Abstract
Fourier transform infrared studies of active-site-methylated rhodopsin (ASMR) show that, as compared to unmodified rhodopsin, the photoreaction is almost unchanged up to the formation of lumirhodopsin. Especially, the deviations are much smaller than those observed for the corresponding intermediates of 13-desmethyl-rhodopsin. In metarhodopsin-I, larger alterations are present with respect to the three internal carboxyl groups. Similar deviations have been observed in meta-I of 13-desmethyl-rhodopsin. This indicates that, in agreement with our previous investigations, these carboxyl groups are located in close proximity to the chromophore. Because this latter pigment is capable, when bleached, of activating transducin, our data provide support for the earlier conclusion that deprotonation of the Schiff base is a prerequisite for transducin activation. The positions of the C = C and C - C stretching modes of the retinal suggest that the redshift observed in ASMR and its photoproducts can be explained by an increased distance of the Schiff base from the counterion(s). It is further shown that the photoreaction does not stop at metarhodopsin-I, but that this intermediate directly decays to a metarhodopsin-III-like species.
Full text
PDF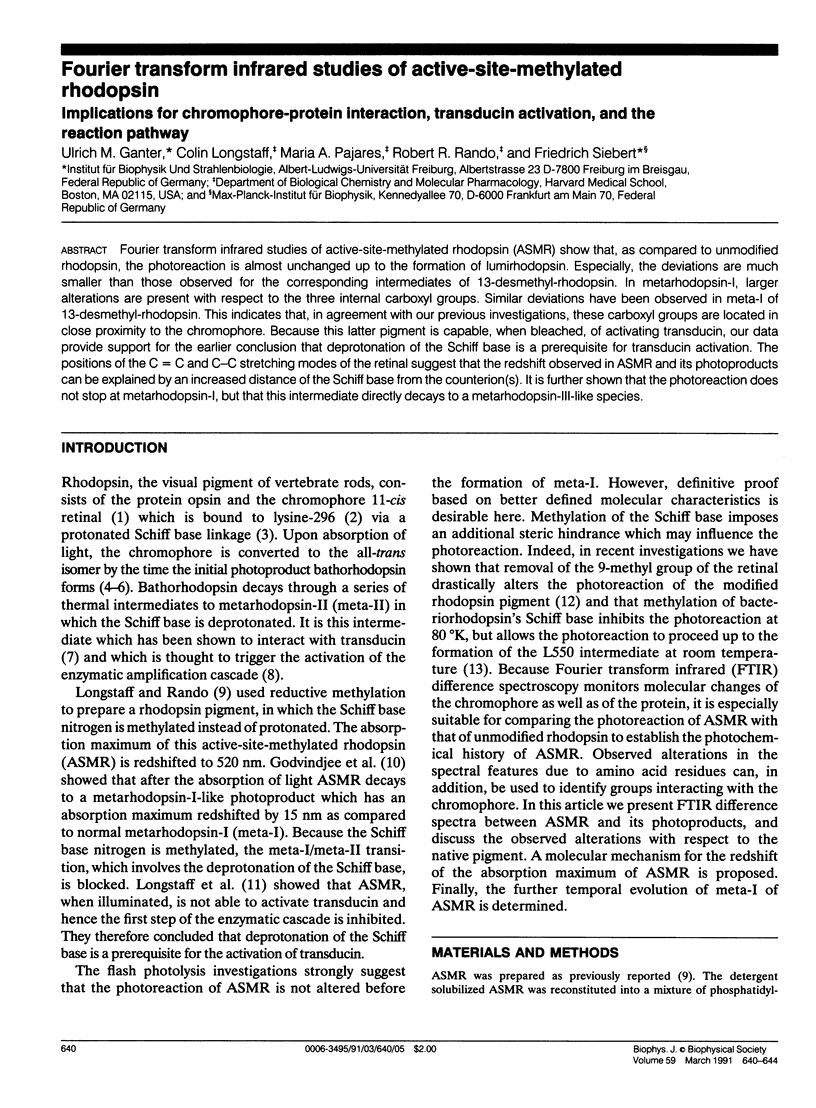
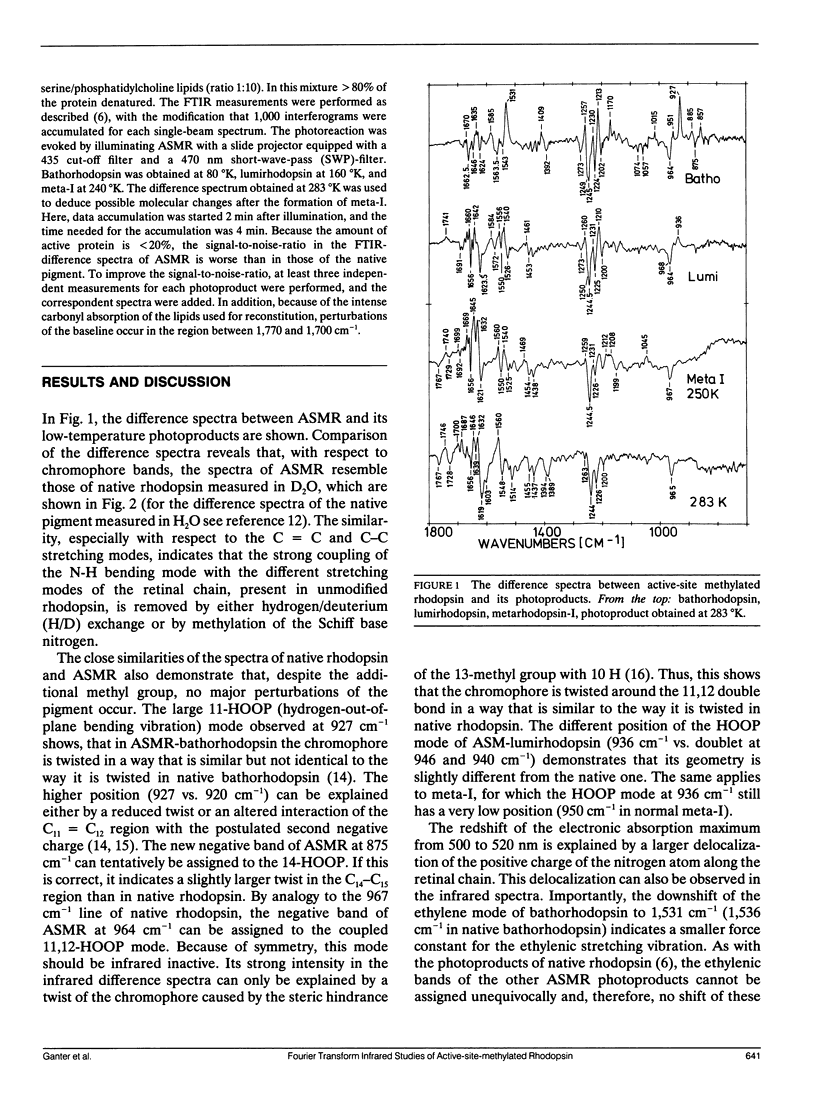
