Abstract
1. The metallochromic indicator dye, arsenazo III, was injected into somata of molluscan neurones from Archidoris monteryensis. Membrane current and dye absorbance change were simultaneously monitored under voltage clamp. 2. Absorbance measured at 660 nm increased during positive-going voltage steps large enough to activate membrane conductances. In situ idifference spectra were qualitatively similar to dye, dye-calcium difference spectra recorded in vitro. The absorbance change was abolished by either a thorough removal of external calcium or internal chelation of calcium by EGTA. It was concluded that the absorbance increase primarily reflected changing internal calcium concentration and that the calcium entered from the outside. 3. Dye absorbance increased in a nearly linear fashion during voltage clamp pulses of 100--300 msec duration. This is in qualitative agreement with electrical stuidies which demonstrated only fractional inactivation of calcium conductance during such periods. Plots of absorbance change vs. Vm peaked at +30 to +40 mV and fell off sharply until approximately +70 mV where the slope became less steep. A null or reversal of the absorbance change was generally observed aroung +110 mV. Evidence is presented that calcium influx was in some cases sufficient to cause sizeable changes in its equilibrium potential. 4. During multisecond voltage clamps the slope of the absorbance change showed a large decline. Where barium substituted for calcium as the influx species in identical clamps, the absorbance at 660 nm also increased but in a much more linear fashion. Except for a slight effect on the initial few pulses, the absorbance signal did not recover after