Abstract
From analyses of the magnetic field dependence of 1/T1 (nuclear magnetic relaxation dispersion [NMRD] profiles) of water protons in solutions of highly purified calf lens gamma II-crystallin, we find that monomers form oligomers at relatively low concentrations, which increase in size with increasing concentration and decreasing temperature. At approximately 16% by volume and -4 degrees C, the mean oligomeric molecular weight is approximately 120-fold greater than the monomeric value of 20 kD. Below this concentration, there is no indication of any substantive change in conformation of the monomeric subunits. At higher concentrations, the tertiary structure of the monomer appears to reconfigure rather abruptly, but reversibly, as evidenced by the appearance of spectra-like 14N peaks in the NMRD profiles. The magnitudes of these peaks, known to arise from cross-relaxation of water protons through access to amide (NH) moieties of the protein backbone, indicate that the high concentration conformation is not compact, but open and extended in a manner that allows enhanced interaction with solvent. The data are analogous to those found for homogenates of calf and chicken lens (Beaulieu, C. F., J. I. Clark, R. D. Brown III, M. Spiller, and S. H. Koenig. 1988. Magn. Reson. Med. 8:47-57; Beaulieu, C. F., R. D. Brown III, J. I. Clark, M. Spiller, and S. H. Koenig. 1989. Magn. Reson. Med. 10:62-72). This unusually large dependence of oligomeric size and conformation on concentration in the physiological range is suggested as the mechanism by which osmotic equilibrium is maintained, at minimal metabolic expense, in the presence of large gradients of protein concentration in the lens in vivo (cf Vérétout and Tardieu, 1989. Eur. Biophys. J. 17:61-68). Finally, the results of the NMRD data provide a ready