Abstract
Theoretical simulations have suggested that interstitial potential (Vis) during action potential propagation affects measurements of the transmembrane action potential in bathed ventricular muscle. To evaluate the Vis experimentally, we obtained Vis and intracellular action potential (Vic) recordings at various depths in paced guinea pig papillary muscles bathed in oxygenated Tyrode's solution. The peak-to-peak amplitude and the maximum dV/dt (dV/dtmax) of the intrinsic downward deflection of the Vis recordings were determined. The transmembrane action potential (TM) was obtained by subtracting each Vis from the corresponding Vic recording, and measurements for the phase zero depolarization and action potential foot of the Vic were compared with the measurements for the TM. At penetration depths of approximately 54 microns, the amplitude and dV/dtmax of the Vis were 13 mV and -38 V/s. When the depth was increased to 200 microns, these parameters increased to 24 mV and -59 V/s (P less than 0.005), and when the depth was further increased to 390 microns, the parameters decreased to 16 mV and -38 V/s. Because of the Vis at the various depths, the Vic underestimated dV/dtmax of phase zero of the TM by 20-31%, which would reduce estimates of Na+ current obtained from dV/dt. Also, the Vic overestimated the time constant of the 2-8 mV foot of the action potential by 48-82%, which would reduce estimates of the "effective" membrane capacitance by 33-45%. These influences of the Vis on measurements may affect results of quantitative studies of the ventricular action potential.
Full text
PDF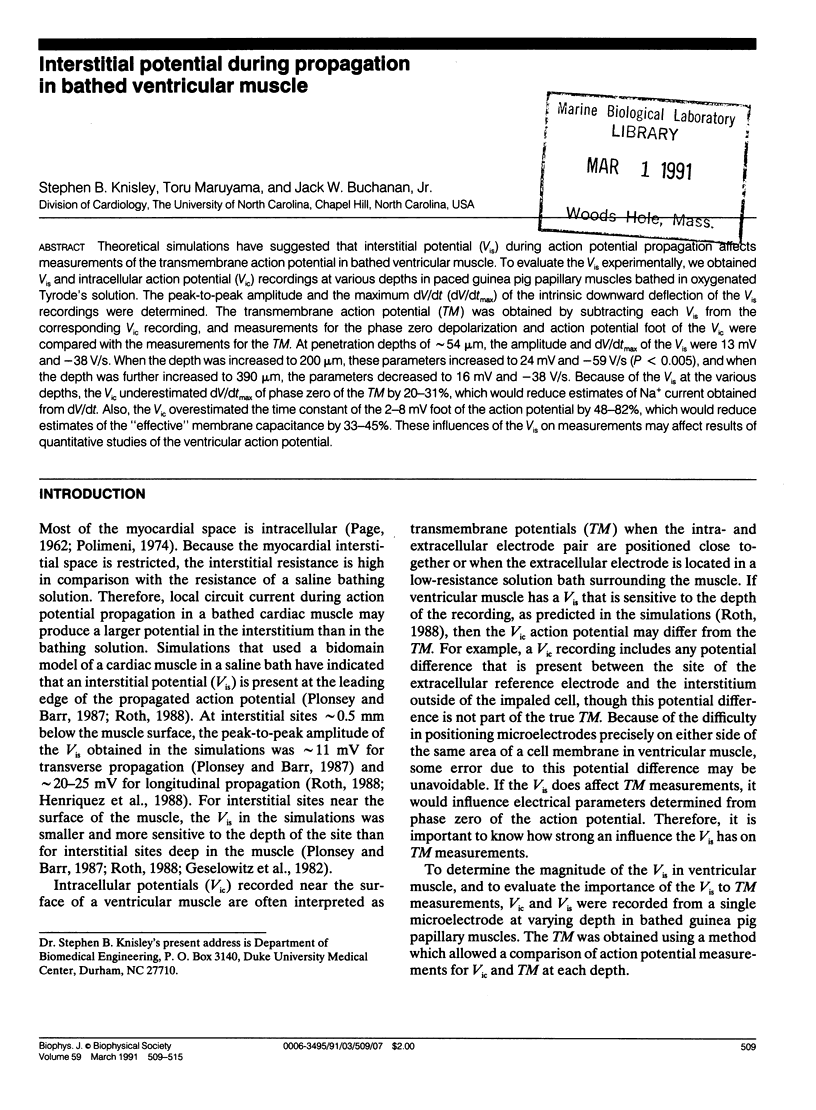
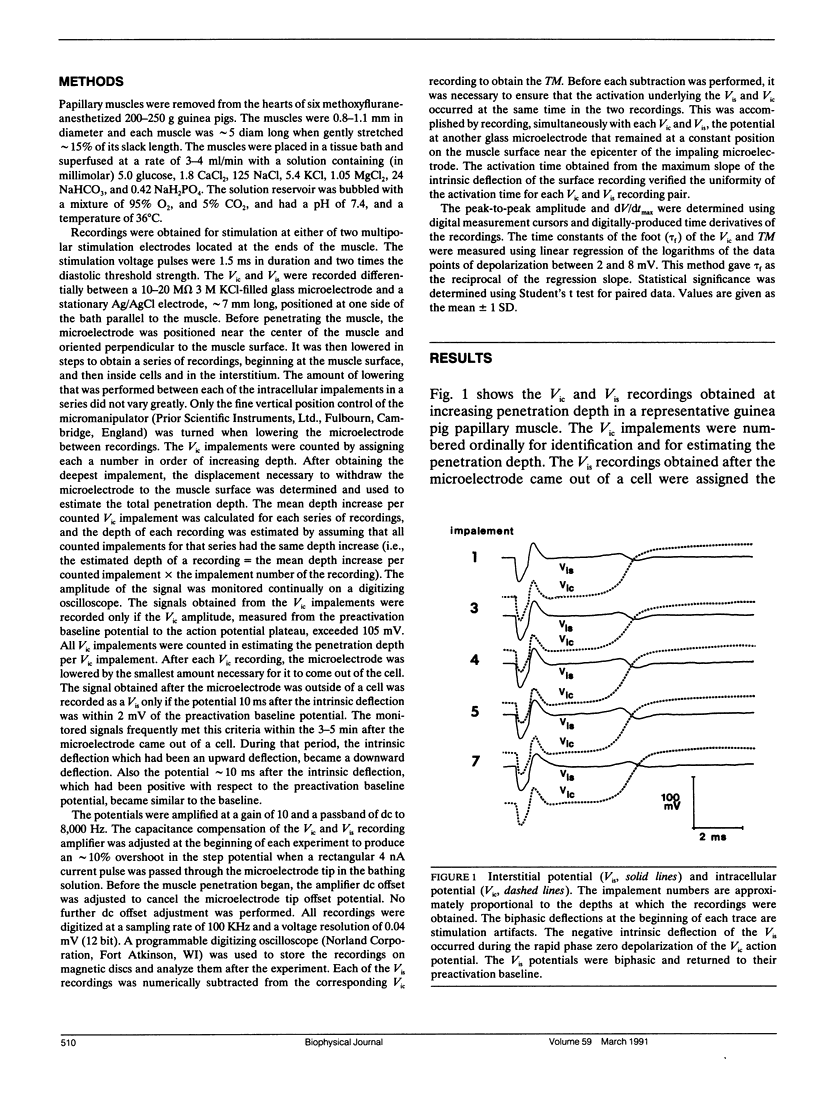