a period of barium influx. These results suggest that part of the slope decline might result from processes related to calcium uptake and not to membrane conductance decrease. 5. Dye absorbance changes during normal and TEA action potentials were measured. Comparison of these changes with voltage clamp records indicated that calcium influx during a spike was capable of raising concentration by roughly 2 X 10(-7) M if the cell were considered to be a uniform sphere with no buffering capacity. Calcium influx during action potentials was increased dramatically by TEA, primarily as a result of a prolonged plateau phase. The existence and duration of the plateau was controlled mainly by potassium conductance systems, however. There was no evidence found for facilitation of the calcium conductance. 6. Following a moderate influx of calcium it required 20--60 sec, depending on the neurone, for the dye absorbance to return to base line (at 9 degrees C). The recovery time course showed a marked difference when examined at different wavelengths. For lambda = 660 nm there was an initial period in which the absorbance decreased rapidly, followed by a slower phase which generally carried the absorbance below the initial (prepulse) value...
Full text
PDF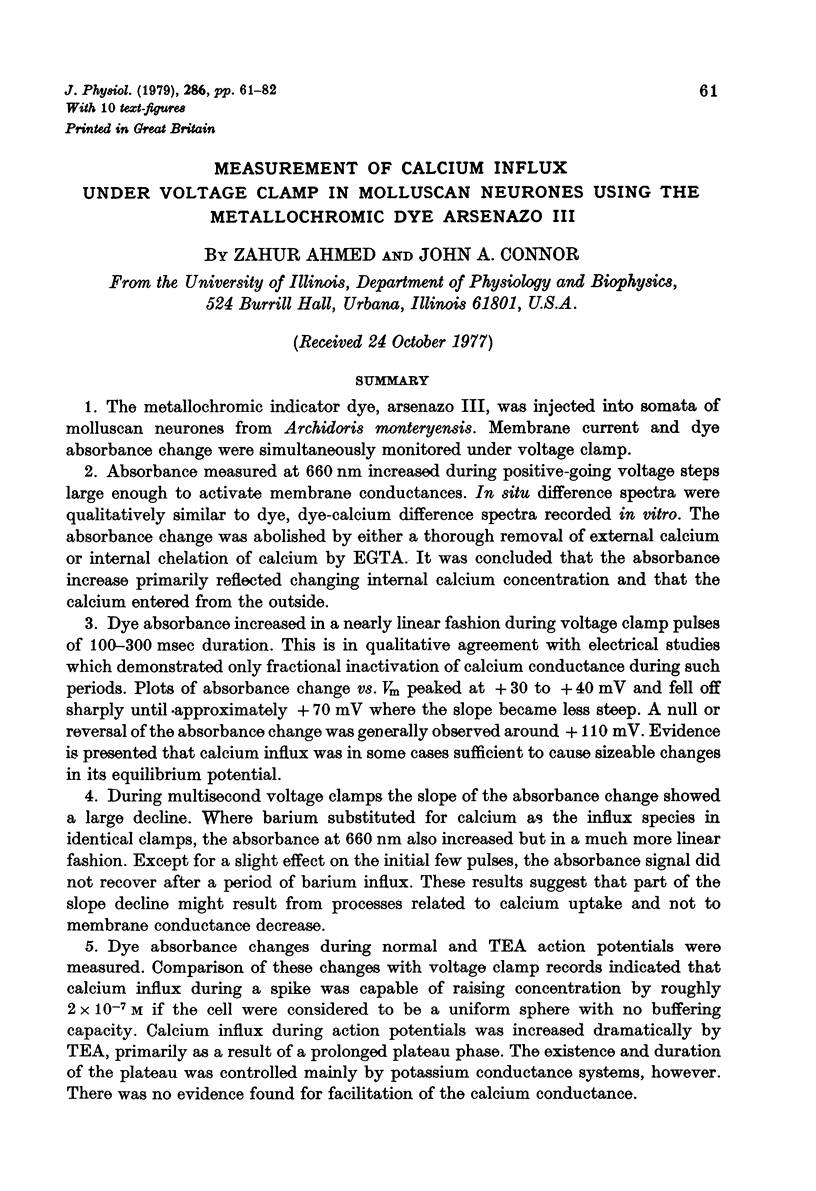
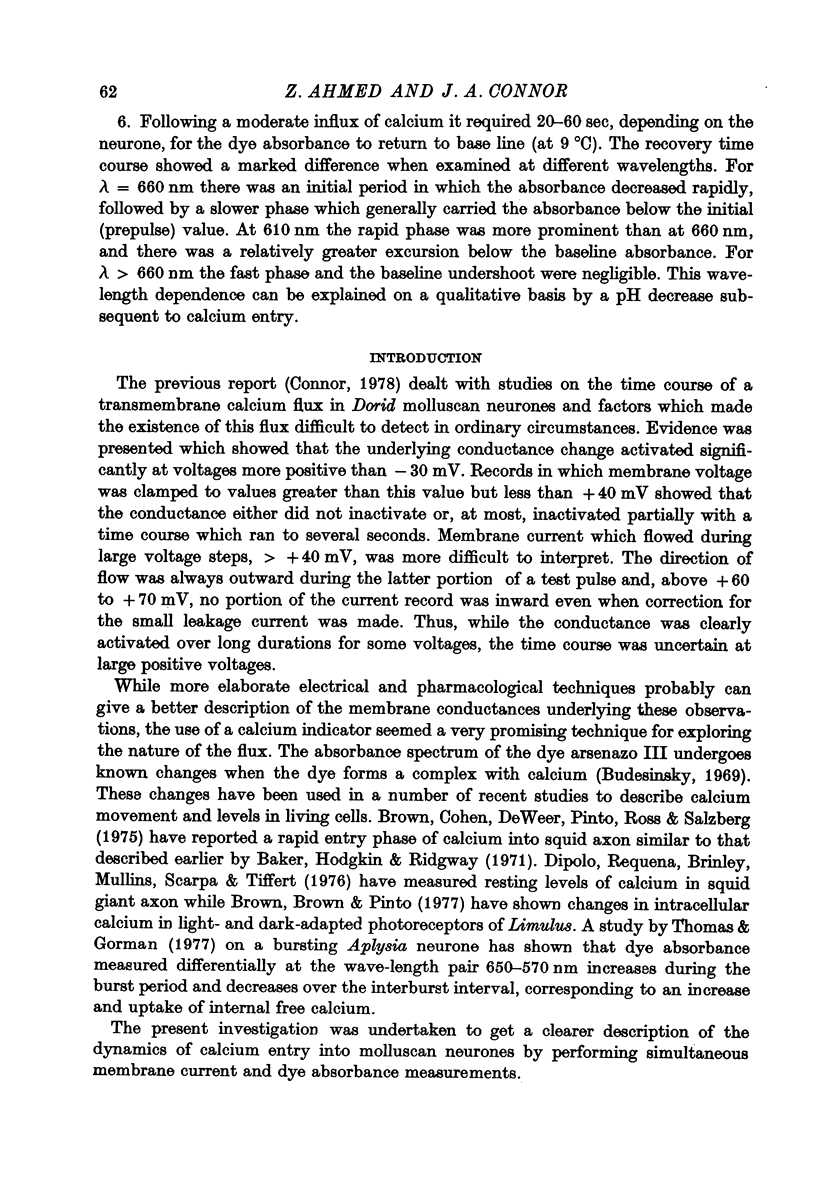
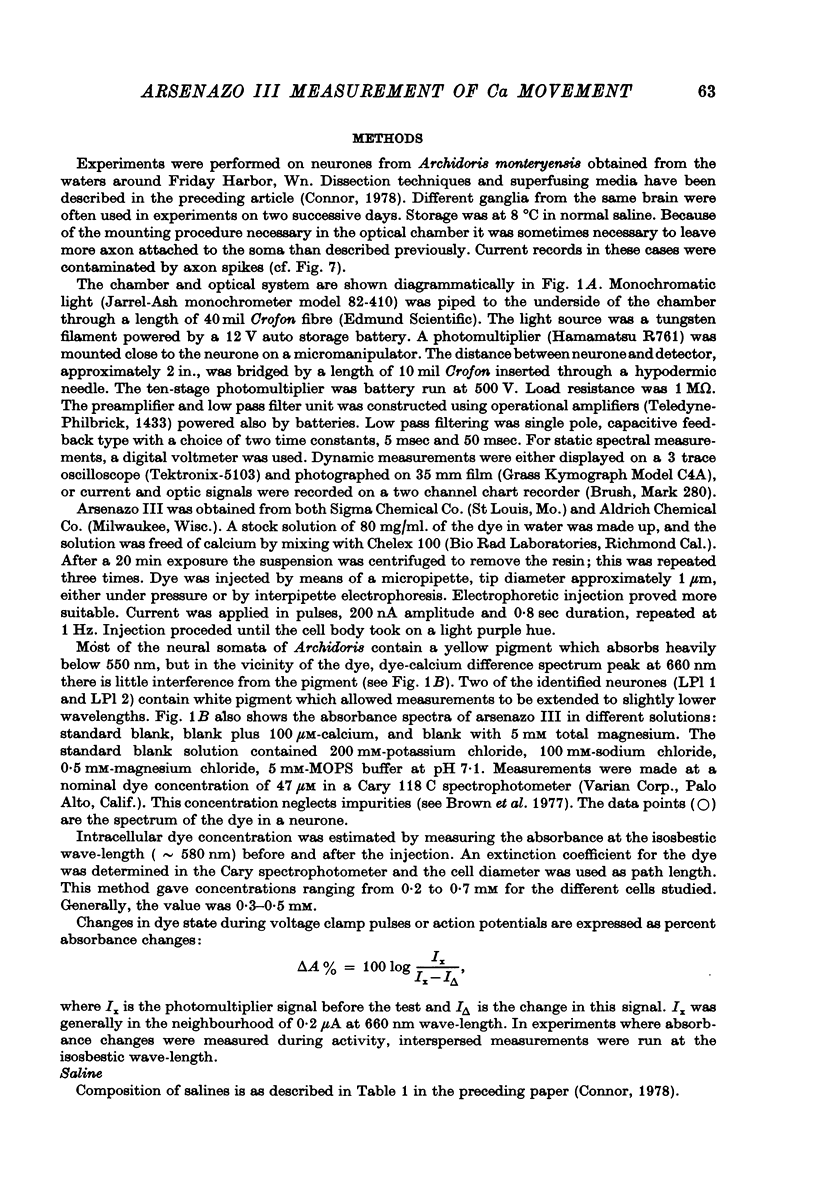
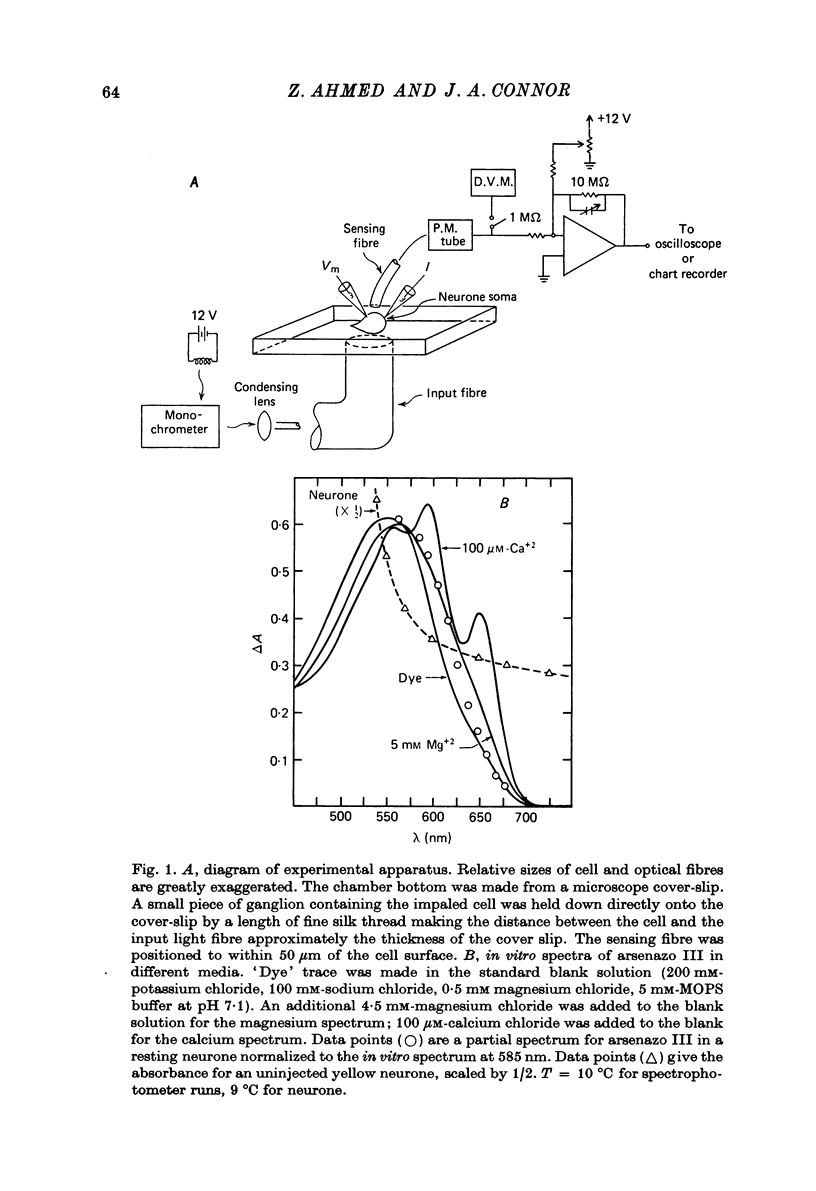
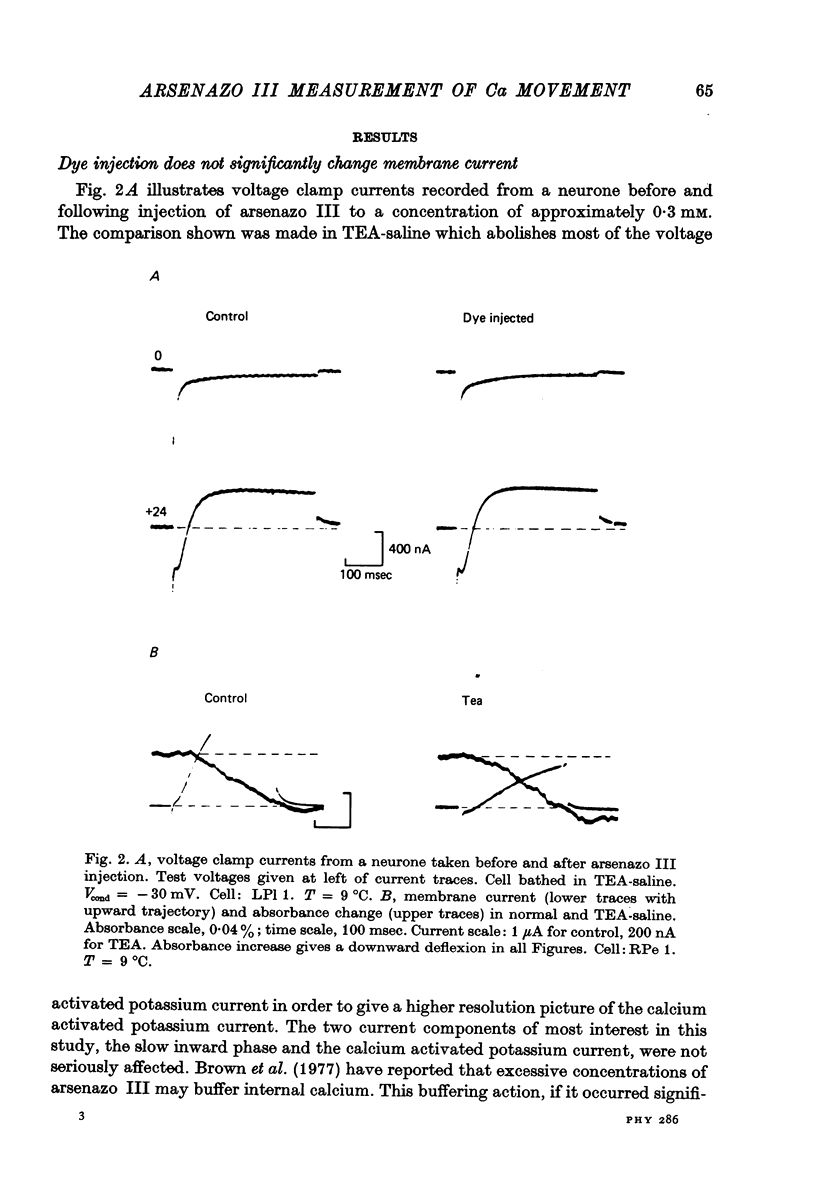
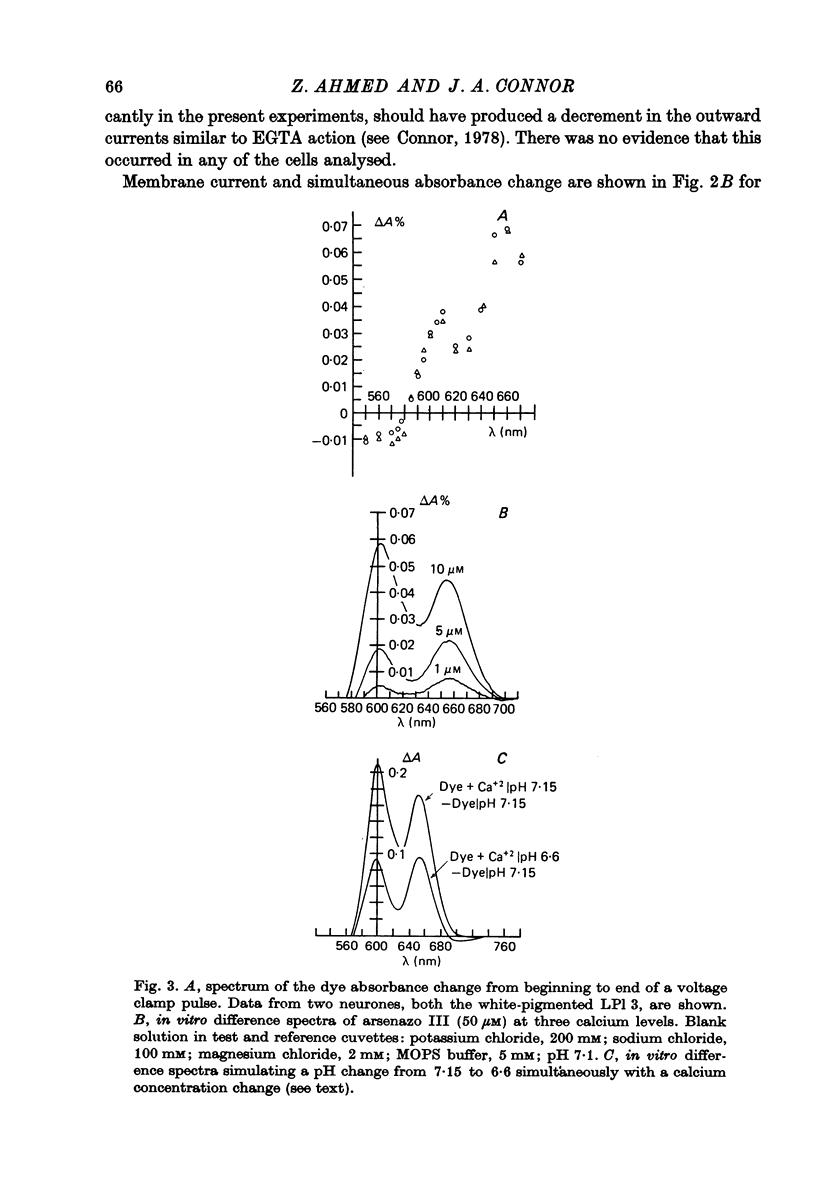
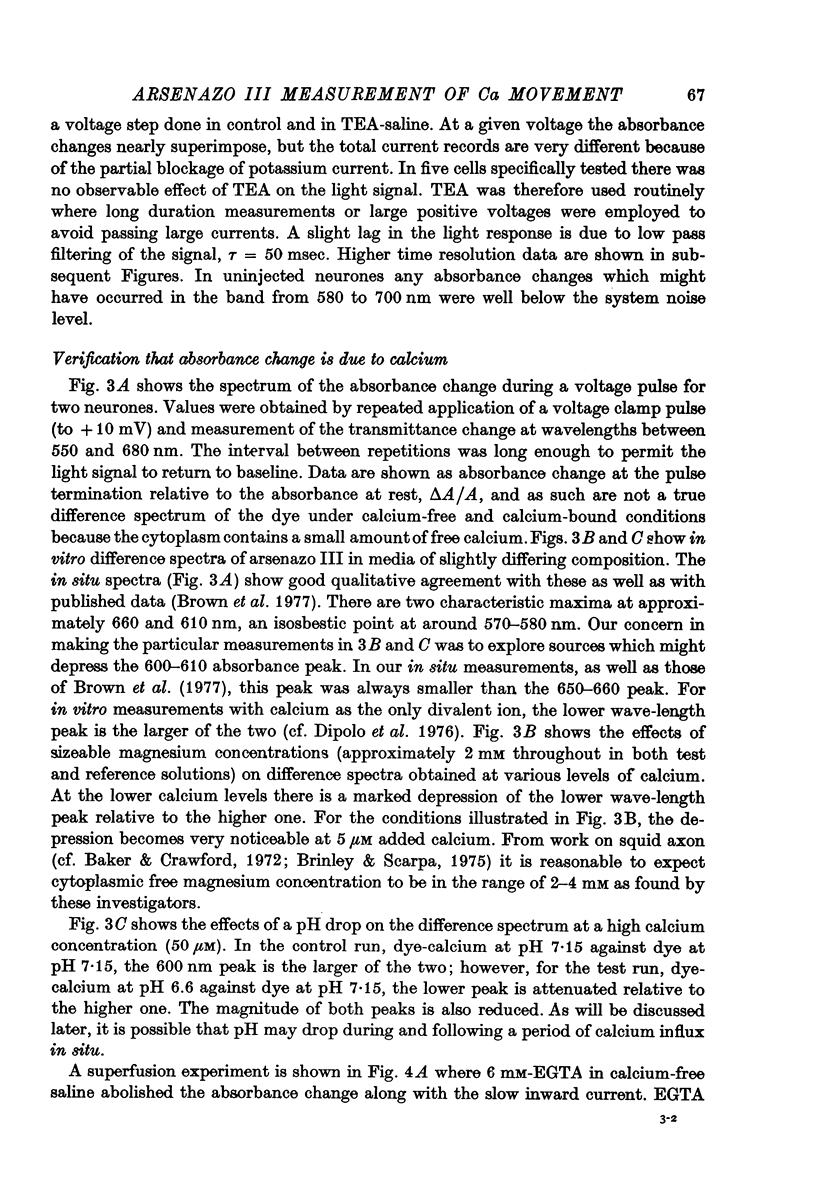
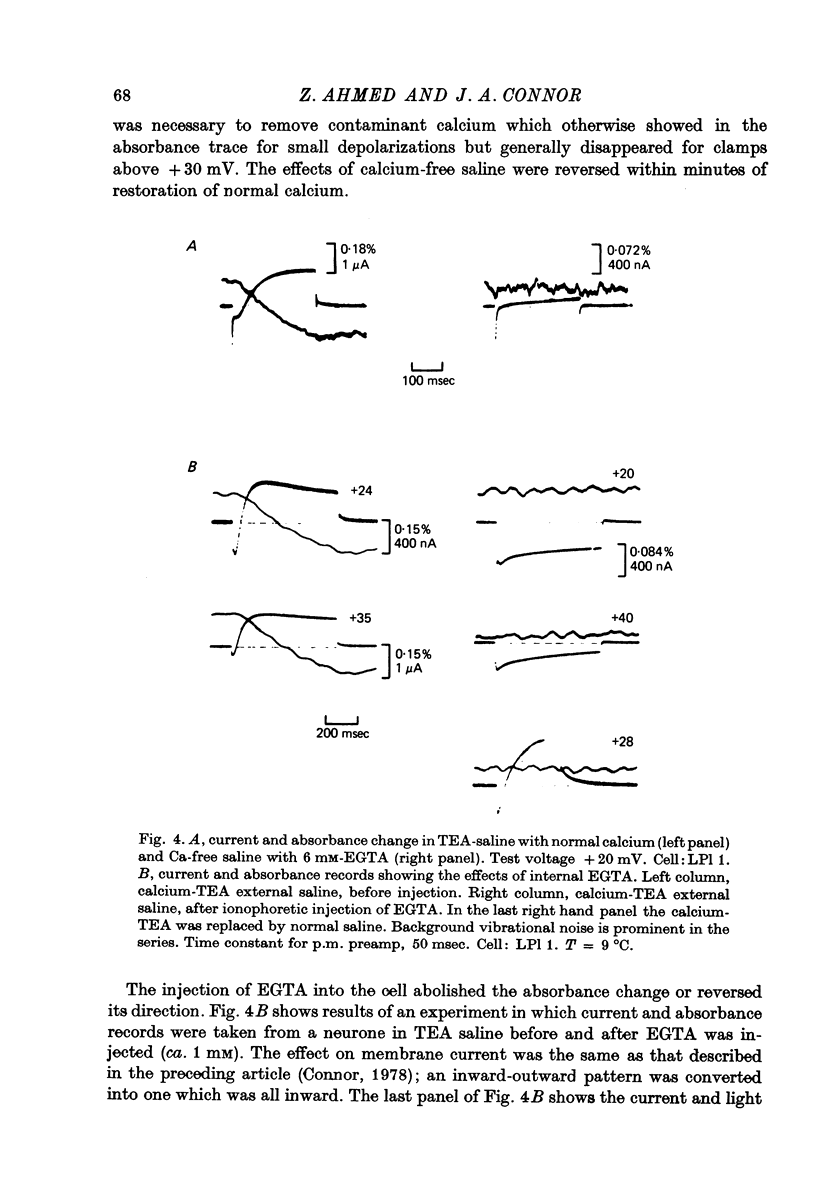
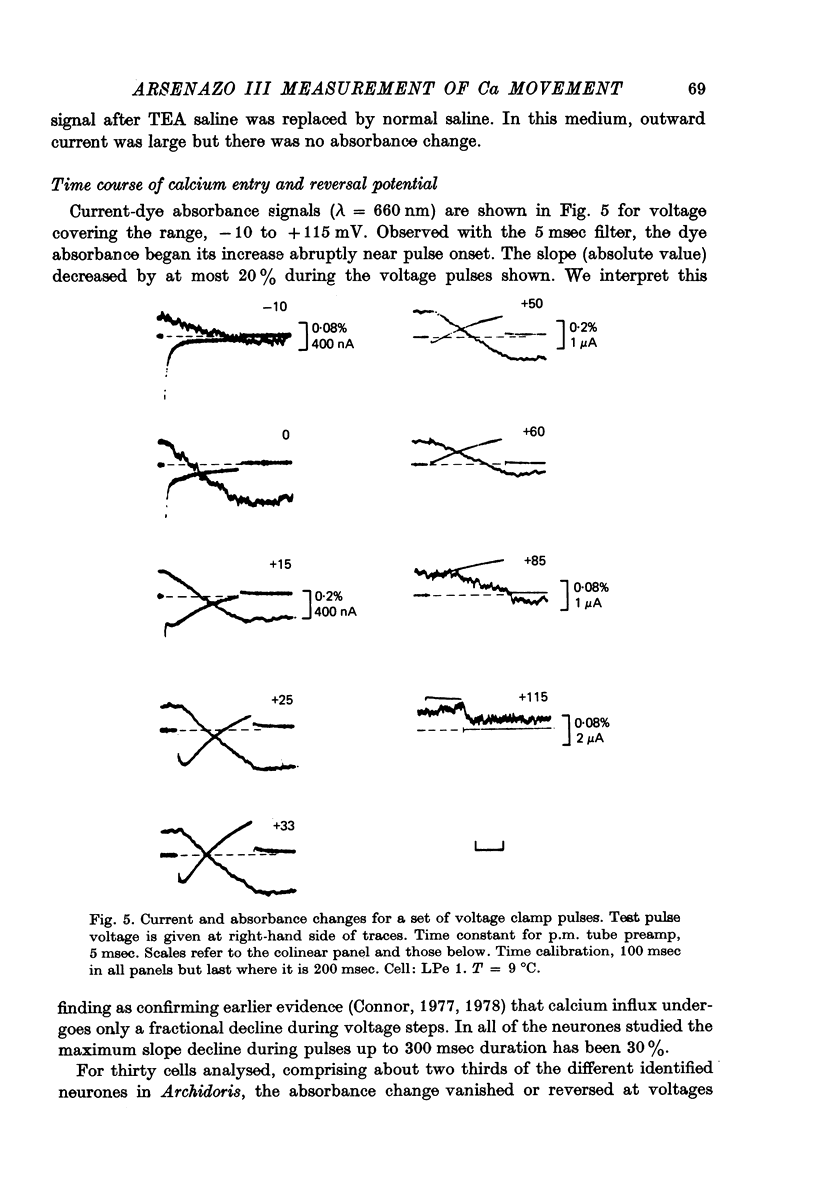
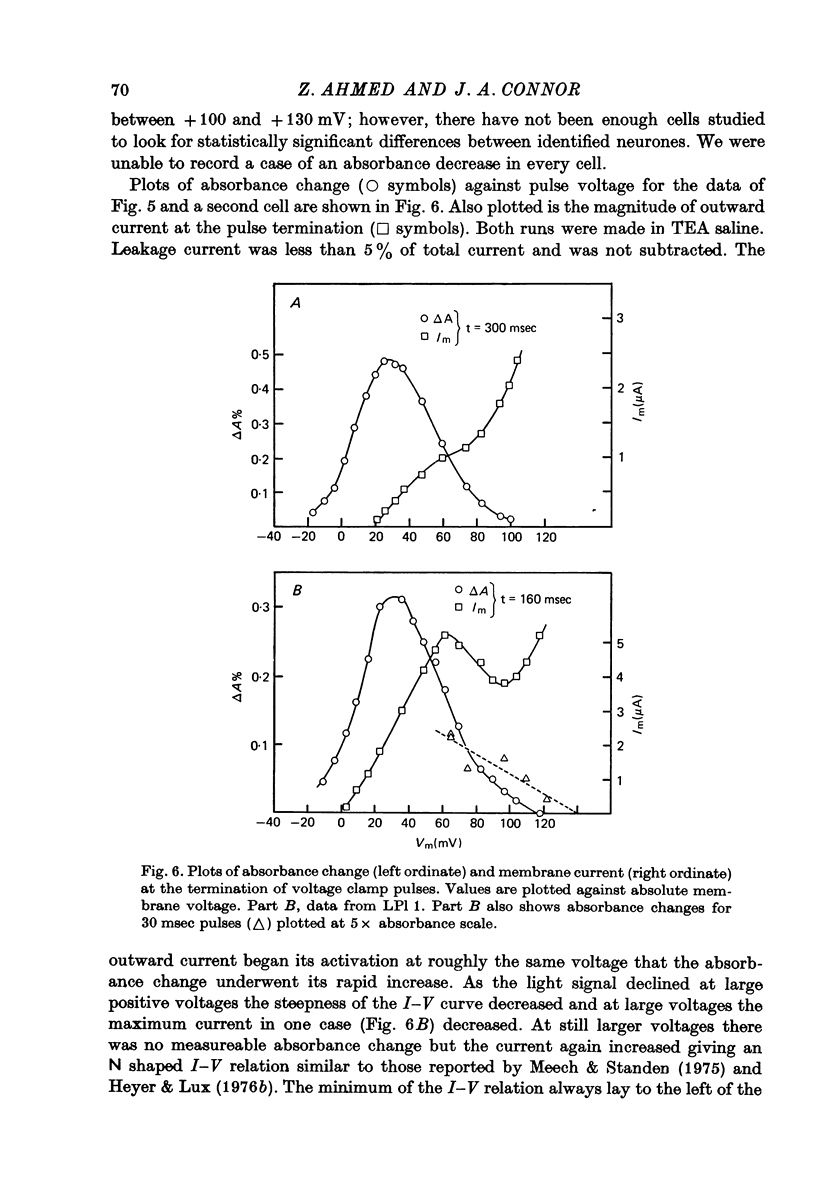
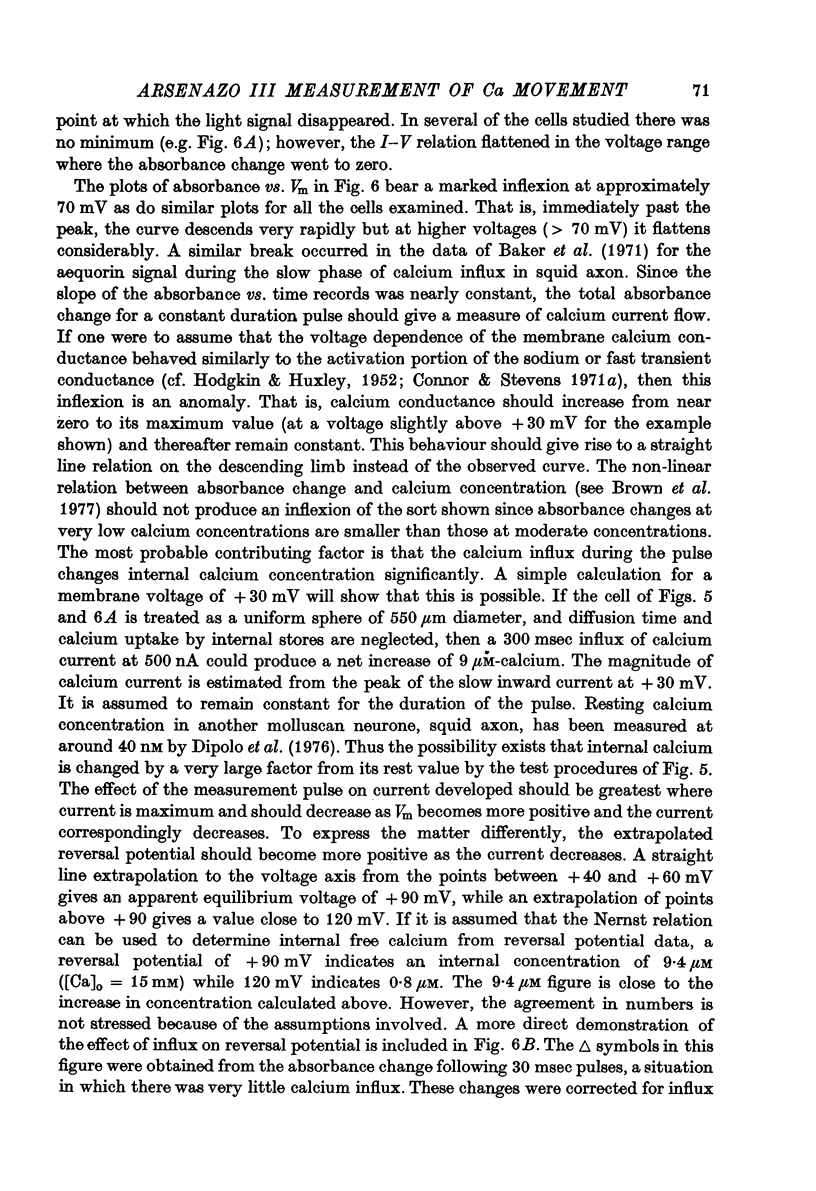
