Abstract
Pancreatic beta-cells coupled by gap junctions in sufficiently large clusters exhibit regular electrical bursting activity, which is described by the Chay-Keizer model and its variants. According to most reports, however, isolated cells exhibit disorganized spiking. We have previously (Sherman, A. J. Rinzel, and J. Keizer, 1988. Biophys. J. 54:411-425) modeled these behaviors by hypothesizing that stochastic channel fluctuations disrupt the bursts. We showed that when cells are coupled by infinite conductance gap junctions, so that the cluster is isopotential and may be viewed as a single "supercell," the fluctuations are shared over a larger membrane area and hence dampened. Bursting emerges when there are more than approximately 50 cells in the cluster. In the model the temporal organization of spikes into bursts increases the amplitude of intracellular calcium oscillations, which may be relevant for insulin secretion. We now extend the previous work by considering the case of a true "multicell" model with finite gap junctional conductance. Whereas the previous study assumed that the cells were synchronized, we can now study the process of synchronization itself. We show that, for sufficiently large clusters, the cells both synchronize and begin to burst with moderate, physiologically reasonable gap junctional conductance. An unexpected finding is that the burst period is longer, and calcium amplitude greater, than when coupling is infinitely strong, with an optimum in the range of 150-250 pS. Our model is in good agreement with recent experimental data of Perez-Armendariz, M., D. C. Spray, and M. V. L. Bennett. (1991. Biophys. J. 59:76-92) showing extensive gap junctions in beta-cell pairs with mean interfacial conductance of 213 +/- 113 pS. The optimality property of our model is noteworthy because simple slow-wave models without spikes do not show the same behavior.
Full text
PDF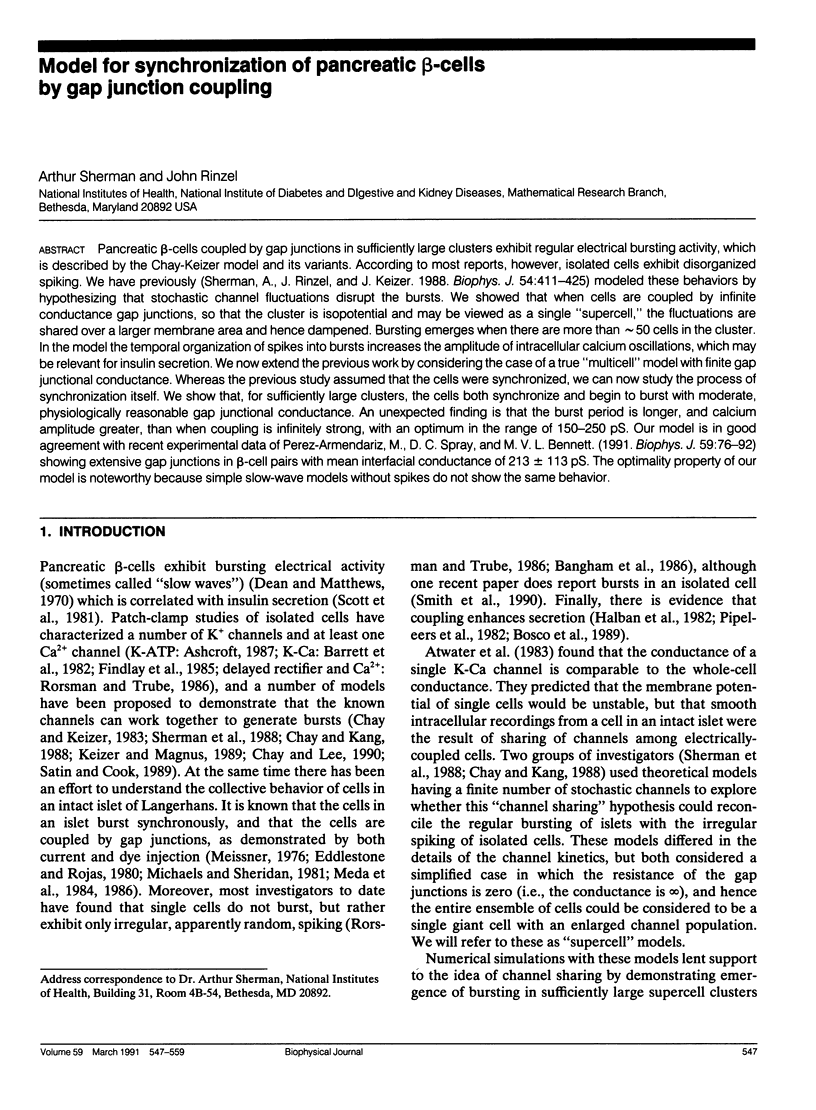
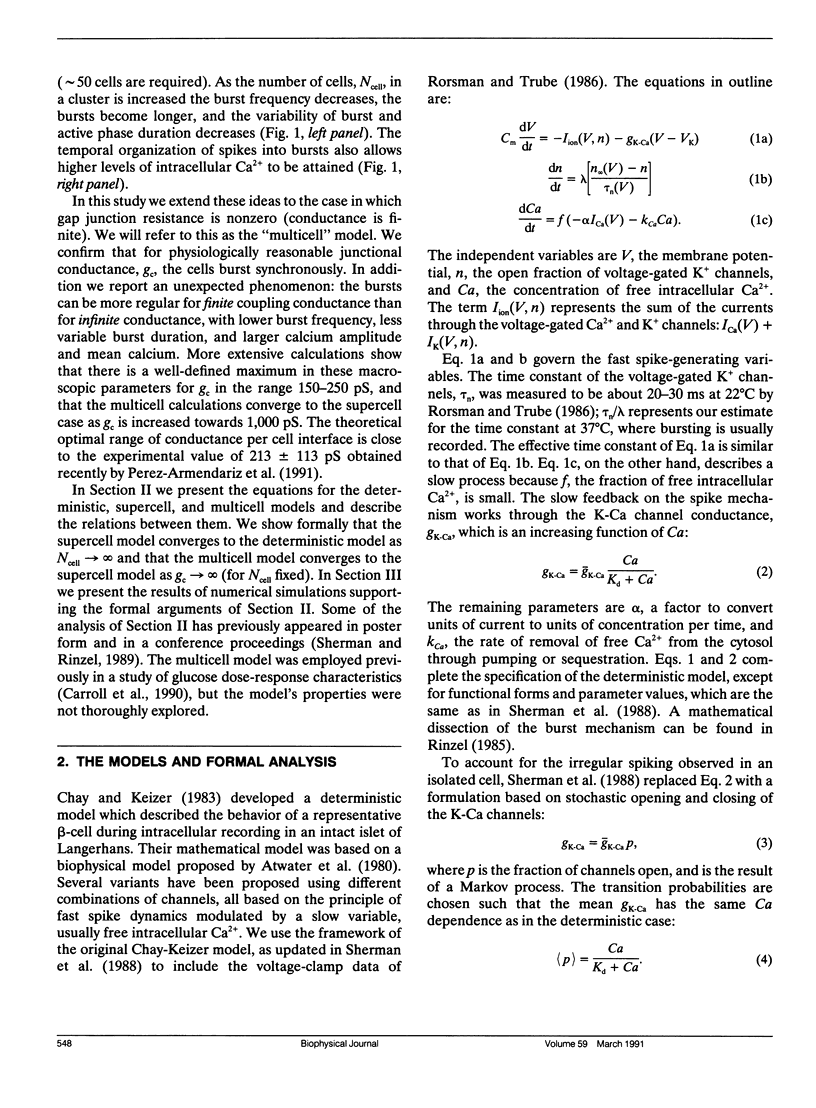
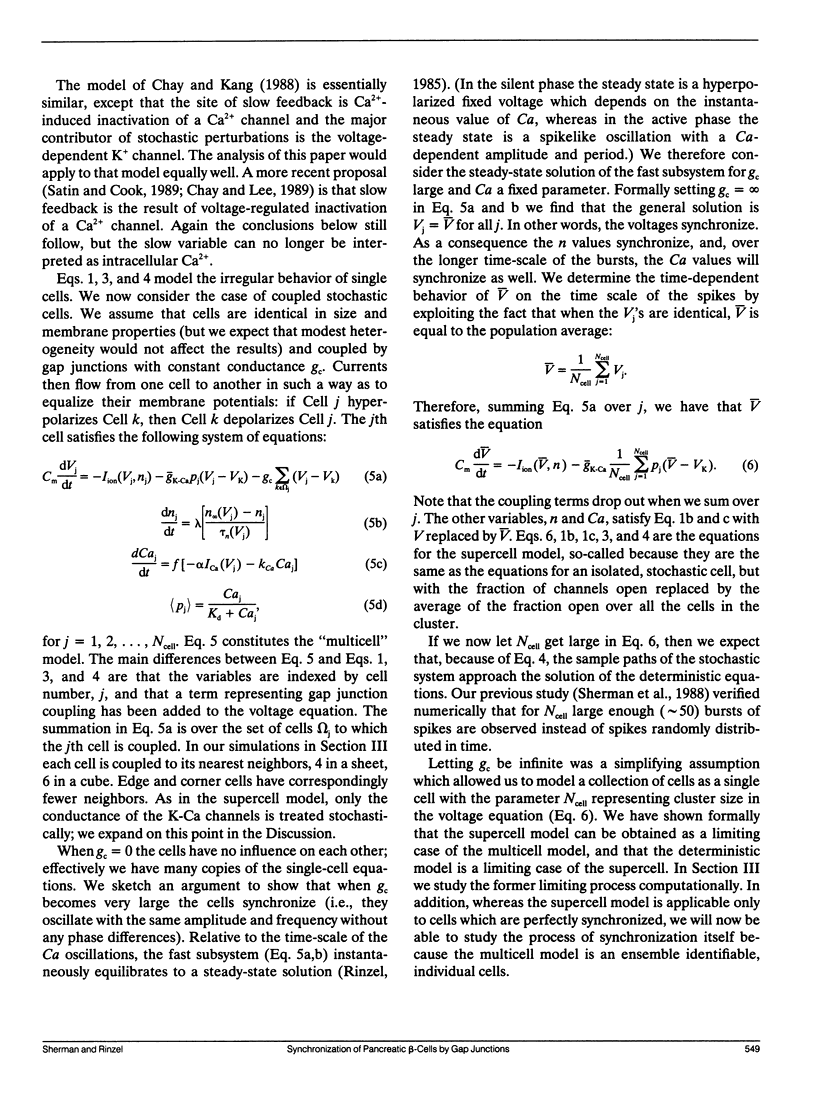
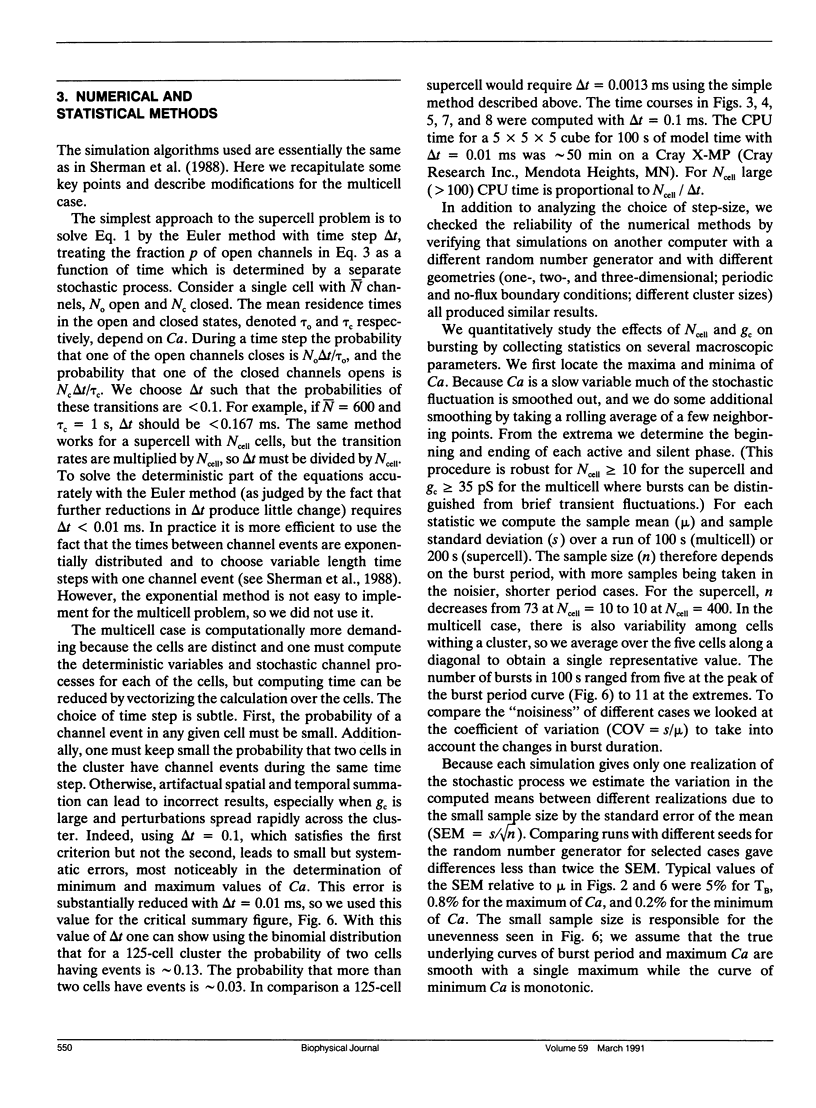
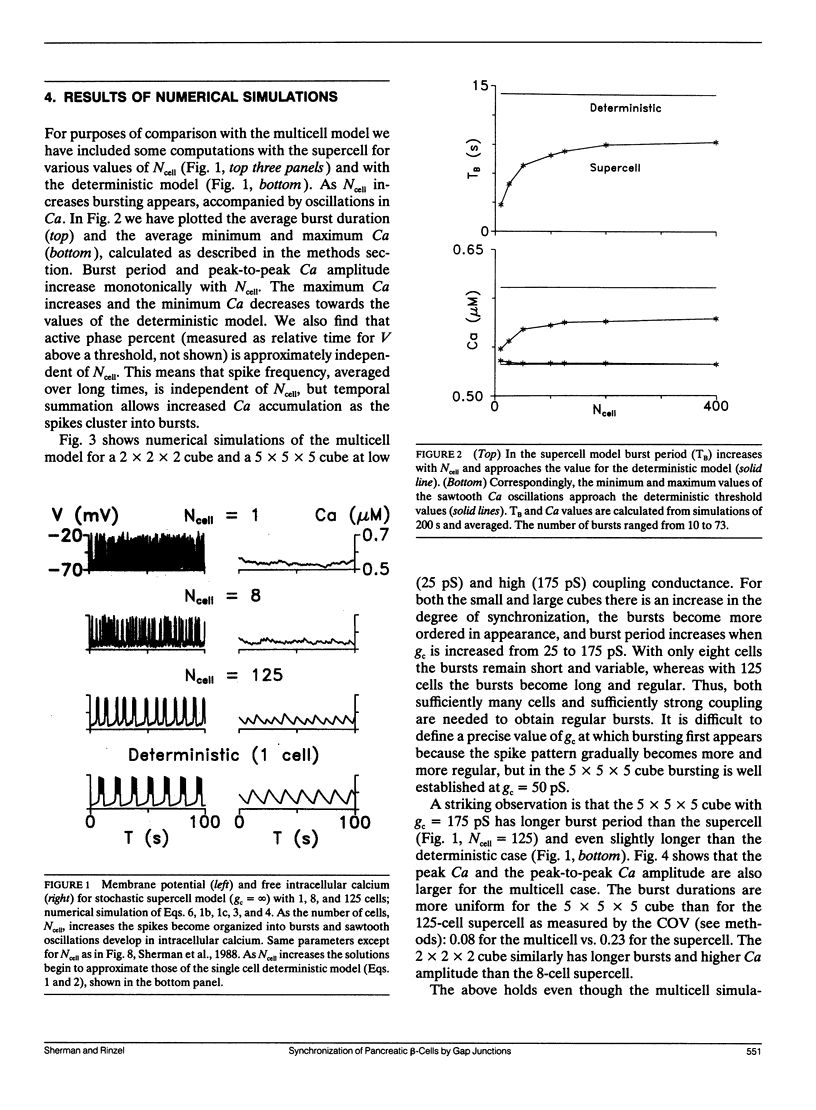
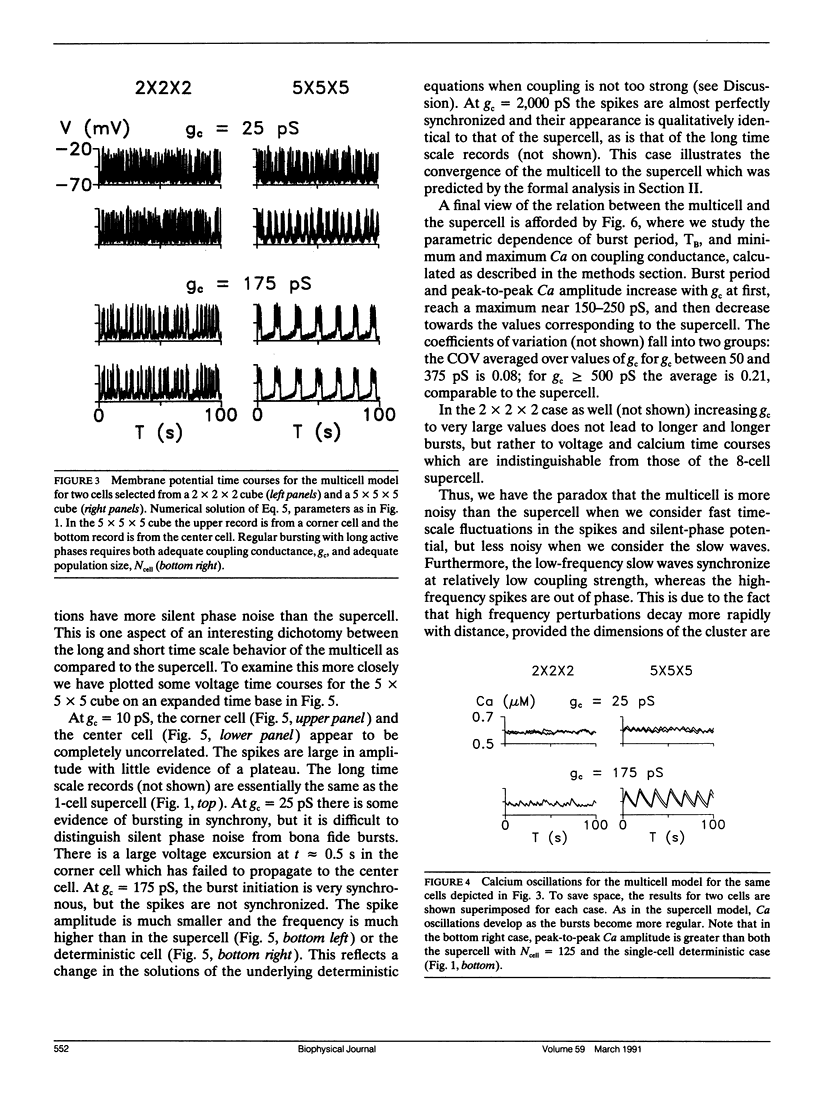
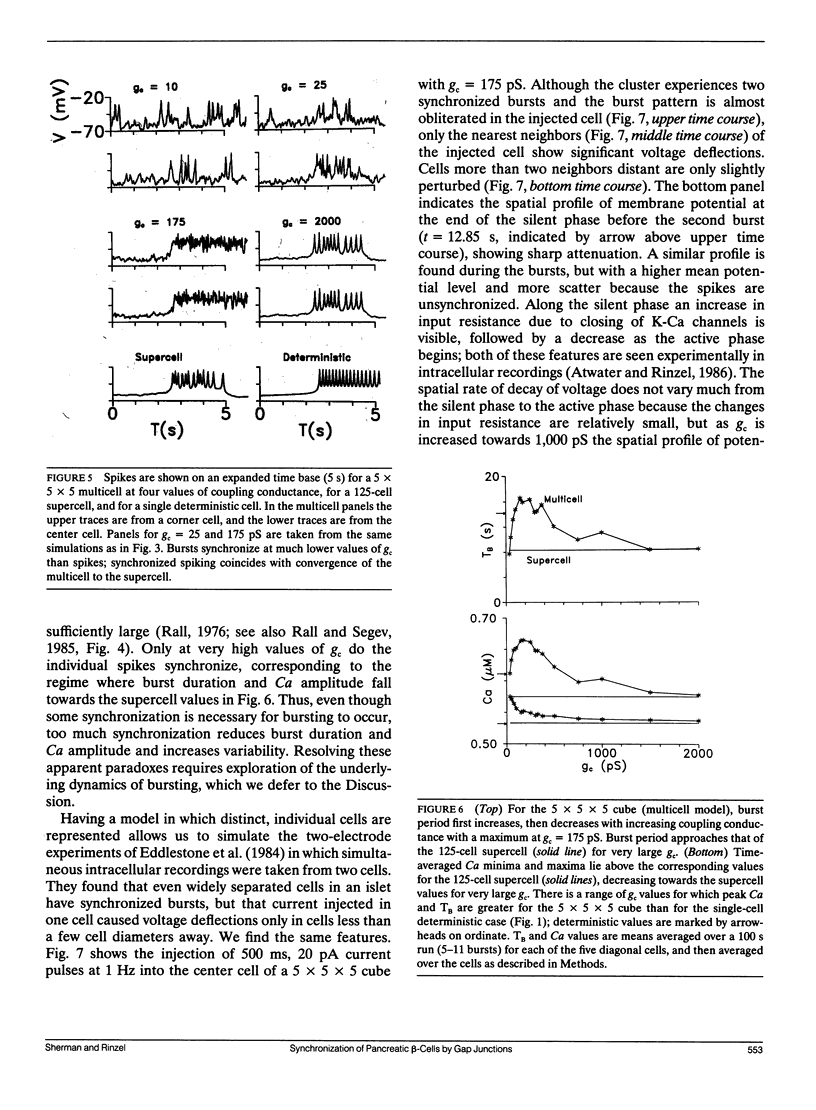
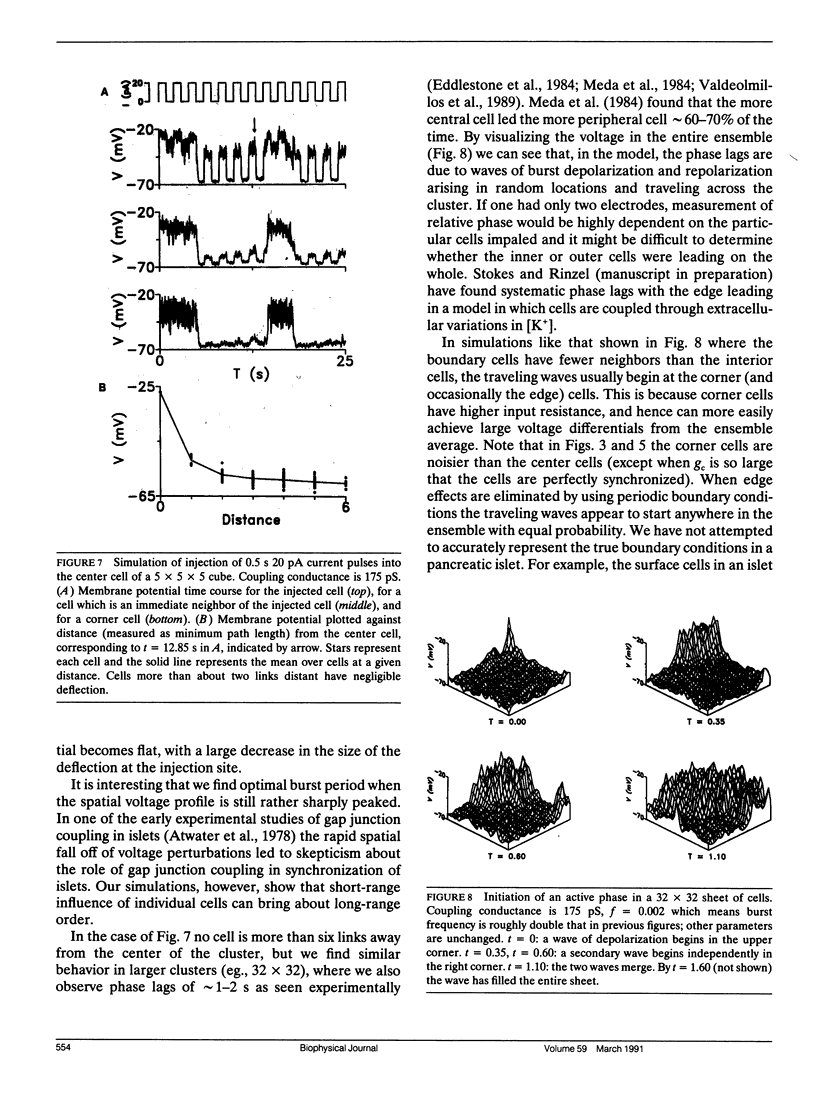
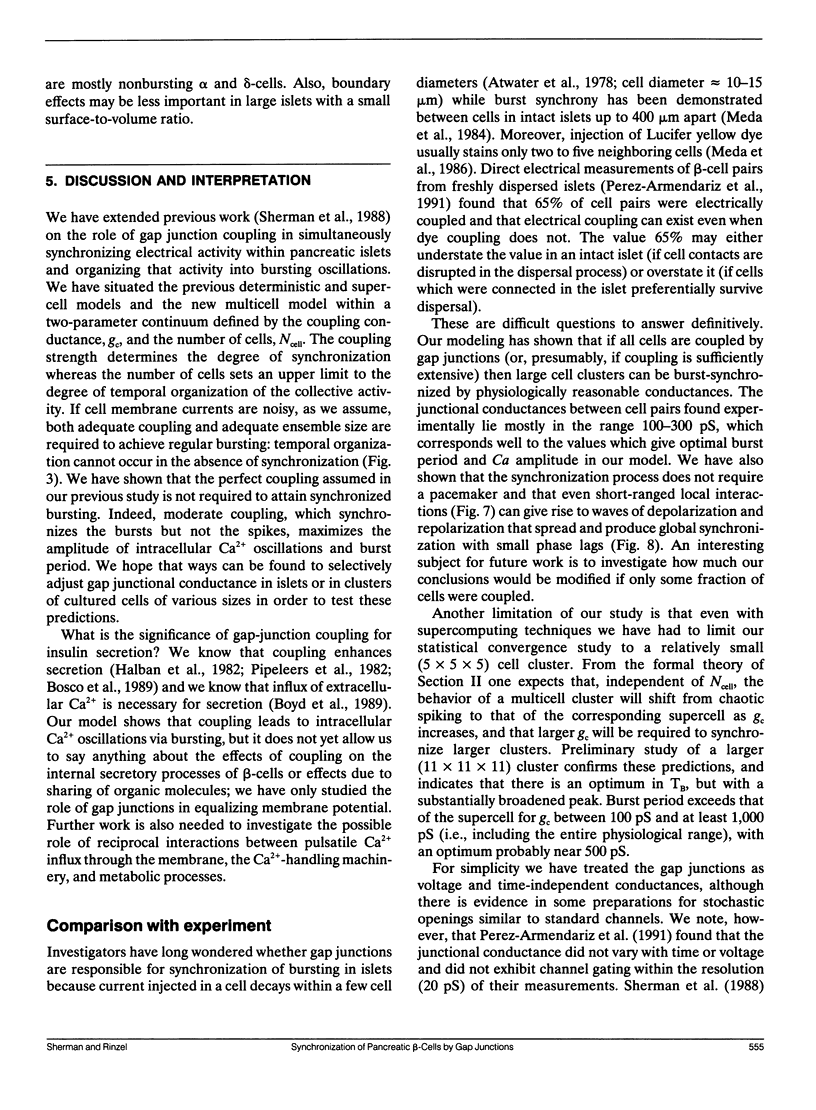
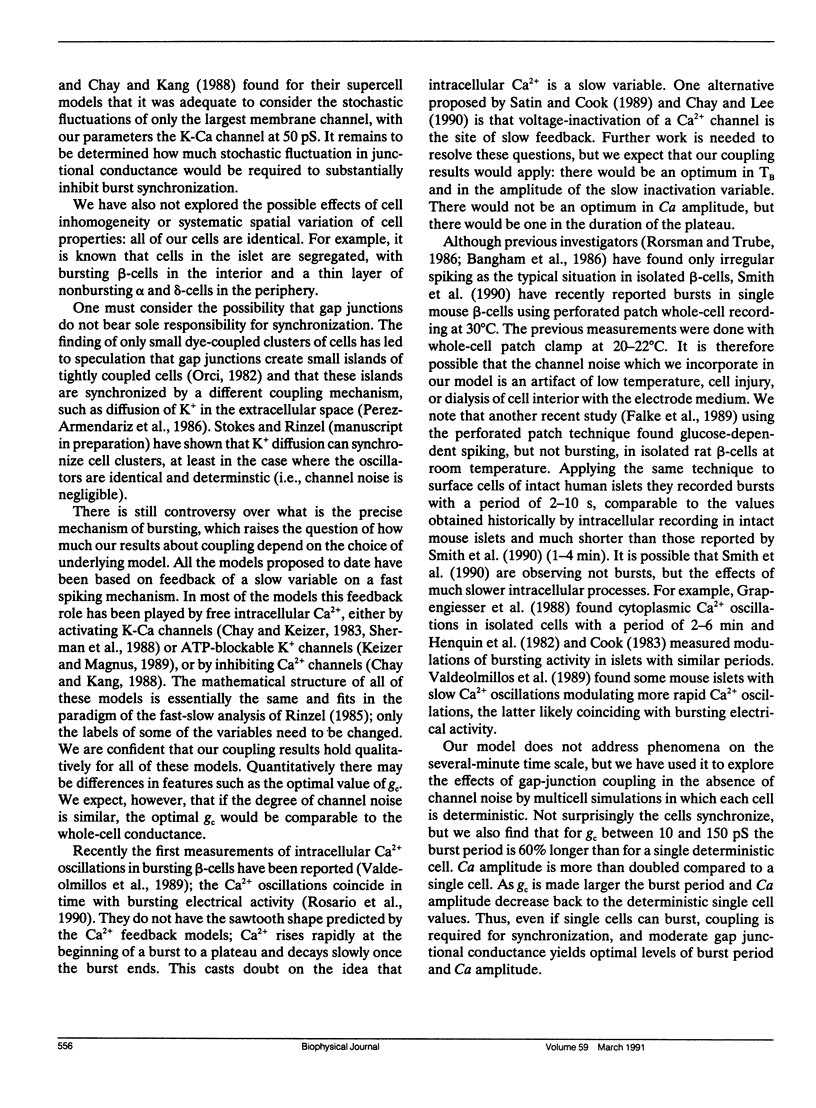
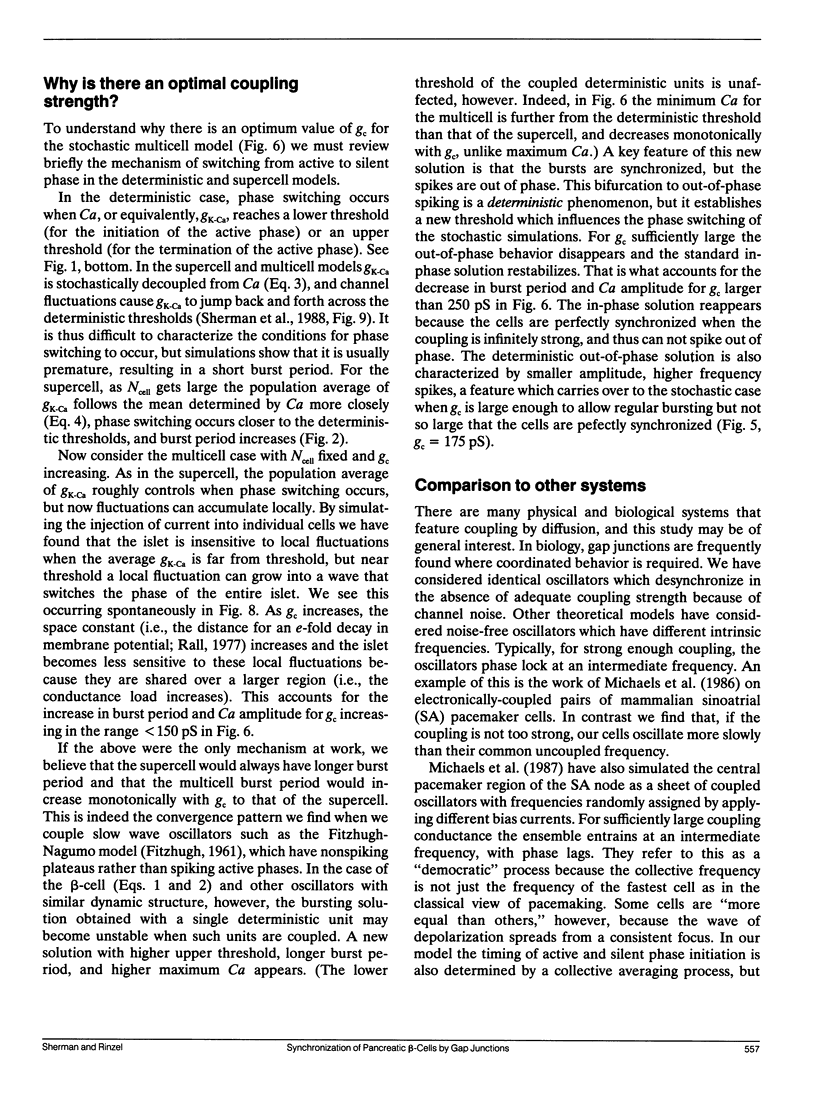
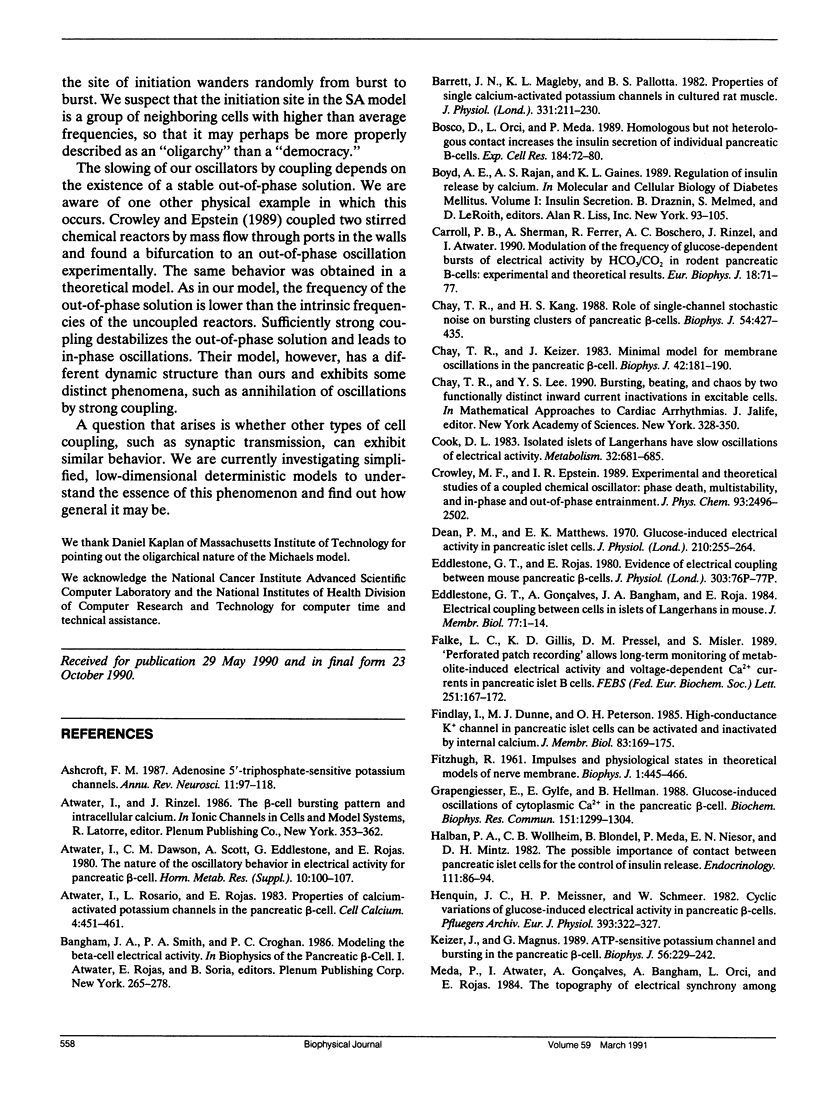
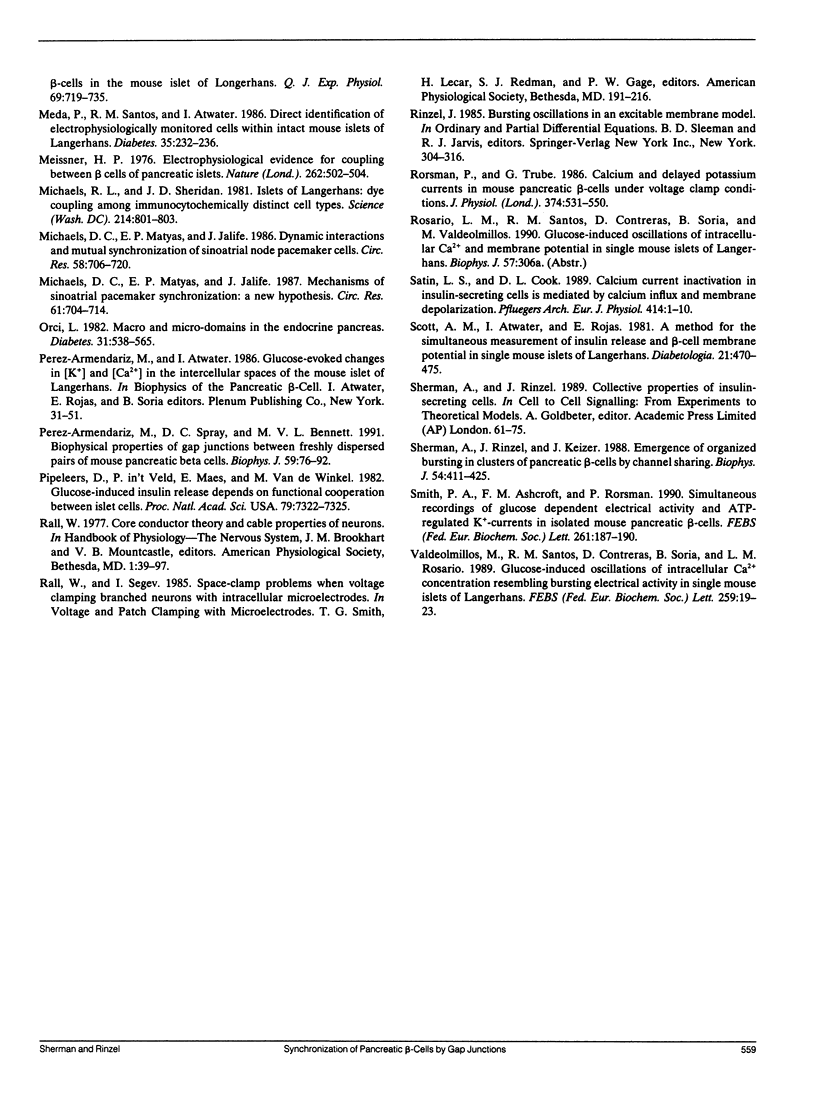
Selected References
These references are in PubMed. This may not be the complete list of references from this article.
- Ashcroft F. M. Adenosine 5'-triphosphate-sensitive potassium channels. Annu Rev Neurosci. 1988;11:97–118. doi: 10.1146/annurev.ne.11.030188.000525. [DOI] [PubMed] [Google Scholar]
