Abstract
Field potentials were recorded along radial tracks in scala tympani and scala vestibuli of the guinea-pig cochlea. A current density analysis revealed standing current density profiles that were qualitatively similar between animals and between the second and third cochlear turns. Radial standing current densities were greatest at or near the spiral ligament. All the scala vestibuli current density profiles were scaled versions of one another while the scala tympani current density profiles showed more variability. Acoustic stimuli modulated the standing current and there was a cochlear microphonic current density peak in scala tympani near the organ of Corti. The results are summarized with a current-density field line model, the key element of which is a constant current pumped into scale media by the stria vascularis. The standing potential gradients drive current from each perilymphatic chamber into the spiral ligament en route to the lateral surface of the stria vascularis. The strial current is divided between the receptor cell pathway and leakage pathways. The standing current through the leakage pathways is indirectly modulated by acoustic stimulation through the modulation of the endocochlear potential. The reciprocal modulation of current between hair cell and leakage pathways suggests that the stria vascularis maintains a constant current during acoustic stimulation. The cochlear standing current is similar to the retinal dark current in its importance for sensory transduction but the fact that the silent current is generated by the stria vascularis and not the receptor cells provides significant benefits for the detection of mechanical stimuli.
Full text
PDF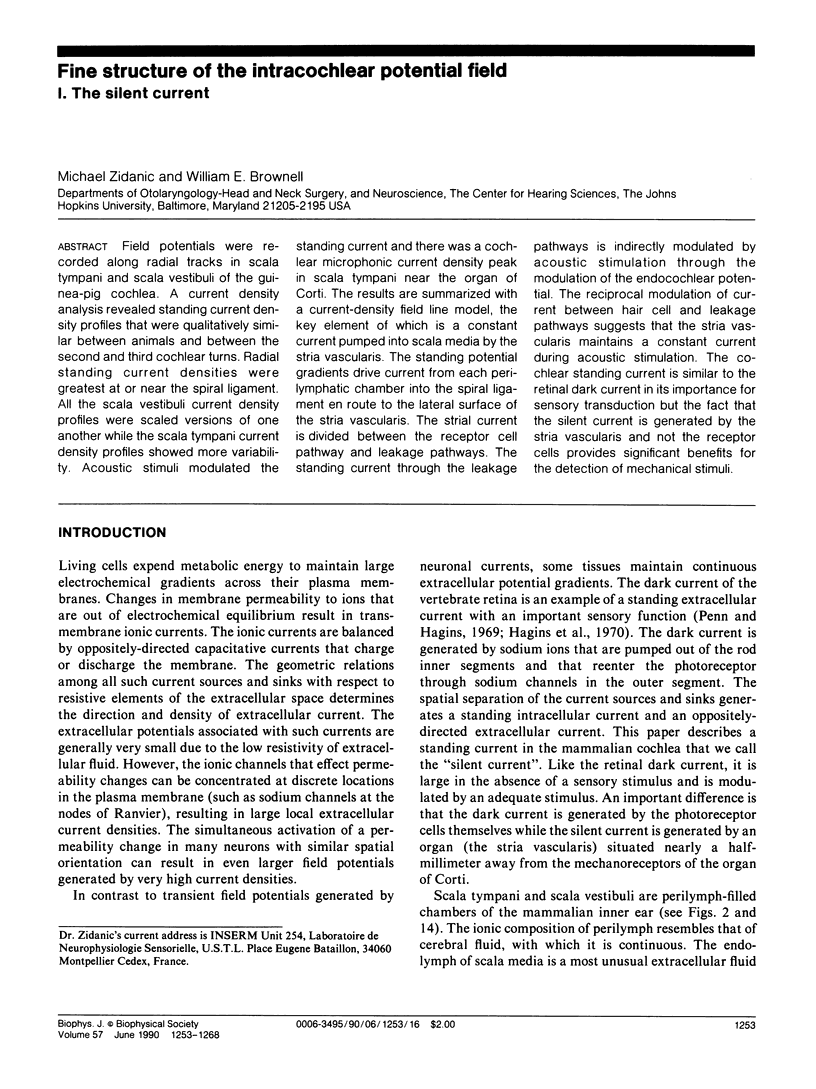
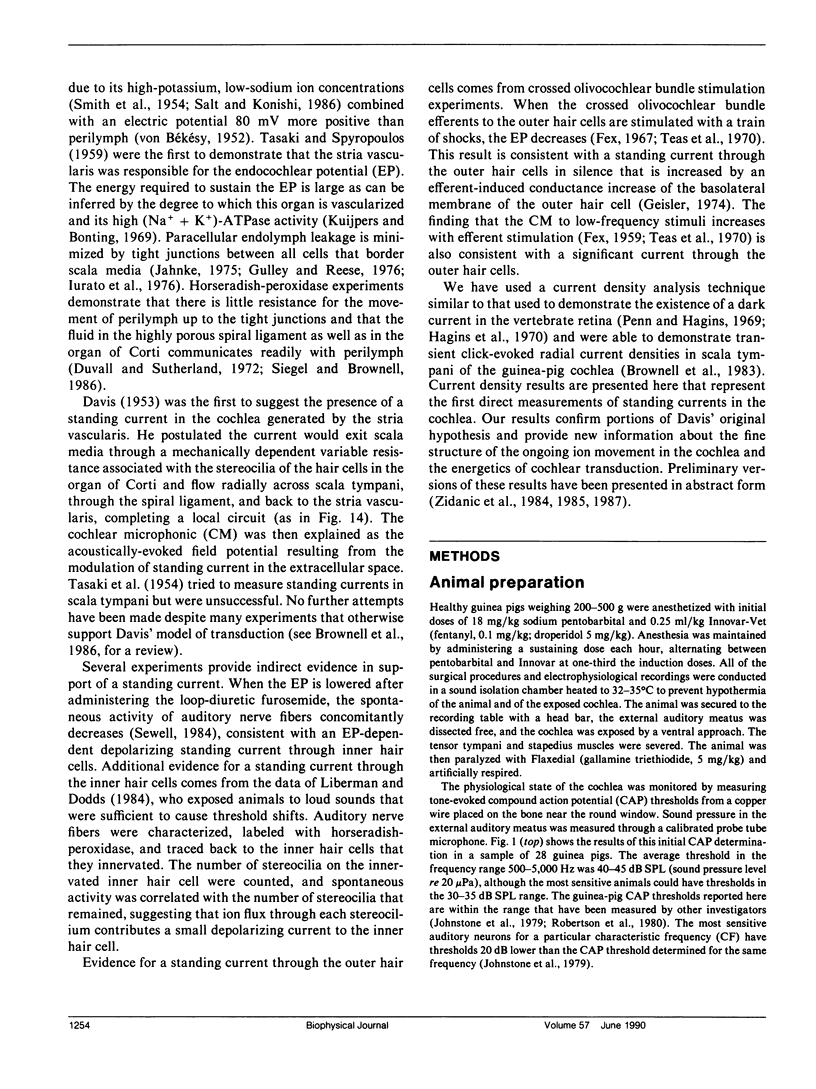
