Abstract
Macromolecular adsorption is known to occur as a complex process, often in a series of steps. Several models are discussed in the literature which describe the microscopic structure of the adsorbate. In the present study we investigated the adsorption of hen egg white lysozyme on alkylated silicon oxide surfaces. A combination of fluorescence excitation in the evanescent field and fluorescence recovery after photobleaching allowed us to measure the amount of adsorbed fluorescent lysozyme and the equilibrium exchange kinetics with molecules in solution. We found that a model with at least three classes of adsorbed molecules is necessary to describe the experimental results. A first layer is formed by the molecules which adsorb within a short time after the beginning of the incubation. These molecules make up approximately 65% of the final coverage. They are quasi-irreversibly adsorbed and do not measurably exchange with bulk molecules within one day even at temperatures up to 55 degrees C. A second layer, which reaches equilibrium only after several hours of incubation, shows a pronounced exchange with bulk molecules. The on-off kinetics show a distinct temperature dependence from which an activation barrier of delta E approximately 22 kcal/mol is derived. A third layer of molecules that exchange rapidly with the bulk can be seen to comprise approximately 10% of the total coverage. The exchange rate is on the order of fractions of a second. The binding of the latter two classes of adsorbed molecules is exothermic. From the temperature dependence of the coverage, the binding enthalpy of the slowly exchanging layer was estimated to be delta Hads approximately 3.8 kcal/mol. The second and third class of molecules remain enzymatically active as a muramidase, which was tested by the lysis of the cell walls of Micrococcus