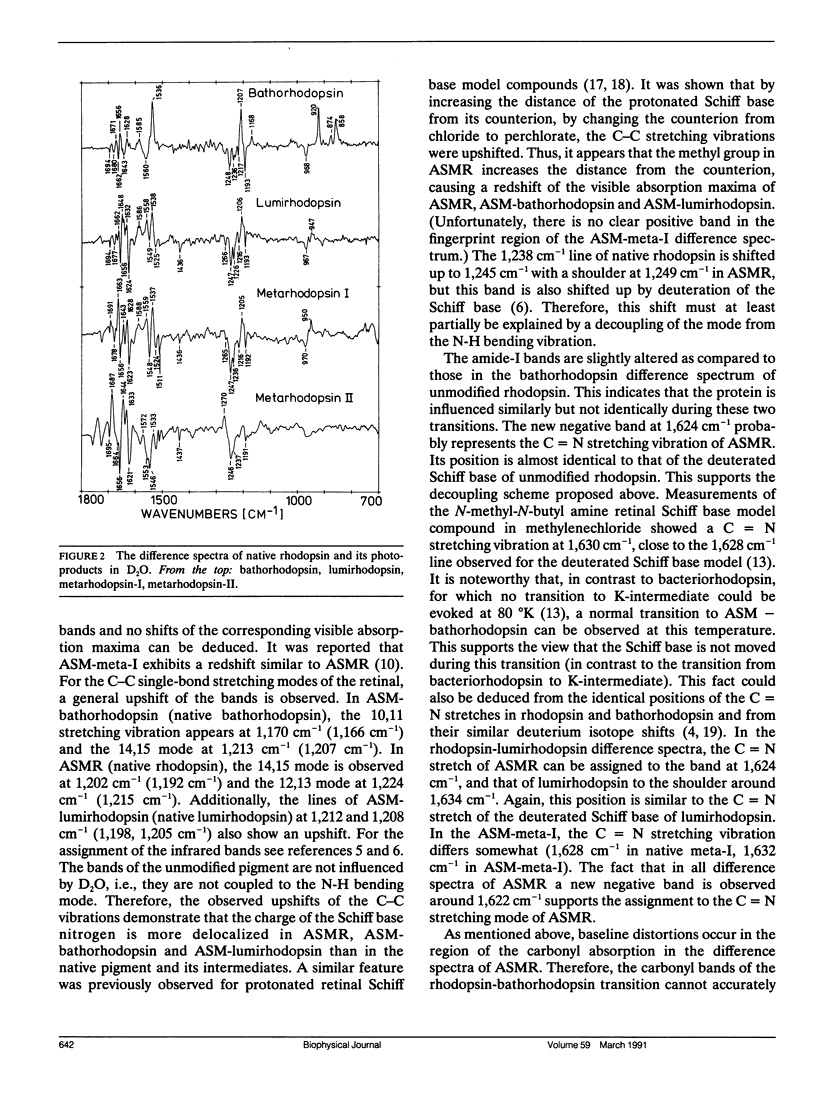
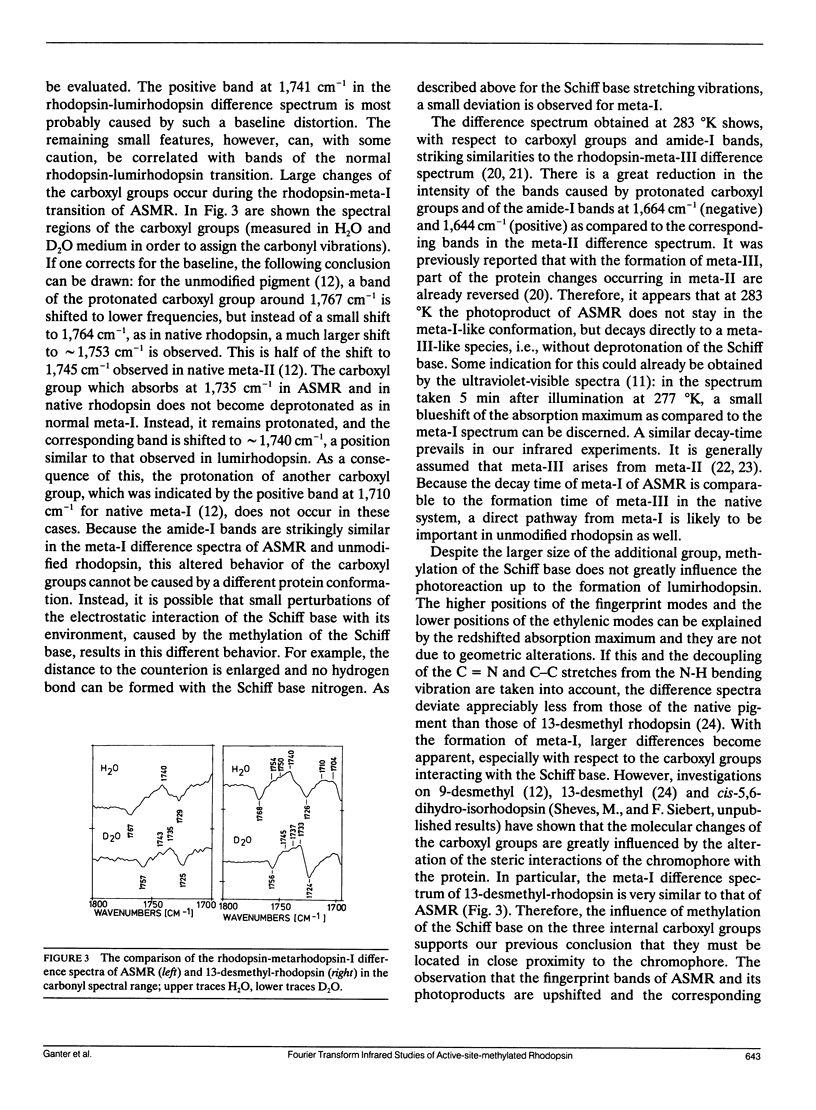
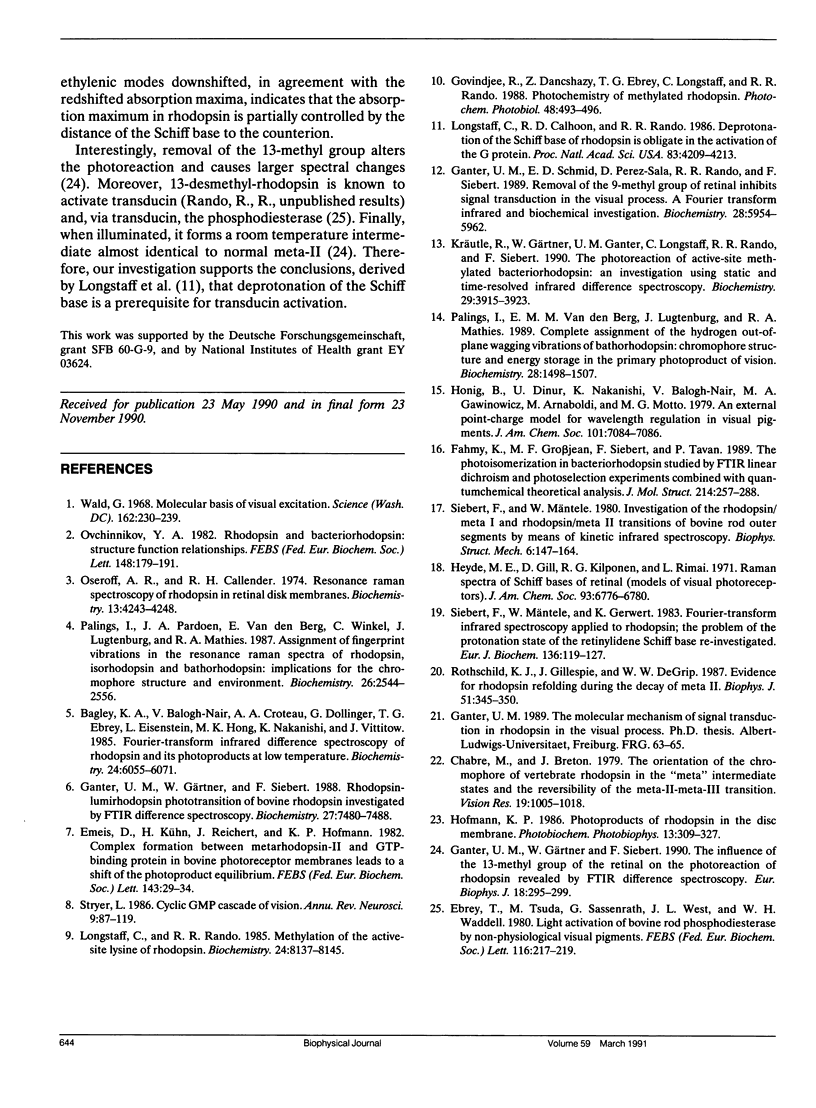
Selected References
These references are in PubMed. This may not be the complete list of references from this article.
- Bagley K. A., Balogh-Nair V., Croteau A. A., Dollinger G., Ebrey T. G., Eisenstein L., Hong M. K., Nakanishi K., Vittitow J. Fourier-transform infrared difference spectroscopy of rhodopsin and its photoproducts at low temperature. Biochemistry. 1985 Oct 22;24(22):6055–6071. doi: 10.1021/bi00343a006. [DOI] [PubMed] [Google Scholar]
- Chabre M., Breton J. The orientation of the chromophore of vertebrate rhodopsin in the "meta" intermediate states and the reversibility of the meta II-meta III transition. Vision Res. 1979;19(9):1005–1018. doi: 10.1016/0042-6989(79)90226-8. [DOI] [PubMed] [Google Scholar]
- Ebrey T., Tsuda M., Sassenrath G., West J. L., Waddell W. H. Light activation of bovine rod phosphodiesterase by non-physiological visual pigments. FEBS Lett. 1980 Jul 28;116(2):217–219. doi: 10.1016/0014-5793(80)80647-8. [DOI] [PubMed] [Google Scholar]
- Emeis D., Kühn H., Reichert J., Hofmann K. P. Complex formation between metarhodopsin II and GTP-binding protein in bovine photoreceptor membranes leads to a shift of the photoproduct equilibrium. FEBS Lett. 1982 Jun 21;143(1):29–34. doi: 10.1016/0014-5793(82)80266-4. [DOI] [PubMed] [Google Scholar]