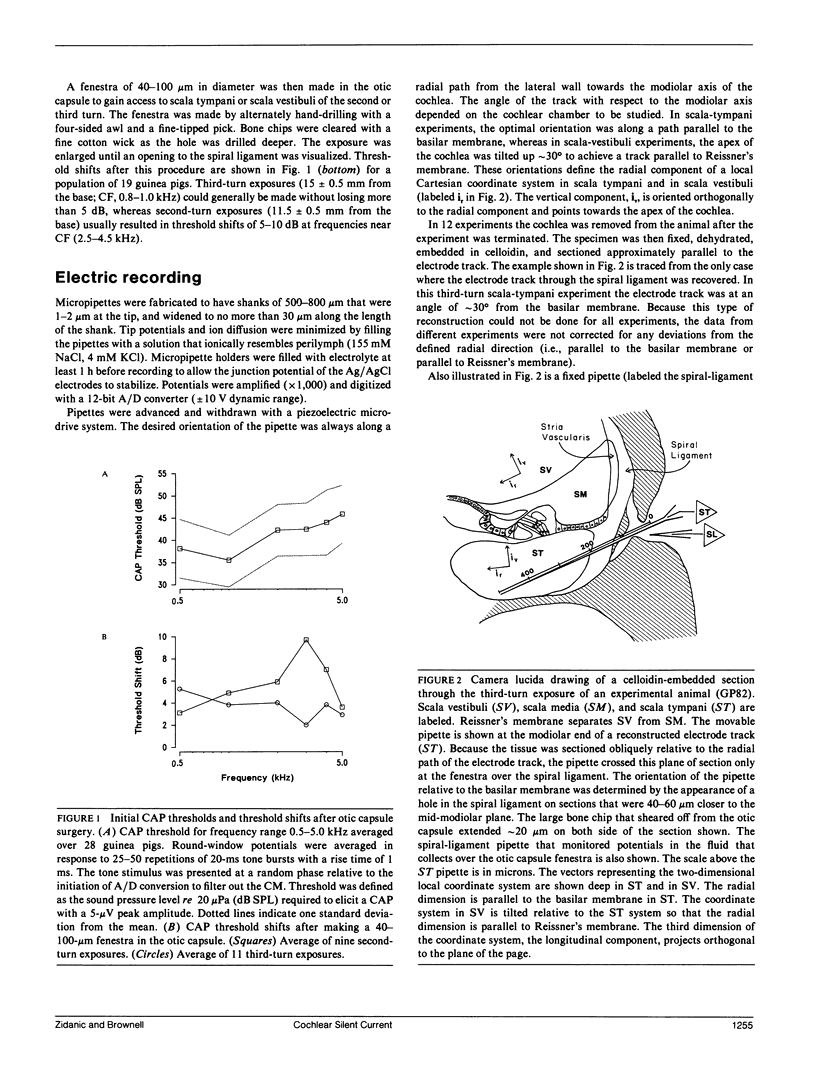
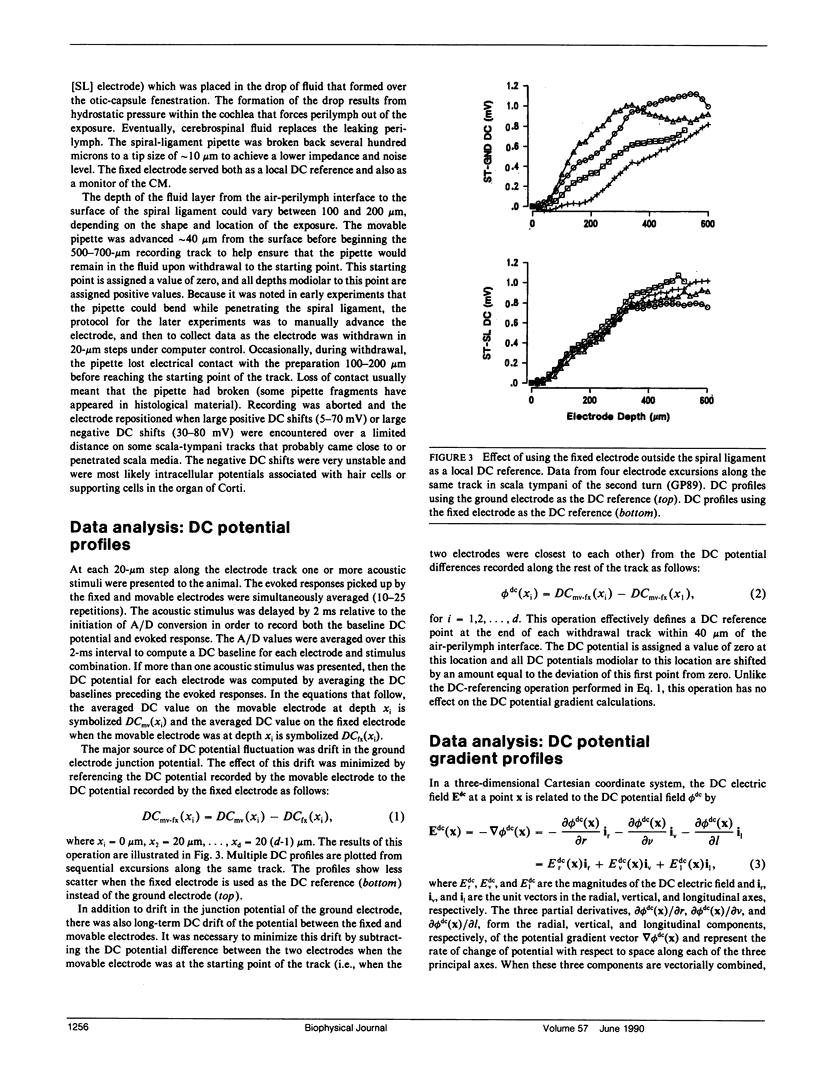
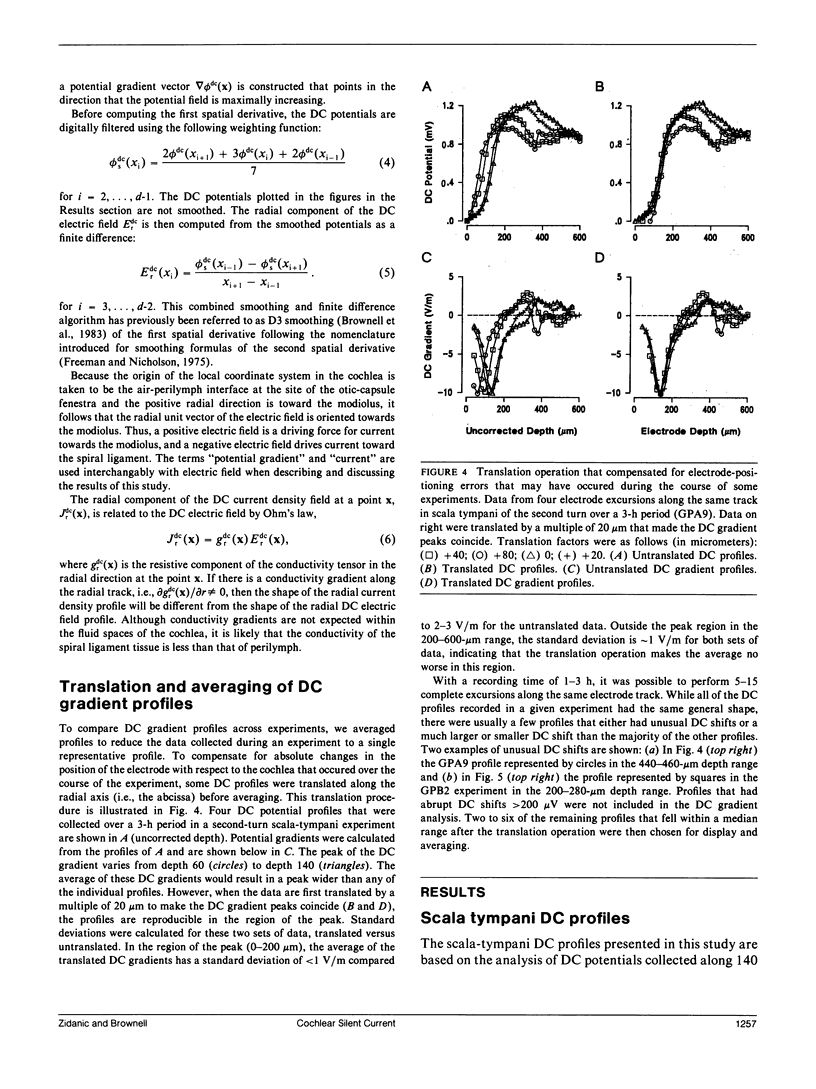
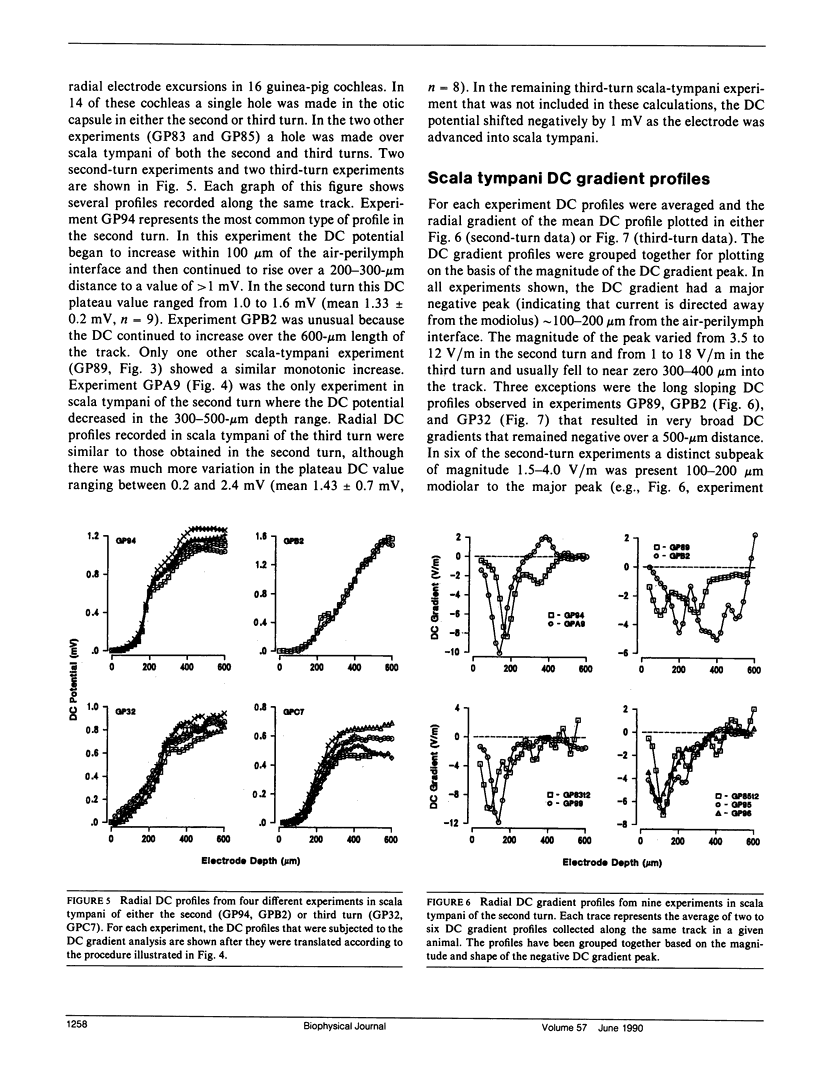
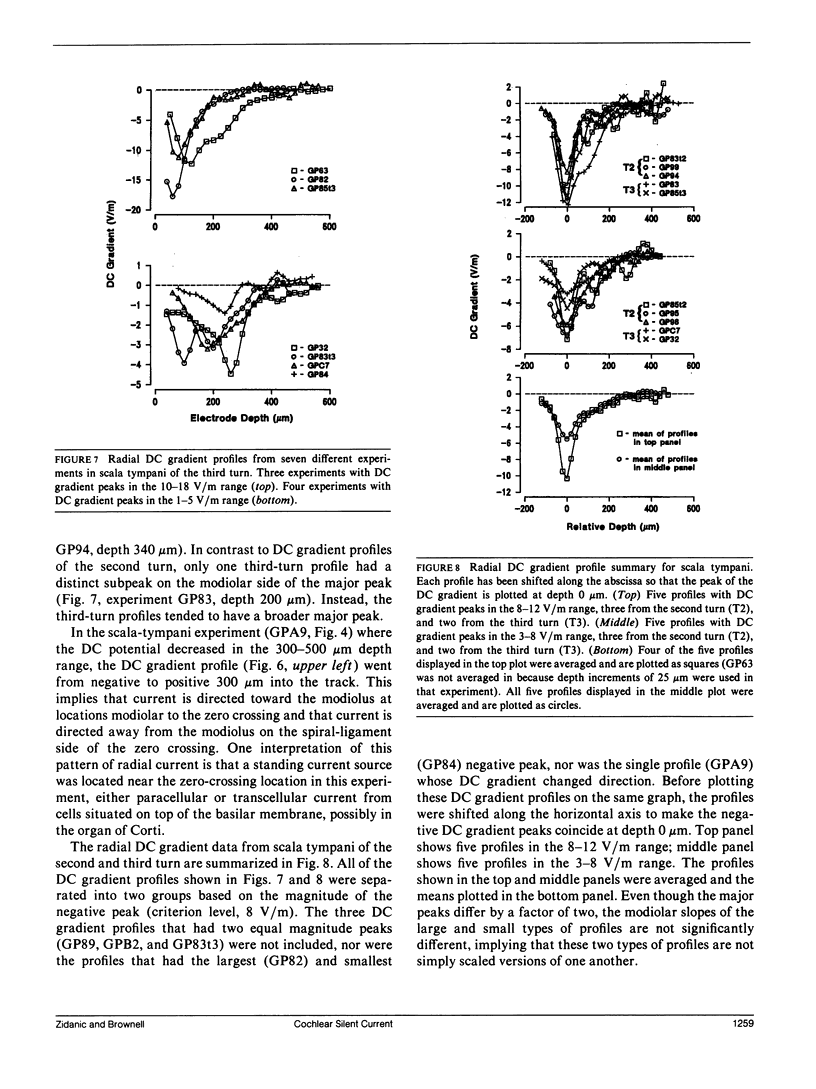
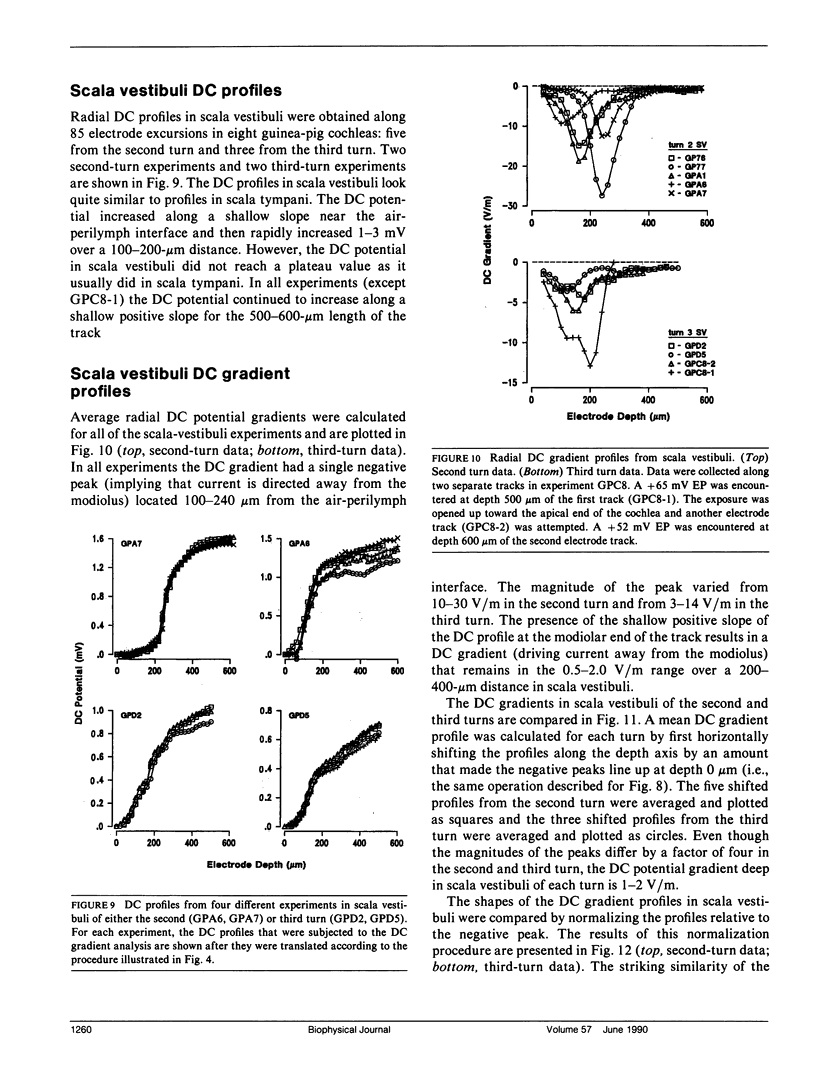
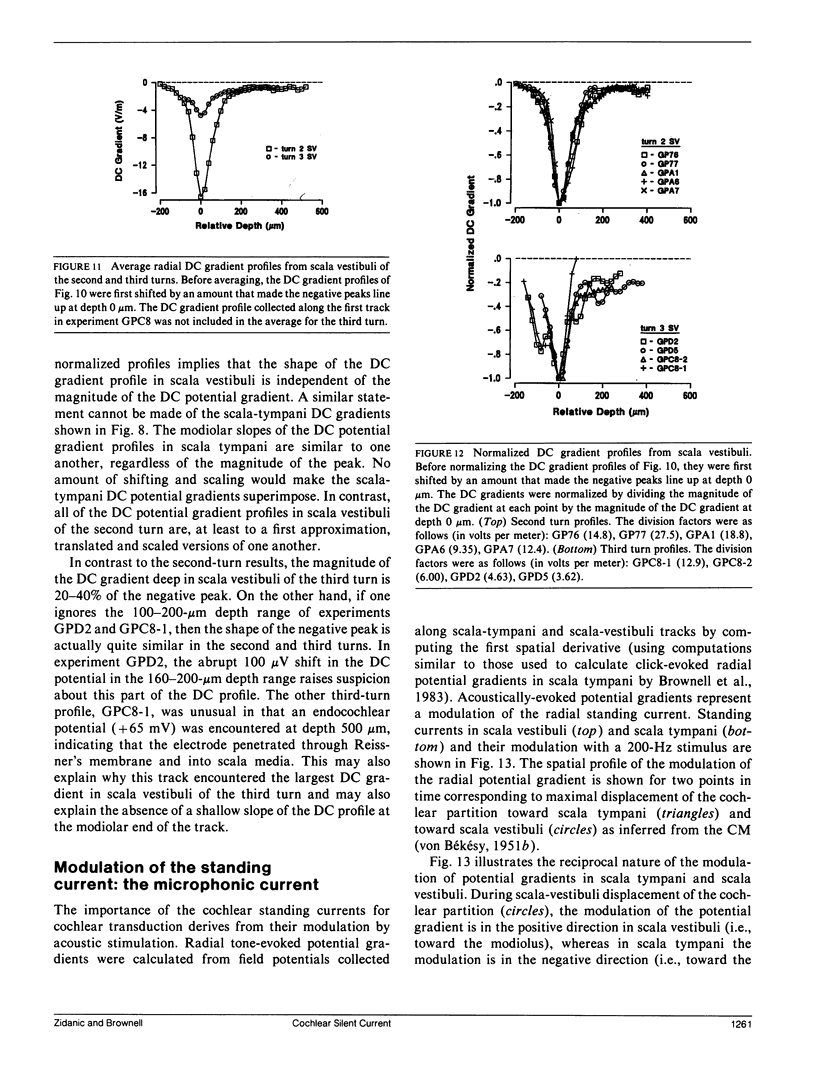
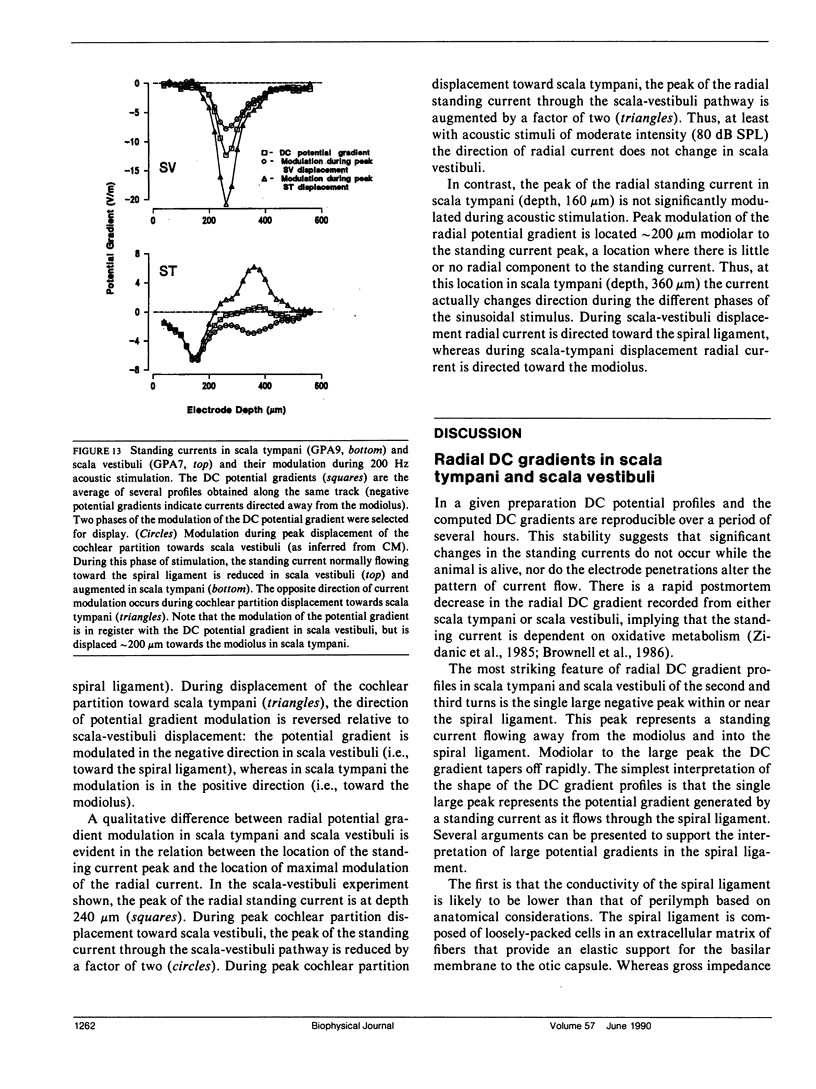
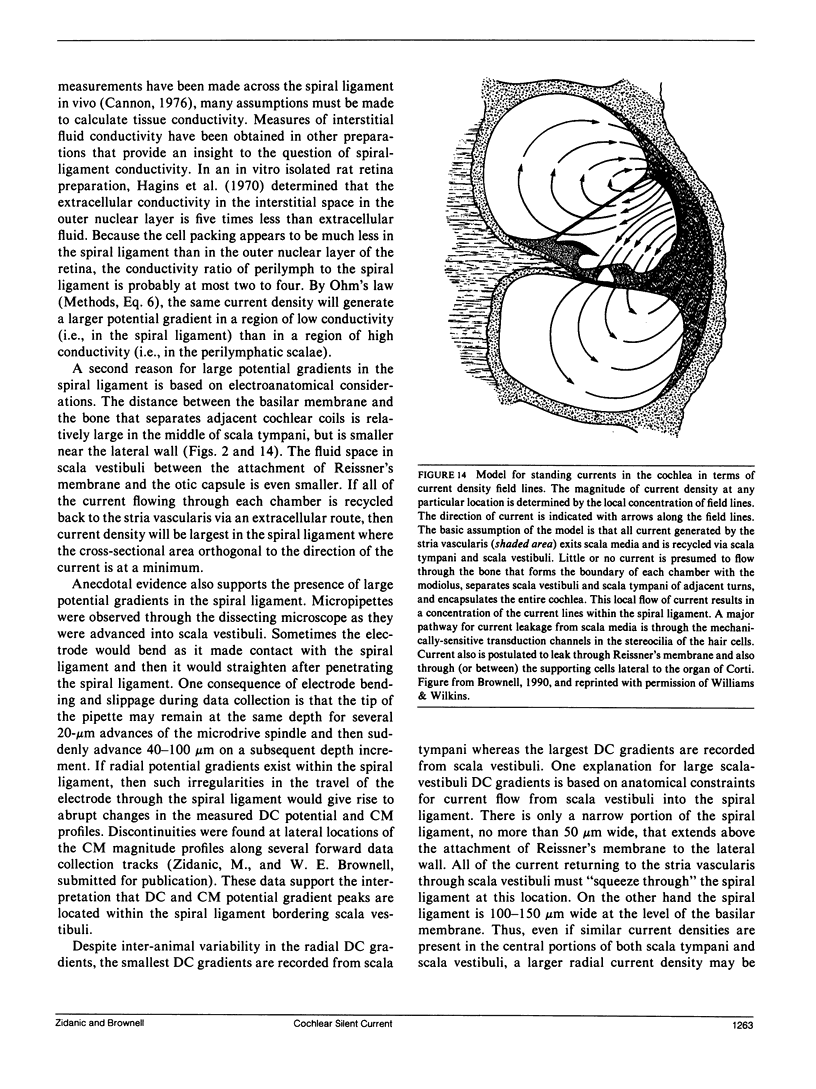