- Atwater I., Dawson C. M., Scott A., Eddlestone G., Rojas E. The nature of the oscillatory behaviour in electrical activity from pancreatic beta-cell. Horm Metab Res Suppl. 1980;Suppl 10:100–107. [PubMed] [Google Scholar]
- Atwater I., Rosario L., Rojas E. Properties of the Ca-activated K+ channel in pancreatic beta-cells. Cell Calcium. 1983 Dec;4(5-6):451–461. doi: 10.1016/0143-4160(83)90021-0. [DOI] [PubMed] [Google Scholar]
- Bangham J. A., Smith P. A., Croghan P. C. Modelling the beta-cell electrical activity. Adv Exp Med Biol. 1986;211:265–278. doi: 10.1007/978-1-4684-5314-0_24. [DOI] [PubMed] [Google Scholar]
- Barrett J. N., Magleby K. L., Pallotta B. S. Properties of single calcium-activated potassium channels in cultured rat muscle. J Physiol. 1982 Oct;331:211–230. doi: 10.1113/jphysiol.1982.sp014370. [DOI] [PMC free article] [PubMed] [Google Scholar]
- Bosco D., Orci L., Meda P. Homologous but not heterologous contact increases the insulin secretion of individual pancreatic B-cells. Exp Cell Res. 1989 Sep;184(1):72–80. doi: 10.1016/0014-4827(89)90365-0. [DOI] [PubMed] [Google Scholar]
- Carroll P. B., Sherman A., Ferrer R., Boschero A. C., Rinzel J., Atwater I. Modulation of the frequency of glucose-dependent bursts of electrical activity by HCO3/CO2 in rodent pancreatic B-cells: experimental and theoretical results. Eur Biophys J. 1990;18(2):71–77. doi: 10.1007/BF00183265. [DOI] [PubMed] [Google Scholar]