- Ganter U. M., Gärtner W., Siebert F. Rhodopsin-lumirhodopsin phototransition of bovine rhodopsin investigated by Fourier transform infrared difference spectroscopy. Biochemistry. 1988 Sep 20;27(19):7480–7488. doi: 10.1021/bi00419a046. [DOI] [PubMed] [Google Scholar]
- Ganter U. M., Schmid E. D., Perez-Sala D., Rando R. R., Siebert F. Removal of the 9-methyl group of retinal inhibits signal transduction in the visual process. A Fourier transform infrared and biochemical investigation. Biochemistry. 1989 Jul 11;28(14):5954–5962. doi: 10.1021/bi00440a036. [DOI] [PubMed] [Google Scholar]
- Govindjee R., Dancshazy Z., Ebrey T. G., Longstaff C., Rando R. R. Photochemistry of methylated rhodopsins. Photochem Photobiol. 1988 Oct;48(4):493–496. doi: 10.1111/j.1751-1097.1988.tb02851.x. [DOI] [PubMed] [Google Scholar]
- Heyde M. E., Gill D., Kilponen R. G., Rimai L. Raman spectra of Schiff bases of retinal (models of visual photoreceptors). J Am Chem Soc. 1971 Dec 15;93(25):6776–6780. doi: 10.1021/ja00754a012. [DOI] [PubMed] [Google Scholar]
- Kräutle R., Gärtner W., Ganter U. M., Longstaff C., Rando R. R., Siebert F. The photoreaction of active-site-methylated bacteriorhodopsin: an investigation using static and time-resolved infrared difference spectroscopy. Biochemistry. 1990 Apr 24;29(16):3915–3923. doi: 10.1021/bi00468a018. [DOI] [PubMed] [Google Scholar]