explanation of the low temperature phase transition, and "cold-cataract" separation of phases, observed in gamma II-crystallin solutions; we suggest that the phases that separate are the two major conformers detected by NMRD.
Full text
PDF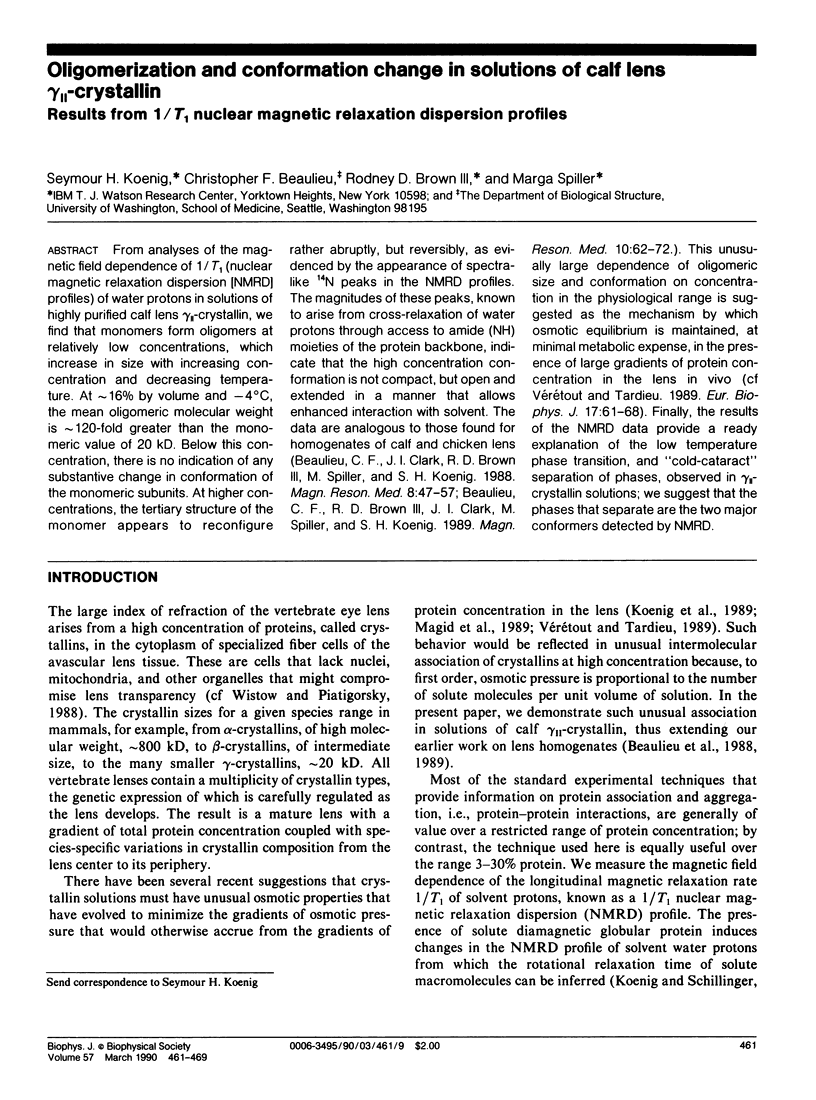
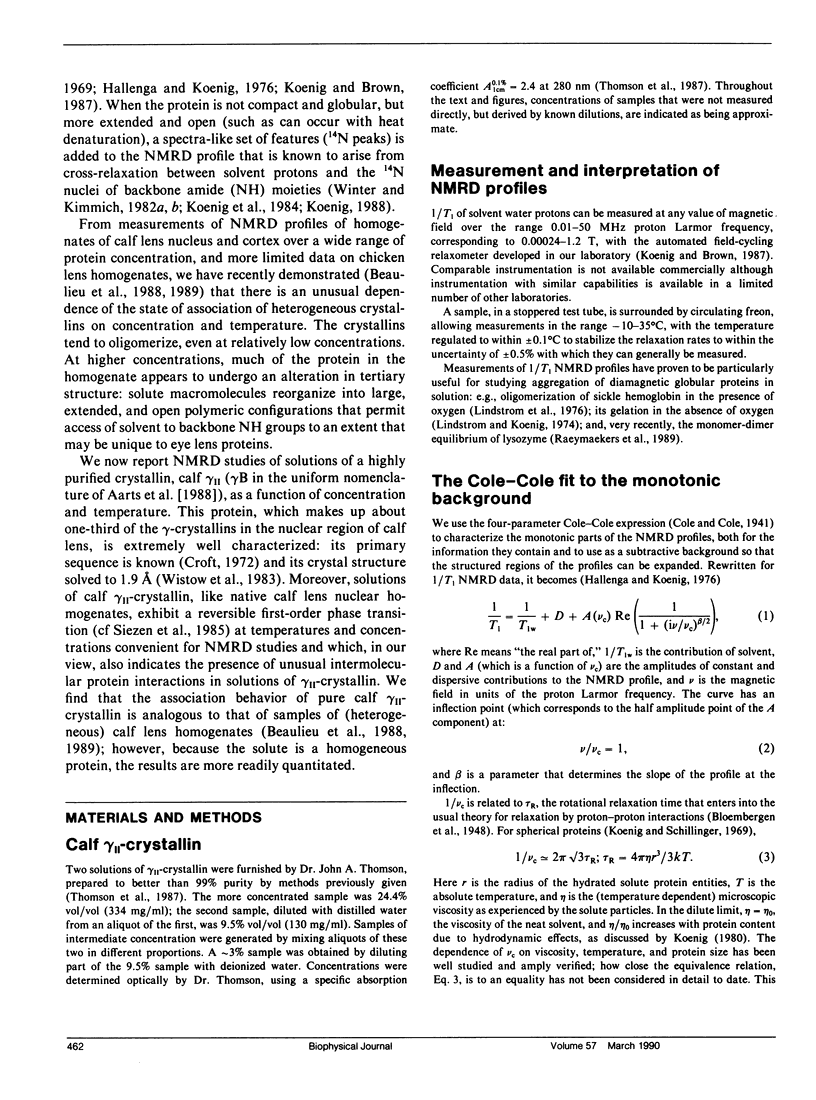
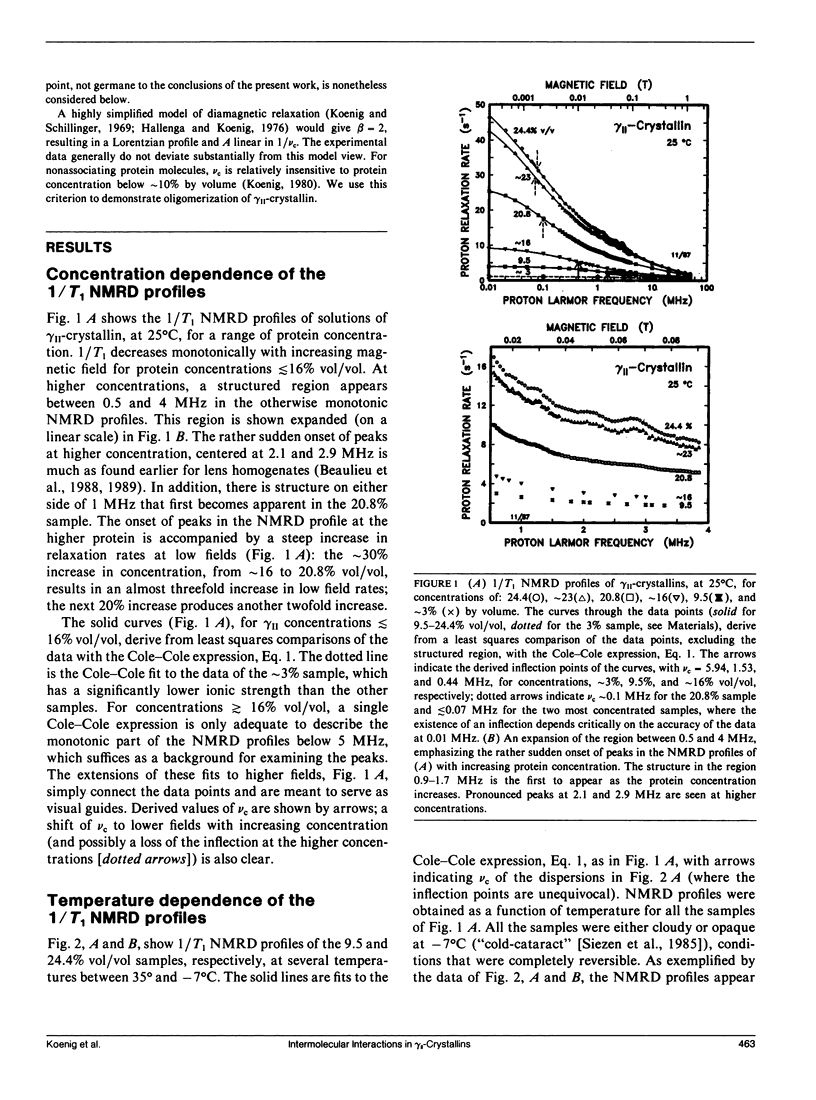
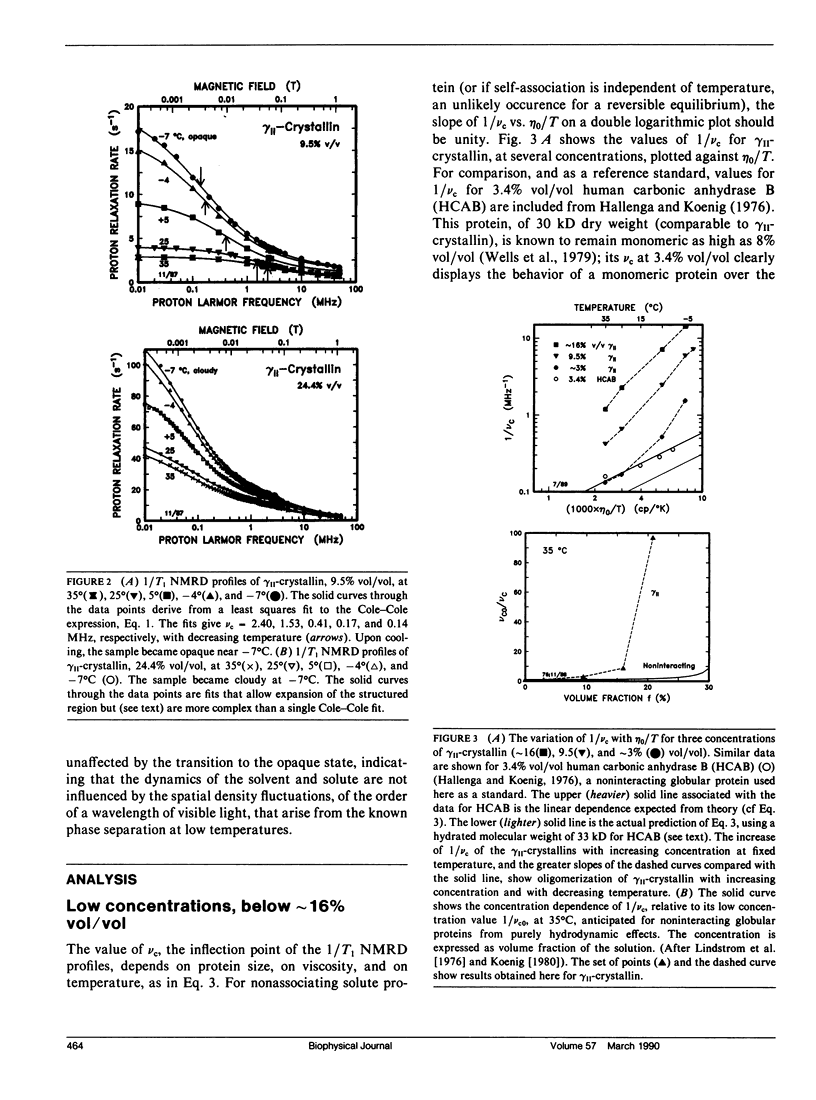
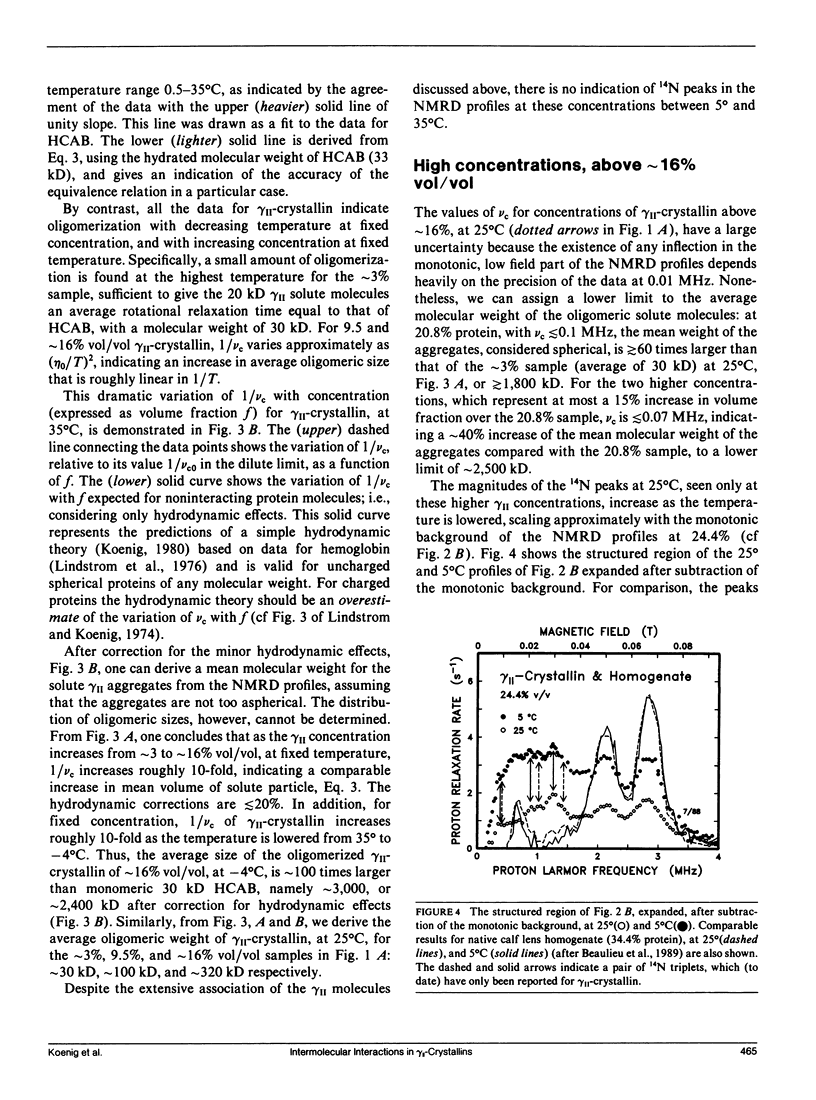
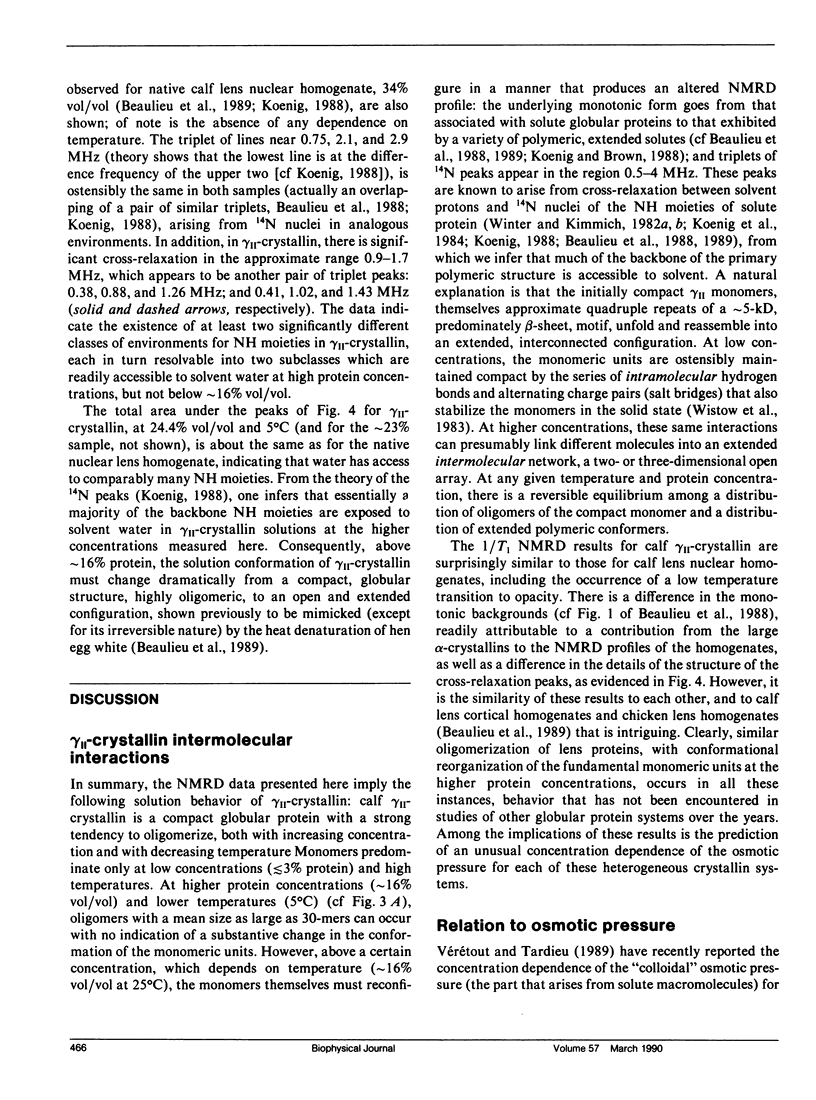
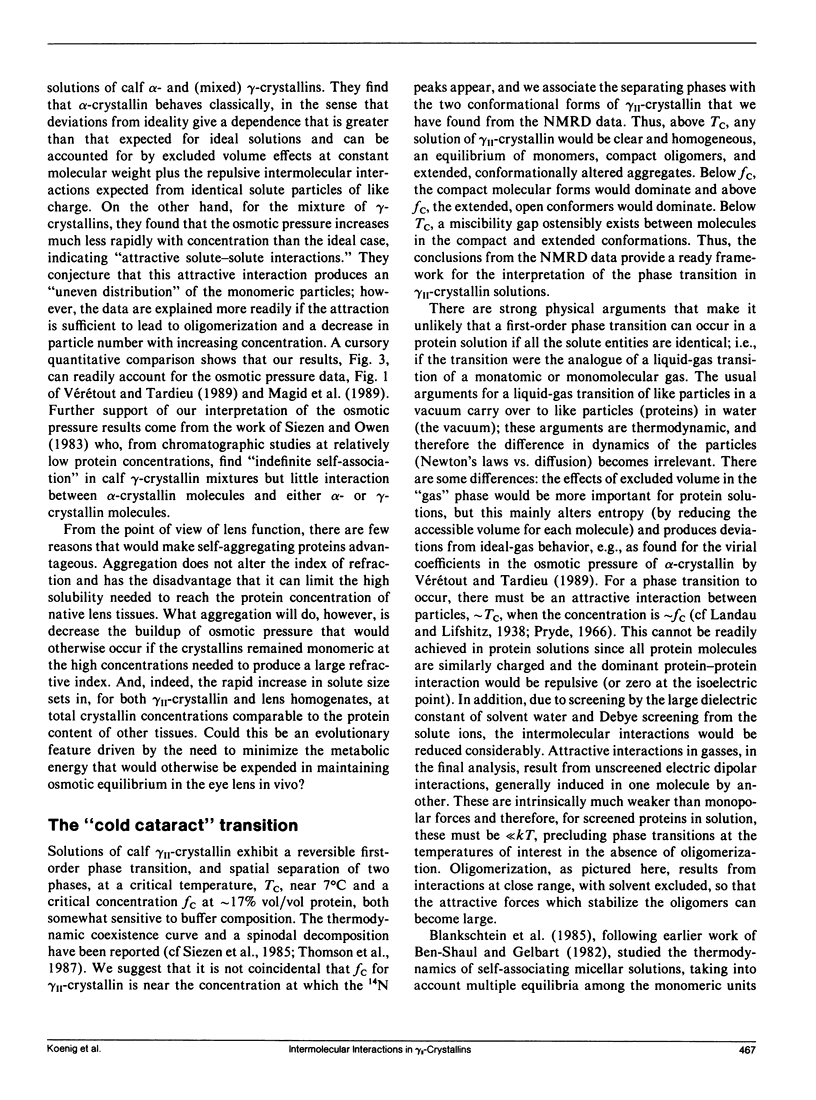
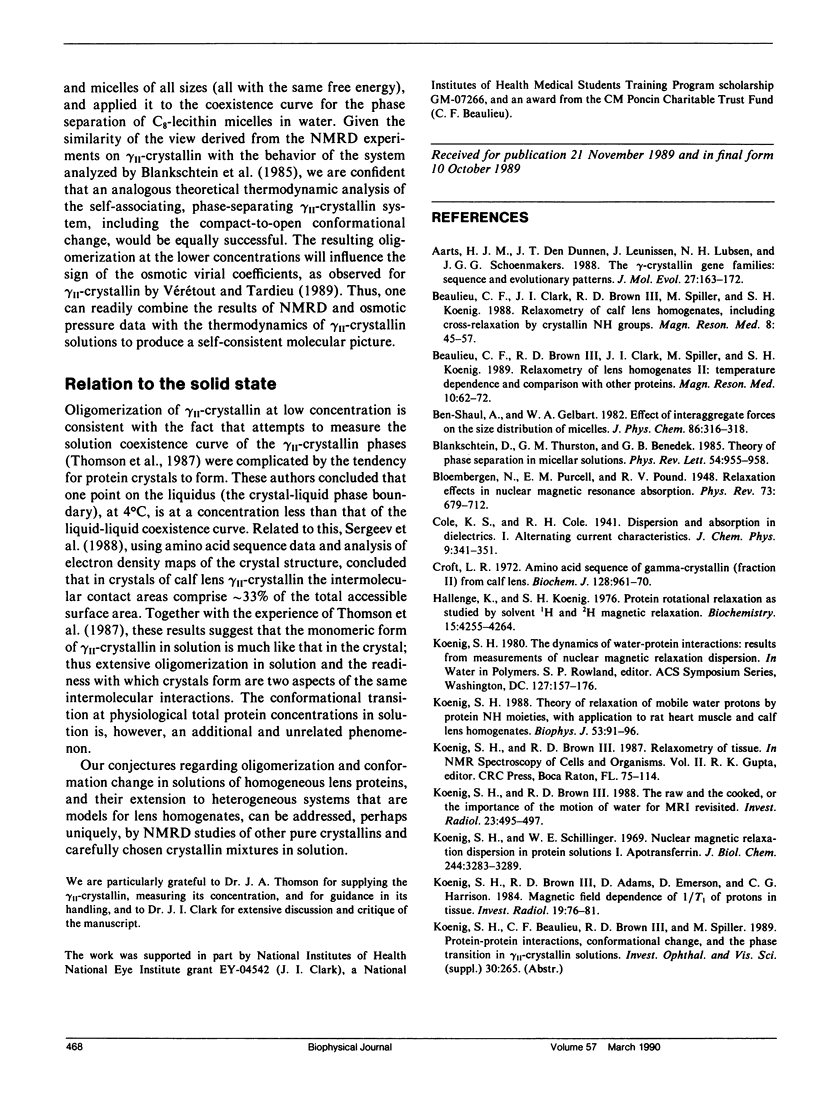
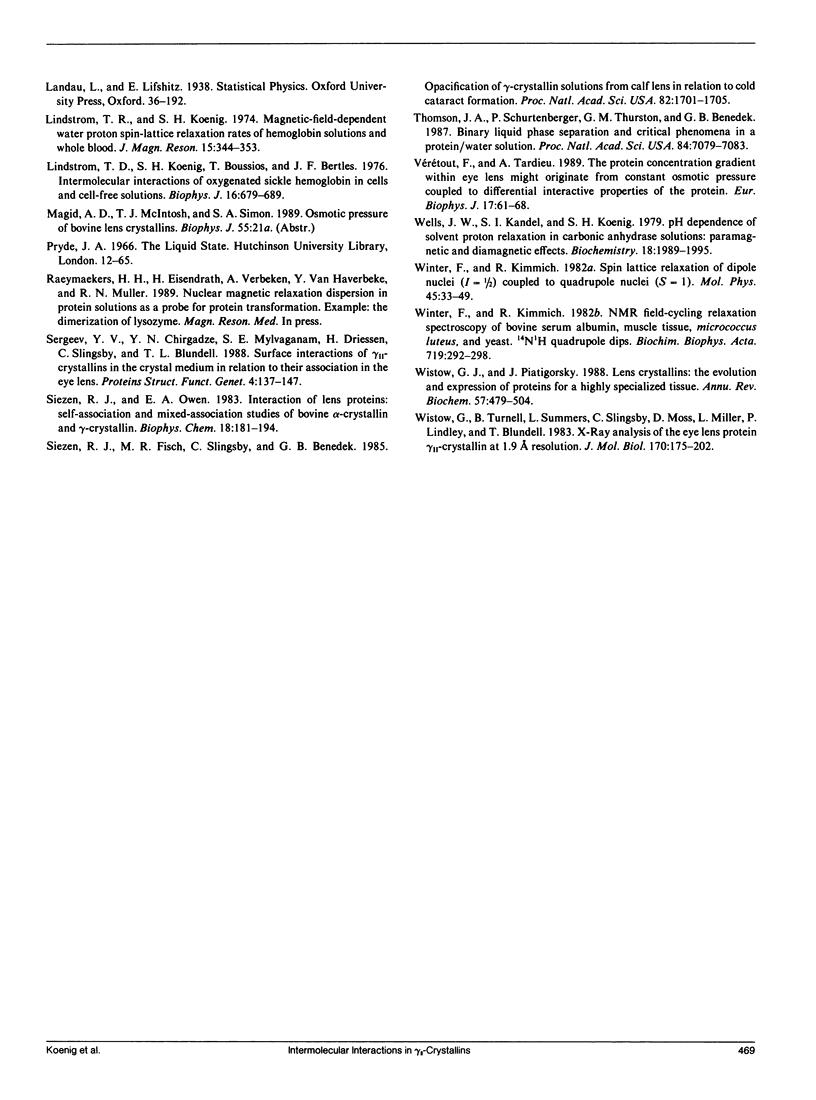
Selected References
These references are in PubMed. This may not be the complete list of references from this article.
- Aarts H. J., den Dunnen J. T., Leunissen J., Lubsen N. H., Schoenmakers J. G. The gamma-crystallin gene families: sequence and evolutionary patterns. J Mol Evol. 1988;27(2):163–172. doi: 10.1007/BF02138377. [DOI] [PubMed] [Google Scholar]