- Chay T. R., Kang H. S. Role of single-channel stochastic noise on bursting clusters of pancreatic beta-cells. Biophys J. 1988 Sep;54(3):427–435. doi: 10.1016/S0006-3495(88)82976-X. [DOI] [PMC free article] [PubMed] [Google Scholar]
- Chay T. R., Keizer J. Minimal model for membrane oscillations in the pancreatic beta-cell. Biophys J. 1983 May;42(2):181–190. doi: 10.1016/S0006-3495(83)84384-7. [DOI] [PMC free article] [PubMed] [Google Scholar]
- Chay T. R., Lee Y. S. Bursting, beating, and chaos by two functionally distinct inward current inactivations in excitable cells. Ann N Y Acad Sci. 1990;591:328–350. doi: 10.1111/j.1749-6632.1990.tb15099.x. [DOI] [PubMed] [Google Scholar]
- Cook D. L. Isolated islets of Langerhans have slow oscillations of electrical activity. Metabolism. 1983 Jul;32(7):681–685. doi: 10.1016/0026-0495(83)90124-5. [DOI] [PubMed] [Google Scholar]
- Dean P. M., Matthews E. K. Glucose-induced electrical activity in pancreatic islet cells. J Physiol. 1970 Sep;210(2):255–264. doi: 10.1113/jphysiol.1970.sp009207. [DOI] [PMC free article] [PubMed] [Google Scholar]
- Eddlestone G. T., Gonçalves A., Bangham J. A., Rojas E. Electrical coupling between cells in islets of Langerhans from mouse. J Membr Biol. 1984;77(1):1–14. doi: 10.1007/BF01871095. [DOI] [PubMed] [Google Scholar]