lysodeiktikus. The molecules in the first layer, on the other hand, showed no enzymatic activity.
Full text
PDF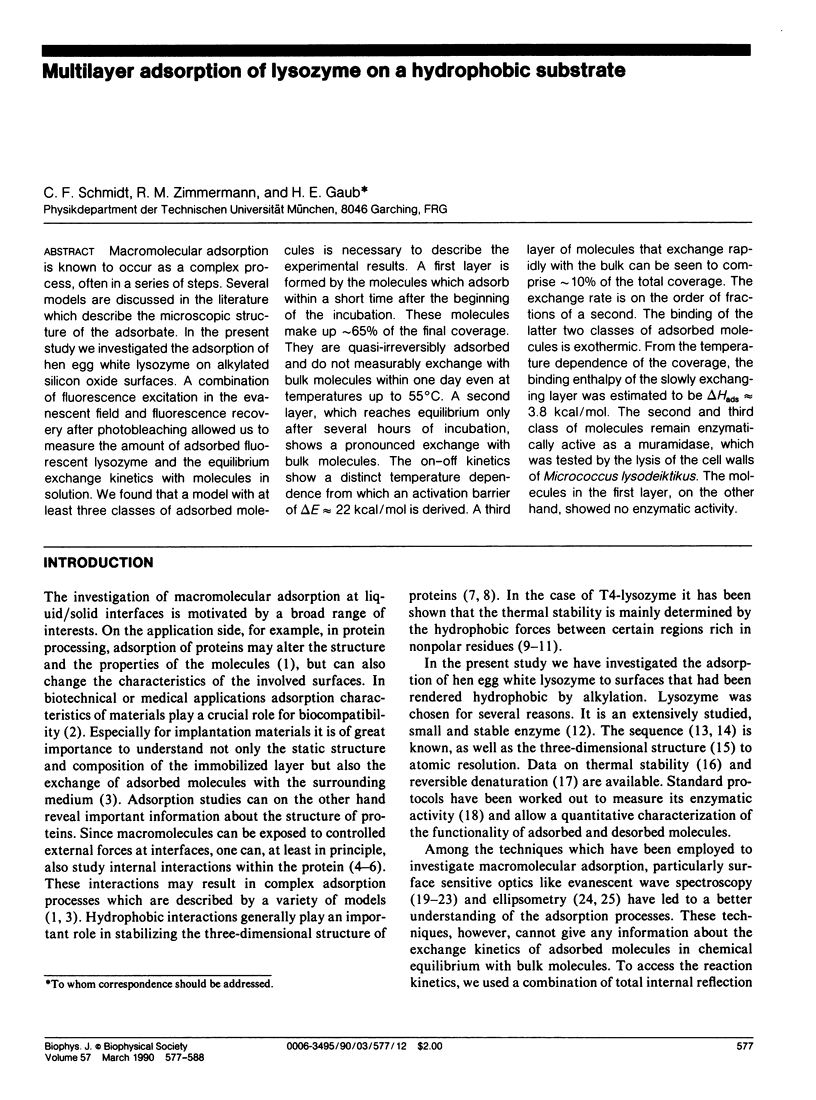
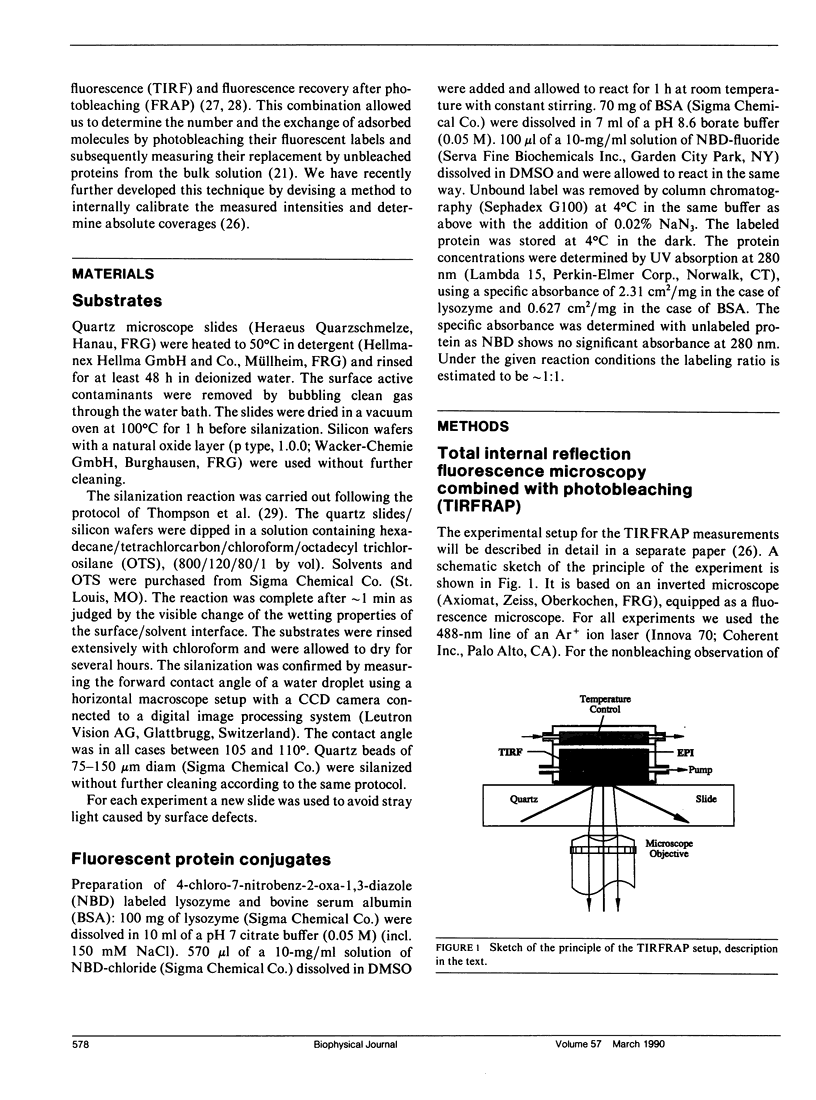
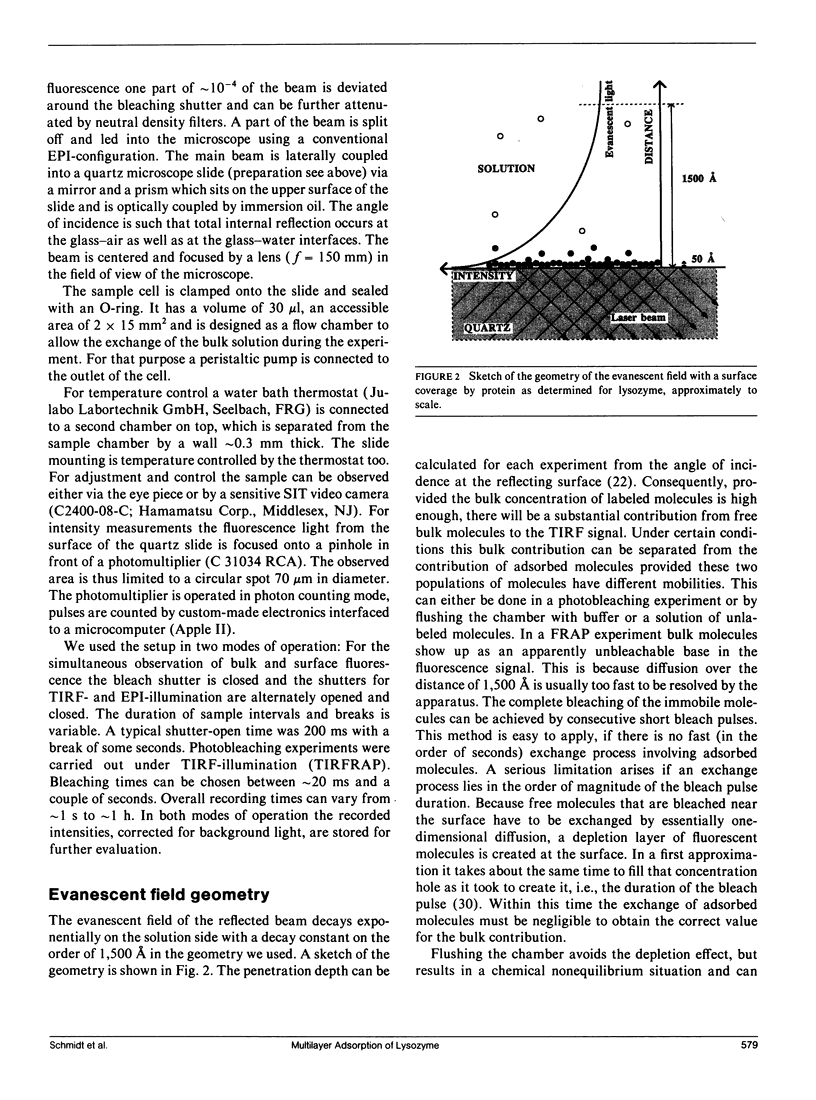
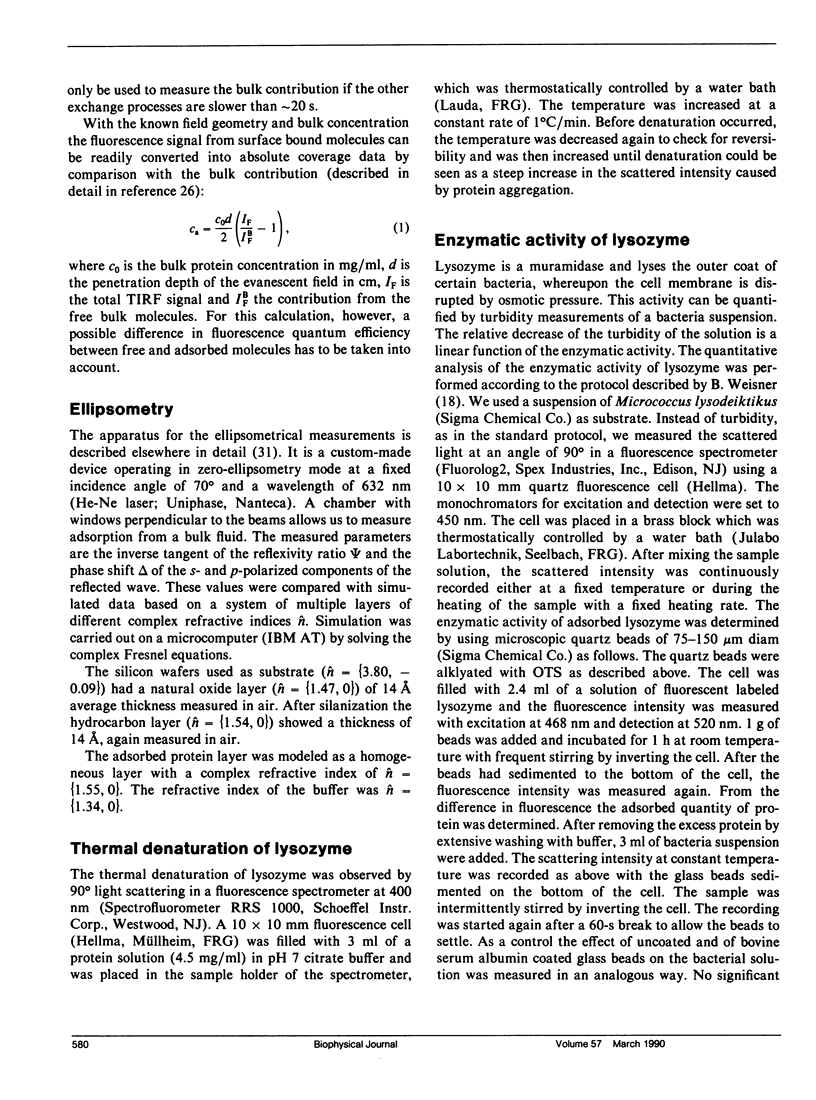
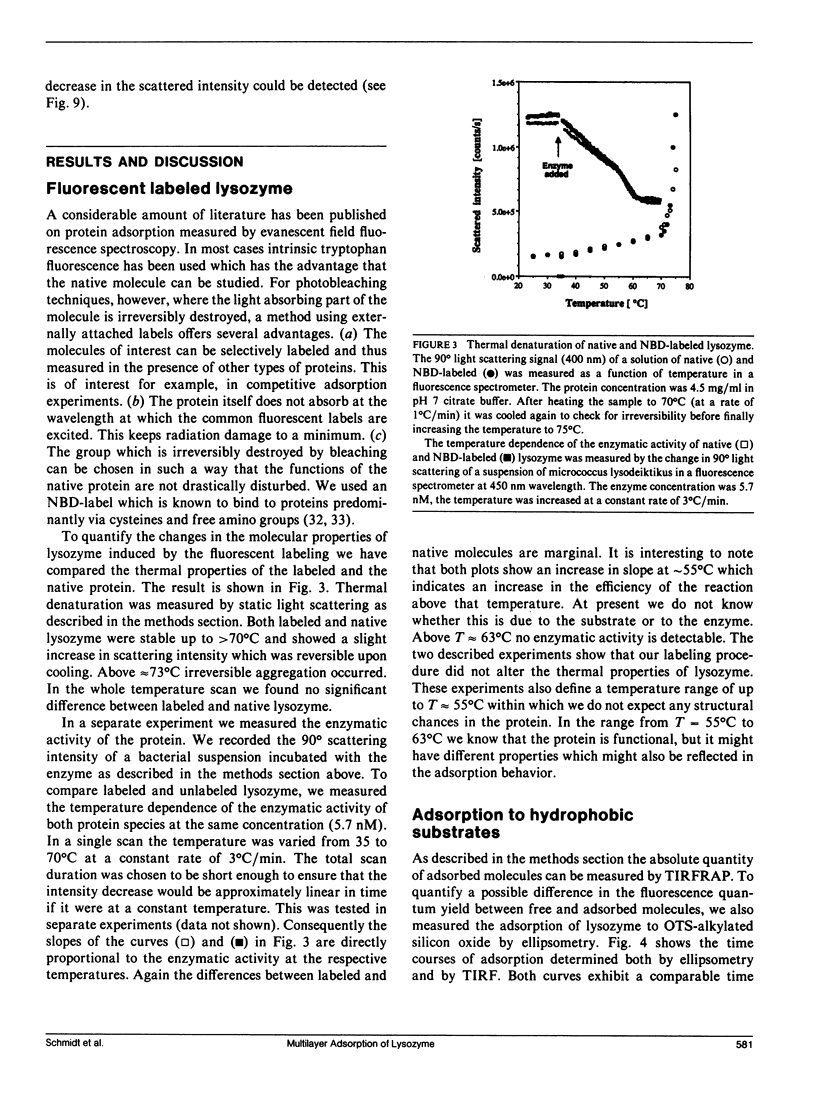
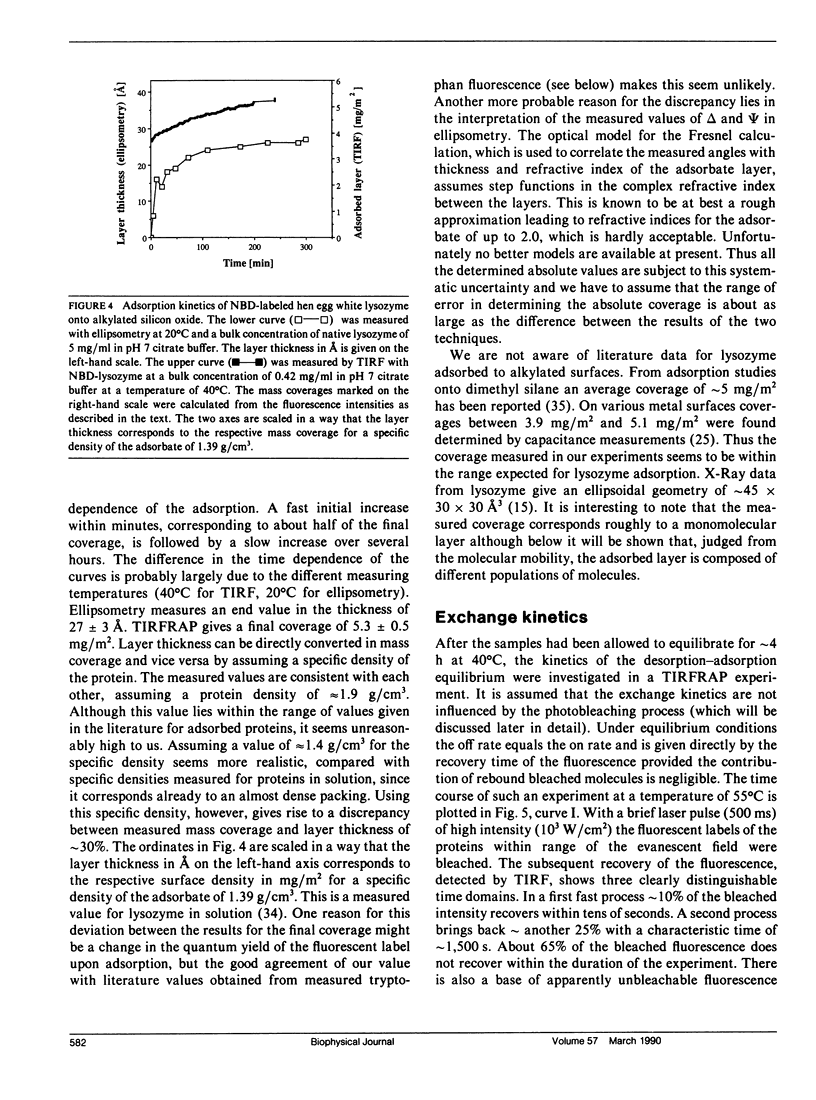