- Beaulieu C. F., Clark J. I., Brown R. D., 3rd, Spiller M., Koenig S. H. Relaxometry of calf lens homogenates, including cross-relaxation by crystallin NH groups. Magn Reson Med. 1988 Sep;8(1):45–57. doi: 10.1002/mrm.1910080106. [DOI] [PubMed] [Google Scholar]
- Blankschtein D, Thurston GM, Benedek GB. Theory of phase separation in micellar solutions. Phys Rev Lett. 1985 Mar 4;54(9):955–955. doi: 10.1103/PhysRevLett.54.955. [DOI] [PubMed] [Google Scholar]
- Croft L. R. The amino acid sequence of -crystallin (fraction II) from calf lens. Biochem J. 1972 Jul;128(4):961–970. doi: 10.1042/bj1280961. [DOI] [PMC free article] [PubMed] [Google Scholar]
- Hallenga K., Koenig S. H. Protein rotational relaxation as studied by solvent 1H and 2H magnetic relaxation. Biochemistry. 1976 Sep 21;15(19):4255–4264. doi: 10.1021/bi00664a019. [DOI] [PubMed] [Google Scholar]
- Koenig S. H., Brown R. D., 3rd, Adams D., Emerson D., Harrison C. G. Magnetic field dependence of 1/T1 of protons in tissue. Invest Radiol. 1984 Mar-Apr;19(2):76–81. doi: 10.1097/00004424-198403000-00002. [DOI] [PubMed] [Google Scholar]
- Koenig S. H., Brown R. D., 3rd The raw and the cooked, or the importance of the motion of water for MRI revisited. Invest Radiol. 1988 Jul;23(7):495–497. doi: 10.1097/00004424-198807000-00002. [DOI] [PubMed] [Google Scholar]
- Koenig S. H., Schillinger W. E. Nuclear magnetic relaxation dispersion in protein solutions. I. Apotransferrin. J Biol Chem. 1969 Jun 25;244(12):3283–3289. [PubMed] [Google Scholar]
- Koenig S. H. Theory of relaxation of mobile water protons induced by protein NH moieties, with application to rat heart muscle and calf lens homogenates. Biophys J. 1988 Jan;53(1):91–96. doi: 10.1016/S0006-3495(88)83069-8. [DOI] [PMC free article] [PubMed] [Google Scholar]