- Longstaff C., Calhoon R. D., Rando R. R. Deprotonation of the Schiff base of rhodopsin is obligate in the activation of the G protein. Proc Natl Acad Sci U S A. 1986 Jun;83(12):4209–4213. doi: 10.1073/pnas.83.12.4209. [DOI] [PMC free article] [PubMed] [Google Scholar]
- Longstaff C., Rando R. R. Methylation of the active-site lysine of rhodopsin. Biochemistry. 1985 Dec 31;24(27):8137–8145. doi: 10.1021/bi00348a045. [DOI] [PubMed] [Google Scholar]
- Oseroff A. R., Callender R. H. Resonance Raman spectroscopy of rhodopsin in retinal disk membranes. Biochemistry. 1974 Sep 24;13(20):4243–4248. doi: 10.1021/bi00717a027. [DOI] [PubMed] [Google Scholar]
- Ovchinnikov YuA Rhodopsin and bacteriorhodopsin: structure-function relationships. FEBS Lett. 1982 Nov 8;148(2):179–191. doi: 10.1016/0014-5793(82)80805-3. [DOI] [PubMed] [Google Scholar]
- Palings I., Pardoen J. A., van den Berg E., Winkel C., Lugtenburg J., Mathies R. A. Assignment of fingerprint vibrations in the resonance Raman spectra of rhodopsin, isorhodopsin, and bathorhodopsin: implications for chromophore structure and environment. Biochemistry. 1987 May 5;26(9):2544–2556. doi: 10.1021/bi00383a021. [DOI] [PubMed] [Google Scholar]