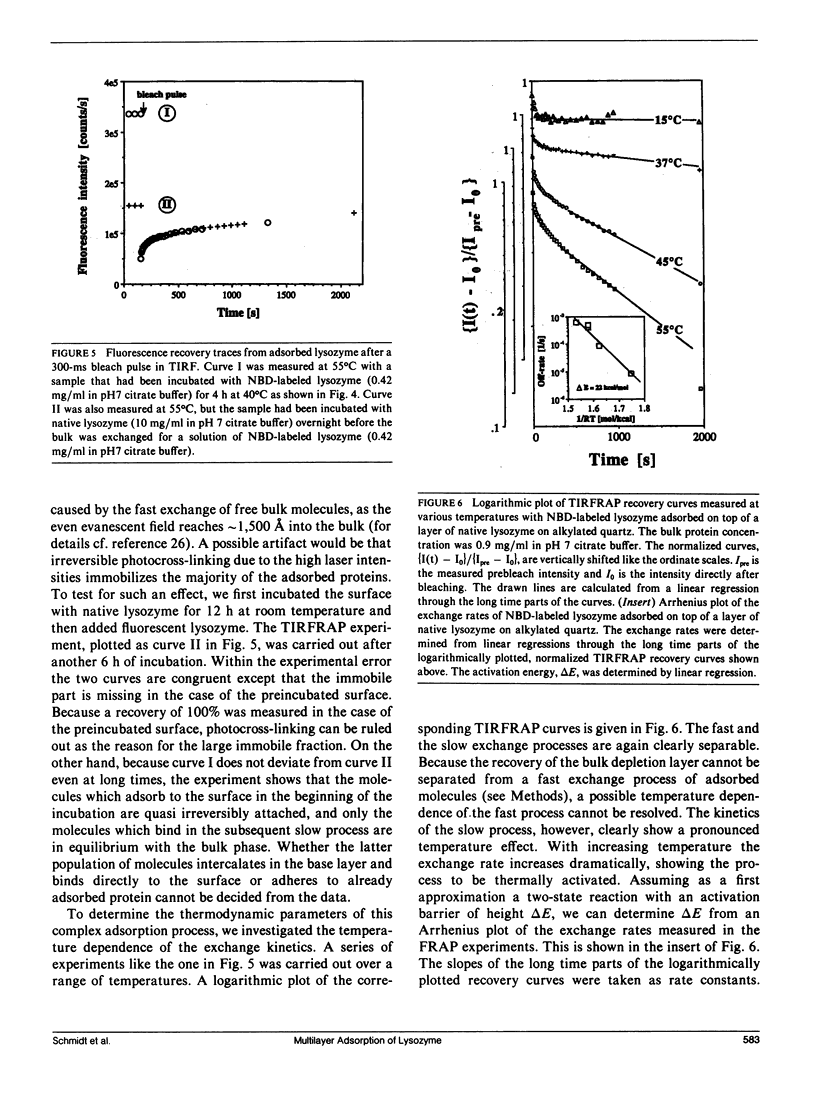
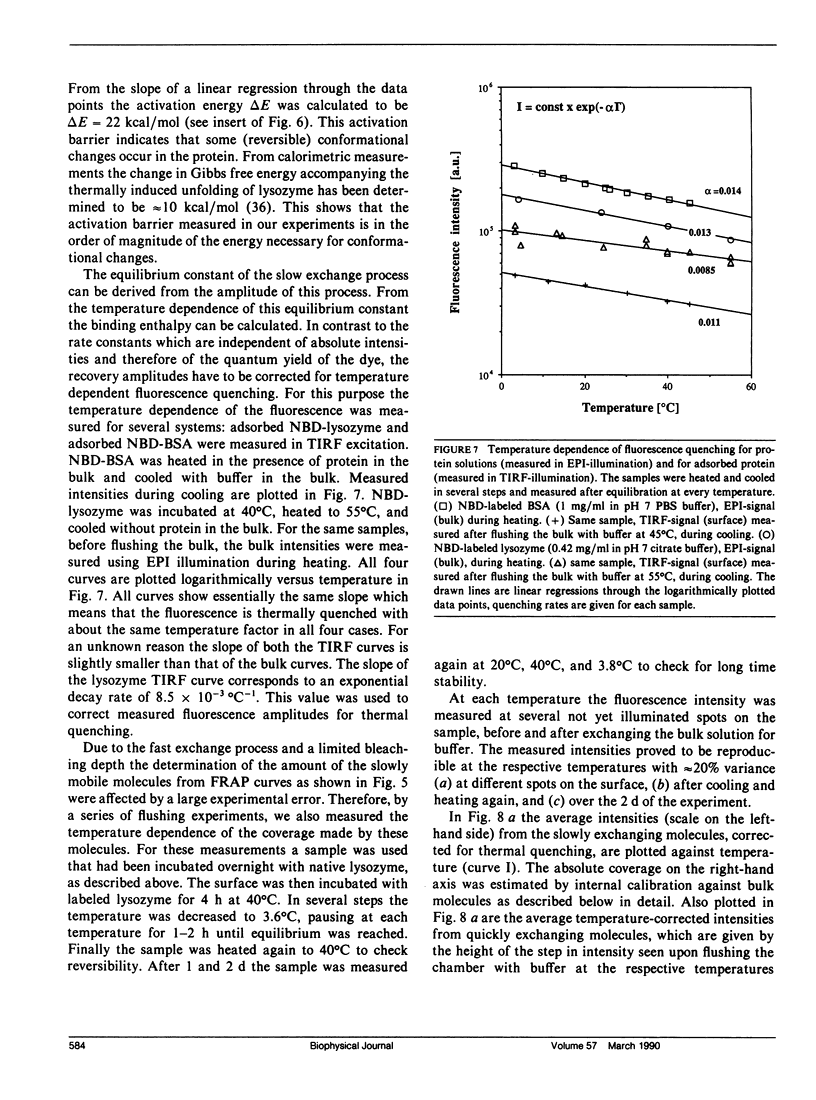
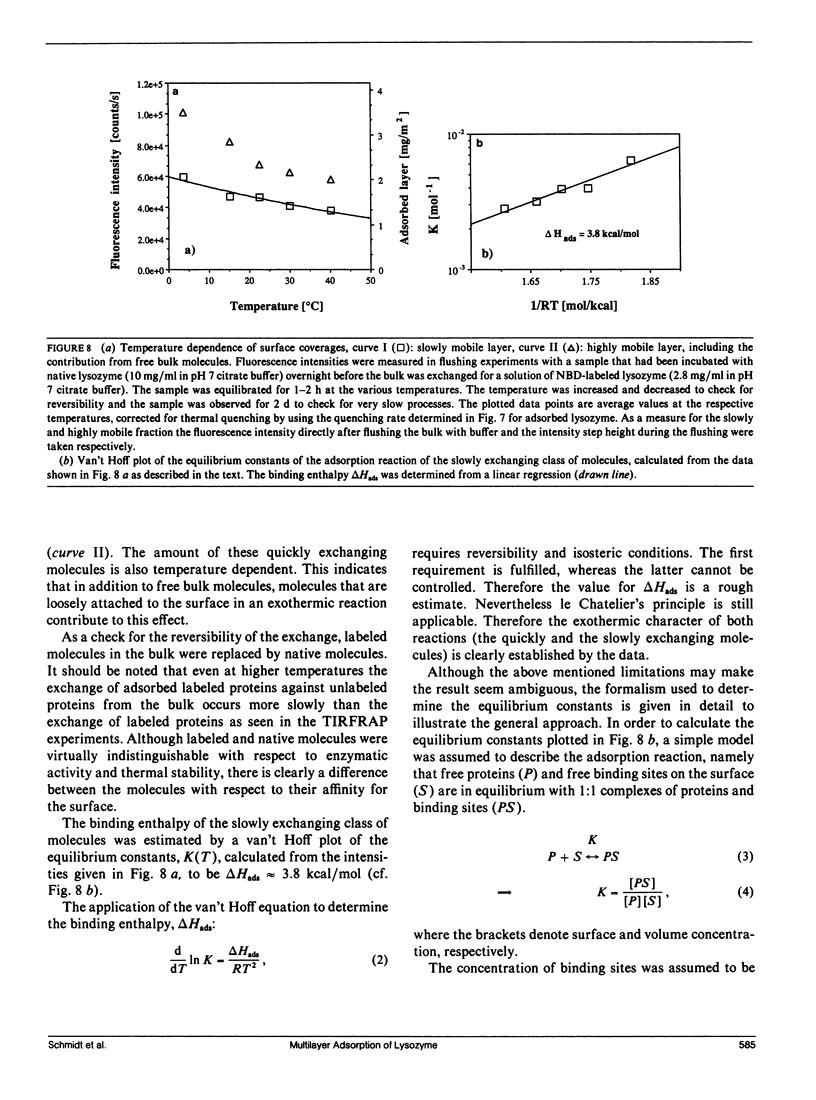
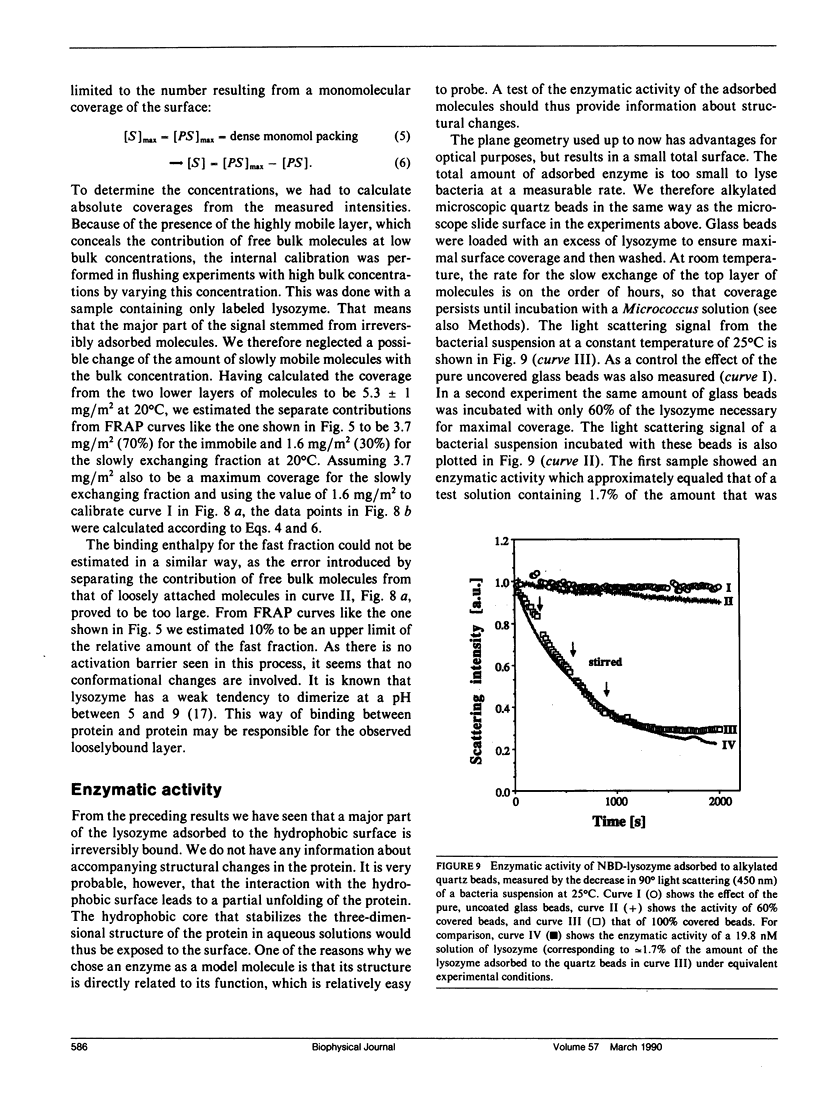
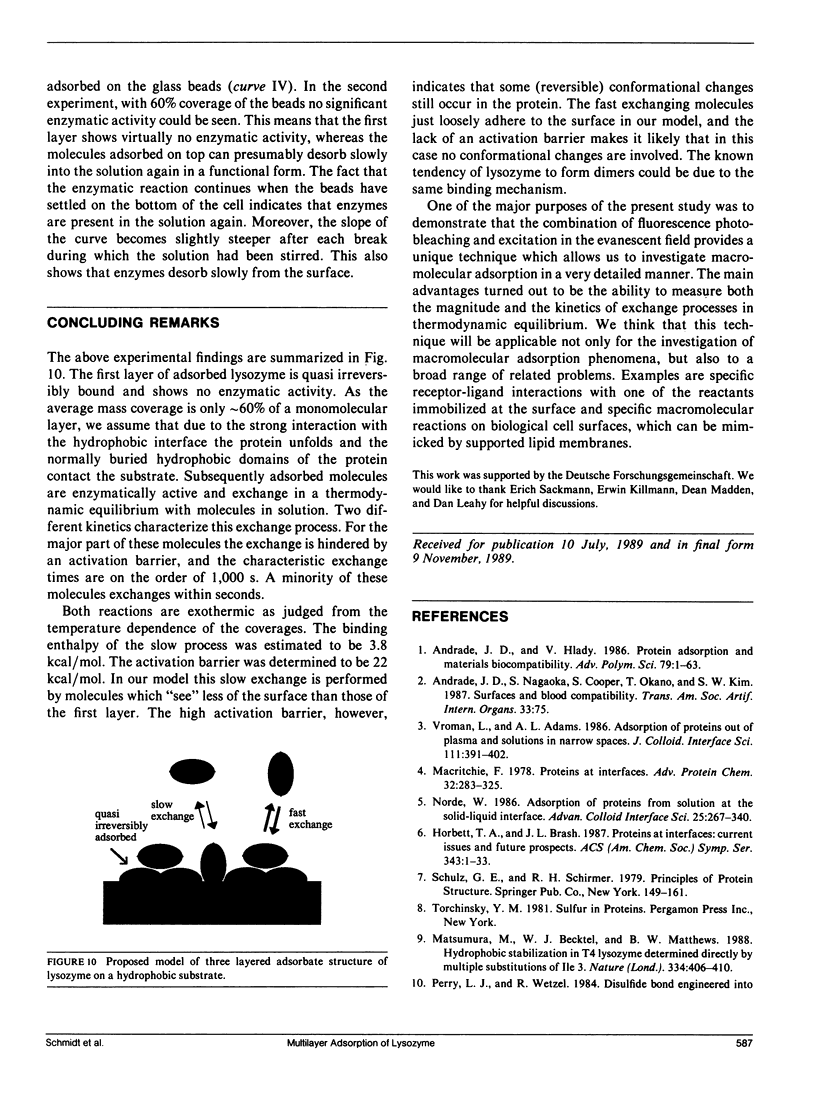
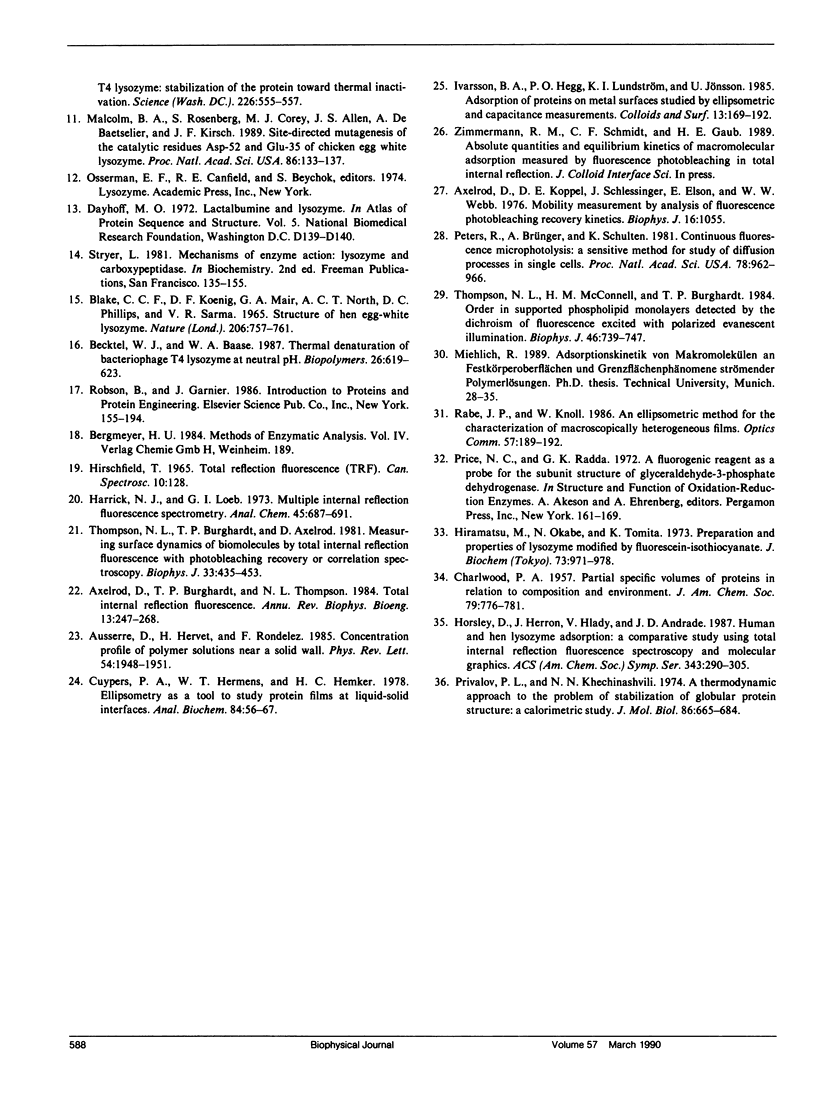
Images in this article
Selected References
These references are in PubMed. This may not be the complete list of references from this article.
