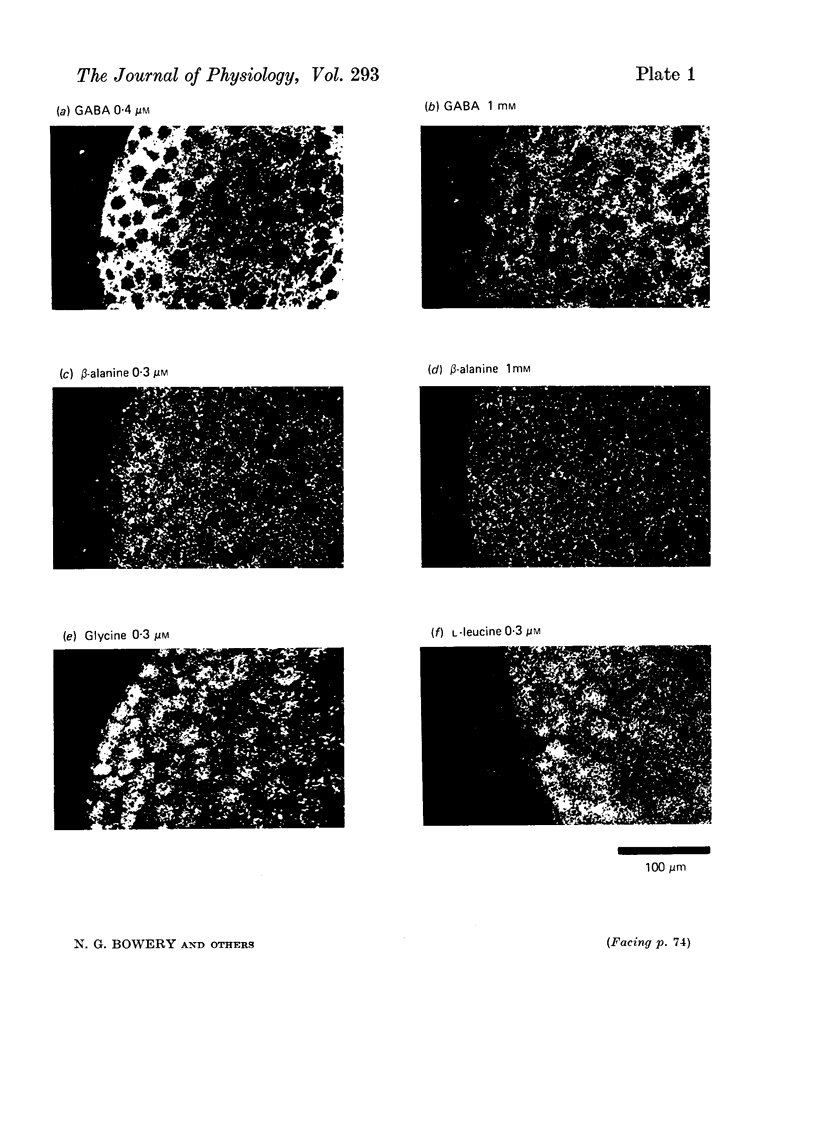
Images in this article
Selected References
These references are in PubMed. This may not be the complete list of references from this article.
- AKEDO H., CHRISTENSEN H. N. Nature of insulin action on amino acid uptake by the isolated diaphragm. J Biol Chem. 1962 Jan;237:118–122. [PubMed] [Google Scholar]
- Adams P. R., Brown D. A. Actions of gamma-aminobutyric acid on sympathetic ganglion cells. J Physiol. 1975 Aug;250(1):85–120. doi: 10.1113/jphysiol.1975.sp011044. [DOI] [PMC free article] [PubMed] [Google Scholar]
- Bertilsson L., Suria A., Costa E. gamma-aminobutyric acid in rat superior cervical ganglion. Nature. 1976 Apr 8;260(5551):540–541. doi: 10.1038/260540a0. [DOI] [PubMed] [Google Scholar]
- Blaustein M. P., King A. C. Influence of membrane potential on the sodium-dependent uptake of gamma-aminobutyric acid by presynaptic nerve terminals: experimental observations and theoretical considerations. J Membr Biol. 1976 Dec 28;30(2):153–173. doi: 10.1007/BF01869665. [DOI] [PubMed] [Google Scholar]
- Bowery N. G., Brown D. A. -Aminobutyric acid uptake by sympathetic ganglia. Nat New Biol. 1972 Jul 19;238(81):89–91. doi: 10.1038/newbio238089a0. [DOI] [PubMed] [Google Scholar]
- Bowery N. G., Brown D. A., Collins G. G., Galvan M., Marsh S., Yamini G. Indirect effects of amino-acids on sympathetic ganglion cells mediated through the release of gamma-aminobutyric acid from glial cells. Br J Pharmacol. 1976 May;57(1):73–91. doi: 10.1111/j.1476-5381.1976.tb07658.x. [DOI] [PMC free article] [PubMed] [Google Scholar]
- Brown D. A., Brownstein M. J., Scholfield C. N. Origin of the after-hyperpolarization that follows removal of depolarizing agents from the isolated superior cervical ganglion of the rat. Br J Pharmacol. 1972 Apr;44(4):651–671. doi: 10.1111/j.1476-5381.1972.tb07305.x. [DOI] [PMC free article] [PubMed] [Google Scholar]
- Brown D. A., Galvan M. Influence of neuroglial transport on the action of gamma-aminobutyric acid on mammalian ganglion cells. Br J Pharmacol. 1977 Feb;59(2):373–378. doi: 10.1111/j.1476-5381.1977.tb07502.x. [DOI] [PMC free article] [PubMed] [Google Scholar]
- Brown D. A., Halliwell J. V., Scholfield C. N. Uptake of nicotine and extracellular space markers by isolated rat ganglia in relation to receptor activation. Br J Pharmacol. 1971 May;42(1):100–113. doi: 10.1111/j.1476-5381.1971.tb07090.x. [DOI] [PMC free article] [PubMed] [Google Scholar]
- Brown D. A., Marsh S. Axonal GABA-receptors in mammalian peripheral nerve trunks. Brain Res. 1978 Nov 3;156(1):187–191. doi: 10.1016/0006-8993(78)90098-7. [DOI] [PubMed] [Google Scholar]
- Brown D. A., Scholfield C. N. Changes of intracellular sodium and potassium ion concentrations in isolated rat superior cervical ganglia induced by depolarizing agents. J Physiol. 1974 Oct;242(2):307–319. doi: 10.1113/jphysiol.1974.sp010709. [DOI] [PMC free article] [PubMed] [Google Scholar]
- Brown D. A., Scholfield C. N. Movements of labelled sodium ions in isolated rat superior cervical ganglia. J Physiol. 1974 Oct;242(2):321–351. doi: 10.1113/jphysiol.1974.sp010710. [DOI] [PMC free article] [PubMed] [Google Scholar]