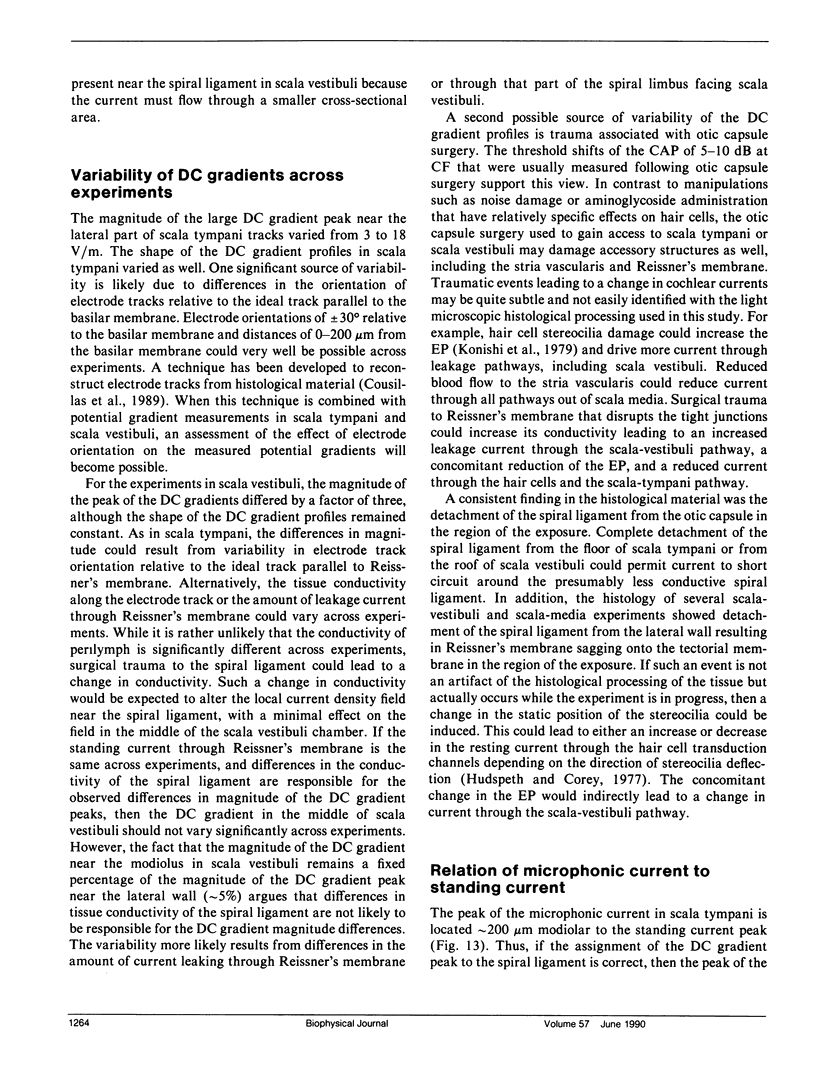
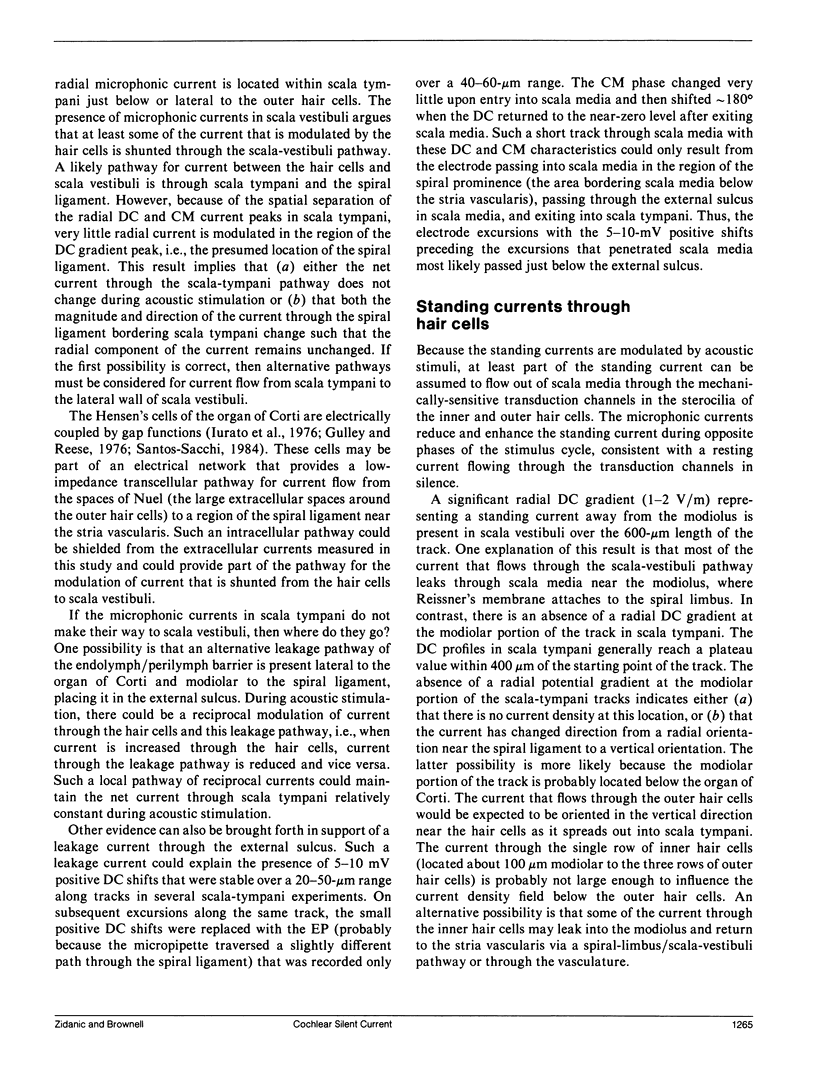
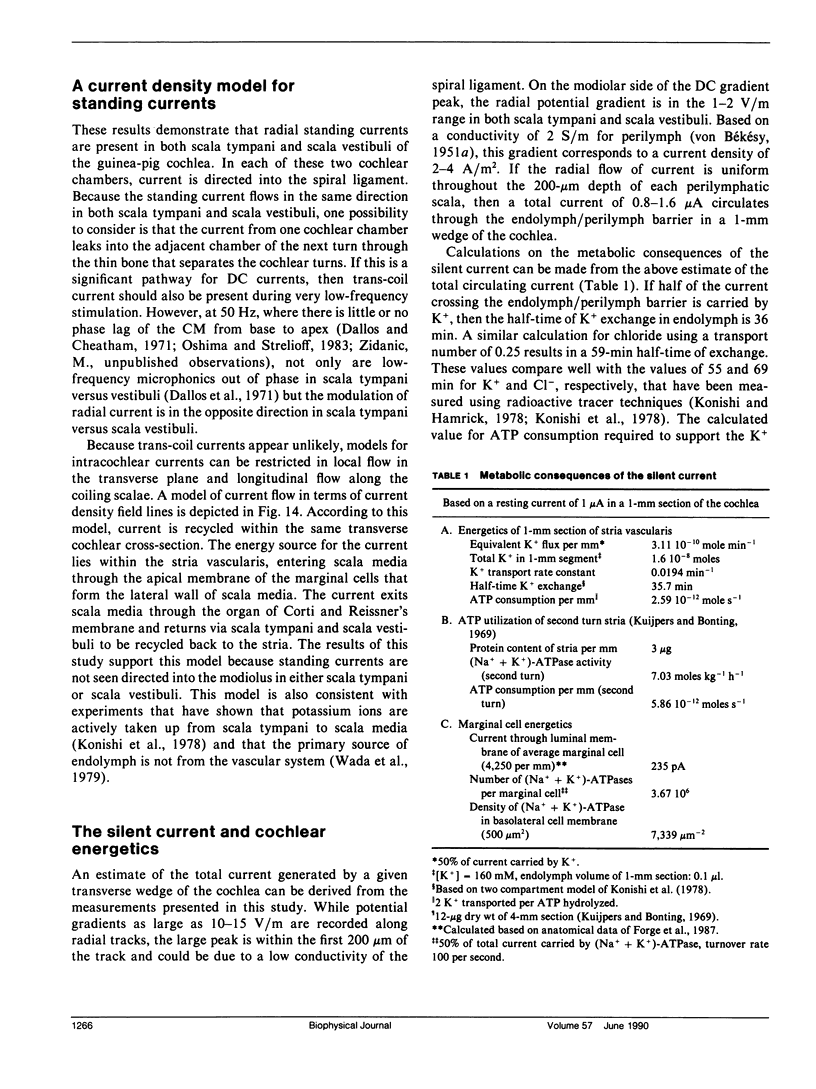
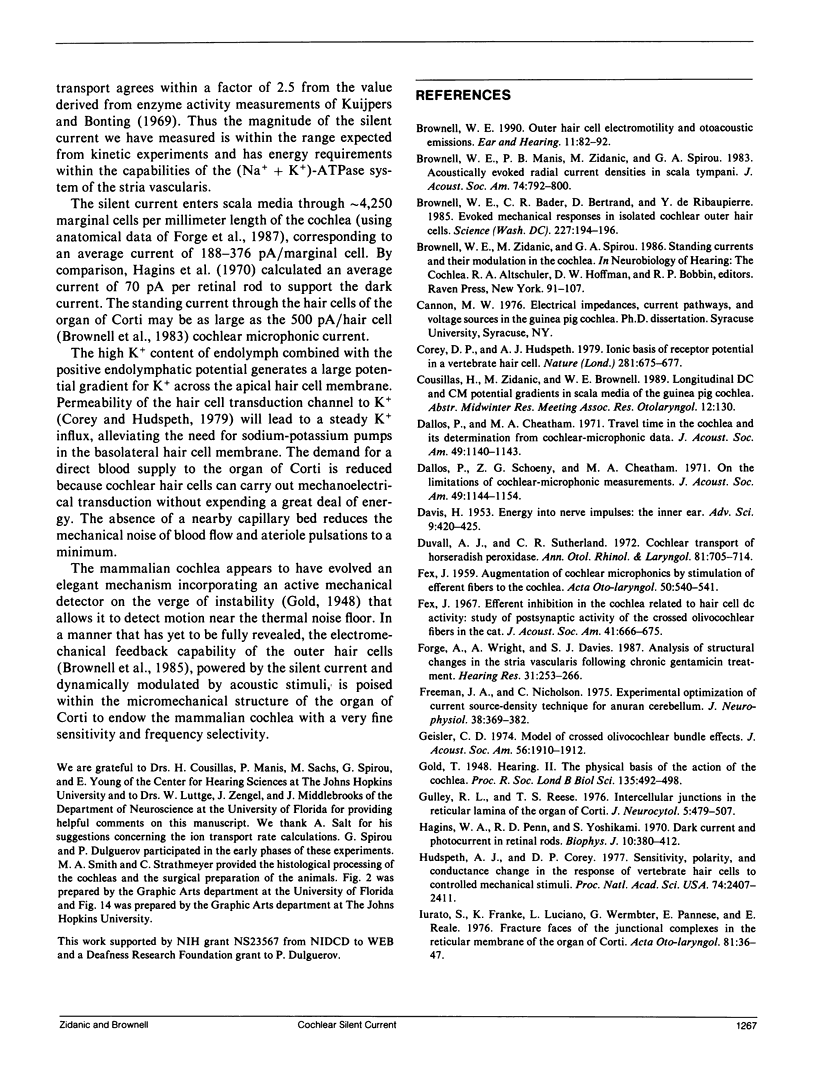
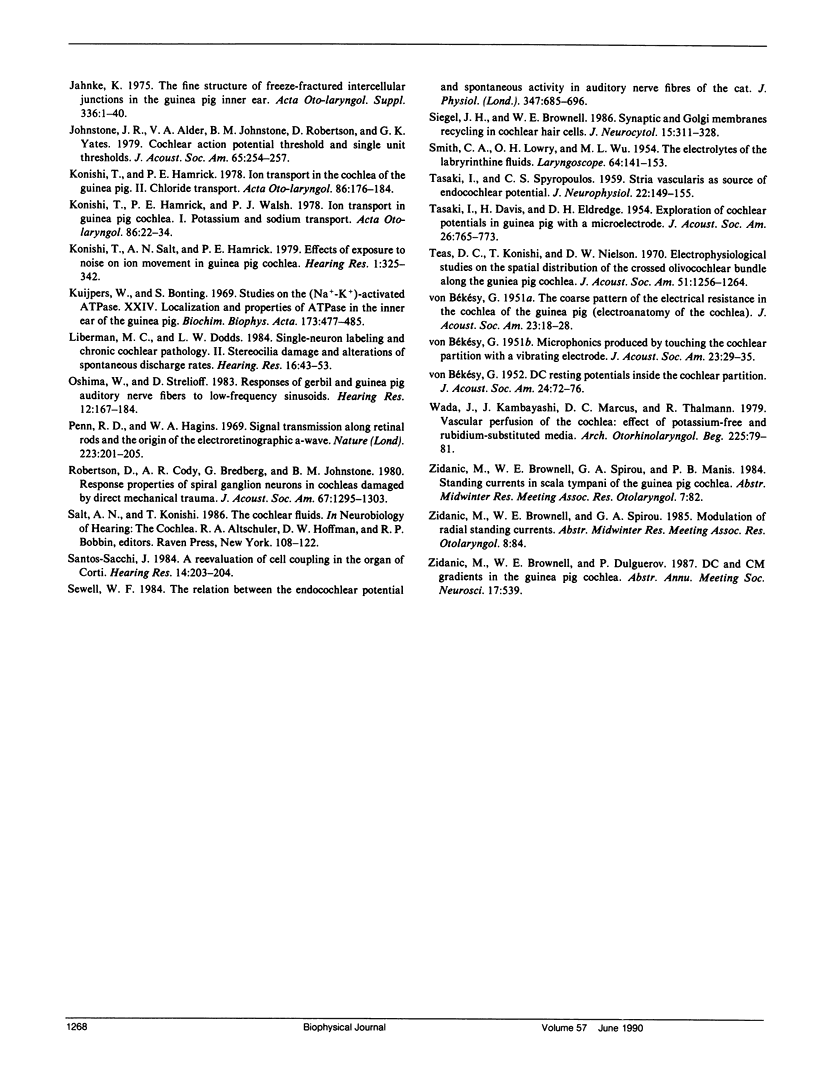
Images in this article
Selected References
These references are in PubMed. This may not be the complete list of references from this article.