- Ausserré D, Hervet H, Rondelez F. Concentration profile of polymer solutions near a solid wall. Phys Rev Lett. 1985 Apr 29;54(17):1948–1951. doi: 10.1103/PhysRevLett.54.1948. [DOI] [PubMed] [Google Scholar]
- Axelrod D., Burghardt T. P., Thompson N. L. Total internal reflection fluorescence. Annu Rev Biophys Bioeng. 1984;13:247–268. doi: 10.1146/annurev.bb.13.060184.001335. [DOI] [PubMed] [Google Scholar]
- Axelrod D., Koppel D. E., Schlessinger J., Elson E., Webb W. W. Mobility measurement by analysis of fluorescence photobleaching recovery kinetics. Biophys J. 1976 Sep;16(9):1055–1069. doi: 10.1016/S0006-3495(76)85755-4. [DOI] [PMC free article] [PubMed] [Google Scholar]
- Becktel W. J., Baase W. A. Thermal denaturation of bacteriophage T4 lysozyme at neutral pH. Biopolymers. 1987 May;26(5):619–623. doi: 10.1002/bip.360260505. [DOI] [PubMed] [Google Scholar]
- Blake C. C., Koenig D. F., Mair G. A., North A. C., Phillips D. C., Sarma V. R. Structure of hen egg-white lysozyme. A three-dimensional Fourier synthesis at 2 Angstrom resolution. Nature. 1965 May 22;206(4986):757–761. doi: 10.1038/206757a0. [DOI] [PubMed] [Google Scholar]
- Cuypers P. A., Hermens W. T., Hemker H. C. Ellipsometry as a tool to study protein films at liquid-solid interfaces. Anal Biochem. 1978 Jan;84(1):56–67. doi: 10.1016/0003-2697(78)90483-9. [DOI] [PubMed] [Google Scholar]
- Hiramatsu M., Okabe N., Tomita K. Preparation and properties of lysozyme modified by fluorescein-isothiocyanate. J Biochem. 1973 May;73(5):971–978. doi: 10.1093/oxfordjournals.jbchem.a130181. [DOI] [PubMed] [Google Scholar]
- Macritchie F. Proteins at interfaces. Adv Protein Chem. 1978;32:283–326. doi: 10.1016/s0065-3233(08)60577-x. [DOI] [PubMed] [Google Scholar]
- Malcolm B. A., Rosenberg S., Corey M. J., Allen J. S., de Baetselier A., Kirsch J. F. Site-directed mutagenesis of the catalytic residues Asp-52 and Glu-35 of chicken egg white lysozyme. Proc Natl Acad Sci U S A. 1989 Jan;86(1):133–137. doi: 10.1073/pnas.86.1.133. [DOI] [PMC free article] [PubMed] [Google Scholar]
- Matsumura M., Becktel W. J., Matthews B. W. Hydrophobic stabilization in T4 lysozyme determined directly by multiple substitutions of Ile 3. Nature. 1988 Aug 4;334(6181):406–410. doi: 10.1038/334406a0. [DOI] [PubMed] [Google Scholar]
- Norde W. Adsorption of proteins from solution at the solid-liquid interface. Adv Colloid Interface Sci. 1986 Sep;25(4):267–340. doi: 10.1016/0001-8686(86)80012-4. [DOI] [PubMed] [Google Scholar]
- Peters R., Brünger A., Schulten K. Continuous fluorescence microphotolysis: A sensitive method for study of diffusion processes in single cells. Proc Natl Acad Sci U S A. 1981 Feb;78(2):962–966. doi: 10.1073/pnas.78.2.962. [DOI] [PMC free article] [PubMed] [Google Scholar]
- Privalov P. L., Khechinashvili N. N. A thermodynamic approach to the problem of stabilization of globular protein structure: a calorimetric study. J Mol Biol. 1974 Jul 5;86(3):665–684. doi: 10.1016/0022-2836(74)90188-0. [DOI] [PubMed] [Google Scholar]
- Thompson N. L., Burghardt T. P., Axelrod D. Measuring surface dynamics of biomolecules by total internal reflection fluorescence with photobleaching recovery or correlation spectroscopy. Biophys J. 1981 Mar;33(3):435–454. doi: 10.1016/S0006-3495(81)84905-3. [DOI] [PMC free article] [PubMed] [Google Scholar]
- Thompson N. L., McConnell H. M., Burhardt T. P. Order in supported phospholipid monolayers detected by the dichroism of fluorescence excited with polarized evanescent illumination. Biophys J. 1984 Dec;46(6):739–747. doi: 10.1016/S0006-3495(84)84072-2. [DOI] [PMC free article] [PubMed] [Google Scholar]