- Brown D. A., Shain W. Cation concentration gradients in cultured sympathetic neuroglial cells [proceedings]. J Physiol. 1977 Jul;269(1):45P–46P. [PubMed] [Google Scholar]
- Brown D. A., Stumpf W. E., Roth L. J. Location of radioactively labelled extracellular fluid indicators in nervous tissue by autoradiography. J Cell Sci. 1969 Jan;4(1):265–288. doi: 10.1242/jcs.4.1.265. [DOI] [PubMed] [Google Scholar]
- Clark R. M., Collins G. G. The release of endogenous amino acids from the rat visual cortex. J Physiol. 1976 Nov;262(2):383–400. doi: 10.1113/jphysiol.1976.sp011600. [DOI] [PMC free article] [PubMed] [Google Scholar]
- Curtis D. R., Game C. J., Lodge D. The in vivo inactivation of GABA and other inhibitory amino acids in the cat nervous system. Exp Brain Res. 1976 Jun 30;25(4):413–428. doi: 10.1007/BF00241731. [DOI] [PubMed] [Google Scholar]
- De Groat W. C. GABA-depolarization of a sensory ganglion: antagonism by picrotoxin and bicuculline. Brain Res. 1972 Mar 24;38(2):429–432. doi: 10.1016/0006-8993(72)90726-3. [DOI] [PubMed] [Google Scholar]
- Deschenes M., Feltz P., Lamour Y. A model for an estimate in vivo of the ionic basis of presynaptic inhibition: an intracellular analysis of the GABA-induced depolarization in rat dorsal root ganglia. Brain Res. 1976 Dec 24;118(3):486–493. doi: 10.1016/0006-8993(76)90318-8. [DOI] [PubMed] [Google Scholar]
- Dunant Y. Organisation topographique et fonctionnelle du ganglion cervical supérieur chez le Rat. J Physiol (Paris) 1967 Jan-Feb;59(1):17–38. [PubMed] [Google Scholar]
- Feltz P., Rasminsky M. A model for the mode of action of GABA on primary afferent terminals: depolarizing effects of GABA applied iontophoretically to neurones of mammalian dorsal root ganglia. Neuropharmacology. 1974 Jun;13(6):553–563. doi: 10.1016/0028-3908(74)90145-2. [DOI] [PubMed] [Google Scholar]
- Gallagher J. P., Higashi H., Nishi S. Characterization and ionic basis of GABA-induced depolarizations recorded in vitro from cat primary afferent neurones. J Physiol. 1978 Feb;275:263–282. doi: 10.1113/jphysiol.1978.sp012189. [DOI] [PMC free article] [PubMed] [Google Scholar]
- Green A. L. The kinetics of enzyme action and inhibition in intact tissues and tissue slices, with special reference to cholinesterase. J Pharm Pharmacol. 1976 Apr;28(4):265–274. doi: 10.1111/j.2042-7158.1976.tb04151.x. [DOI] [PubMed] [Google Scholar]
- HEINZ E. Kinetic studies on the influx of glycine-1-C14 into the Ehrlich mouse ascites carcinoma cell. J Biol Chem. 1954 Dec;211(2):781–790. [PubMed] [Google Scholar]
- Iversen L. L., Bloom F. E. Studies of the uptake of 3 H-gaba and ( 3 H)glycine in slices and homogenates of rat brain and spinal cord by electron microscopic autoradiography. Brain Res. 1972 Jun 8;41(1):131–143. doi: 10.1016/0006-8993(72)90621-x. [DOI] [PubMed] [Google Scholar]
- Iversen L. L., Johnston G. A. GABA uptake in rat central nervous system: comparison of uptake in slices and homogenates and the effects of some inhibitors. J Neurochem. 1971 Oct;18(10):1939–1950. doi: 10.1111/j.1471-4159.1971.tb09600.x. [DOI] [PubMed] [Google Scholar]