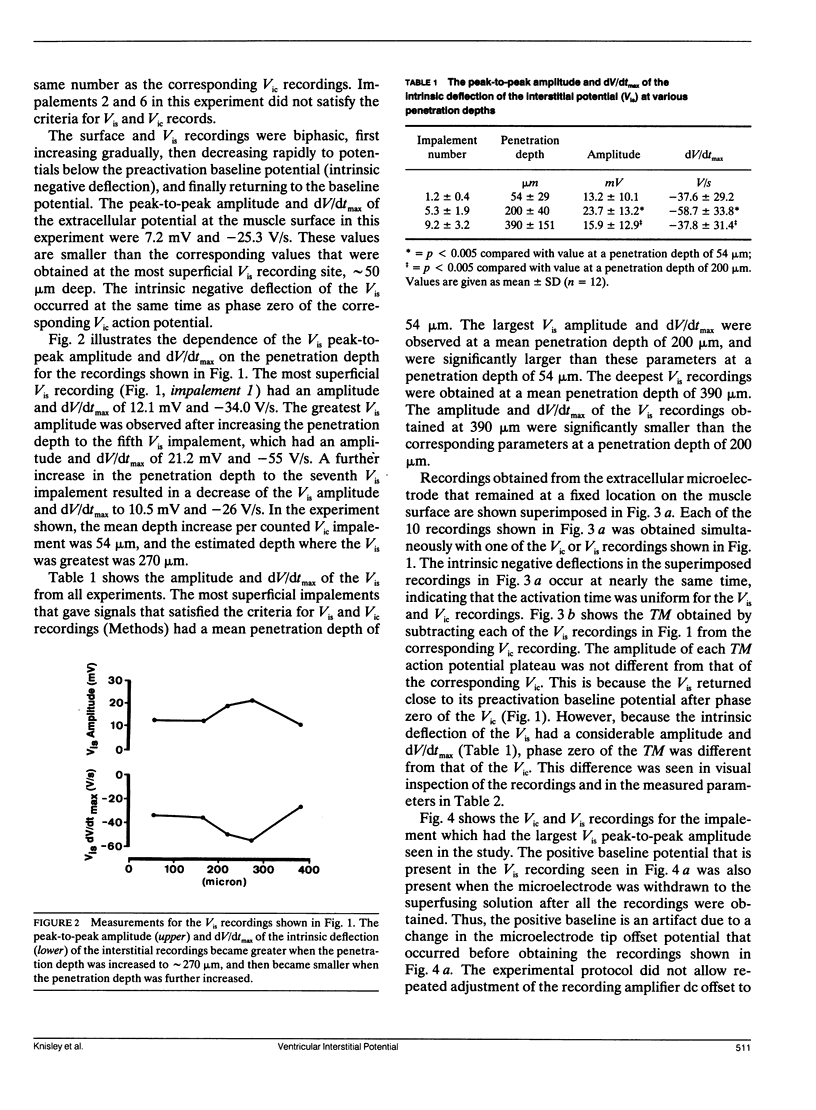
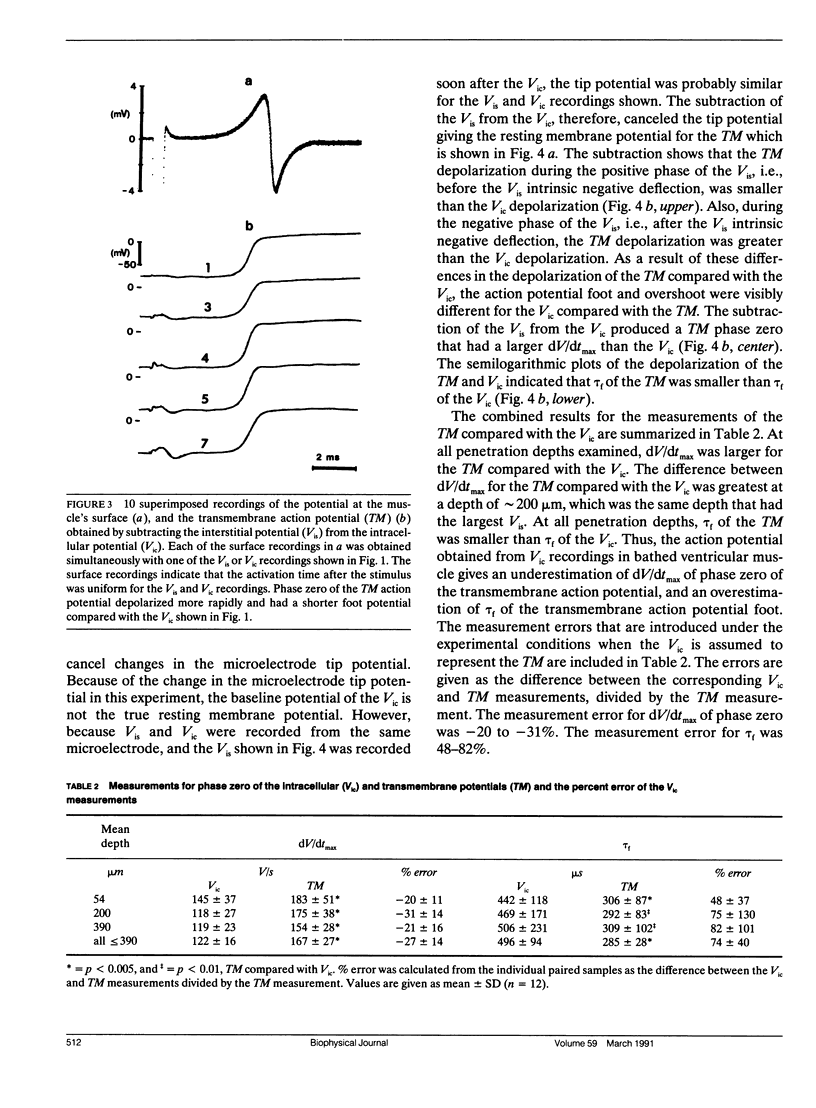
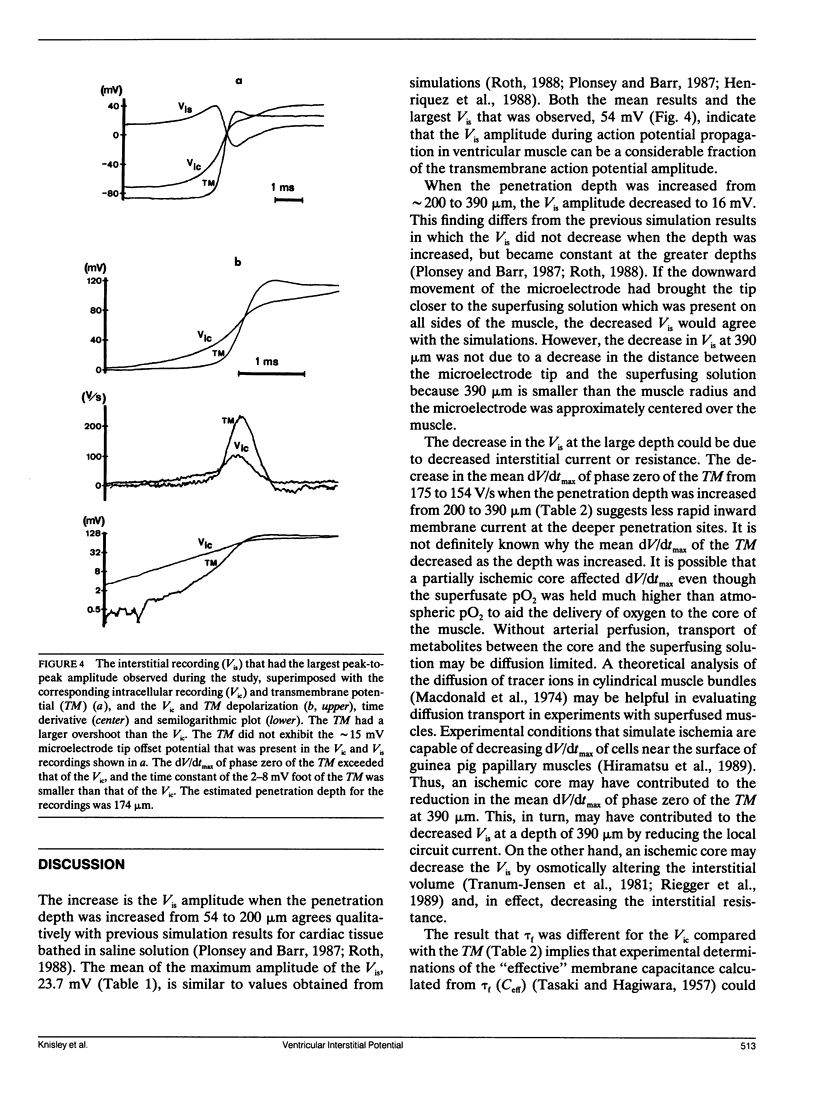
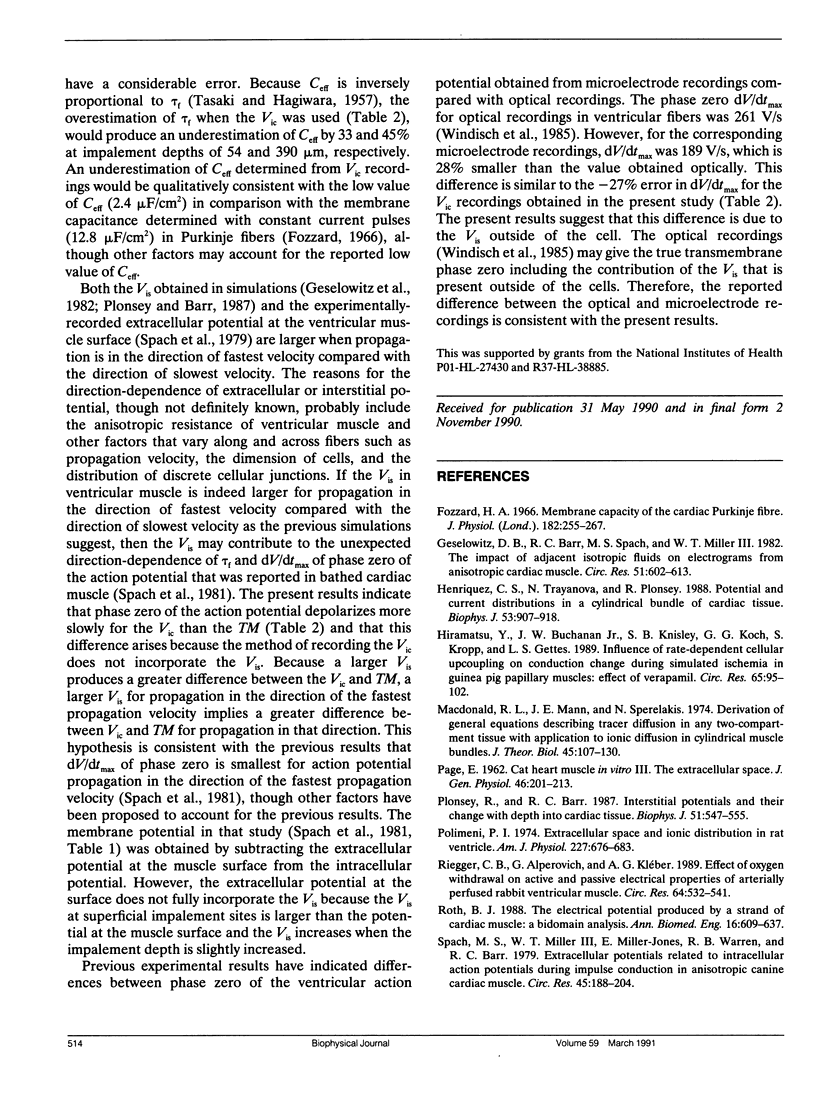
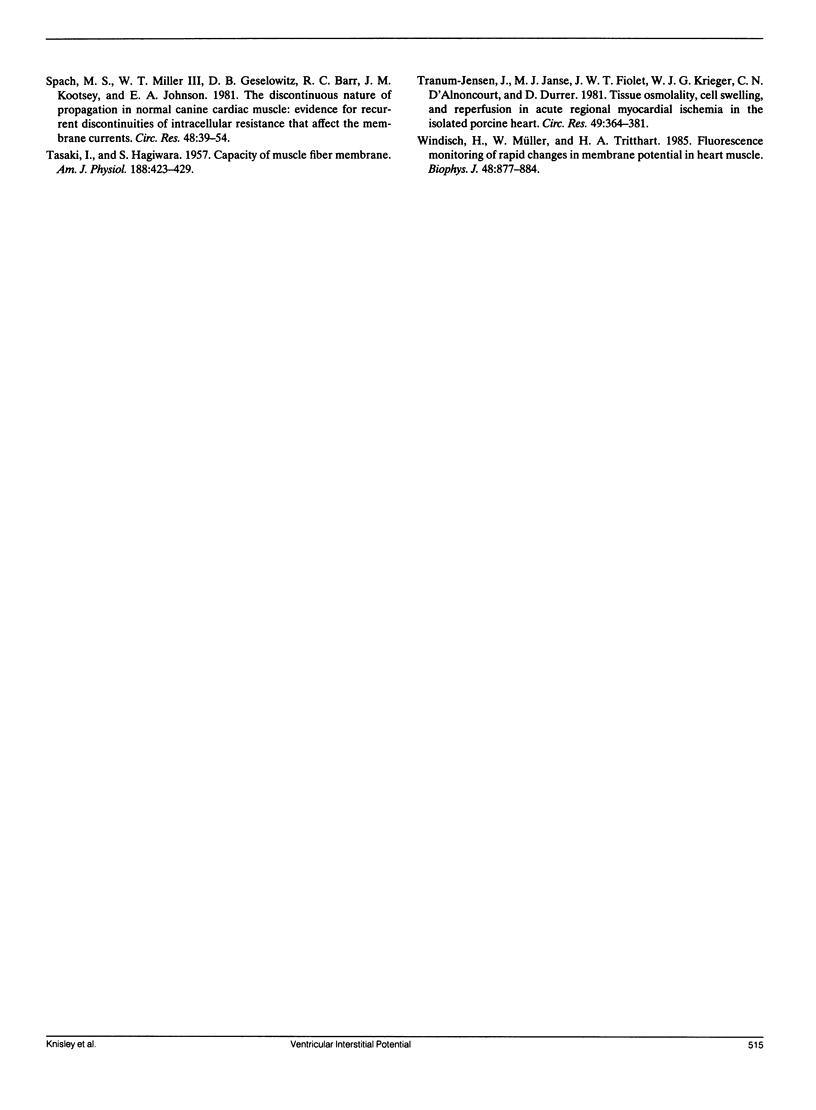
Selected References
These references are in PubMed. This may not be the complete list of references from this article.