- Falke L. C., Gillis K. D., Pressel D. M., Misler S. 'Perforated patch recording' allows long-term monitoring of metabolite-induced electrical activity and voltage-dependent Ca2+ currents in pancreatic islet B cells. FEBS Lett. 1989 Jul 17;251(1-2):167–172. doi: 10.1016/0014-5793(89)81448-6. [DOI] [PubMed] [Google Scholar]
- Findlay I., Dunne M. J., Petersen O. H. High-conductance K+ channel in pancreatic islet cells can be activated and inactivated by internal calcium. J Membr Biol. 1985;83(1-2):169–175. doi: 10.1007/BF01868748. [DOI] [PubMed] [Google Scholar]
- Fitzhugh R. Impulses and Physiological States in Theoretical Models of Nerve Membrane. Biophys J. 1961 Jul;1(6):445–466. doi: 10.1016/s0006-3495(61)86902-6. [DOI] [PMC free article] [PubMed] [Google Scholar]
- Grapengiesser E., Gylfe E., Hellman B. Glucose-induced oscillations of cytoplasmic Ca2+ in the pancreatic beta-cell. Biochem Biophys Res Commun. 1988 Mar 30;151(3):1299–1304. doi: 10.1016/s0006-291x(88)80503-5. [DOI] [PubMed] [Google Scholar]
- Halban P. A., Wollheim C. B., Blondel B., Meda P., Niesor E. N., Mintz D. H. The possible importance of contact between pancreatic islet cells for the control of insulin release. Endocrinology. 1982 Jul;111(1):86–94. doi: 10.1210/endo-111-1-86. [DOI] [PubMed] [Google Scholar]
- Henquin J. C., Meissner H. P., Schmeer W. Cyclic variations of glucose-induced electrical activity in pancreatic B cells. Pflugers Arch. 1982 Jun;393(4):322–327. doi: 10.1007/BF00581418. [DOI] [PubMed] [Google Scholar]
