Abstract
Electrostatic calculation of the gramicidin channel is performed on the basis of a three-dielectric model in which the peptide backbone of the channel is added as a third dielectric region to the conventional two-dielectric channel model (whose pore radius is often referred to as the effective pore radius reff). A basic principle for calculating electrostatic fields in three-dielectric models is introduced. It is shown that the gramicidin channel has no unique value of reff. The reff with respect to the "self-image energy" (i.e., the image energy in the presence of a single ion) is 2.6-2.7 A, slightly depending upon the position of the ion (the least-square value over the whole length of the pore is 2.6 A). In contrast, the reff with respect to the electric potential due to an ion (and hence the reff with respect to the interaction energy between two ions) is dependent upon the distance s of separation; it ranges from 2.6 to greater than 5 A, increasing with an increase in s. However, for the purpose of rough estimation, the reff with respect to the self-image energy can also be used in calculating the electric potential and the interaction energy, because the error introduced by this approximation is an overestimation of the order of 30% at most. It is also shown that the apparent dielectric constant for the interaction between two charges depends markedly upon the positions of the charges. In the course of this study, the dielectric constant and polarizability of the peptide backbone in the beta-sheet structure is estimated to be 10 and 8.22 A3.
Full text
PDF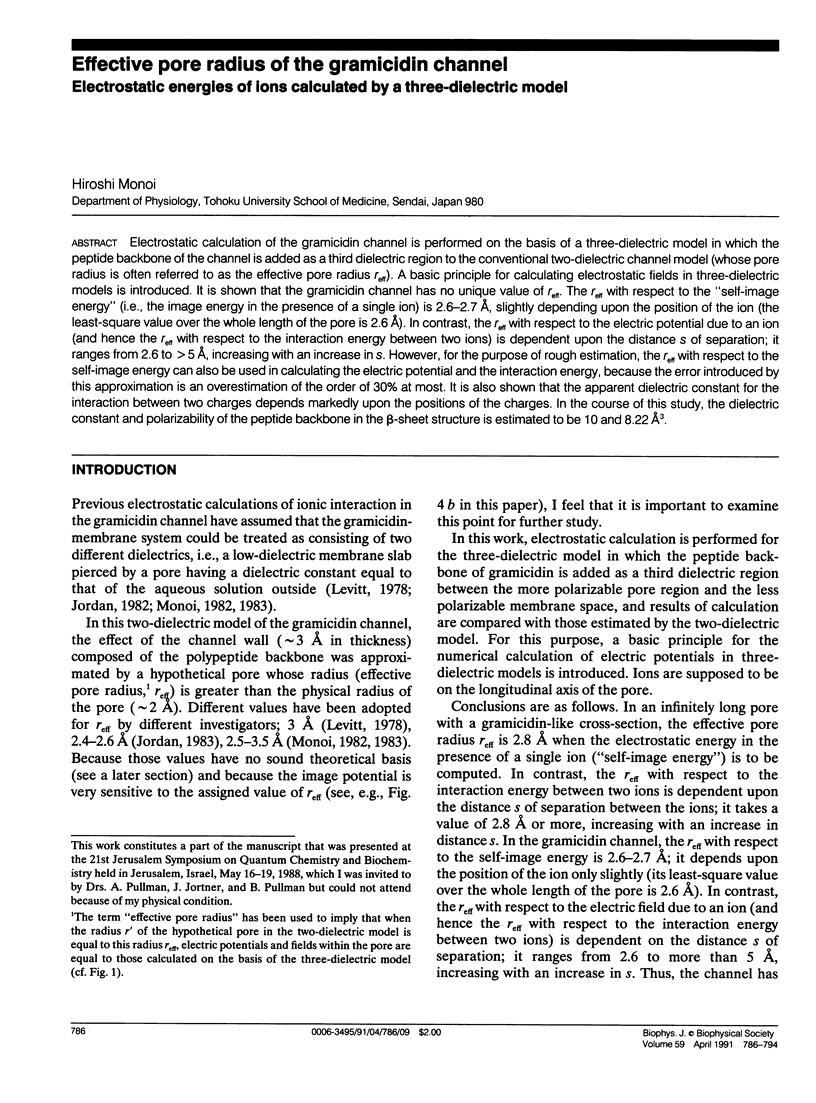
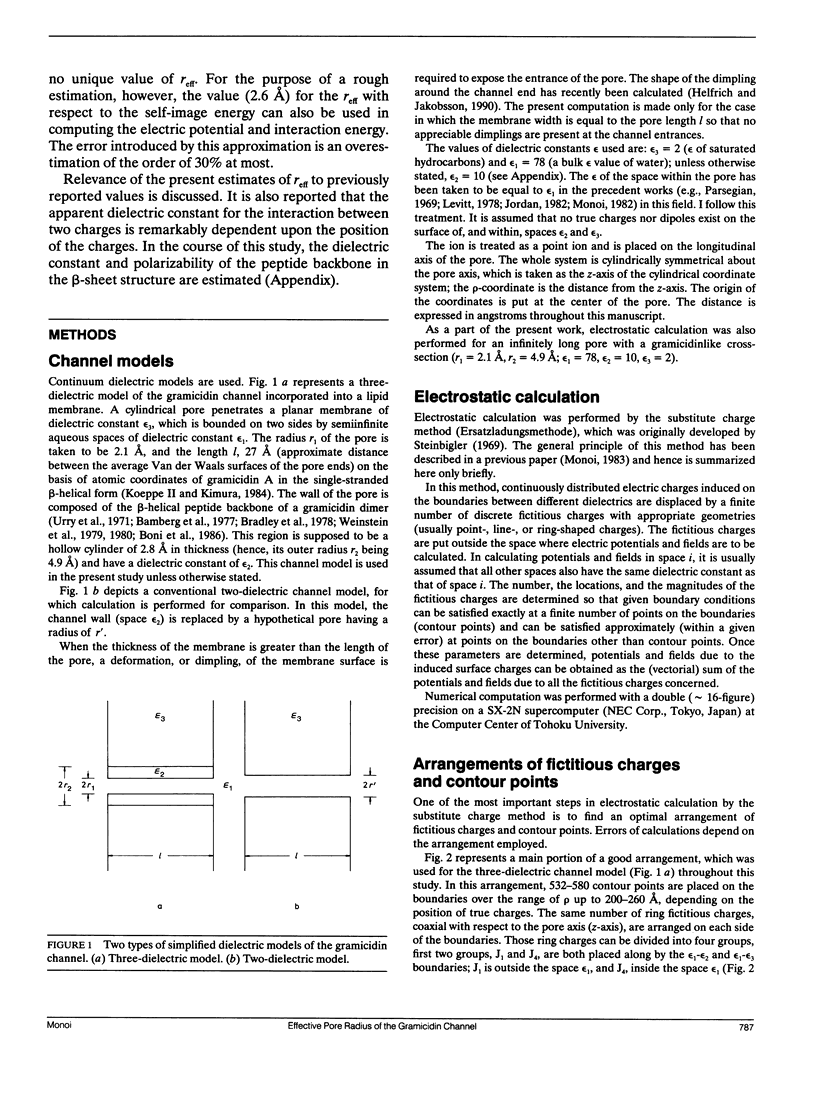
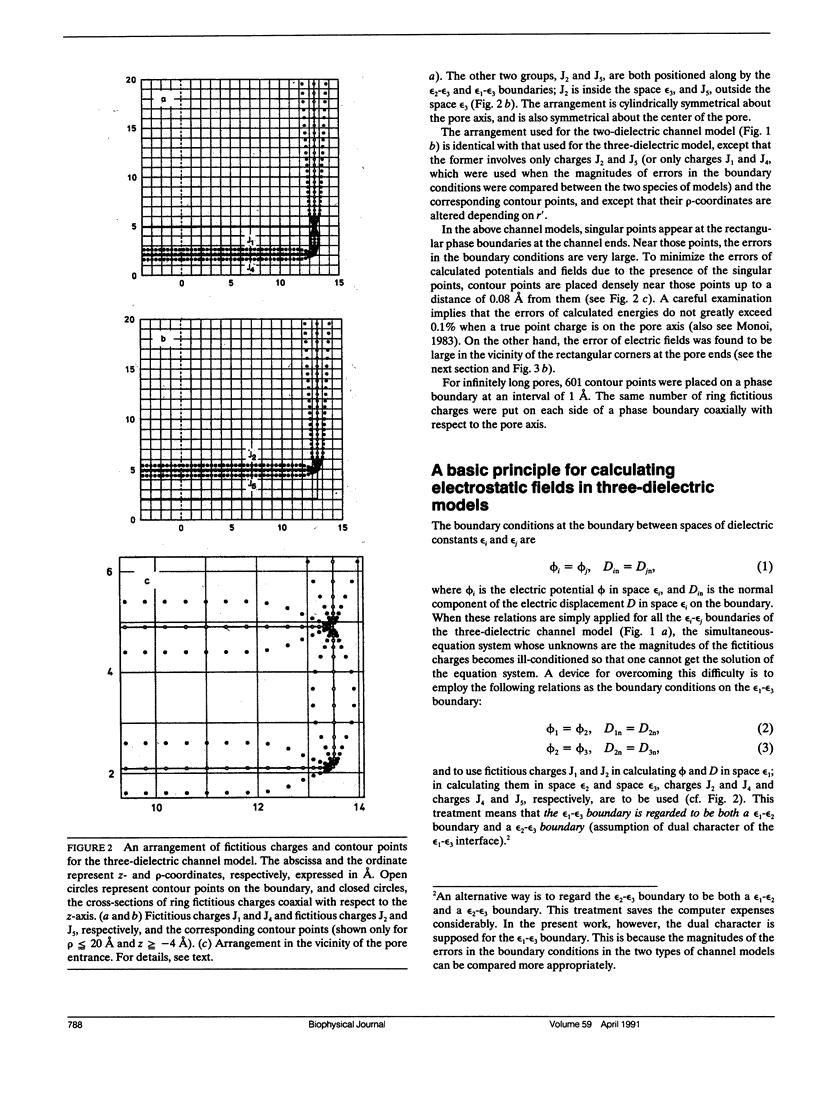