- Palings I., van den Berg E. M., Lugtenburg J., Mathies R. A. Complete assignment of the hydrogen out-of-plane wagging vibrations of bathorhodopsin: chromophore structure and energy storage in the primary photoproduct of vision. Biochemistry. 1989 Feb 21;28(4):1498–1507. doi: 10.1021/bi00430a012. [DOI] [PubMed] [Google Scholar]
- Rothschild K. J., Gillespie J., DeGrip W. J. Evidence for rhodopsin refolding during the decay of Meta II. Biophys J. 1987 Feb;51(2):345–350. doi: 10.1016/S0006-3495(87)83341-6. [DOI] [PMC free article] [PubMed] [Google Scholar]
- Siebert F., Mäntele W., Gerwert K. Fourier-transform infrared spectroscopy applied to rhodopsin. The problem of the protonation state of the retinylidene Schiff base re-investigated. Eur J Biochem. 1983 Oct 17;136(1):119–127. doi: 10.1111/j.1432-1033.1983.tb07714.x. [DOI] [PubMed] [Google Scholar]
- Siebert F., Mäntele W. Investigations of the rhodopsin/Meta I and rhodopsin/Meta II transitions of bovine rod outer segments by means of kinetic infrared spectroscopy. Biophys Struct Mech. 1980;6(2):147–164. doi: 10.1007/BF00535751. [DOI] [PubMed] [Google Scholar]
- Stryer L. Cyclic GMP cascade of vision. Annu Rev Neurosci. 1986;9:87–119. doi: 10.1146/annurev.ne.09.030186.000511. [DOI] [PubMed] [Google Scholar]
- Wald G. Molecular basis of visual excitation. Science. 1968 Oct 11;162(3850):230–239. doi: 10.1126/science.162.3850.230. [DOI] [PubMed] [Google Scholar]