- Keizer J., Magnus G. ATP-sensitive potassium channel and bursting in the pancreatic beta cell. A theoretical study. Biophys J. 1989 Aug;56(2):229–242. doi: 10.1016/S0006-3495(89)82669-4. [DOI] [PMC free article] [PubMed] [Google Scholar]
- Meda P., Atwater I., Gonçalves A., Bangham A., Orci L., Rojas E. The topography of electrical synchrony among beta-cells in the mouse islet of Langerhans. Q J Exp Physiol. 1984 Oct;69(4):719–735. [PubMed] [Google Scholar]
- Meda P., Santos R. M., Atwater I. Direct identification of electrophysiologically monitored cells within intact mouse islets of Langerhans. Diabetes. 1986 Feb;35(2):232–236. doi: 10.2337/diab.35.2.232. [DOI] [PubMed] [Google Scholar]
- Meissner H. P. Electrophysiological evidence for coupling between beta cells of pancreatic islets. Nature. 1976 Aug 5;262(5568):502–504. doi: 10.1038/262502a0. [DOI] [PubMed] [Google Scholar]
- Michaels D. C., Matyas E. P., Jalife J. Dynamic interactions and mutual synchronization of sinoatrial node pacemaker cells. A mathematical model. Circ Res. 1986 May;58(5):706–720. doi: 10.1161/01.res.58.5.706. [DOI] [PubMed] [Google Scholar]
- Michaels D. C., Matyas E. P., Jalife J. Mechanisms of sinoatrial pacemaker synchronization: a new hypothesis. Circ Res. 1987 Nov;61(5):704–714. doi: 10.1161/01.res.61.5.704. [DOI] [PubMed] [Google Scholar]