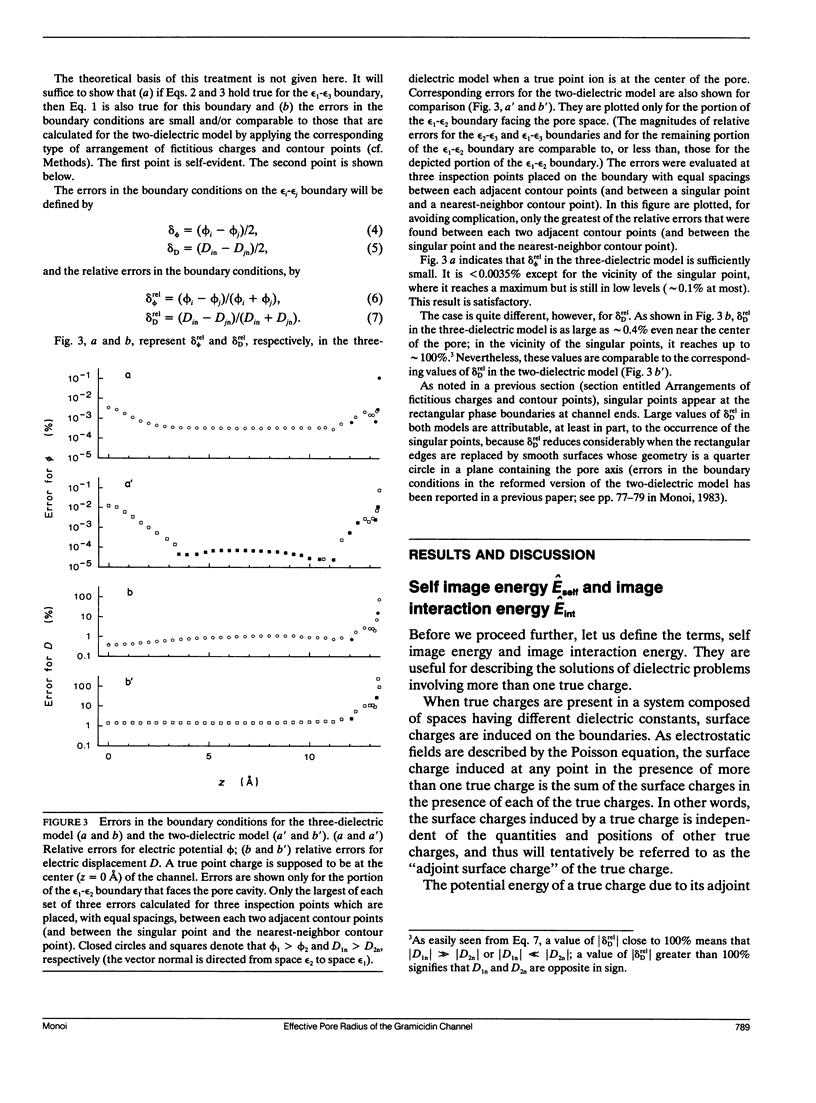
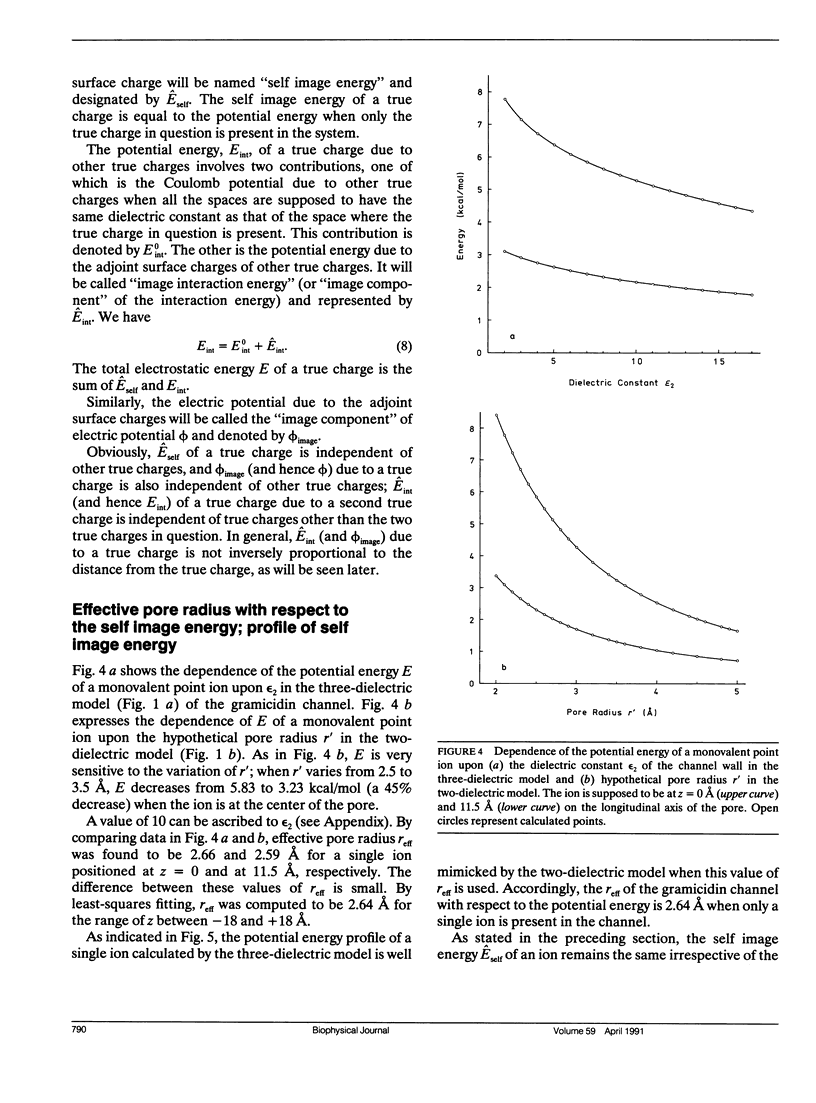
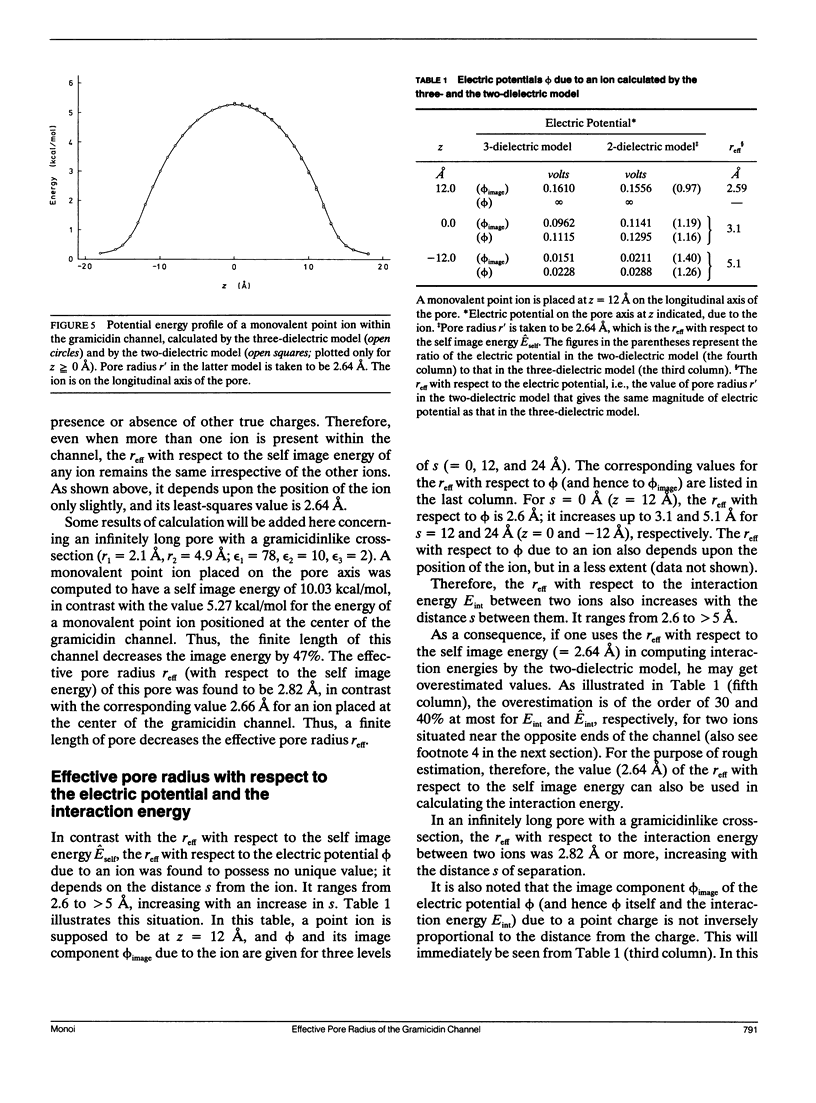
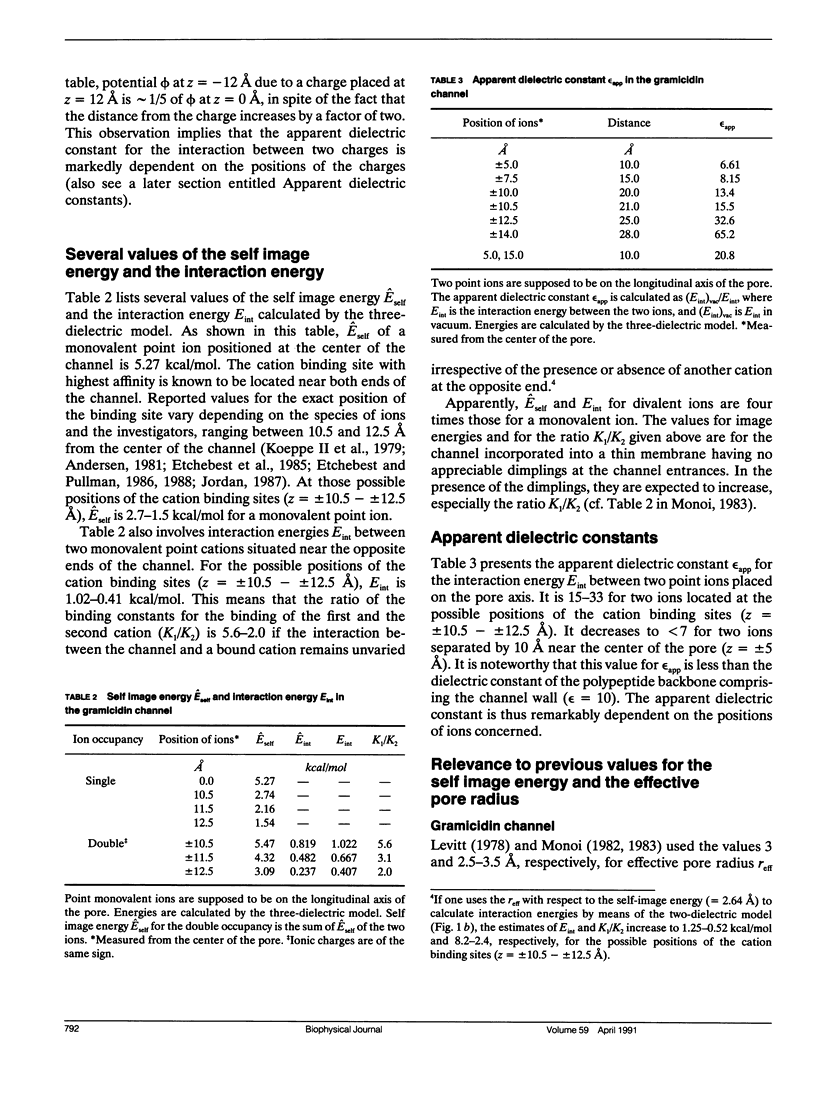
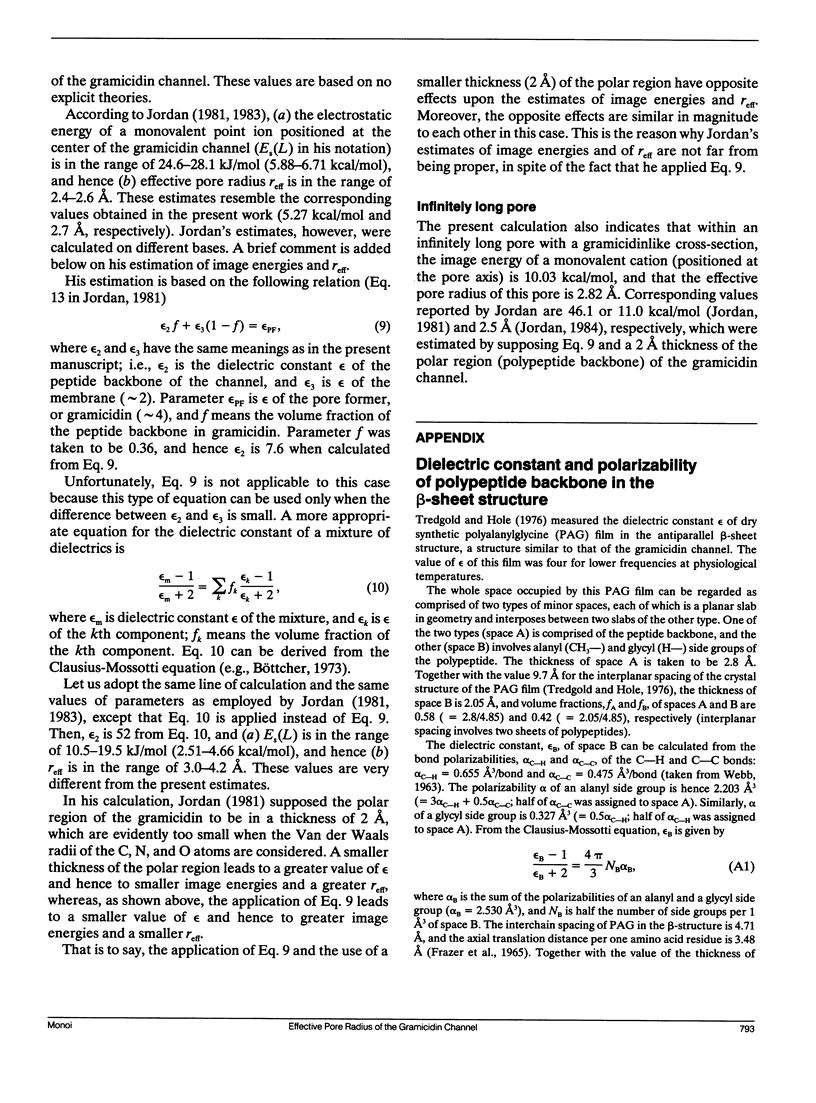
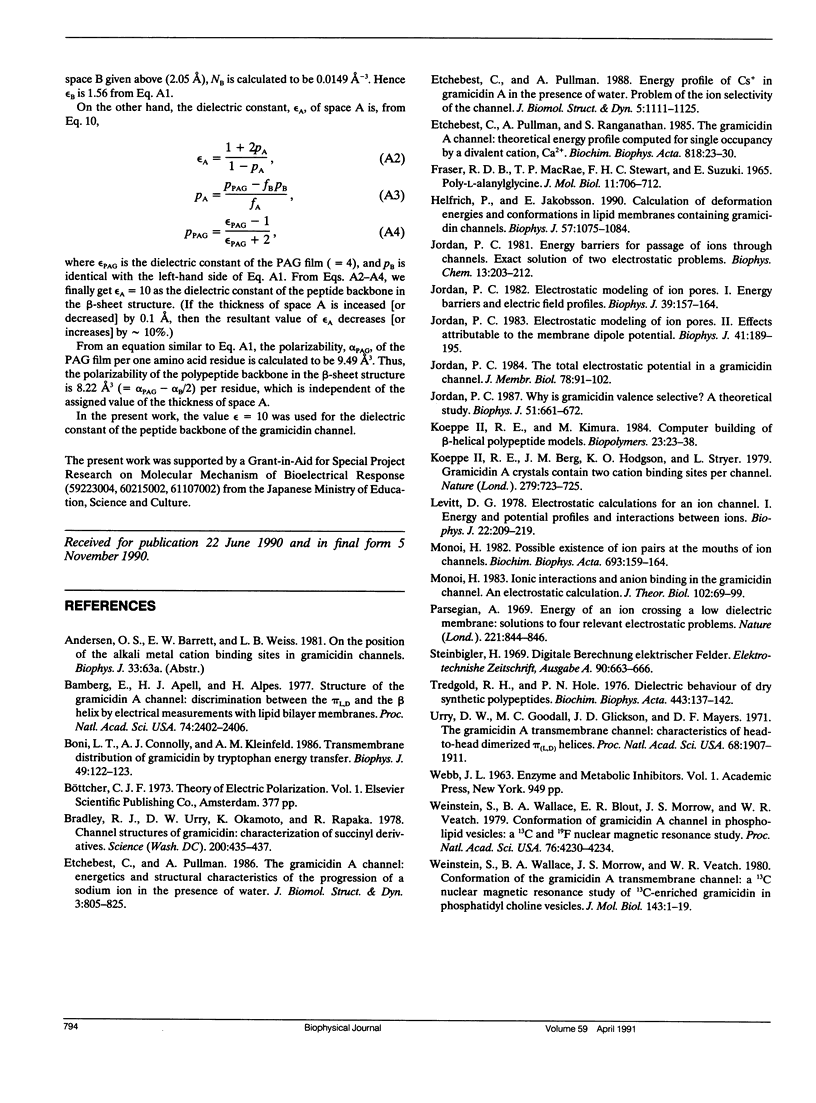
Selected References
These references are in PubMed. This may not be the complete list of references from this article.
- Bamberg E., Apell H. J., Alpes H. Structure of the gramicidin A channel: discrimination between the piL,D and the beta helix by electrical measurements with lipid bilayer membranes. Proc Natl Acad Sci U S A. 1977 Jun;74(6):2402–2406. doi: 10.1073/pnas.74.6.2402. [DOI] [PMC free article] [PubMed] [Google Scholar]
- Boni L. T., Connolly A. J., Kleinfeld A. M. Transmembrane distribution of gramicidin by tryptophan energy transfer. Biophys J. 1986 Jan;49(1):122–123. doi: 10.1016/S0006-3495(86)83619-0. [DOI] [PMC free article] [PubMed] [Google Scholar]