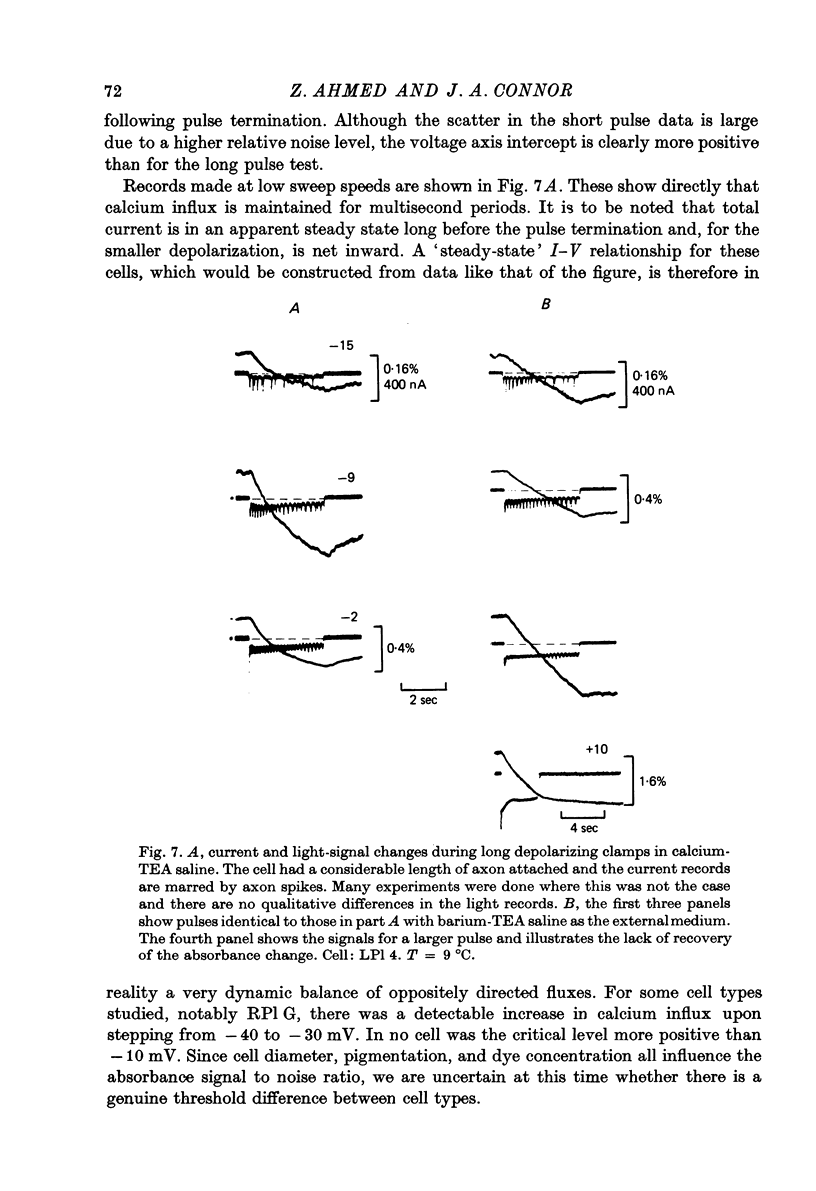
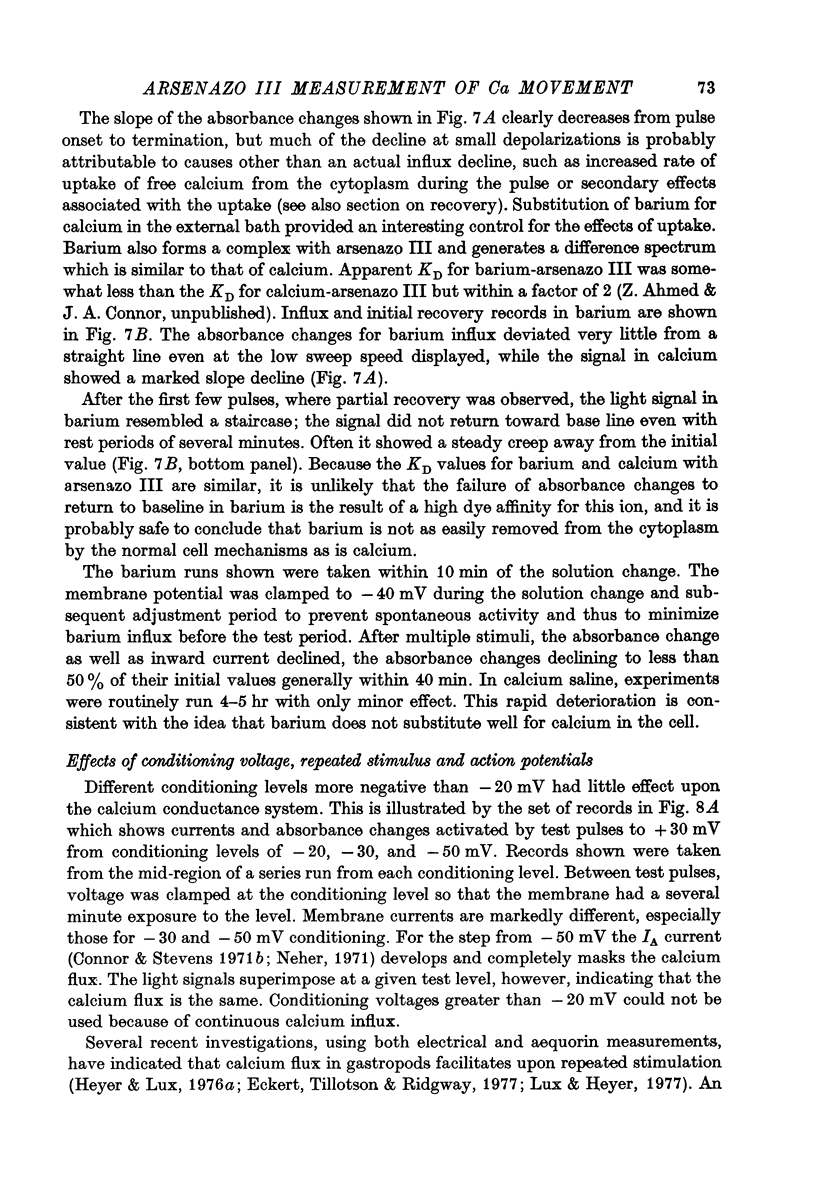
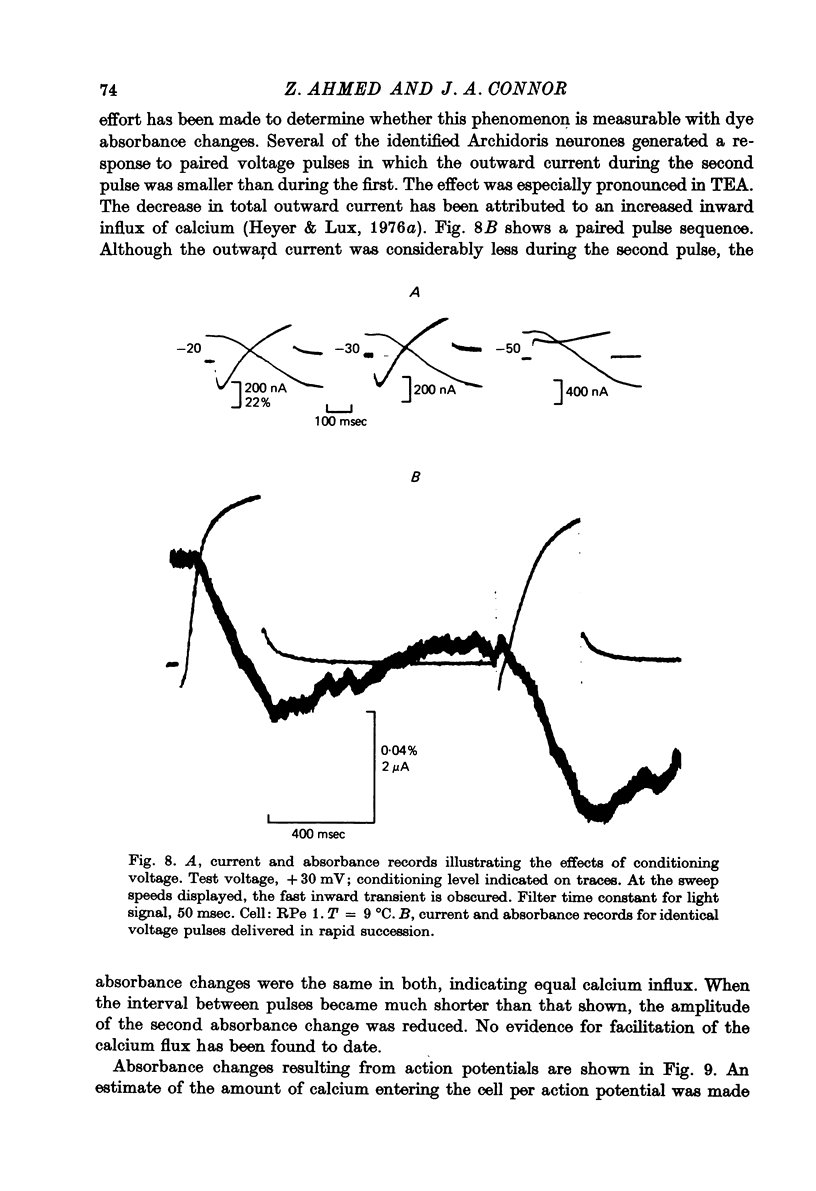
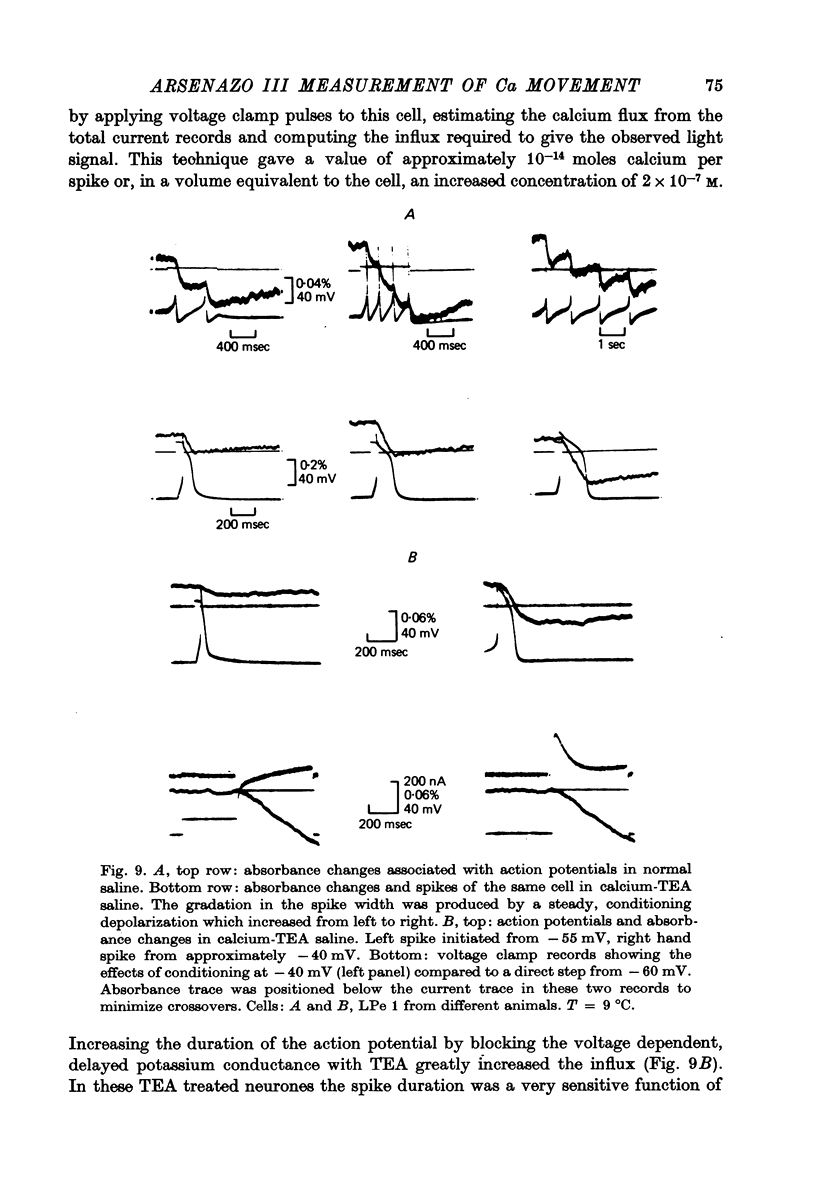
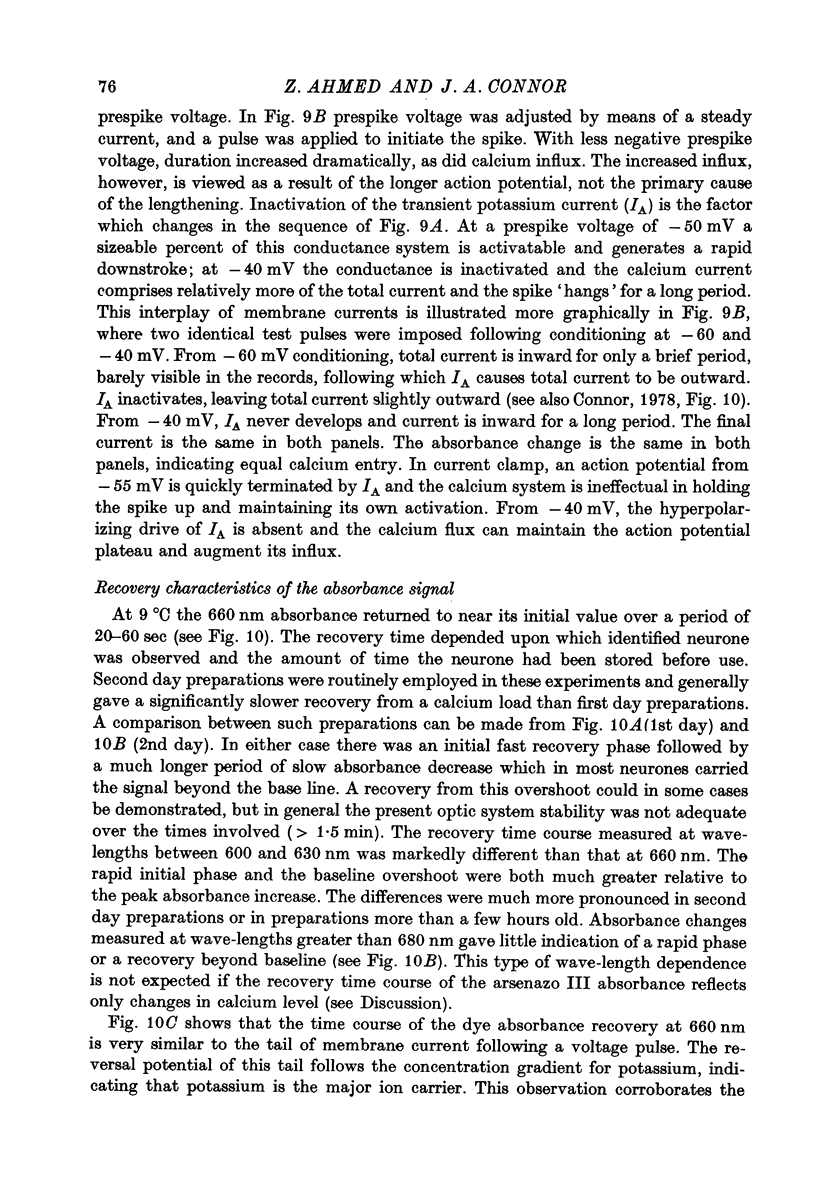
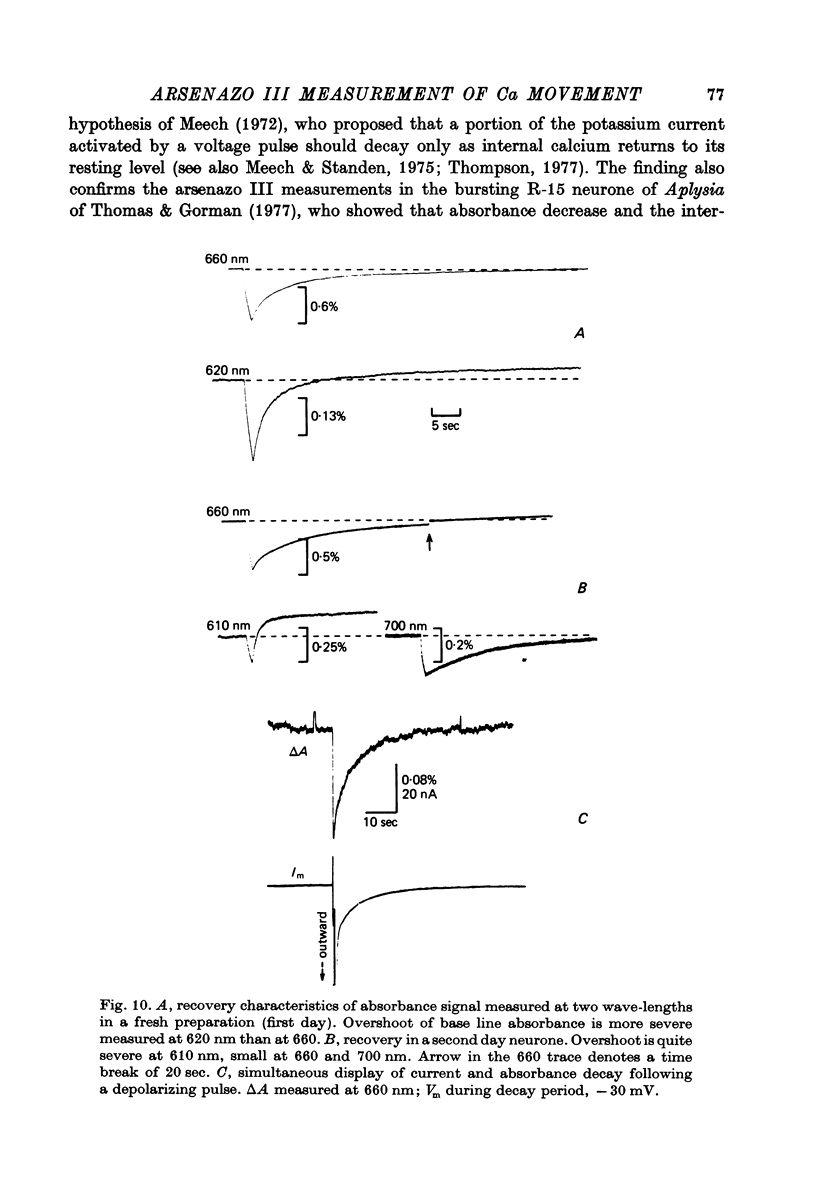
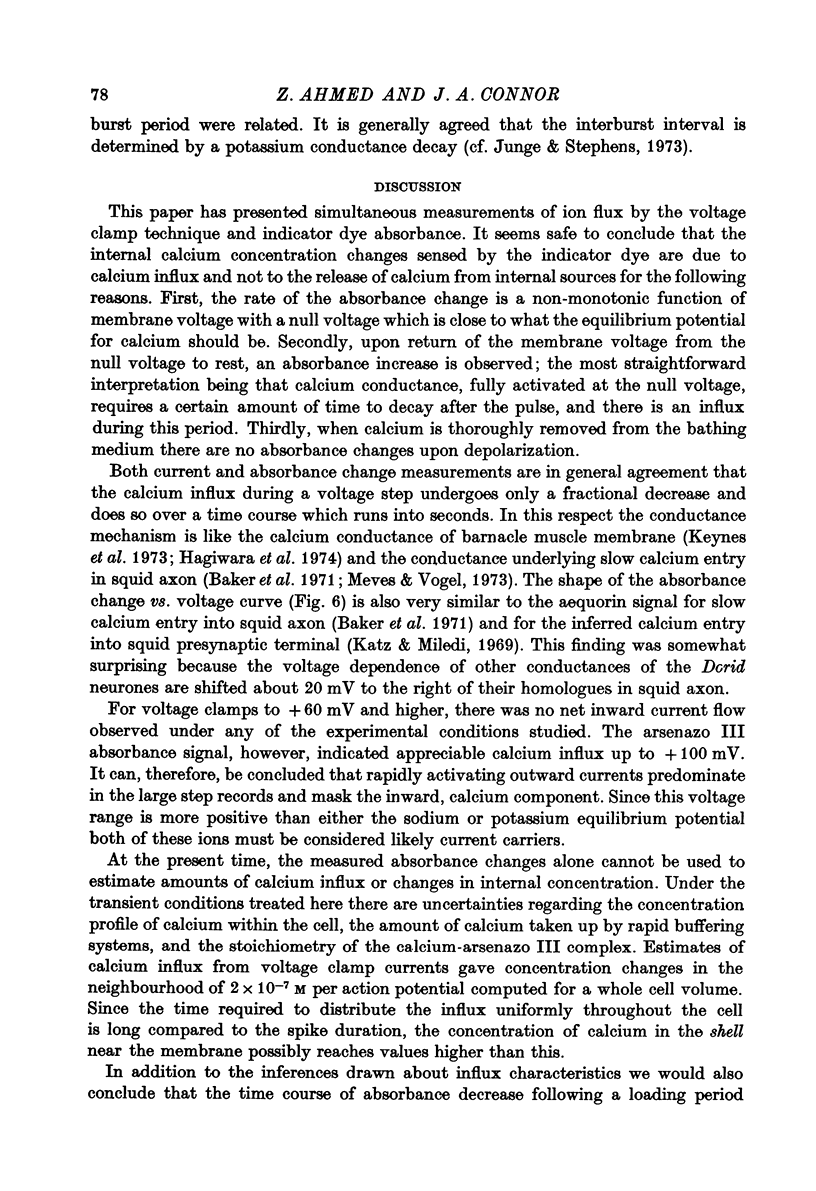
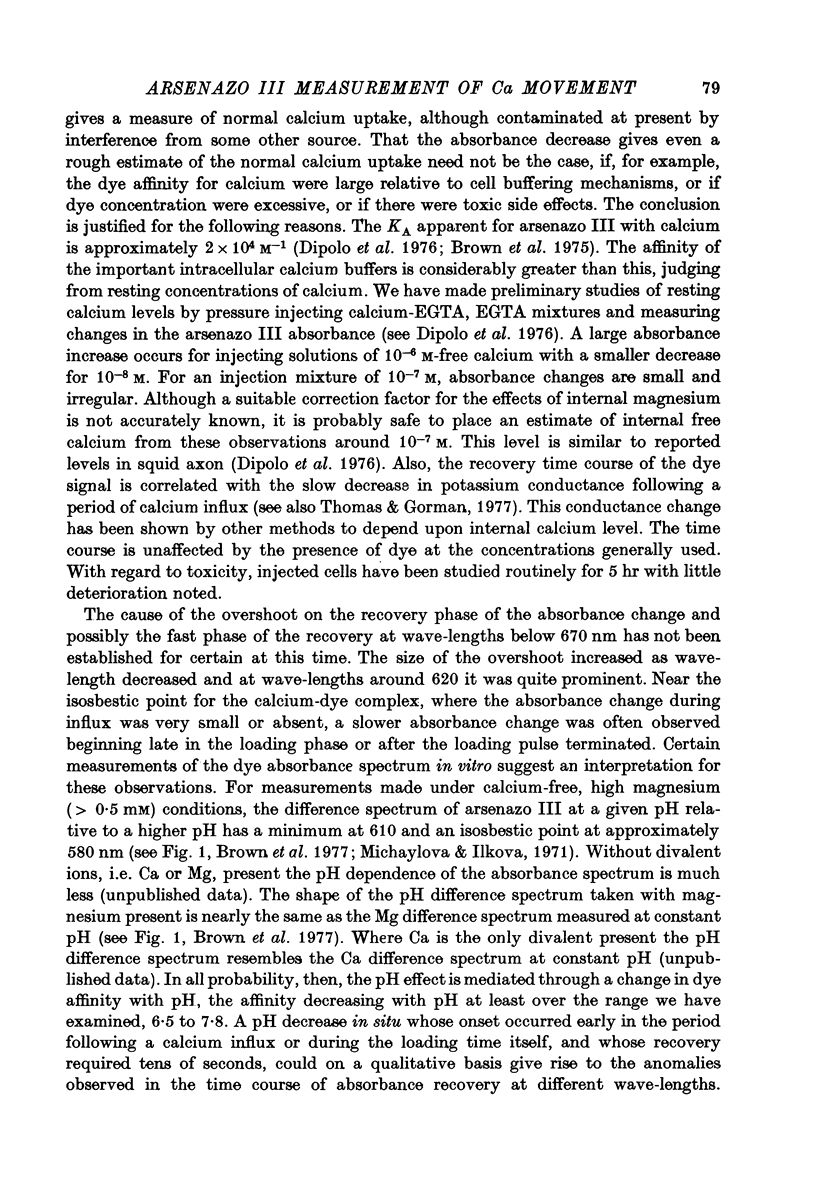
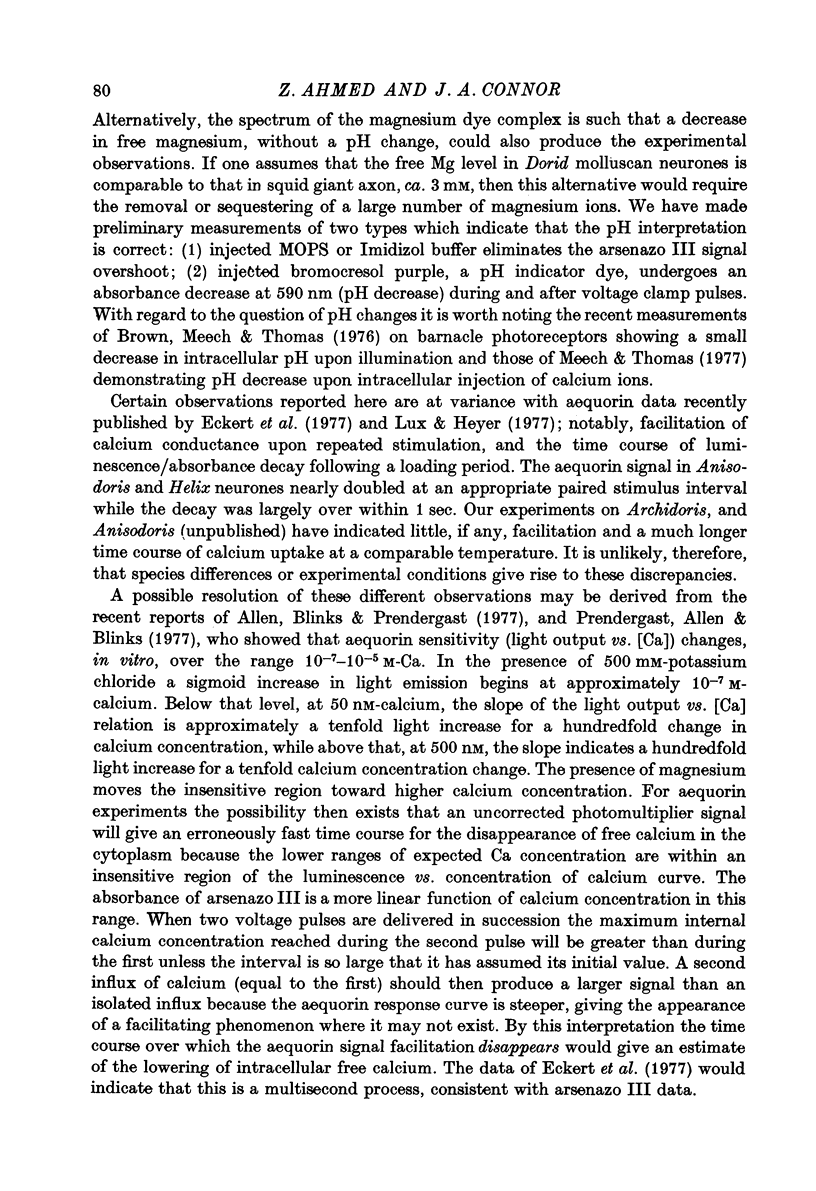
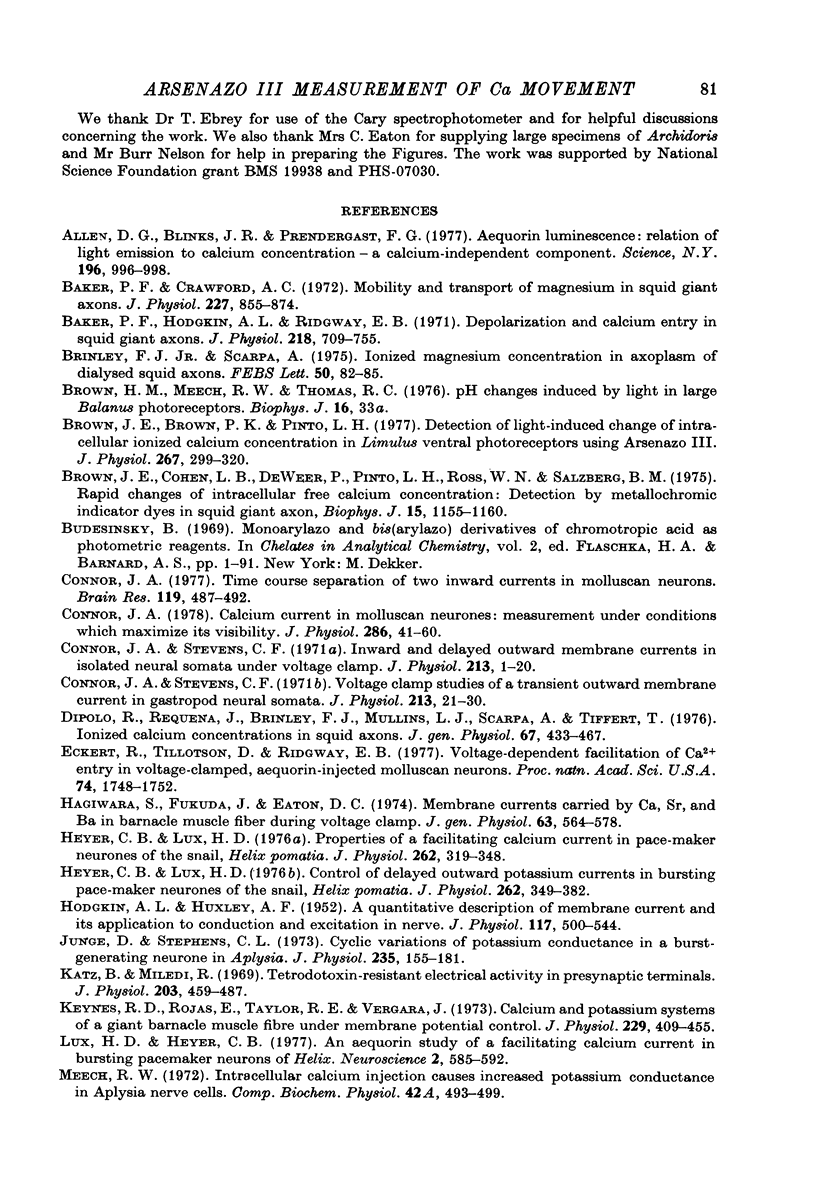
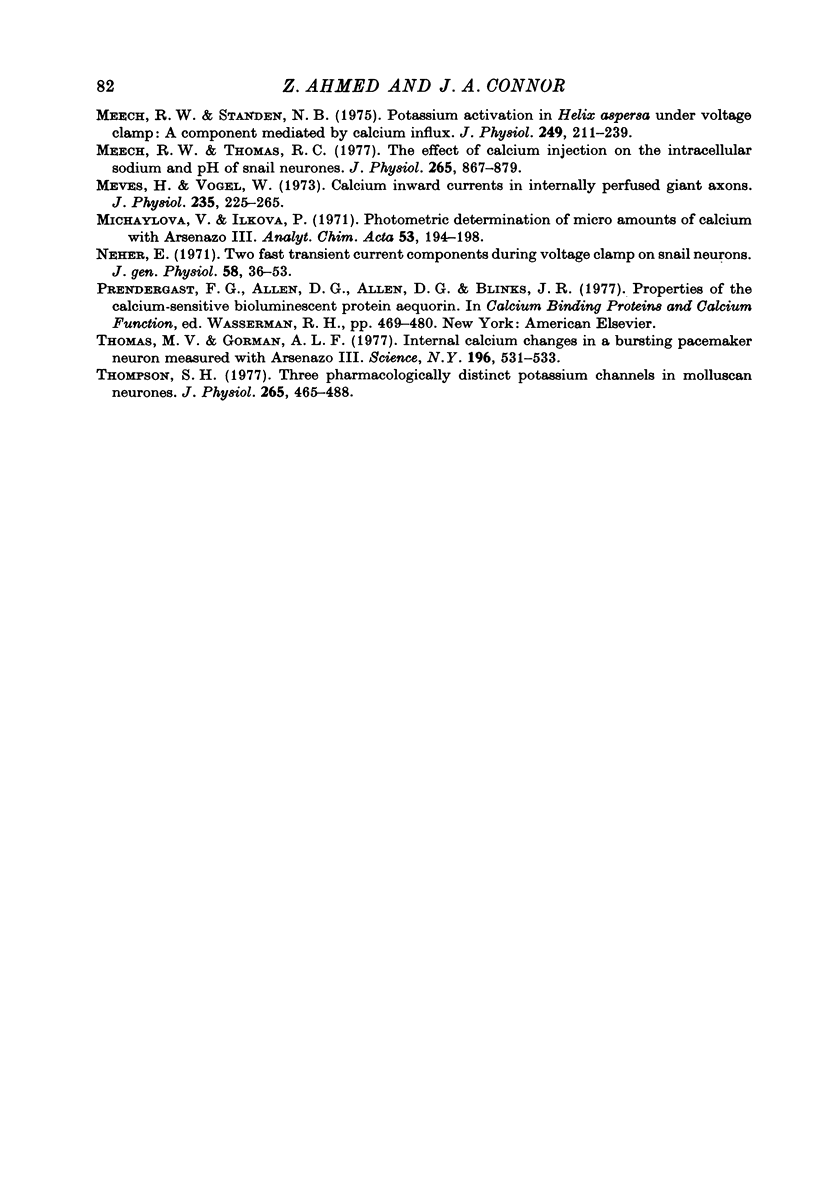
Selected References
These references are in PubMed. This may not be the complete list of references from this article.
