Abstract
1. A method has been developed for studying the differentiation in tissue culture of ectoderm and mesoderm derivatives, dissected from amphibian embryos which have just completed neurulation. 2. Neurones, striated muscle cells and pigment cells, together with other unidentifiable cell types, differentiated as a monolayer with approximately the same time course as in the whole embryo. The proportion of different cell types in the cultures was measured quantitatively by cell counting. 3. Treatment of embryos during neurulation with the cardiac glycoside strophanthidin reduced the number of neurones which subsequently differentiated in culture. Other cell types were not affected. 4. The relationship between inhibition of neural differentiation and strophanthidin concentration was sigmoid, with maximum inhibition at 10(-5) M-strophanthidin and the mid-point at 5 X 10(-7) M-strophanthidin. 35% of neurones differentiating in culture were not affected by glycoside treatment. 5. The glycoside hexahydroscillaren A had no effect on neural differentiation. 6. Increasing extracellular potassium to 100 nM during strophanthidin treatment completely protected differentiating neurones from the inhibitory effect of strophanthidin. 7. Treatment of embryos with 100 mM-KCl during neurulation had no effect on the subsequent differentiation of neurones. 8. Treatment of cultures with an antibody to mouse salivary gland Nerve Growth Factor reduced the number of neurones by 30%. 9. Exposure to strophanthidin while the embryo moved from the early neural fold stage to the late neural fold stage was as effective in reducing subsequent neural differentiation as treatment throughout neurulation. 10. The proportion of nerve cells in the cultures was not affected if strophanthidin treatment ended before the early neural fold stage or did not begin until the late neural fold stage. 11. Embryos treated with strophanthidin during neurulation and then allowed to grow into tadpoles developed abnormal nervous systems. 10(-6) M-strophanthidin had little effect on the volume of grey matter, but reduced the white matter by 50%. 