- Bradley R. J., Urry D. W., Okamoto K., Rapaka R. Channel structures of gramicidin: characterization of succinyl derivatives. Science. 1978 Apr 28;200(4340):435–437. doi: 10.1126/science.77040. [DOI] [PubMed] [Google Scholar]
- Etchebest C., Pullman A. Energy profile of Cs+ in gramicidin A in the presence of water. Problem of the ion selectivity of the channel. J Biomol Struct Dyn. 1988 Apr;5(5):1111–1125. doi: 10.1080/07391102.1988.10506452. [DOI] [PubMed] [Google Scholar]
- Etchebest C., Pullman A. The gramicidin A channel: energetics and structural characteristics of the progression of a sodium ion in the presence of water. J Biomol Struct Dyn. 1986 Feb;3(4):805–825. doi: 10.1080/07391102.1986.10508463. [DOI] [PubMed] [Google Scholar]
- FRASER R. D., MACRAE T. P., STEWART F. H., SUZUKI E. POLY-L-ALANYLGLYCINE. J Mol Biol. 1965 Apr;11:706–712. doi: 10.1016/s0022-2836(65)80028-6. [DOI] [PubMed] [Google Scholar]
- Helfrich P., Jakobsson E. Calculation of deformation energies and conformations in lipid membranes containing gramicidin channels. Biophys J. 1990 May;57(5):1075–1084. doi: 10.1016/S0006-3495(90)82625-4. [DOI] [PMC free article] [PubMed] [Google Scholar]
- Jordan P. C. Electrostatic modeling of ion pores. Energy barriers and electric field profiles. Biophys J. 1982 Aug;39(2):157–164. doi: 10.1016/S0006-3495(82)84503-7. [DOI] [PMC free article] [PubMed] [Google Scholar]
- Jordan P. C. Electrostatic modeling of ion pores. II. Effects attributable to the membrane dipole potential. Biophys J. 1983 Feb;41(2):189–195. doi: 10.1016/S0006-3495(83)84419-1. [DOI] [PMC free article] [PubMed] [Google Scholar]