- Allen D. G., Blinks J. R., Prendergast F. G. Aequorin luminescence: relation of light emission to calcium concentration--a calcium-independent component. Science. 1977 Mar 11;195(4282):996–998. doi: 10.1126/science.841325. [DOI] [PubMed] [Google Scholar]
- Baker P. F., Crawford A. C. Mobility and transport of magnesium in squid giant axons. J Physiol. 1972 Dec;227(3):855–874. doi: 10.1113/jphysiol.1972.sp010062. [DOI] [PMC free article] [PubMed] [Google Scholar]
- Baker P. F., Hodgkin A. L., Ridgway E. B. Depolarization and calcium entry in squid giant axons. J Physiol. 1971 Nov;218(3):709–755. doi: 10.1113/jphysiol.1971.sp009641. [DOI] [PMC free article] [PubMed] [Google Scholar]
- Brinley F. J., Jr, Scarpa A. Ionized magnesium concentration in axoplasm of dialyzed squid axons. FEBS Lett. 1975 Jan 15;50(1):82–85. doi: 10.1016/0014-5793(75)81046-5. [DOI] [PubMed] [Google Scholar]
- Brown J. E., Brown P. K., Pinto L. H. Detection of light-induced changes of intracellular ionized calcium concentration in Limulus ventral photoreceptors using arsenazo III. J Physiol. 1977 May;267(2):299–320. doi: 10.1113/jphysiol.1977.sp011814. [DOI] [PMC free article] [PubMed] [Google Scholar]
- Brown J. E., Cohen L. B., De Weer P., Pinto L. H., Ross W. N., Salzberg B. M. Rapid changes in intracellular free calcium concentration. Detection by metallochromic indicator dyes in squid giant axon. Biophys J. 1975 Nov;15(11):1155–1160. doi: 10.1016/S0006-3495(75)85891-7. [DOI] [PMC free article] [PubMed] [Google Scholar]
- Connor J. A. Calcium current in molluscan neurones: measurement under conditions which maximize its visibility. J Physiol. 1979 Jan;286:41–60. doi: 10.1113/jphysiol.1979.sp012606. [DOI] [PMC free article] [PubMed] [Google Scholar]
- Connor J. A., Stevens C. F. Voltage clamp studies of a transient outward membrane current in gastropod neural somata. J Physiol. 1971 Feb;213(1):21–30. doi: 10.1113/jphysiol.1971.sp009365. [DOI] [PMC free article] [PubMed] [Google Scholar]
- Connor J. A. Time course separation of two inward currents in molluscan neurons. Brain Res. 1977 Jan 7;119(2):487–492. doi: 10.1016/0006-8993(77)90330-4. [DOI] [PubMed] [Google Scholar]
- Dipolo R., Requena J., Brinley F. J., Jr, Mullins L. J., Scarpa A., Tiffert T. Ionized calcium concentrations in squid axons. J Gen Physiol. 1976 Apr;67(4):433–467. doi: 10.1085/jgp.67.4.433. [DOI] [PMC free article] [PubMed] [Google Scholar]
- Eckert R., Tillotson D., Ridgway E. B. Voltage-dependent facilitation of Ca2+ entry in voltage-clamped, aequorin-injected molluscan neurons. Proc Natl Acad Sci U S A. 1977 Apr;74(4):1748–1752. doi: 10.1073/pnas.74.4.1748. [DOI] [PMC free article] [PubMed] [Google Scholar]
- HODGKIN A. L., HUXLEY A. F. A quantitative description of membrane current and its application to conduction and excitation in nerve. J Physiol. 1952 Aug;117(4):500–544. doi: 10.1113/jphysiol.1952.sp004764. [DOI] [PMC free article] [PubMed] [Google Scholar]
- Hagiwara S., Fukuda J., Eaton D. C. Membrane currents carried by Ca, Sr, and Ba in barnacle muscle fiber during voltage clamp. J Gen Physiol. 1974 May;63(5):564–578. doi: 10.1085/jgp.63.5.564. [DOI] [PMC free article] [PubMed] [Google Scholar]
- Heyer C. B., Lux H. D. Control of the delayed outward potassium currents in bursting pace-maker neurones of the snail, Helix pomatia. J Physiol. 1976 Nov;262(2):349–382. doi: 10.1113/jphysiol.1976.sp011599. [DOI] [PMC free article] [PubMed] [Google Scholar]
- Heyer C. B., Lux H. D. Properties of a facilitating calcium current in pace-maker neurones of the snail, Helix pomatia. J Physiol. 1976 Nov;262(2):319–348. doi: 10.1113/jphysiol.1976.sp011598. [DOI] [PMC free article] [PubMed] [Google Scholar]
- Junge D., Stephens C. L. Cyclic variation of potassium conductance in a burst-generating neurone in Aplysia. J Physiol. 1973 Nov;235(1):155–181. doi: 10.1113/jphysiol.1973.sp010382. [DOI] [PMC free article] [PubMed] [Google Scholar]
- Katz B., Miledi R. Tetrodotoxin-resistant electric activity in presynaptic terminals. J Physiol. 1969 Aug;203(2):459–487. doi: 10.1113/jphysiol.1969.sp008875. [DOI] [PMC free article] [PubMed] [Google Scholar]
- Keynes R. D., Rojas E., Taylor R. E., Vergara J. Calcium and potassium systems of a giant barnacle muscle fibre under membrane potential control. J Physiol. 1973 Mar;229(2):409–455. doi: 10.1113/jphysiol.1973.sp010146. [DOI] [PMC free article] [PubMed] [Google Scholar]
- Lux H. D., Heyer C. B. An aequorin study of a facilitating calcium current in bursting pacemaker neurons of Helix. Neuroscience. 1977;2(4):585–592. doi: 10.1016/0306-4522(77)90054-9. [DOI] [PubMed] [Google Scholar]
- Meech R. W. Intracellular calcium injection causes increased potassium conductance in Aplysia nerve cells. Comp Biochem Physiol A Comp Physiol. 1972 Jun 1;42(2):493–499. doi: 10.1016/0300-9629(72)90128-4. [DOI] [PubMed] [Google Scholar]
- Meech R. W., Standen N. B. Potassium activation in Helix aspersa neurones under voltage clamp: a component mediated by calcium influx. J Physiol. 1975 Jul;249(2):211–239. doi: 10.1113/jphysiol.1975.sp011012. [DOI] [PMC free article] [PubMed] [Google Scholar]
- Meech R. W., Thomas R. C. The effect of calcium injection on the intracellular sodium and pH of snail neurones. J Physiol. 1977 Mar;265(3):867–879. doi: 10.1113/jphysiol.1977.sp011749. [DOI] [PMC free article] [PubMed] [Google Scholar]
- Meves H., Vogel W. Calcium inward currents in internally perfused giant axons. J Physiol. 1973 Nov;235(1):225–265. doi: 10.1113/jphysiol.1973.sp010386. [DOI] [PMC free article] [PubMed] [Google Scholar]
- Neher E. Two fast transient current components during voltage clamp on snail neurons. J Gen Physiol. 1971 Jul;58(1):36–53. doi: 10.1085/jgp.58.1.36. [DOI] [PMC free article] [PubMed] [Google Scholar]
- Thomas M. V., Gorman A. L. Internal calcium changes in a bursting pacemaker neuron measured with arsenazo III. Science. 1977 Apr 29;196(4289):531–533. doi: 10.1126/science.850795. [DOI] [PubMed] [Google Scholar]
- Thompson S. H. Three pharmacologically distinct potassium channels in molluscan neurones. J Physiol. 1977 Feb;265(2):465–488. doi: 10.1113/jphysiol.1977.sp011725. [DOI] [PMC free article] [PubMed] [Google Scholar]