12. The results are consistent with the view that strophanthidin achieves its effect on neural differentiation by inhibiting the sodium pump. They are discussed in the light of the suggestion that activation of the sodium pump is an essential part of nerual differentiation.
Full text
PDF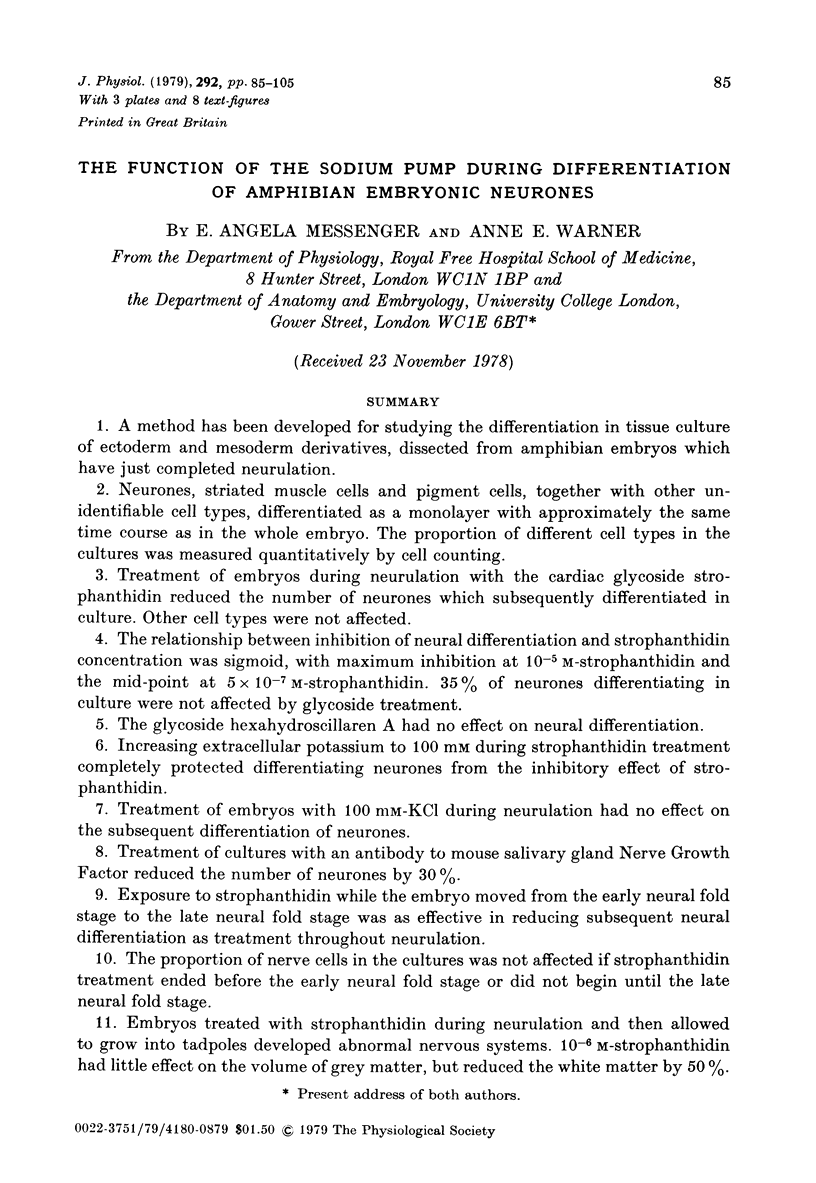
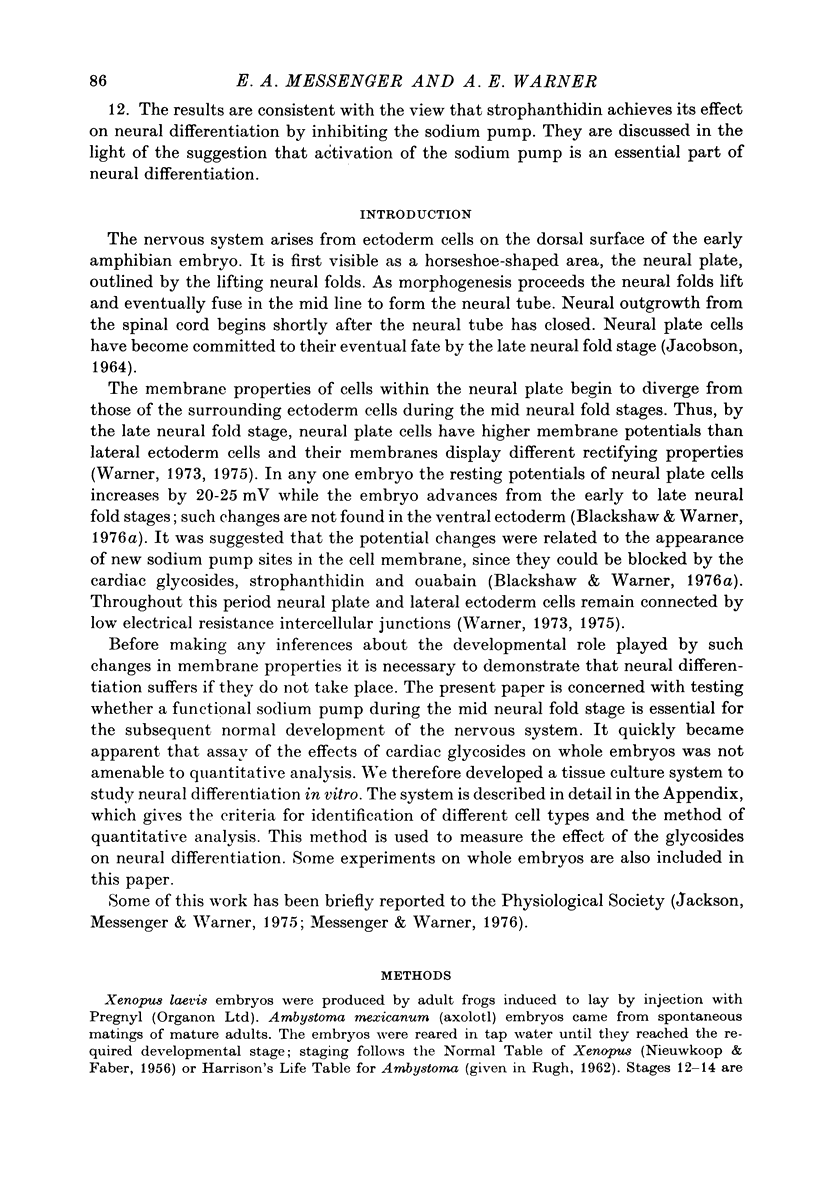
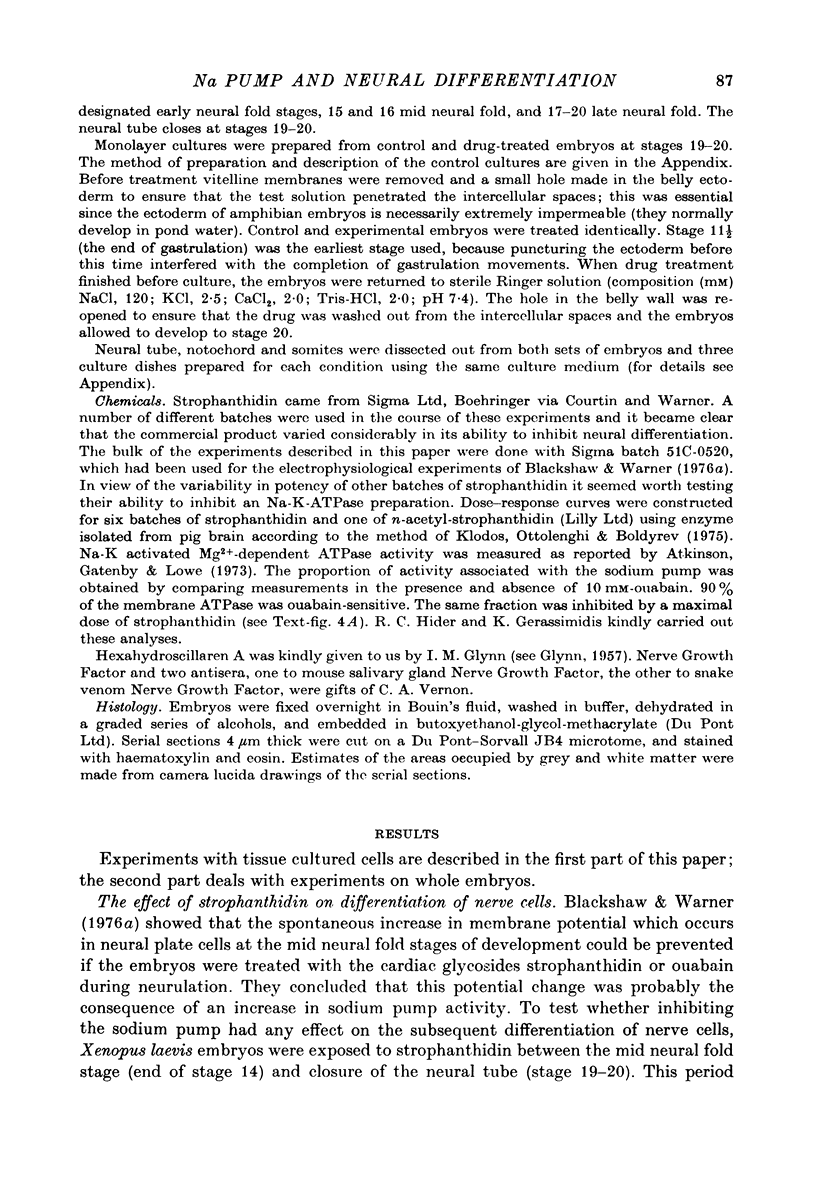
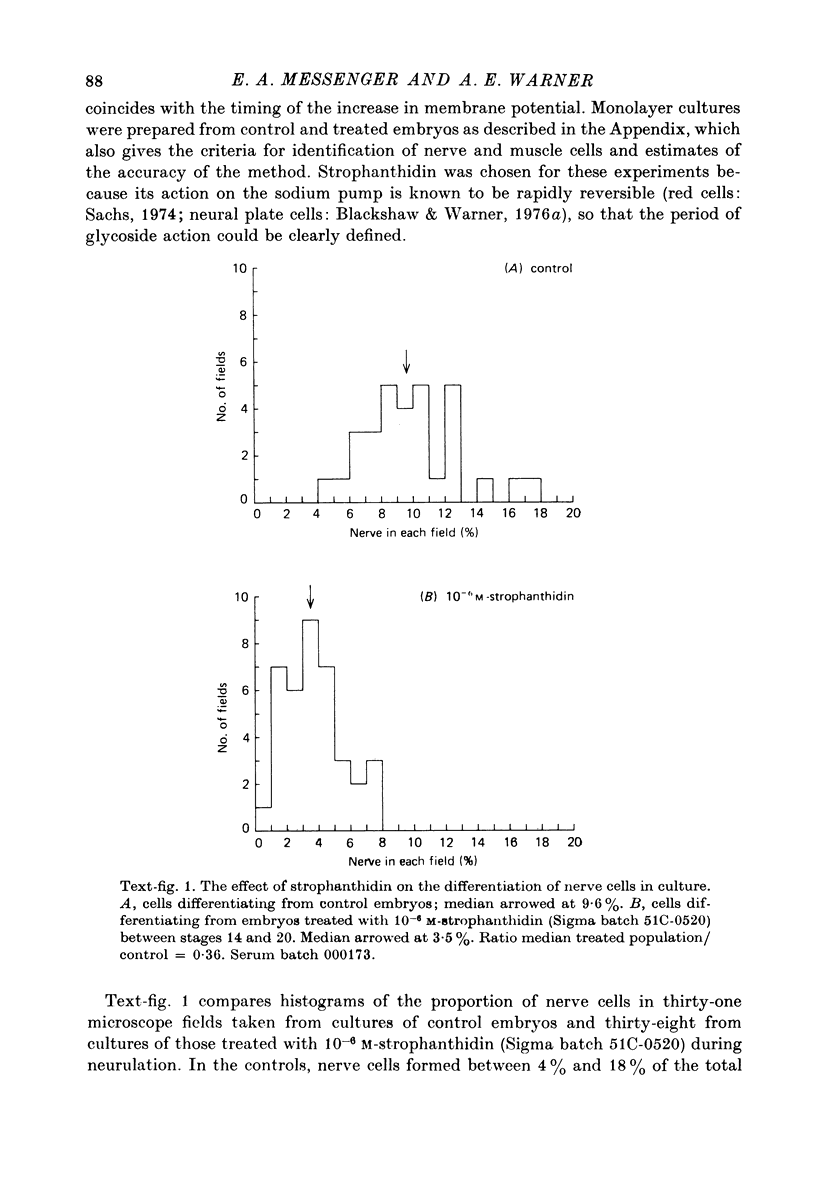
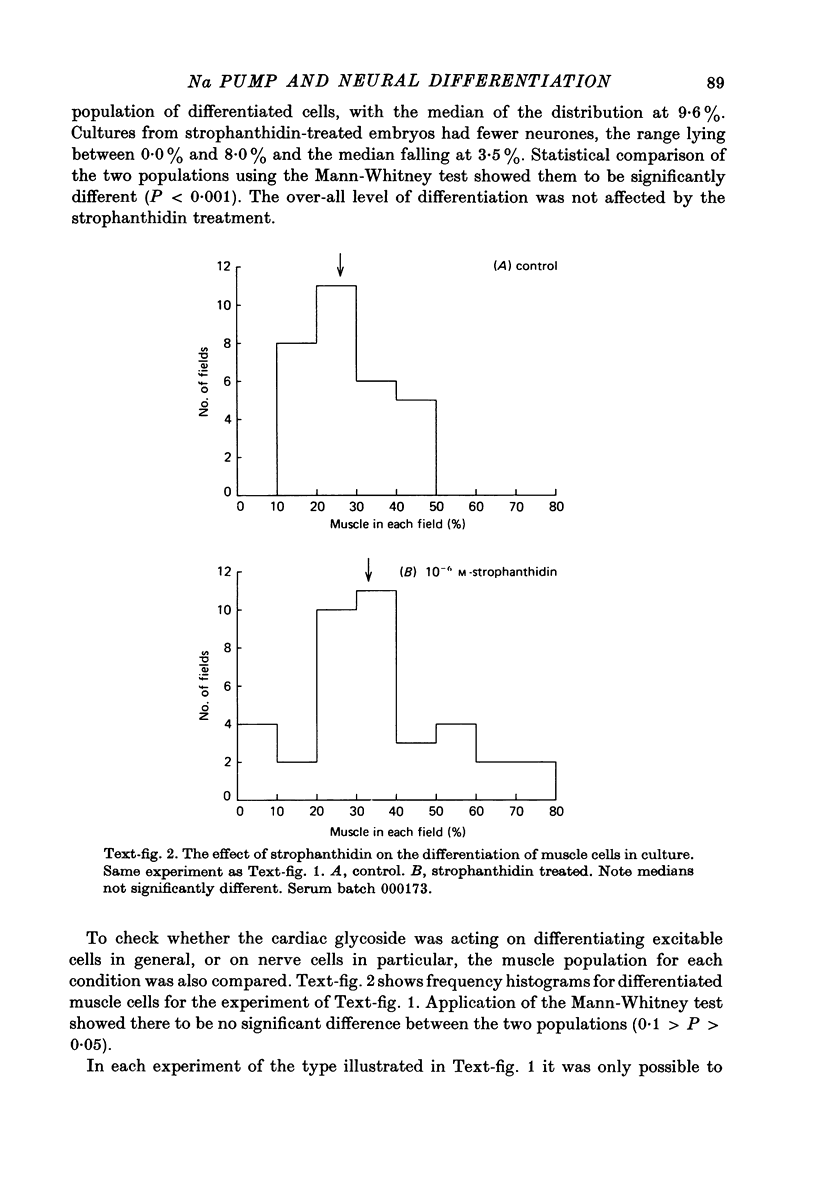
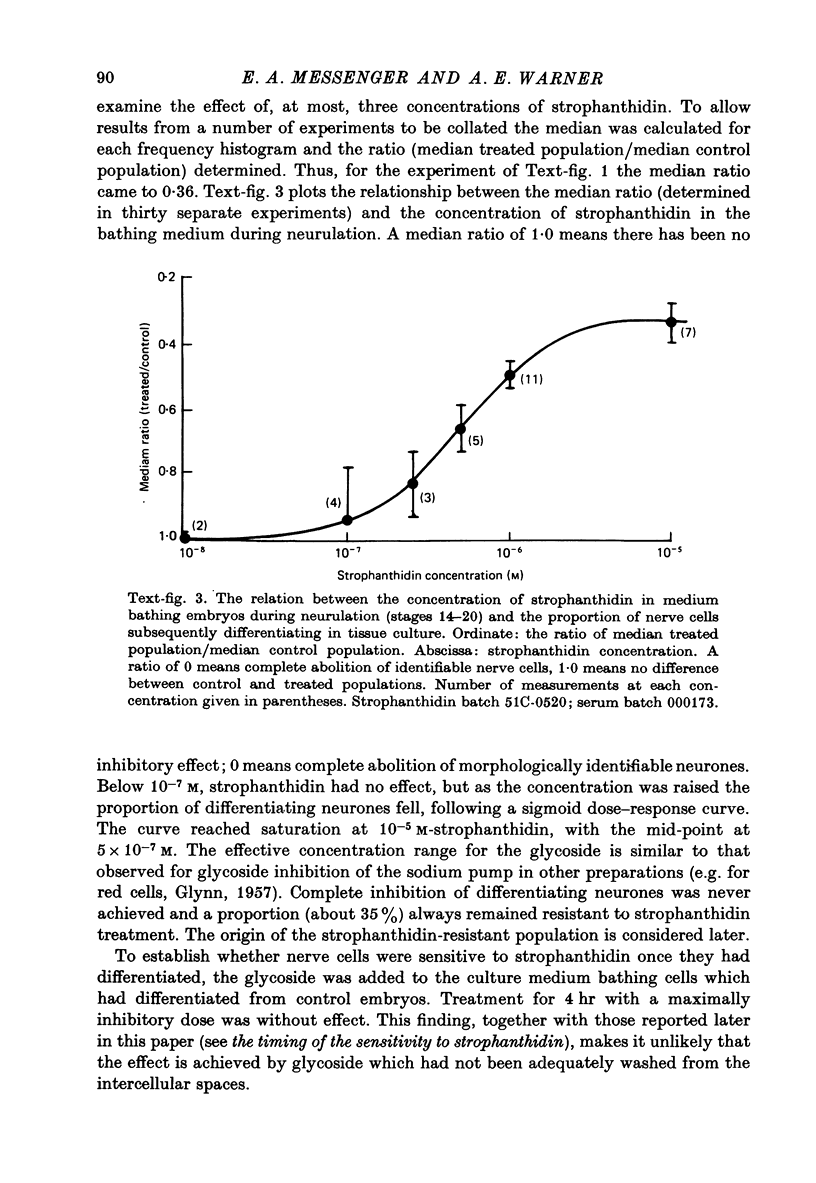
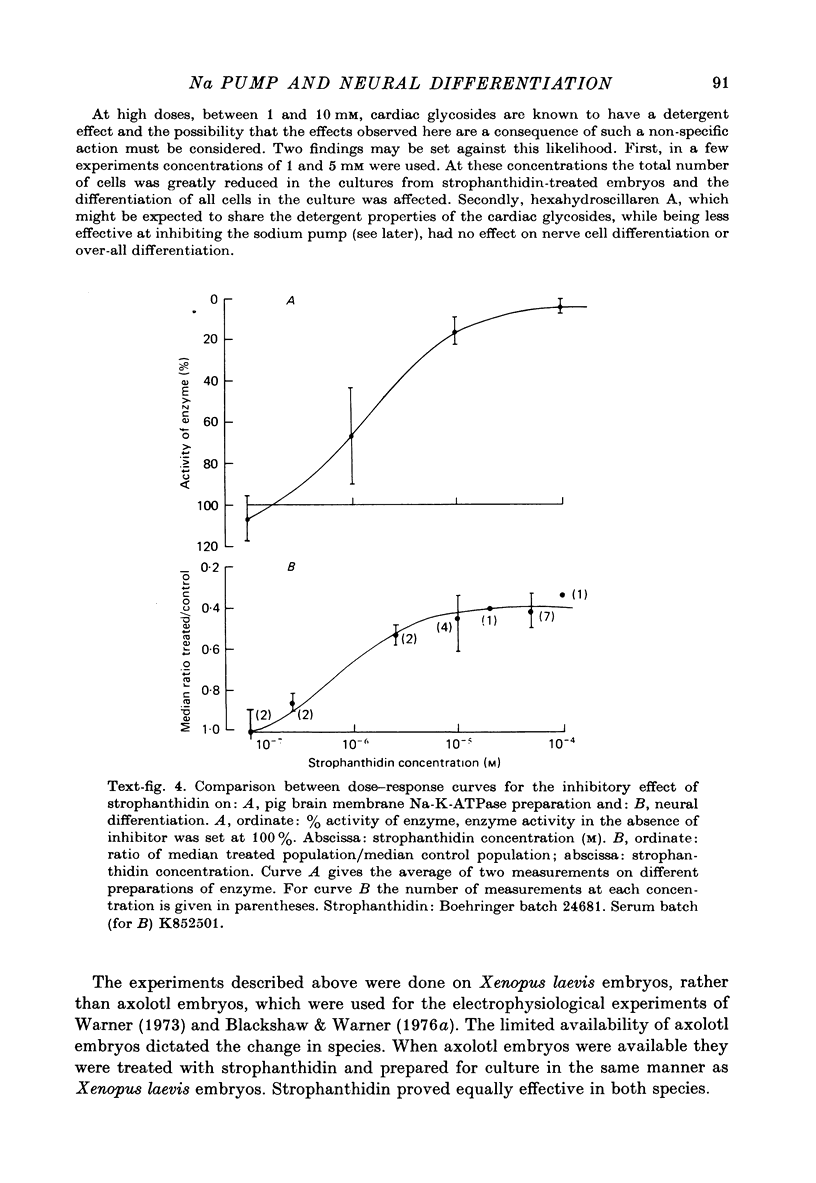
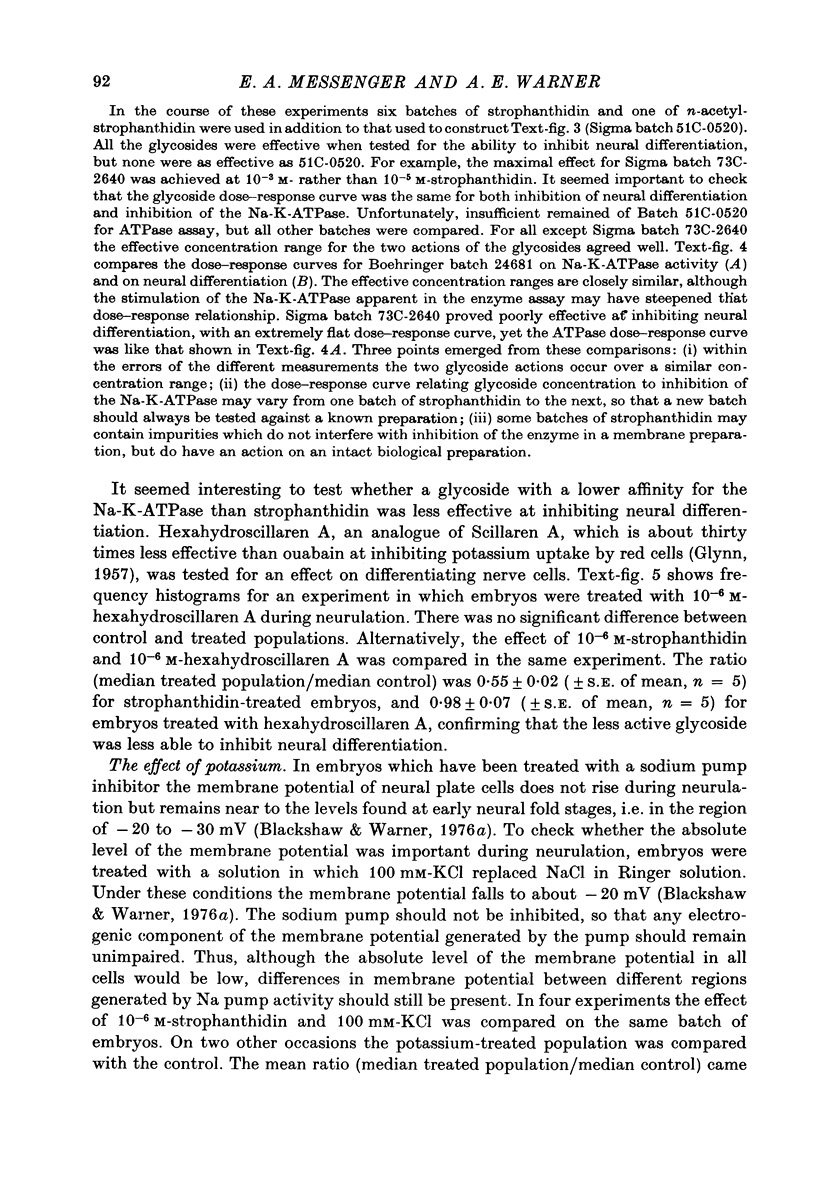
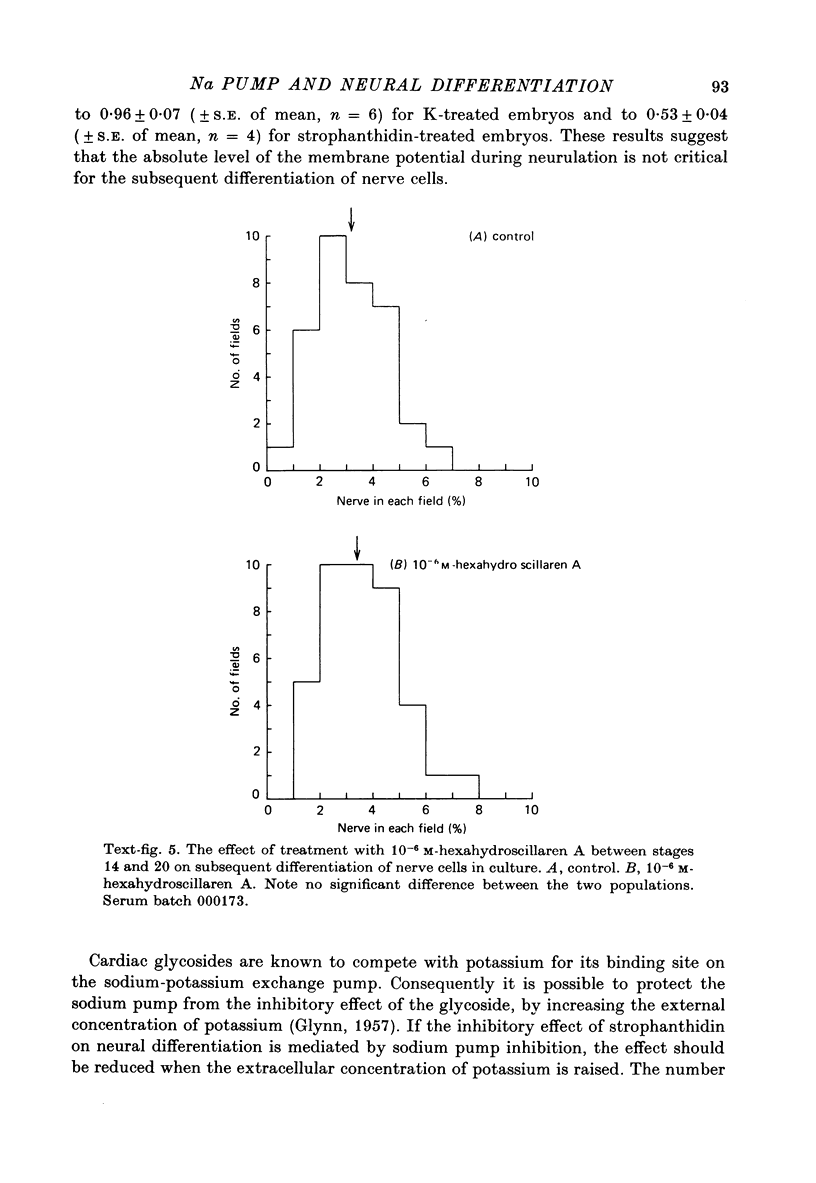
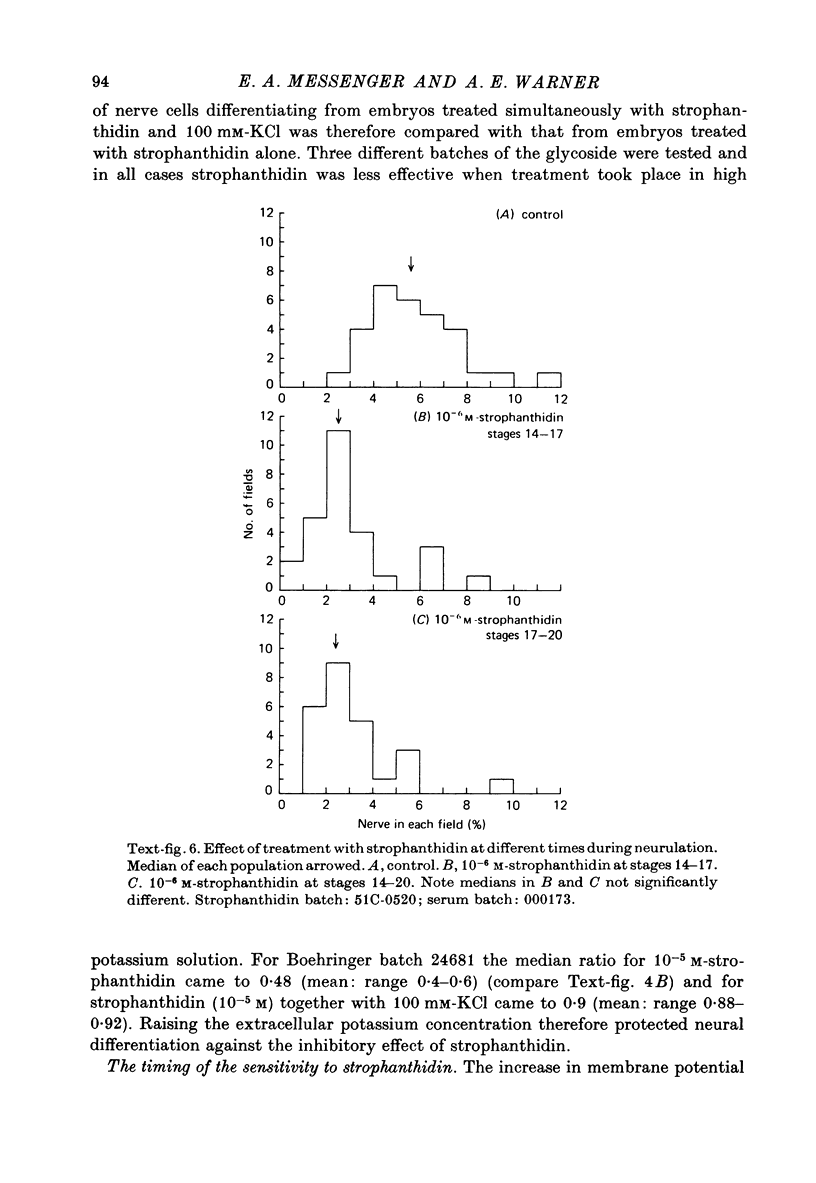
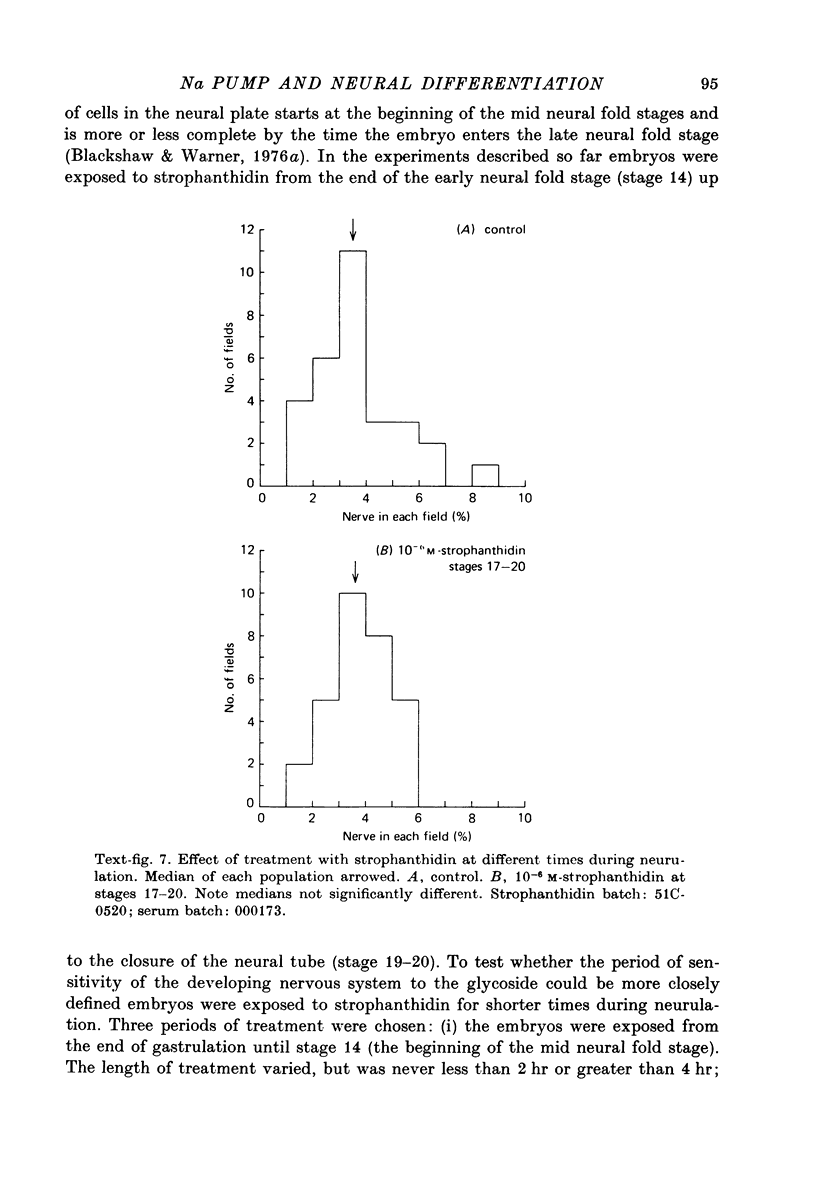
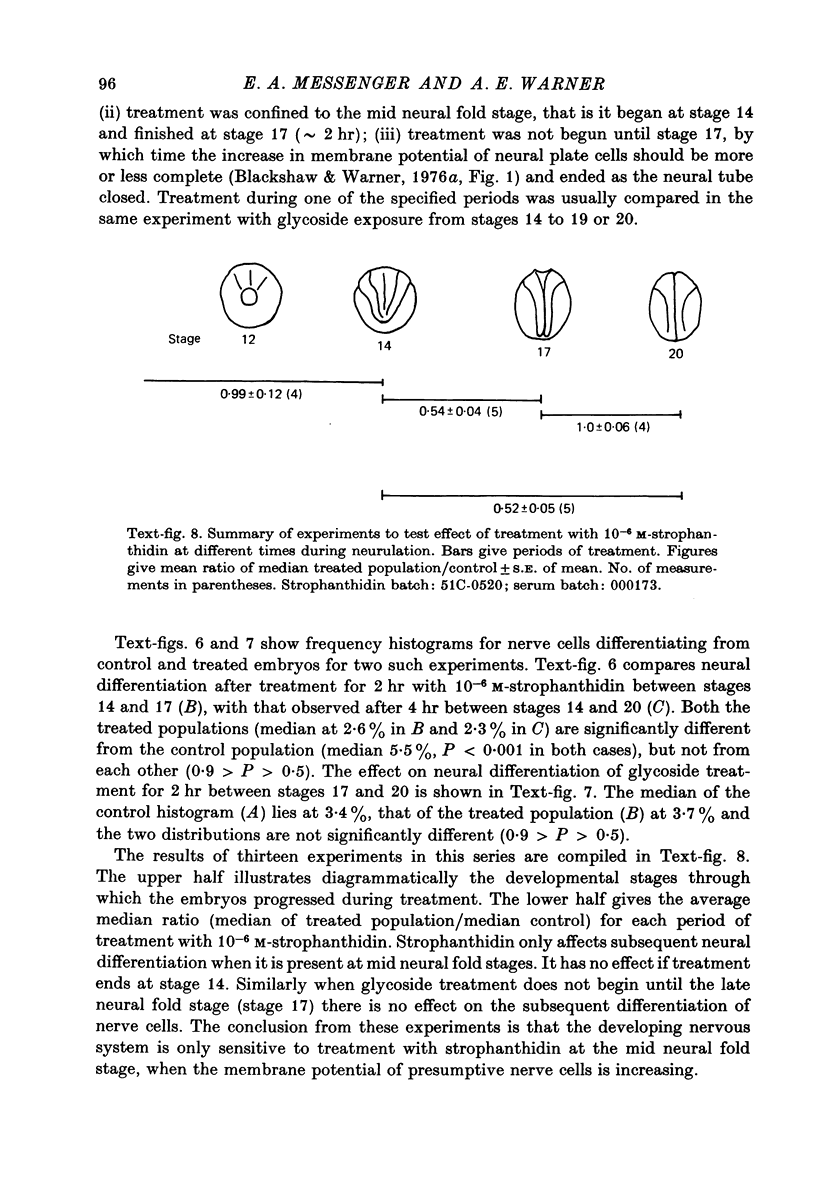
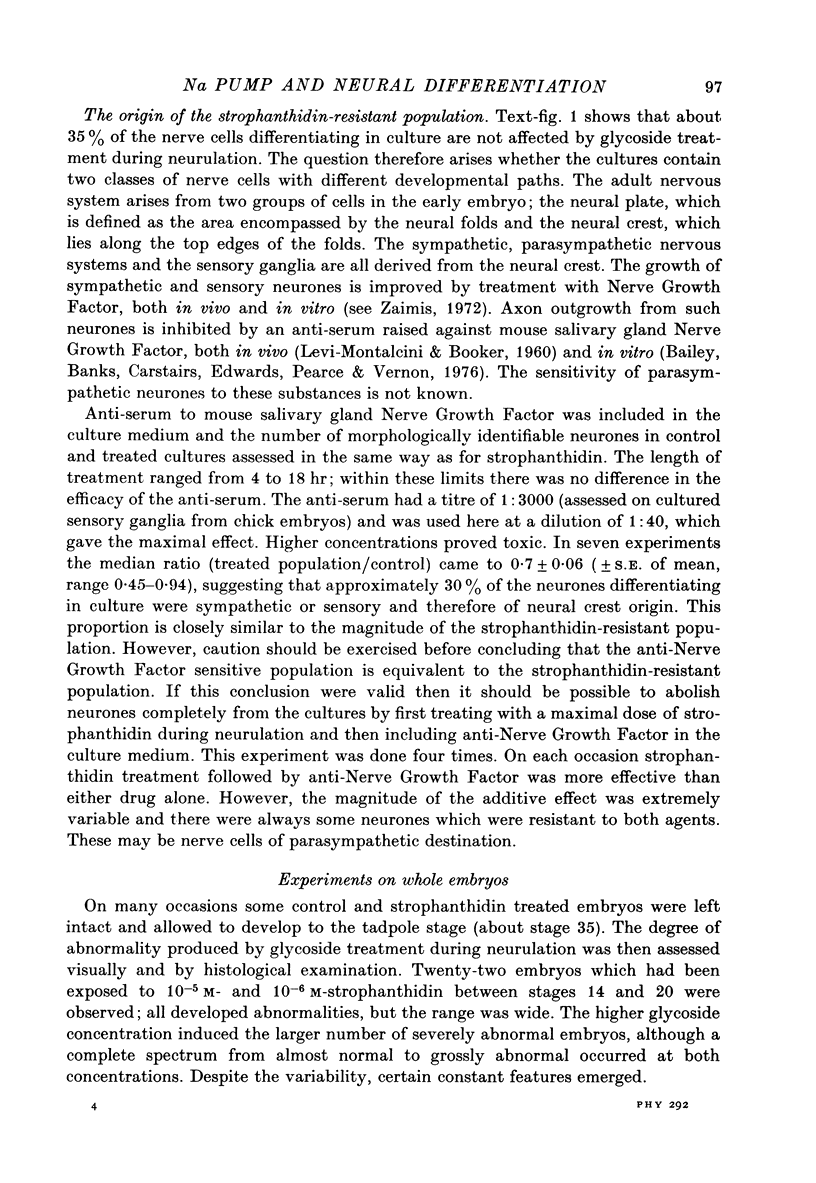
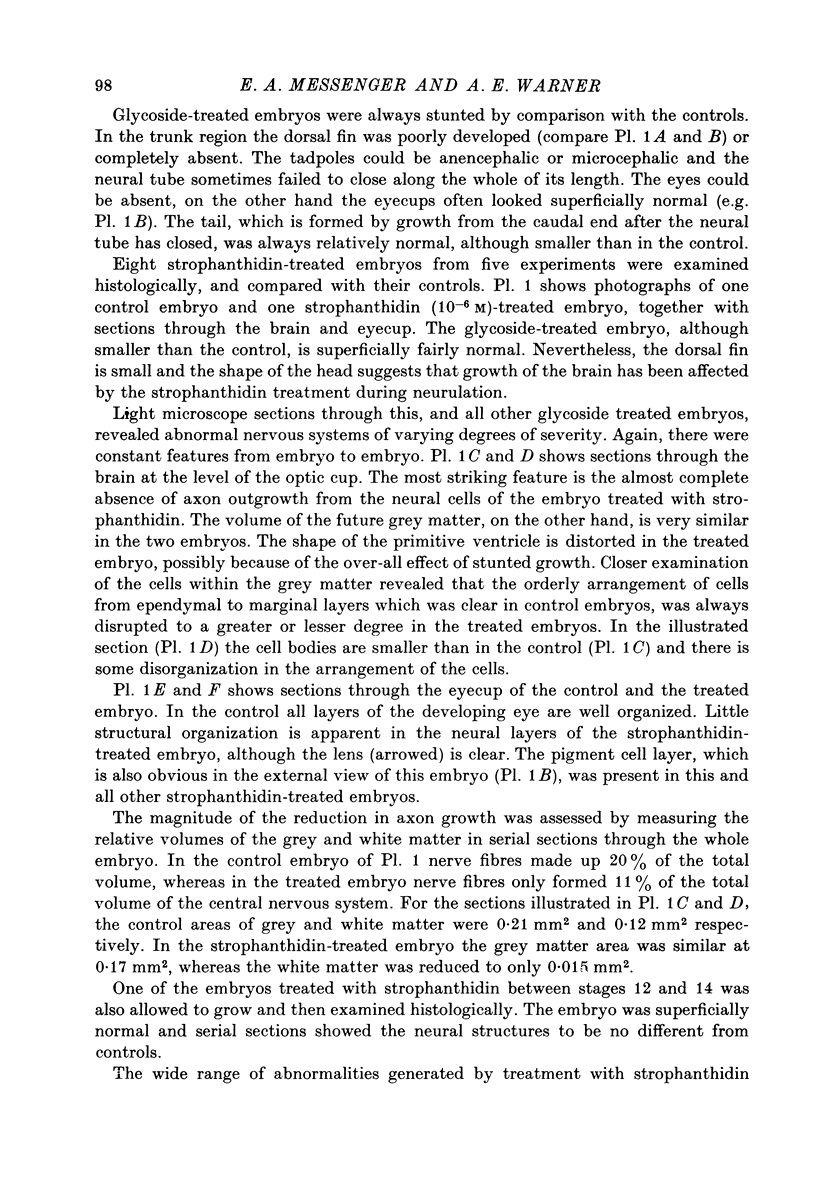
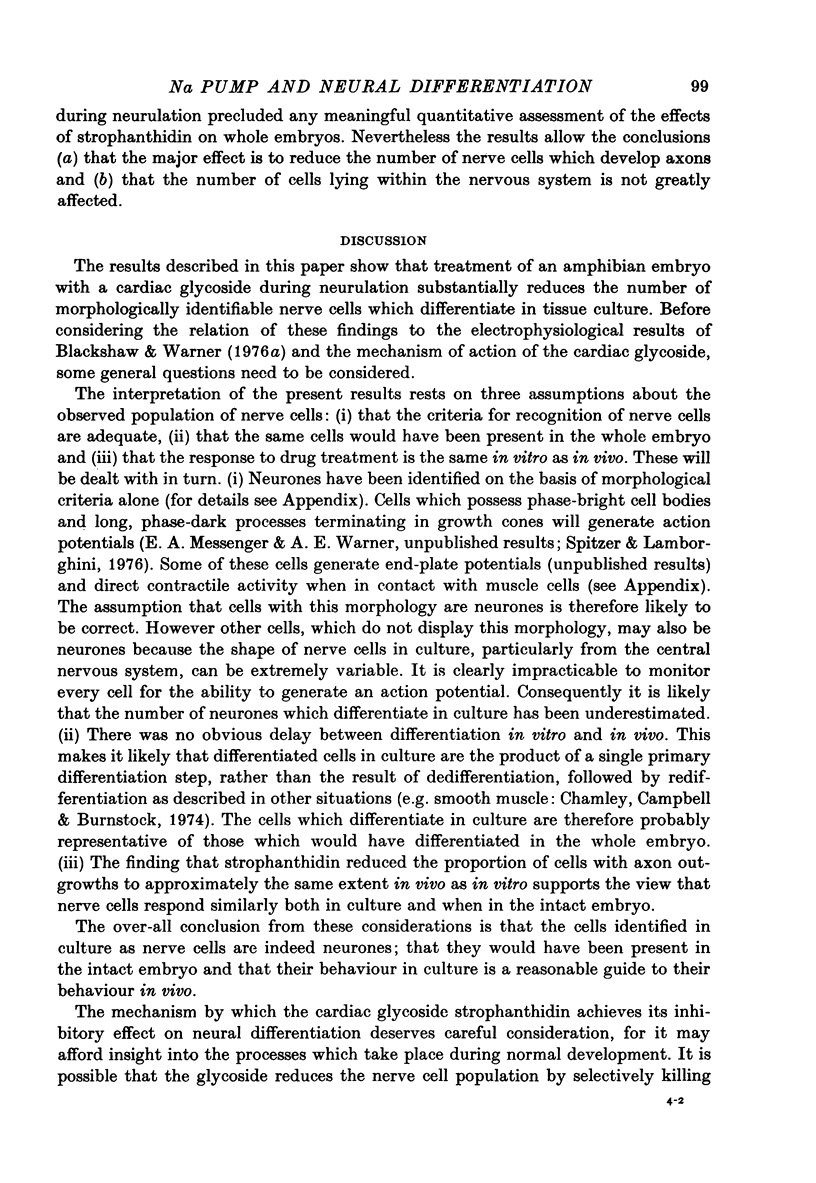
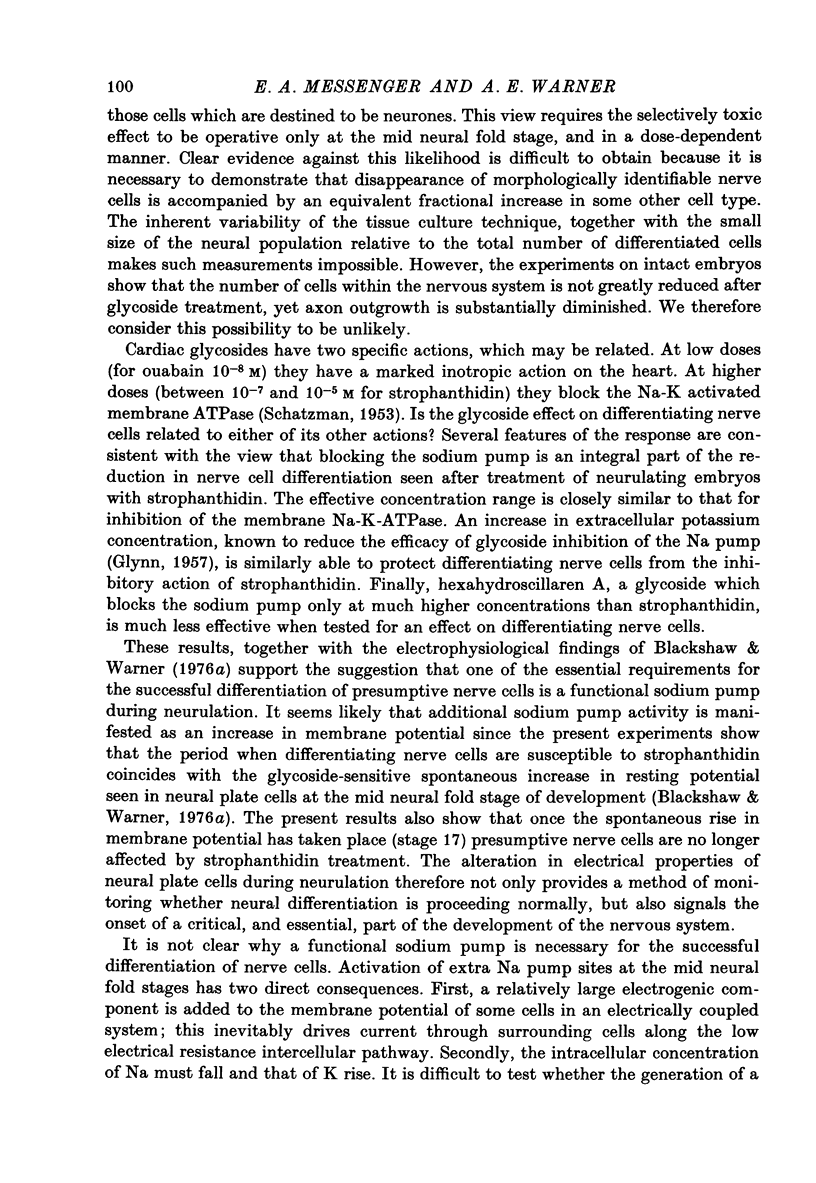
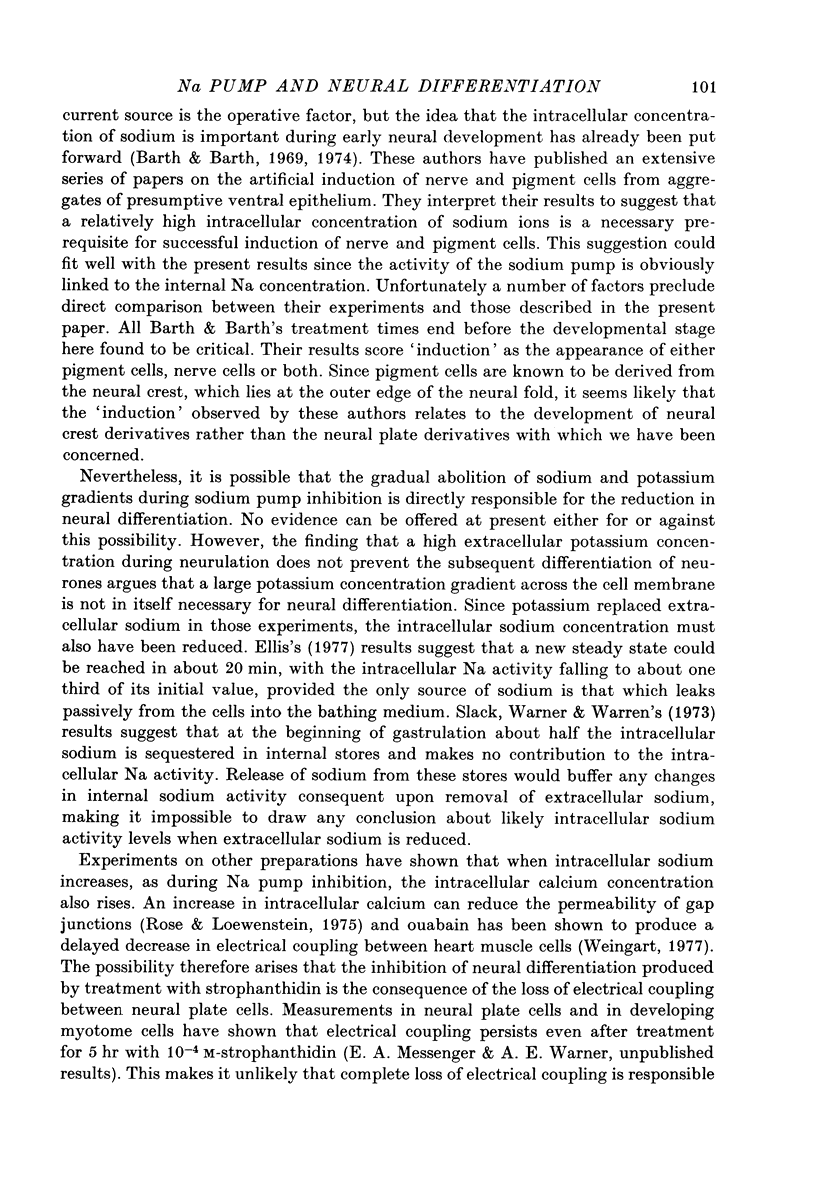
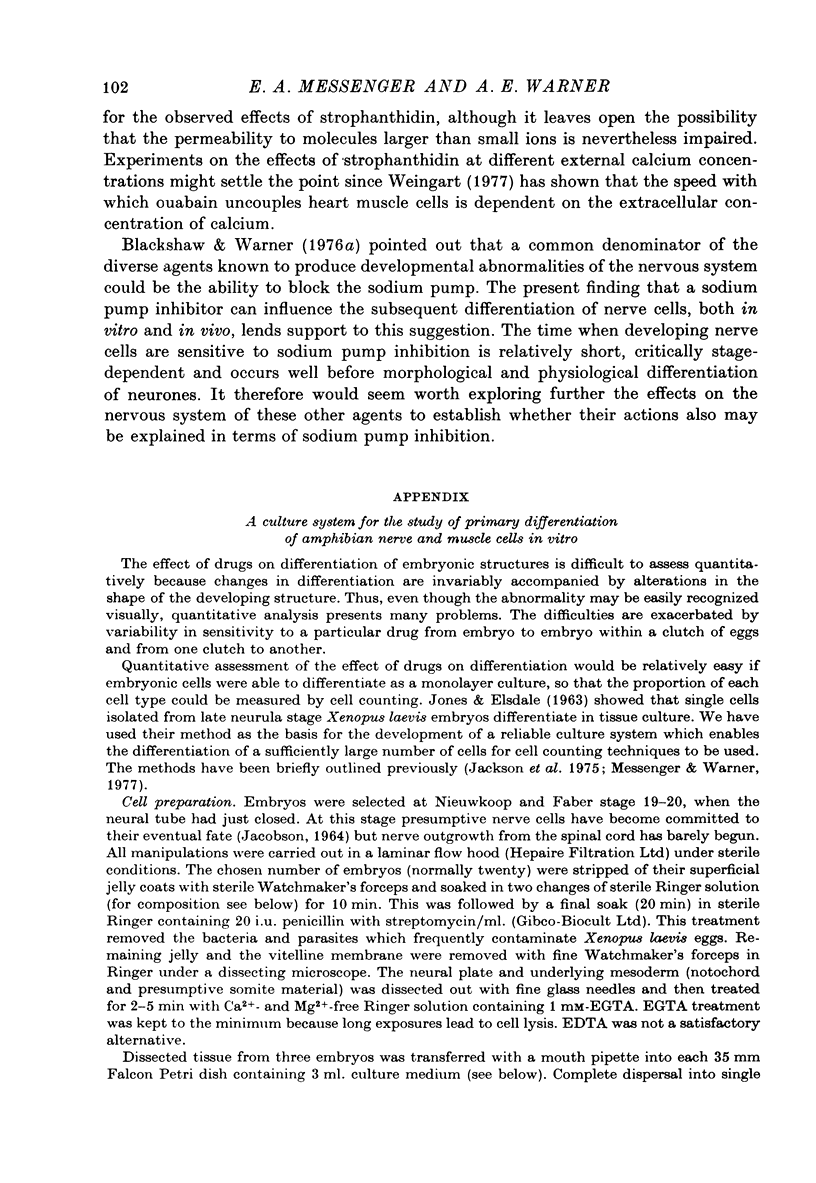
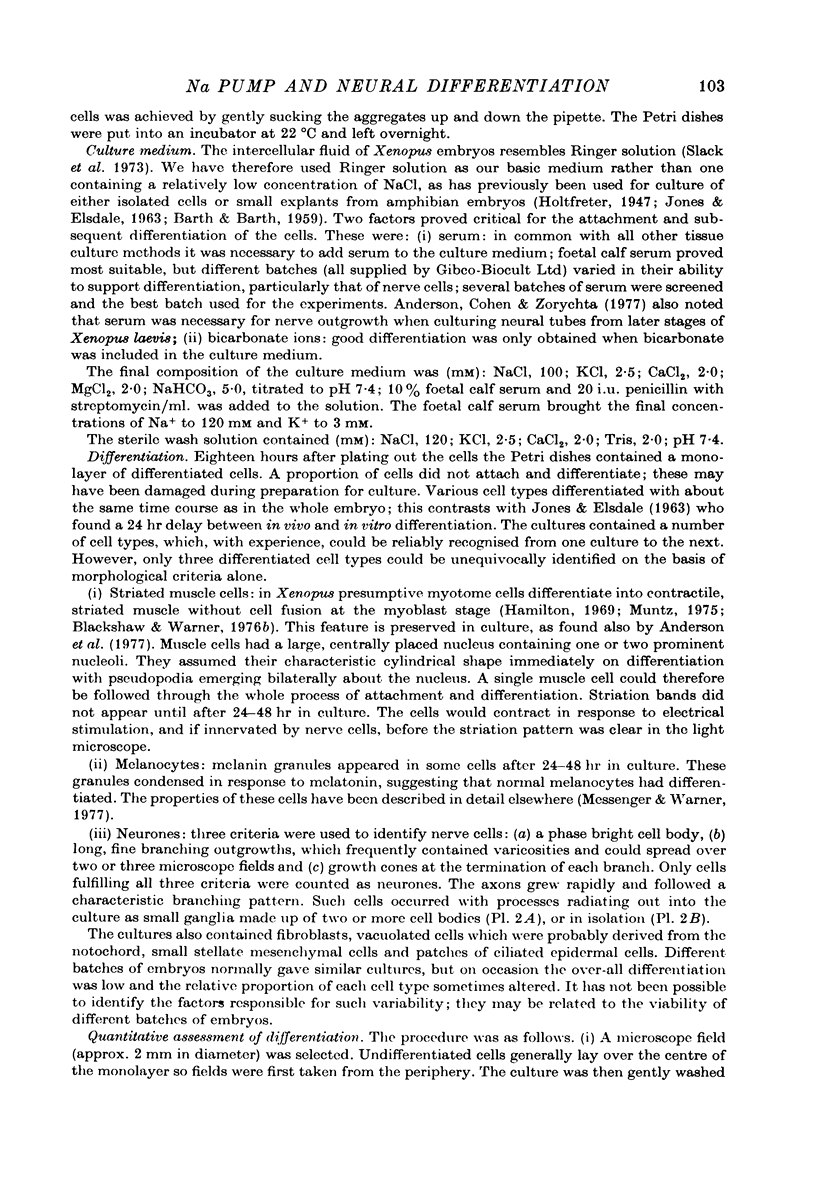
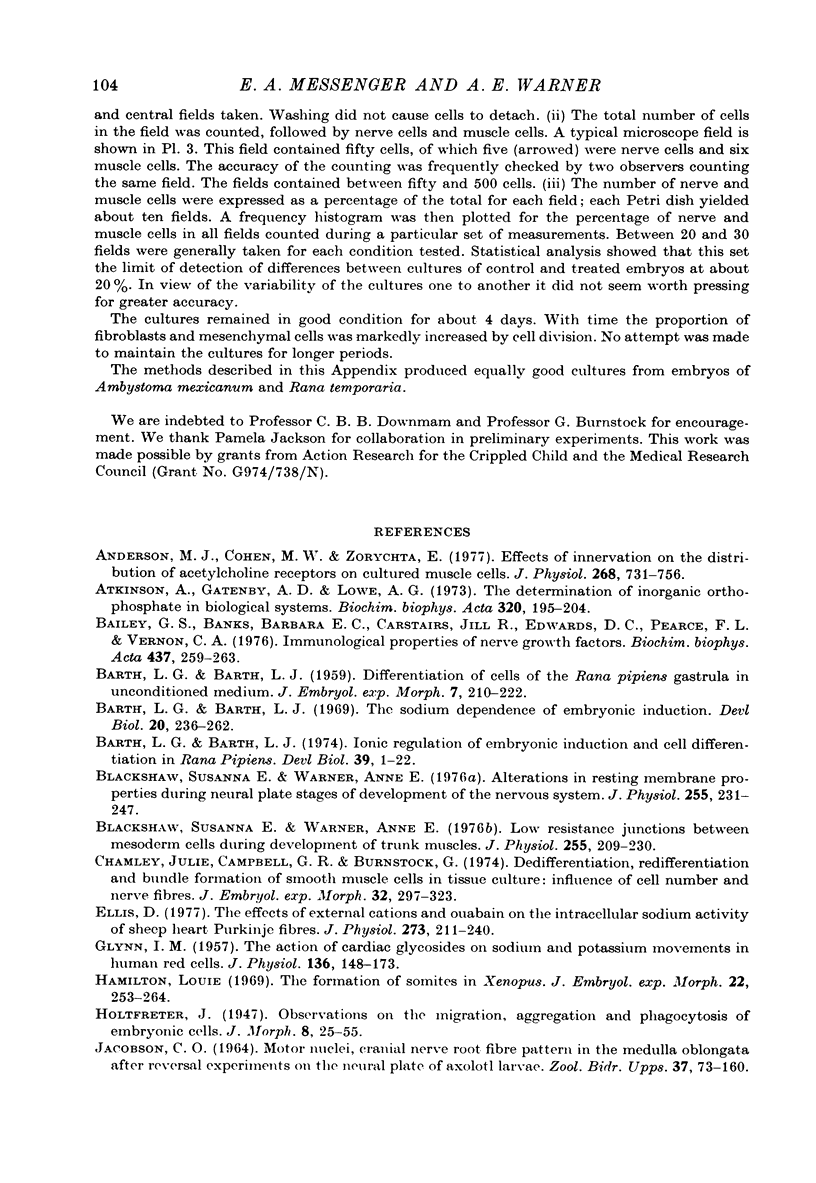