- Jordan P. C. Energy barriers for passage of ions through channels. Exact solution of two electrostatic problems. Biophys Chem. 1981 Jun;13(3):203–212. doi: 10.1016/0301-4622(81)80002-6. [DOI] [PubMed] [Google Scholar]
- Koeppe R. E., 2nd, Berg J. M., Hodgson K. O., Stryer L. Gramicidin A crystals contain two cation binding sites per channel. Nature. 1979 Jun 21;279(5715):723–725. doi: 10.1038/279723a0. [DOI] [PubMed] [Google Scholar]
- Levitt D. G. Electrostatic calculations for an ion channel. I. Energy and potential profiles and interactions between ions. Biophys J. 1978 May;22(2):209–219. doi: 10.1016/S0006-3495(78)85485-X. [DOI] [PMC free article] [PubMed] [Google Scholar]
- Monoi H. Ionic interactions and anion binding in the gramicidin channel. An electrostatic calculation. J Theor Biol. 1983 May 7;102(1):69–99. doi: 10.1016/0022-5193(83)90263-1. [DOI] [PubMed] [Google Scholar]
- Monoi H. Possible existence of ion pairs at the mouths of ion channels. Biochim Biophys Acta. 1982 Dec 8;693(1):159–164. doi: 10.1016/0005-2736(82)90482-5. [DOI] [PubMed] [Google Scholar]
- Parsegian A. Energy of an ion crossing a low dielectric membrane: solutions to four relevant electrostatic problems. Nature. 1969 Mar 1;221(5183):844–846. doi: 10.1038/221844a0. [DOI] [PubMed] [Google Scholar]
- Sung S. S., Jordan P. C. Why is gramicidin valence selective? A theoretical study. Biophys J. 1987 Apr;51(4):661–672. doi: 10.1016/S0006-3495(87)83391-X. [DOI] [PMC free article] [PubMed] [Google Scholar]
- Tredgold R. H., Hole P. N. Dielectric behaviour of dry synthetic polypeptides. Biochim Biophys Acta. 1976 Aug 4;443(1):137–142. doi: 10.1016/0005-2736(76)90497-1. [DOI] [PubMed] [Google Scholar]
- Urry D. W., Goodall M. C., Glickson J. D., Mayers D. F. The gramicidin A transmembrane channel: characteristics of head-to-head dimerized (L,D) helices. Proc Natl Acad Sci U S A. 1971 Aug;68(8):1907–1911. doi: 10.1073/pnas.68.8.1907. [DOI] [PMC free article] [PubMed] [Google Scholar]
- Weinstein S., Wallace B. A., Blout E. R., Morrow J. S., Veatch W. Conformation of gramicidin A channel in phospholipid vesicles: a 13C and 19F nuclear magnetic resonance study. Proc Natl Acad Sci U S A. 1979 Sep;76(9):4230–4234. doi: 10.1073/pnas.76.9.4230. [DOI] [PMC free article] [PubMed] [Google Scholar]
- Weinstein S., Wallace B. A., Morrow J. S., Veatch W. R. Conformation of the gramicidin A transmembrane channel: A 13C nuclear magnetic resonance study of 13C-enriched gramicidin in phosphatidylcholine vesicles. J Mol Biol. 1980 Oct 15;143(1):1–19. doi: 10.1016/0022-2836(80)90121-7. [DOI] [PubMed] [Google Scholar]