- Lindstrom T. R., Koenig S. H., Boussios T., Bertles J. F. Intermolecular interactions of oxygenated sickle hemoglobin molecules in cells and cell-free solutions. Biophys J. 1976 Jun;16(6):679–689. doi: 10.1016/S0006-3495(76)85721-9. [DOI] [PMC free article] [PubMed] [Google Scholar]
- Sergeev Y. V., Chirgadze Y. N., Mylvaganam S. E., Driessen H., Slingsby C., Blundell T. L. Surface interactions of gamma-crystallins in the crystal medium in relation to their association in the eye lens. Proteins. 1988;4(2):137–147. doi: 10.1002/prot.340040207. [DOI] [PubMed] [Google Scholar]
- Siezen R. J., Fisch M. R., Slingsby C., Benedek G. B. Opacification of gamma-crystallin solutions from calf lens in relation to cold cataract formation. Proc Natl Acad Sci U S A. 1985 Mar;82(6):1701–1705. doi: 10.1073/pnas.82.6.1701. [DOI] [PMC free article] [PubMed] [Google Scholar]
- Siezen R. J., Owen E. A. Interactions of lens proteins. Self-association and mixed-association studies of bovine alpha-crystallin and gamma-crystallin. Biophys Chem. 1983 Oct;18(3):181–194. doi: 10.1016/0301-4622(83)80030-1. [DOI] [PubMed] [Google Scholar]
- Thomson J. A., Schurtenberger P., Thurston G. M., Benedek G. B. Binary liquid phase separation and critical phenomena in a protein/water solution. Proc Natl Acad Sci U S A. 1987 Oct;84(20):7079–7083. doi: 10.1073/pnas.84.20.7079. [DOI] [PMC free article] [PubMed] [Google Scholar]
- Vérétout F., Tardieu A. The protein concentration gradient within eye lens might originate from constant osmotic pressure coupled to differential interactive properties of crystallins. Eur Biophys J. 1989;17(2):61–68. doi: 10.1007/BF00257103. [DOI] [PubMed] [Google Scholar]
- Wells J. W., Kandel S. I., Koenig S. H. pH dependence of solvent proton relaxation in carbonic anhydrase solutions: paramagnetic and diamagnetic effects. Biochemistry. 1979 May 15;18(10):1989–1995. doi: 10.1021/bi00577a022. [DOI] [PubMed] [Google Scholar]
- Winter F., Kimmich R. NMR field-cycling relaxation spectroscopy of bovine serum albumin, muscle tissue, Micrococcus luteus and yeast. 14N1H-quadrupole dips. Biochim Biophys Acta. 1982 Nov 24;719(2):292–298. doi: 10.1016/0304-4165(82)90101-5. [DOI] [PubMed] [Google Scholar]
- Wistow G. J., Piatigorsky J. Lens crystallins: the evolution and expression of proteins for a highly specialized tissue. Annu Rev Biochem. 1988;57:479–504. doi: 10.1146/annurev.bi.57.070188.002403. [DOI] [PubMed] [Google Scholar]
- Wistow G., Turnell B., Summers L., Slingsby C., Moss D., Miller L., Lindley P., Blundell T. X-ray analysis of the eye lens protein gamma-II crystallin at 1.9 A resolution. J Mol Biol. 1983 Oct 15;170(1):175–202. doi: 10.1016/s0022-2836(83)80232-0. [DOI] [PubMed] [Google Scholar]