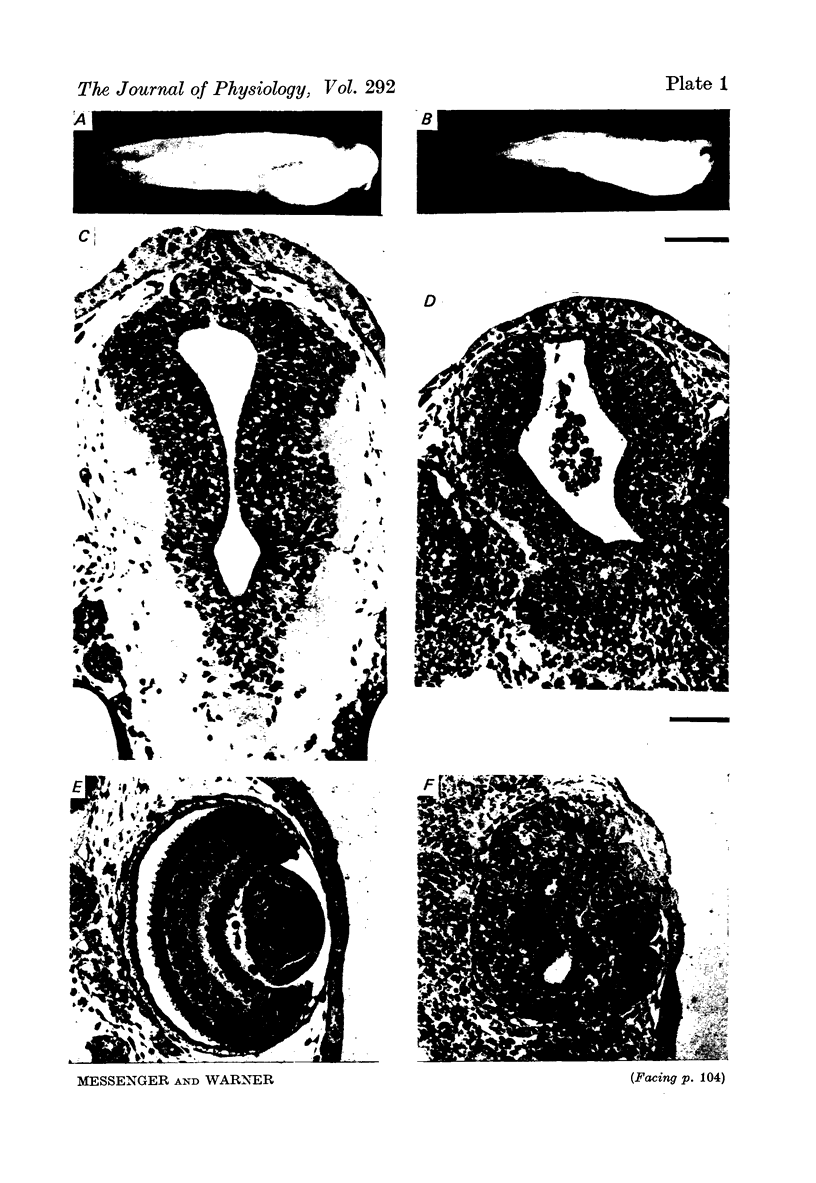
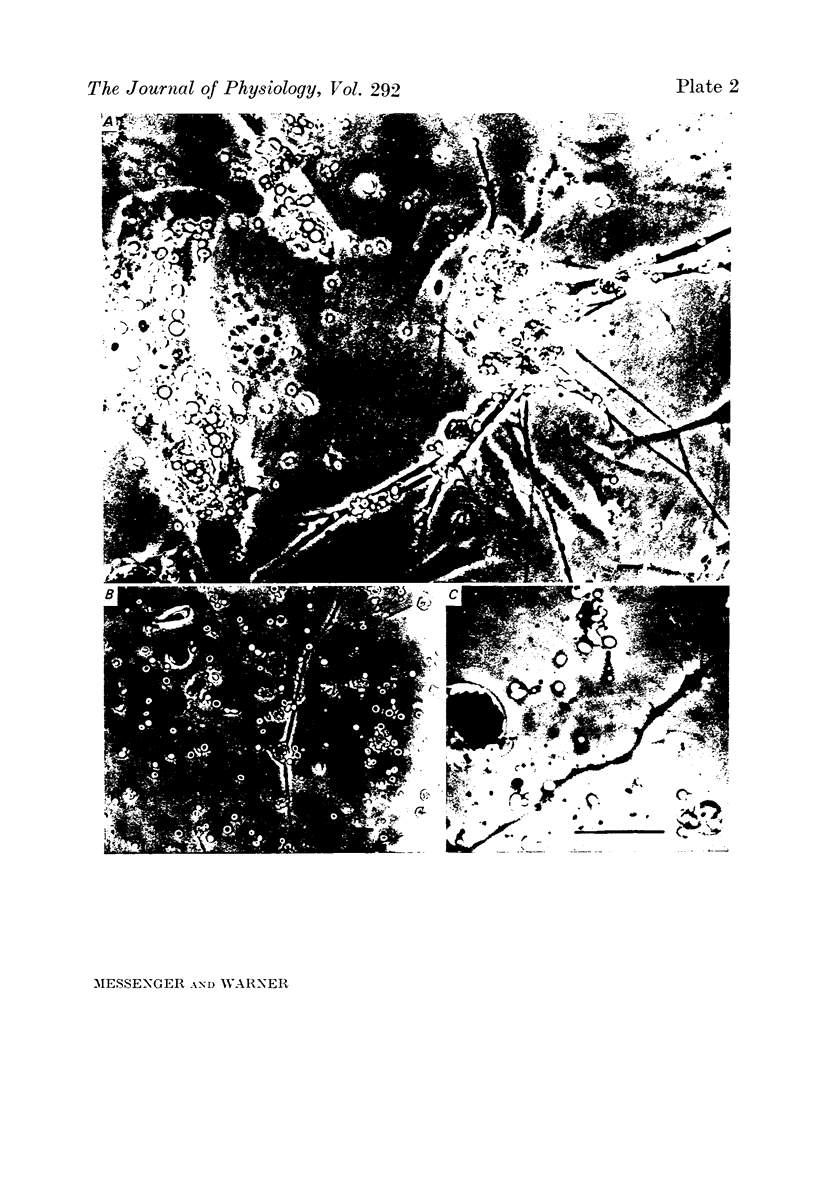
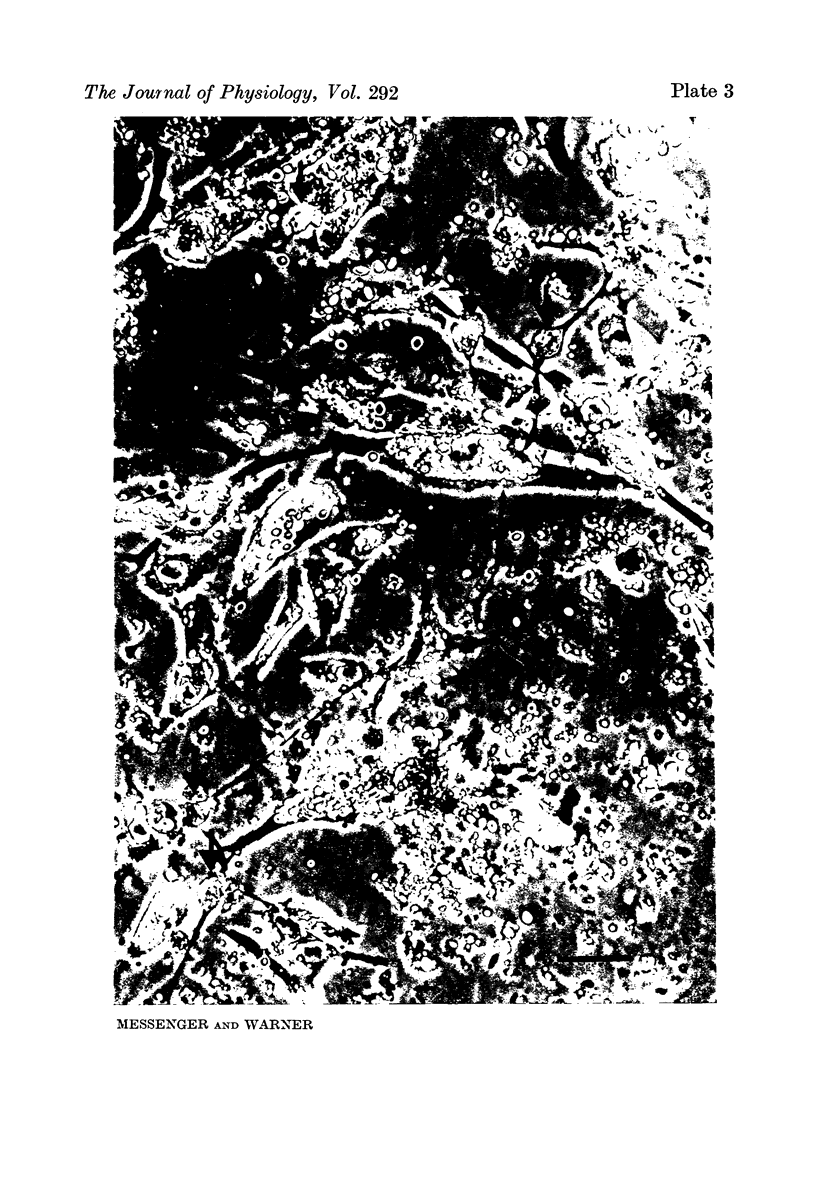
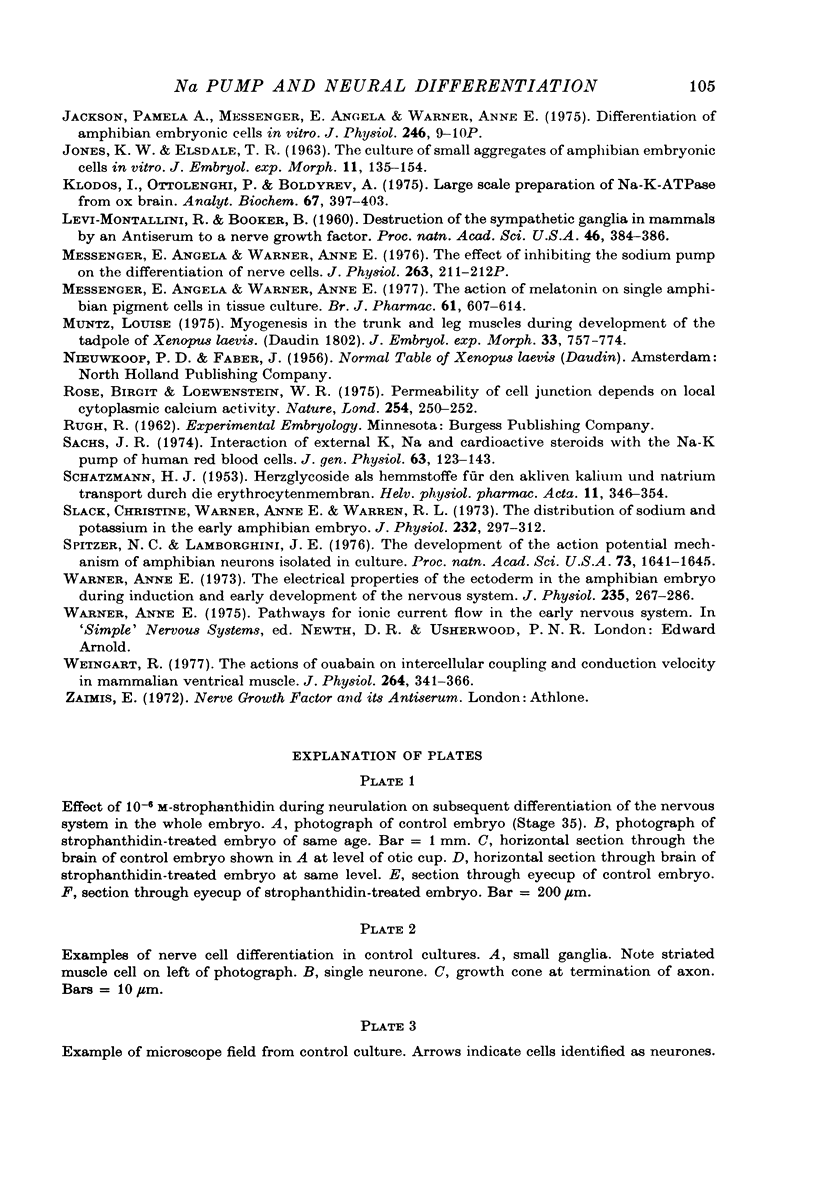
Images in this article
Selected References
These references are in PubMed. This may not be the complete list of references from this article.
- Anderson M. J., Cohen M. W., Zorychta E. Effects of innervation on the distribution of acetylcholine receptors on cultured muscle cells. J Physiol. 1977 Jul;268(3):731–756. doi: 10.1113/jphysiol.1977.sp011879. [DOI] [PMC free article] [PubMed] [Google Scholar]
- Atkinson A., Gatenby A. D., Lowe A. G. The determination of inorganic orthophosphate in biological systems. Biochim Biophys Acta. 1973 Aug 17;320(1):195–204. doi: 10.1016/0304-4165(73)90178-5. [DOI] [PubMed] [Google Scholar]
- BARTH L. G., BARTH L. J. Differentiation of cells of the Rana pipiens gastrula in unconditioned medium. J Embryol Exp Morphol. 1959 Jun;7:210–222. [PubMed] [Google Scholar]
- Bailey G. S., Banks B. E., Carstairs J. R., Edward D. C., Pearce F. L., Vernon C. A. Immunological properties of nerve growth factors. Biochim Biophys Acta. 1976 Jun 23;437(1):259–263. doi: 10.1016/0304-4165(76)90368-8. [DOI] [PubMed] [Google Scholar]