- Michaels R. L., Sheridan J. D. Islets of Langerhans: dye coupling among immunocytochemically distinct cell types. Science. 1981 Nov 13;214(4522):801–803. doi: 10.1126/science.6117129. [DOI] [PubMed] [Google Scholar]
- Orci L. Macro- and micro-domains in the endocrine pancreas. Diabetes. 1982 Jun;31(6 Pt 1):538–565. doi: 10.2337/diab.31.6.538. [DOI] [PubMed] [Google Scholar]
- Perez-Armendariz E., Atwater I. Glucose-evoked changes in [K+] and [Ca2+] in the intercellular spaces of the mouse islet of Langerhans. Adv Exp Med Biol. 1986;211:31–51. doi: 10.1007/978-1-4684-5314-0_3. [DOI] [PubMed] [Google Scholar]
- Pipeleers D., in't Veld P. I., Maes E., Van De Winkel M. Glucose-induced insulin release depends on functional cooperation between islet cells. Proc Natl Acad Sci U S A. 1982 Dec;79(23):7322–7325. doi: 10.1073/pnas.79.23.7322. [DOI] [PMC free article] [PubMed] [Google Scholar]
- Pérez-Armendariz M., Roy C., Spray D. C., Bennett M. V. Biophysical properties of gap junctions between freshly dispersed pairs of mouse pancreatic beta cells. Biophys J. 1991 Jan;59(1):76–92. doi: 10.1016/S0006-3495(91)82200-7. [DOI] [PMC free article] [PubMed] [Google Scholar]
- Rorsman P., Trube G. Calcium and delayed potassium currents in mouse pancreatic beta-cells under voltage-clamp conditions. J Physiol. 1986 May;374:531–550. doi: 10.1113/jphysiol.1986.sp016096. [DOI] [PMC free article] [PubMed] [Google Scholar]