- Iversen L. L., Kelly J. S. Uptake and metabolism of gamma-aminobutyric acid by neurones and glial cells. Biochem Pharmacol. 1975 May 1;24(9):933–938. doi: 10.1016/0006-2952(75)90422-0. [DOI] [PubMed] [Google Scholar]
- Iversen L. L., Neal M. J. The uptake of [3H]GABA by slices of rat cerebral cortex. J Neurochem. 1968 Oct;15(10):1141–1149. doi: 10.1111/j.1471-4159.1968.tb06831.x. [DOI] [PubMed] [Google Scholar]
- Kanazawa I., Iversen L. L., Kelly J. S. Glutamate decarboxylase activity in the rat posterior pituitary, pineal gland, dorsal root ganglion and superior cervical ganglion. J Neurochem. 1976 Nov;27(5):1267–1269. doi: 10.1111/j.1471-4159.1976.tb00341.x. [DOI] [PubMed] [Google Scholar]
- Martin D. L. Kinetics of the sodium-dependent transport of gamma-aminobutyric acid by synaptosomes. J Neurochem. 1973 Aug;21(2):345–356. doi: 10.1111/j.1471-4159.1973.tb04255.x. [DOI] [PubMed] [Google Scholar]
- Oja H. K., Oja S. S., Hasan J. Calibration of stripping film autoradiography in sections of rat liver labelled with tritium. Exp Cell Res. 1967 Jan;45(1):1–10. doi: 10.1016/0014-4827(67)90106-1. [DOI] [PubMed] [Google Scholar]
- Orkand P. M., Kravitz E. A. Localization of the sites of gamma-aminobutyric acid (GABA) uptake in lobster nerve-muscle preparations. J Cell Biol. 1971 Apr;49(1):75–89. doi: 10.1083/jcb.49.1.75. [DOI] [PMC free article] [PubMed] [Google Scholar]
- Ottaway J. H. Normalization in the fitting of data by iterative methods. Application to tracer kinetics and enzyme kinetics. Biochem J. 1973 Jul;134(3):729–736. doi: 10.1042/bj1340729. [DOI] [PMC free article] [PubMed] [Google Scholar]
- Schultz S. G., Curran P. F. Coupled transport of sodium and organic solutes. Physiol Rev. 1970 Oct;50(4):637–718. doi: 10.1152/physrev.1970.50.4.637. [DOI] [PubMed] [Google Scholar]
- Sellstrom A., Venema R., Henn F. Functional assessment of GABA uptake or exchange by synaptosomal fractions. Nature. 1976 Dec 16;264(5587):652–653. doi: 10.1038/264652a0. [DOI] [PubMed] [Google Scholar]
- Simon J. R., Martin D. L. The effects of L-2,4-diaminobutyric acid on the uptake of gamma-aminobutyric acid by a synaptosomal fraction from rat brain. Arch Biochem Biophys. 1973 Aug;157(2):348–355. doi: 10.1016/0003-9861(73)90649-8. [DOI] [PubMed] [Google Scholar]
- Walsh J. M., Bowery N. G., Brown D. A., Clark J. B. Metabolism of gamma-aminobutyric acid (GABA) by peripheral nervous tissue. J Neurochem. 1974 Jun;22(6):1145–1147. doi: 10.1111/j.1471-4159.1974.tb04350.x. [DOI] [PubMed] [Google Scholar]
- Winne D. Unstirred layer, source of biased Michaelis constant in membrane transport. Biochim Biophys Acta. 1973 Feb 27;298(1):27–31. doi: 10.1016/0005-2736(73)90005-9. [DOI] [PubMed] [Google Scholar]
- Young J. A., Brown D. A., Kelly J. S., Schon F. Autoradiographic localization of sites of (3H)gamma-aminobutyric acid accumulation in peripheral autonomic ganglia. Brain Res. 1973 Dec 7;63:479–486. doi: 10.1016/0006-8993(73)90128-5. [DOI] [PubMed] [Google Scholar]