- Barth L. G., Barth L. J. Ionic regulation of embryonic induction and cell differentiation in Rana pipiens. Dev Biol. 1974 Jul;39(1):1–22. doi: 10.1016/s0012-1606(74)80004-7. [DOI] [PubMed] [Google Scholar]
- Barth L. G., Barth L. J. The sodium dependence of embryonic induction. Dev Biol. 1969 Sep;20(3):236–262. doi: 10.1016/0012-1606(69)90014-1. [DOI] [PubMed] [Google Scholar]
- Blackshaw S. E., Warner A. E. Alterations in resting membrane properties during neural plate stages of development of the nervous system. J Physiol. 1976 Feb;255(1):231–247. doi: 10.1113/jphysiol.1976.sp011277. [DOI] [PMC free article] [PubMed] [Google Scholar]
- Blackshaw S. E., Warner A. E. Low resistance junctions between mesoderm cells during development of trunk muscles. J Physiol. 1976 Feb;255(1):209–230. doi: 10.1113/jphysiol.1976.sp011276. [DOI] [PMC free article] [PubMed] [Google Scholar]
- Chamley J. H., Campbell G. R., Burnstock G. Dedifferentiation, redifferentiation and bundle formation of smooth muscle cells in tissue culture: the influence of cell number and nerve fibres. J Embryol Exp Morphol. 1974 Oct;32(2):297–323. [PubMed] [Google Scholar]
- GLYNN I. M. The action of cardiac glycosides on sodium and potassium movements in human red cells. J Physiol. 1957 Apr 3;136(1):148–173. doi: 10.1113/jphysiol.1957.sp005749. [DOI] [PMC free article] [PubMed] [Google Scholar]
- Hamilton L. The formation of somites in Xenopus. J Embryol Exp Morphol. 1969 Sep;22(2):253–264. [PubMed] [Google Scholar]
- Klodos I., Ottolenghi P. Large-scale preparation of Na, K-ATPase from ox brain. Anal Biochem. 1975 Aug;67(2):397–403. doi: 10.1016/0003-2697(75)90311-5. [DOI] [PubMed] [Google Scholar]
- Levi-Montalcini R., Booker B. DESTRUCTION OF THE SYMPATHETIC GANGLIA IN MAMMALS BY AN ANTISERUM TO A NERVE-GROWTH PROTEIN. Proc Natl Acad Sci U S A. 1960 Mar;46(3):384–391. doi: 10.1073/pnas.46.3.384. [DOI] [PMC free article] [PubMed] [Google Scholar]