- Brownell W. E., Bader C. R., Bertrand D., de Ribaupierre Y. Evoked mechanical responses of isolated cochlear outer hair cells. Science. 1985 Jan 11;227(4683):194–196. doi: 10.1126/science.3966153. [DOI] [PubMed] [Google Scholar]
- Brownell W. E., Manis P. B., Zidanic M., Spirou G. A. Acoustically evoked radial current densities in scala tympani. J Acoust Soc Am. 1983 Sep;74(3):792–800. doi: 10.1121/1.389866. [DOI] [PubMed] [Google Scholar]
- Brownell W. E. Outer hair cell electromotility and otoacoustic emissions. Ear Hear. 1990 Apr;11(2):82–92. doi: 10.1097/00003446-199004000-00003. [DOI] [PMC free article] [PubMed] [Google Scholar]
- Corey D. P., Hudspeth A. J. Ionic basis of the receptor potential in a vertebrate hair cell. Nature. 1979 Oct 25;281(5733):675–677. doi: 10.1038/281675a0. [DOI] [PubMed] [Google Scholar]
- Dallos P., Cheatham M. A. Travel time in the cochlea and its determination from cochlear-microphonic data. J Acoust Soc Am. 1971 Apr;49(4 Suppl):1140+–1140+. doi: 10.1121/1.1912475. [DOI] [PubMed] [Google Scholar]
- Dallos P., Schoeny Z. G., Cheatham M. A. On the limitations of cochlear-microphonic measurements. J Acoust Soc Am. 1971 Apr;49(4 Suppl):1144+–1144+. [PubMed] [Google Scholar]
- Duvall A. J., 3rd Cochlear transport of horseradish peroxidase. Ann Otol Rhinol Laryngol. 1972 Oct;81(5):705–713. doi: 10.1177/000348947208100512. [DOI] [PubMed] [Google Scholar]
- FEX J. Augmentation of cochlear microphonic by stimulation of efferent fibres to the cochlea; preliminary report. Acta Otolaryngol. 1959 Nov-Dec;50:540–541. doi: 10.3109/00016485909129230. [DOI] [PubMed] [Google Scholar]
- Fex J. Efferent inhibition in the cochlea related to hair-cell dc activity: study of postsynaptic activity of the crossed olivocochlear fibres in the cat. J Acoust Soc Am. 1967 Mar;41(3):666–675. doi: 10.1121/1.1910395. [DOI] [PubMed] [Google Scholar]
- Forge A., Wright A., Davies S. J. Analysis of structural changes in the stria vascularis following chronic gentamicin treatment. Hear Res. 1987 Dec 31;31(3):253–265. doi: 10.1016/0378-5955(87)90195-x. [DOI] [PubMed] [Google Scholar]
- Freeman J. A., Nicholson C. Experimental optimization of current source-density technique for anuran cerebellum. J Neurophysiol. 1975 Mar;38(2):369–382. doi: 10.1152/jn.1975.38.2.369. [DOI] [PubMed] [Google Scholar]
- Geisler C. D. Model of crossed olivocochlear bundle effects. J Acoust Soc Am. 1974 Dec;56(6):1910–1912. doi: 10.1121/1.1903533. [DOI] [PubMed] [Google Scholar]
- Gulley R. L., Reese T. S. Intercellular junctions in the reticular lamina of the organ of Corti. J Neurocytol. 1976 Aug;5(4):479–507. doi: 10.1007/BF01181652. [DOI] [PubMed] [Google Scholar]
- Hagins W. A., Penn R. D., Yoshikami S. Dark current and photocurrent in retinal rods. Biophys J. 1970 May;10(5):380–412. doi: 10.1016/S0006-3495(70)86308-1. [DOI] [PMC free article] [PubMed] [Google Scholar]
- Hudspeth A. J., Corey D. P. Sensitivity, polarity, and conductance change in the response of vertebrate hair cells to controlled mechanical stimuli. Proc Natl Acad Sci U S A. 1977 Jun;74(6):2407–2411. doi: 10.1073/pnas.74.6.2407. [DOI] [PMC free article] [PubMed] [Google Scholar]
- Jahnke K. The fine structure of freeze-fractured intercellular junctions in the guinea pig inner ear. Acta Otolaryngol Suppl. 1975;336:1–40. [PubMed] [Google Scholar]
- Johnstone J. R., Alder V. A., Johnstone B. M., Robertson D., Yates G. K. Cochlear action potential threshold and single unit thresholds. J Acoust Soc Am. 1979 Jan;65(1):254–257. doi: 10.1121/1.382244. [DOI] [PubMed] [Google Scholar]
- Konishi T., Hamrick P. E. Ion transport in the cochlea of guinea pig. II. Chloride transport. Acta Otolaryngol. 1978 Sep-Oct;86(3-4):176–184. doi: 10.3109/00016487809124734. [DOI] [PubMed] [Google Scholar]
- Konishi T., Hamrick P. E., Walsh P. J. Ion transport in guinea pig cochlea. I. Potassium and sodium transport. Acta Otolaryngol. 1978 Jul-Aug;86(1-2):22–34. doi: 10.3109/00016487809124717. [DOI] [PubMed] [Google Scholar]
- Konishi T., Salt A. N., Hamrick P. E. Effects of exposure to noise on ion movement in guinea pig cochlea. Hear Res. 1979 Dec;1(4):325–342. doi: 10.1016/0378-5955(79)90004-2. [DOI] [PubMed] [Google Scholar]
- Kuijpers W., Bonting S. L. Studies on (Na+-K+)-activated ATPase. XXIV. Localization and properties of ATPase in the inner ear of the guinea pig. Biochim Biophys Acta. 1969 Apr;173(3):477–485. doi: 10.1016/0005-2736(69)90012-1. [DOI] [PubMed] [Google Scholar]
- Liberman M. C., Dodds L. W. Single-neuron labeling and chronic cochlear pathology. II. Stereocilia damage and alterations of spontaneous discharge rates. Hear Res. 1984 Oct;16(1):43–53. doi: 10.1016/0378-5955(84)90024-8. [DOI] [PubMed] [Google Scholar]
- Oshima W., Strelioff D. Responses of gerbil and guinea pig auditory nerve fibers to low-frequency sinusoids. Hear Res. 1983 Nov;12(2):167–184. doi: 10.1016/0378-5955(83)90104-1. [DOI] [PubMed] [Google Scholar]
- Penn R. D., Hagins W. A. Signal transmission along retinal rods and the origin of the electroretinographic a-wave. Nature. 1969 Jul 12;223(5202):201–204. doi: 10.1038/223201a0. [DOI] [PubMed] [Google Scholar]
- Robertson D., Cody A. R., Bredberg G., Johnstone B. M. Response properties of spiral ganglion neurons in cochleas damaged by direct mechanical trauma. J Acoust Soc Am. 1980 Apr;67(4):1295–1303. doi: 10.1121/1.384182. [DOI] [PubMed] [Google Scholar]
- SMITH C. A., LOWRY O. H., WU M. L. The electrolytes of the labyrinthine fluids. Laryngoscope. 1954 Mar;64(3):141–153. doi: 10.1288/00005537-195403000-00001. [DOI] [PubMed] [Google Scholar]
- Santos-Sacchi J. A re-evaluation of cell coupling in the organ of Corti. Hear Res. 1984 May;14(2):203–204. doi: 10.1016/0378-5955(84)90019-4. [DOI] [PubMed] [Google Scholar]
- Sewell W. F. The relation between the endocochlear potential and spontaneous activity in auditory nerve fibres of the cat. J Physiol. 1984 Feb;347:685–696. doi: 10.1113/jphysiol.1984.sp015090. [DOI] [PMC free article] [PubMed] [Google Scholar]
- Siegel J. H., Brownell W. E. Synaptic and Golgi membrane recycling in cochlear hair cells. J Neurocytol. 1986 Jun;15(3):311–328. doi: 10.1007/BF01611434. [DOI] [PubMed] [Google Scholar]
- TASAKI I., SPYROPOULOS C. S. Stria vascularis as source of endocochlear potential. J Neurophysiol. 1959 Mar;22(2):149–155. doi: 10.1152/jn.1959.22.2.149. [DOI] [PubMed] [Google Scholar]
- Teas D. C., Konishi T., Nielsen D. W. Electrophysiological studies on the spatial distribution of the crossed olivocochlear bundle along the guinea pig cochlea. J Acoust Soc Am. 1972 Apr;51(4):1256–1264. doi: 10.1121/1.1912969. [DOI] [PubMed] [Google Scholar]
- Wada J., Kambayashi J., Marcus D. C., Thalmann R. Vascular perfusion of the cochlea: effect of potassium-free and rubidium-substituted media. Arch Otorhinolaryngol. 1979;225(2):79–81. doi: 10.1007/BF00455206. [DOI] [PubMed] [Google Scholar]