- Fozzard H. A. Membrane capacity of the cardiac Purkinje fibre. J Physiol. 1966 Jan;182(2):255–267. doi: 10.1113/jphysiol.1966.sp007823. [DOI] [PMC free article] [PubMed] [Google Scholar]
- Geselowitz D. B., Barr R. C., Spach M. S., Miller W. T., 3rd The impact of adjacent isotropic fluids on electrograms from anisotropic cardiac muscle. A modeling study. Circ Res. 1982 Nov;51(5):602–613. doi: 10.1161/01.res.51.5.602. [DOI] [PubMed] [Google Scholar]
- Henriquez C. S., Trayanova N., Plonsey R. Potential and current distributions in a cylindrical bundle of cardiac tissue. Biophys J. 1988 Jun;53(6):907–918. doi: 10.1016/S0006-3495(88)83172-2. [DOI] [PMC free article] [PubMed] [Google Scholar]
- Hiramatsu Y., Buchanan J. W., Jr, Knisley S. B., Koch G. G., Kropp S., Gettes L. S. Influence of rate-dependent cellular uncoupling on conduction change during simulated ischemia in guinea pig papillary muscles: effect of verapamil. Circ Res. 1989 Jul;65(1):95–102. doi: 10.1161/01.res.65.1.95. [DOI] [PubMed] [Google Scholar]
- Macdonald R. L., Mann J. E., Jr, Sperelakis N. Derivation of general equations describing tracer diffusion in any two-compartment tissue with application to ionic diffusion in cylindrical muscle bundles. J Theor Biol. 1974 May;45(1):107–130. doi: 10.1016/0022-5193(74)90046-0. [DOI] [PubMed] [Google Scholar]
- PAGE E. Cat heart muscle in vitro. III. The extracellular space. J Gen Physiol. 1962 Nov;46:201–213. doi: 10.1085/jgp.46.2.201. [DOI] [PMC free article] [PubMed] [Google Scholar]
- Plonsey R., Barr R. C. Interstitial potentials and their change with depth into cardiac tissue. Biophys J. 1987 Apr;51(4):547–555. doi: 10.1016/S0006-3495(87)83380-5. [DOI] [PMC free article] [PubMed] [Google Scholar]
- Polimeni P. I. Extracellular space and ionic distribution in rat ventricle. Am J Physiol. 1974 Sep;227(3):676–683. doi: 10.1152/ajplegacy.1974.227.3.676. [DOI] [PubMed] [Google Scholar]
- Riegger C. B., Alperovich G., Kléber A. G. Effect of oxygen withdrawal on active and passive electrical properties of arterially perfused rabbit ventricular muscle. Circ Res. 1989 Mar;64(3):532–541. doi: 10.1161/01.res.64.3.532. [DOI] [PubMed] [Google Scholar]
- Roth B. J. The electrical potential produced by a strand of cardiac muscle: a bidomain analysis. Ann Biomed Eng. 1988;16(6):609–637. doi: 10.1007/BF02368018. [DOI] [PubMed] [Google Scholar]
- Spach M. S., Miller W. T., 3rd, Geselowitz D. B., Barr R. C., Kootsey J. M., Johnson E. A. The discontinuous nature of propagation in normal canine cardiac muscle. Evidence for recurrent discontinuities of intracellular resistance that affect the membrane currents. Circ Res. 1981 Jan;48(1):39–54. doi: 10.1161/01.res.48.1.39. [DOI] [PubMed] [Google Scholar]
- Spach M. S., Miller W. T., 3rd, Miller-Jones E., Warren R. B., Barr R. C. Extracellular potentials related to intracellular action potentials during impulse conduction in anisotropic canine cardiac muscle. Circ Res. 1979 Aug;45(2):188–204. doi: 10.1161/01.res.45.2.188. [DOI] [PubMed] [Google Scholar]
- TASAKI I., HAGIWARA S. Capacity of muscle fiber membrane. Am J Physiol. 1957 Mar;188(3):423–429. doi: 10.1152/ajplegacy.1957.188.3.423. [DOI] [PubMed] [Google Scholar]
- Tranum-Jensen J., Janse M. J., Fiolet W. T., Krieger W. J., D'Alnoncourt C. N., Durrer D. Tissue osmolality, cell swelling, and reperfusion in acute regional myocardial ischemia in the isolated porcine heart. Circ Res. 1981 Aug;49(2):364–381. doi: 10.1161/01.res.49.2.364. [DOI] [PubMed] [Google Scholar]
- Windisch H., Müller W., Tritthart H. A. Fluorescence monitoring of rapid changes in membrane potential in heart muscle. Biophys J. 1985 Dec;48(6):877–884. doi: 10.1016/S0006-3495(85)83849-2. [DOI] [PMC free article] [PubMed] [Google Scholar]