- Messenger E. A., Warner A. E. The action of melatonin on single amphibian pigment cells in tissue culture. Br J Pharmacol. 1977 Dec;61(4):607–614. doi: 10.1111/j.1476-5381.1977.tb07554.x. [DOI] [PMC free article] [PubMed] [Google Scholar]
- Messenger E. A., Warner A. E. The effect of inhibiting the sodium pump on the differentiation of nerve cells [proceedings]. J Physiol. 1976 Dec;263(1):211P–212P. [PubMed] [Google Scholar]
- Muntz L. Myogenesis in the trunk and leg during development of the tadpole of Xenopus laevis (Daudin 1802). J Embryol Exp Morphol. 1975 Jun;33(3):757–774. [PubMed] [Google Scholar]
- Rose B., Loewenstein W. R. Permeability of cell junction depends on local cytoplasmic calcium activity. Nature. 1975 Mar 20;254(5497):250–252. doi: 10.1038/254250a0. [DOI] [PubMed] [Google Scholar]
- SCHATZMANN H. J. Herzglykoside als Hemmstoffe für den aktiven Kalium- und Natriumtransport durch die Erythrocytenmembran. Helv Physiol Pharmacol Acta. 1953;11(4):346–354. [PubMed] [Google Scholar]
- Sachs J. R. Interaction of external K, Na, and cardioactive steroids with the Na-K pump of the human red blood cell. J Gen Physiol. 1974 Feb;63(2):123–143. doi: 10.1085/jgp.63.2.123. [DOI] [PMC free article] [PubMed] [Google Scholar]
- Slack C., Warner A. E., Warren R. L. The distribution of sodium and potassium in amphibian embryos during early development. J Physiol. 1973 Jul;232(2):297–312. doi: 10.1113/jphysiol.1973.sp010271. [DOI] [PMC free article] [PubMed] [Google Scholar]
- Spitzer N. C., Lamborghini J. E. The development of the action potential mechanism of amphibian neurons isolated in culture. Proc Natl Acad Sci U S A. 1976 May;73(5):1641–1645. doi: 10.1073/pnas.73.5.1641. [DOI] [PMC free article] [PubMed] [Google Scholar]
- Warner A. E. The electrical properties of the ectoderm in the amphibian embryo during induction and early development of the nervous system. J Physiol. 1973 Nov;235(1):267–286. doi: 10.1113/jphysiol.1973.sp010387. [DOI] [PMC free article] [PubMed] [Google Scholar]
- Weingart R. The actions of ouabain on intercellular coupling and conduction velocity in mammalian ventricular muscle. J Physiol. 1977 Jan;264(2):341–365. doi: 10.1113/jphysiol.1977.sp011672. [DOI] [PMC free article] [PubMed] [Google Scholar]