- Satin L. S., Cook D. L. Calcium current inactivation in insulin-secreting cells is mediated by calcium influx and membrane depolarization. Pflugers Arch. 1989 May;414(1):1–10. doi: 10.1007/BF00585619. [DOI] [PubMed] [Google Scholar]
- Scott A. M., Atwater I., Rojas E. A method for the simultaneous measurement of insulin release and B cell membrane potential in single mouse islets of Langerhans. Diabetologia. 1981 Nov;21(5):470–475. doi: 10.1007/BF00257788. [DOI] [PubMed] [Google Scholar]
- Sherman A., Rinzel J., Keizer J. Emergence of organized bursting in clusters of pancreatic beta-cells by channel sharing. Biophys J. 1988 Sep;54(3):411–425. doi: 10.1016/S0006-3495(88)82975-8. [DOI] [PMC free article] [PubMed] [Google Scholar]
- Smith P. A., Ashcroft F. M., Rorsman P. Simultaneous recordings of glucose dependent electrical activity and ATP-regulated K(+)-currents in isolated mouse pancreatic beta-cells. FEBS Lett. 1990 Feb 12;261(1):187–190. doi: 10.1016/0014-5793(90)80667-8. [DOI] [PubMed] [Google Scholar]
- Valdeolmillos M., Santos R. M., Contreras D., Soria B., Rosario L. M. Glucose-induced oscillations of intracellular Ca2+ concentration resembling bursting electrical activity in single mouse islets of Langerhans. FEBS Lett. 1989 Dec 18;259(1):19–23. doi: 10.1016/0014-5793(89)81484-x. [DOI] [PubMed] [Google Scholar]