Abstract
1. Application of voltage clamp pulses (1--10 sec) to frog ventricular strips causes temporary changes in the extracellular K concentration. 2. The changes in the extracellular K concentration can be estimated from (a) slowly decaying post-clamp after-potentials, (b) changes in the action potential duration, and (c) measurements with a K-selective micro-electrode. 3. The depolarization of the resting potential and the shortening of the action potential are present in approximately the same proportions during voltage-clamp induced extracellular K accumulation and during perfusion with a K-ricn Ringer solution but small consistent differences are noticed. 4. The measurements of the after-potential, the action potential shortening, and the K-electrode response were analysed as indicators of extracellular K+ activity and it was concluded that the after-potential provides the most convenient and reliable estimate of the absolute magnitude of the voltage-clamp induced extracellular K accumulation. 5. The depolarizing after-potentials decay more slowly than the hyperpolarizing after-potentials but it is found that this reflects the selectivity of the membrane to K+ concentrations as predicted by the Nernst or the Goldman equations. 6. Analysis of the redistribution of accumulated K+ from the decay of the after-potential suggests that the major part of the redistribution process can be described by a single time constant (2--4 sec). A much longer time constant is required for a smaller component of the 'tail' in order to bring [K]o to the normal resting state. 7. N-shaped relations similar to the 'steady state' current-voltage relation are obtained when the post-clamp after-potential, the action potential shortening, and the K-electrode response are plotted versus the clamped membrane potential. The maxima of these curves are located around -40 mV and the minima around -20 mV. 8. In spite of a significant outward membrane current (1--1.5 microamperemeter) in the minimum region (-20 mV), the post-clamp after-potential is often hyperpolarizing in nature suggesting extracellular K depletion. 9. These findings indicate that the K efflux is lower at -20 mV than at both higher and lower potentials and suggest that the N-shape 'steady state' current-voltage relation mainly reflects the voltage dependency of the K current. 10. A theory for K accumulation in a single compartment is presented which predicts that a simple linear RC-circuit may describe the electrical response of the preparation in a limited potential range around the resting potential. The extracellular accumulation space was estimated to be 13--16% of the total volume of the preparation. It is tentatively suggested that the accumulation space is equivalent to the subendothelial fraction of the extracellular space.
Full text
PDF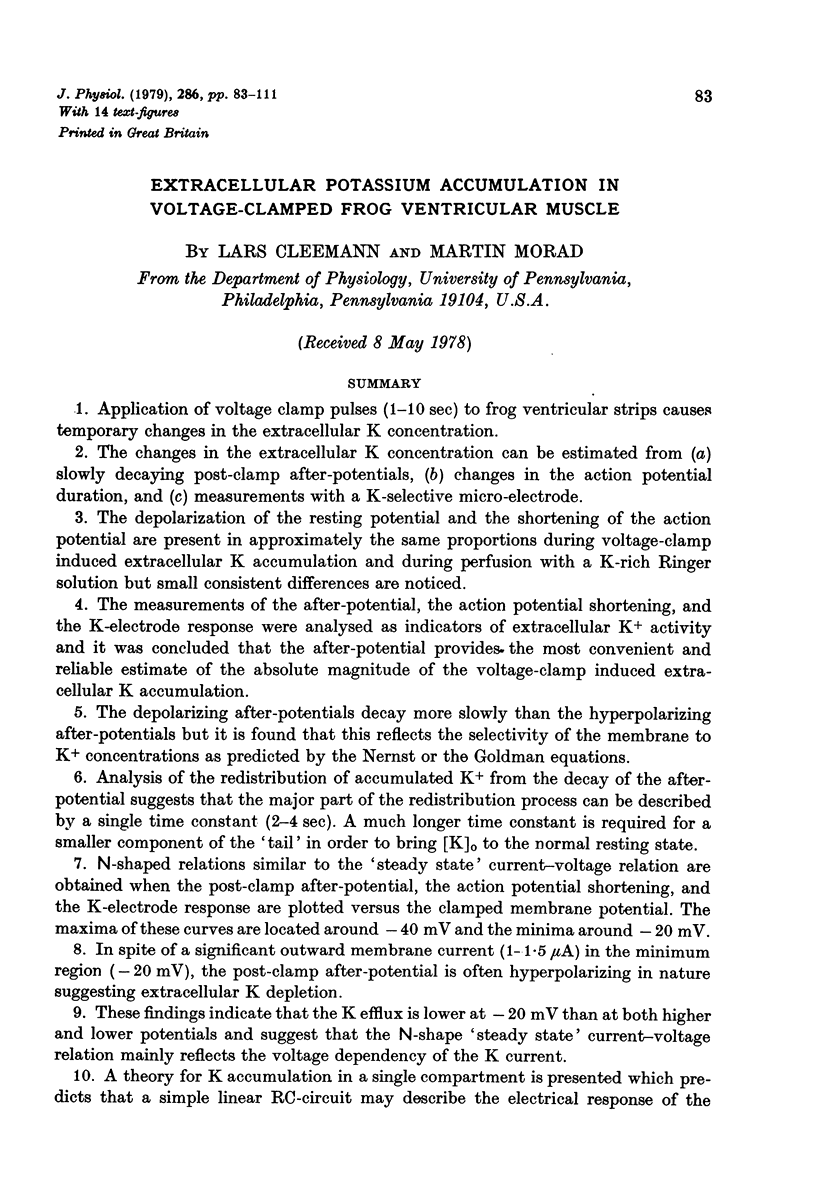
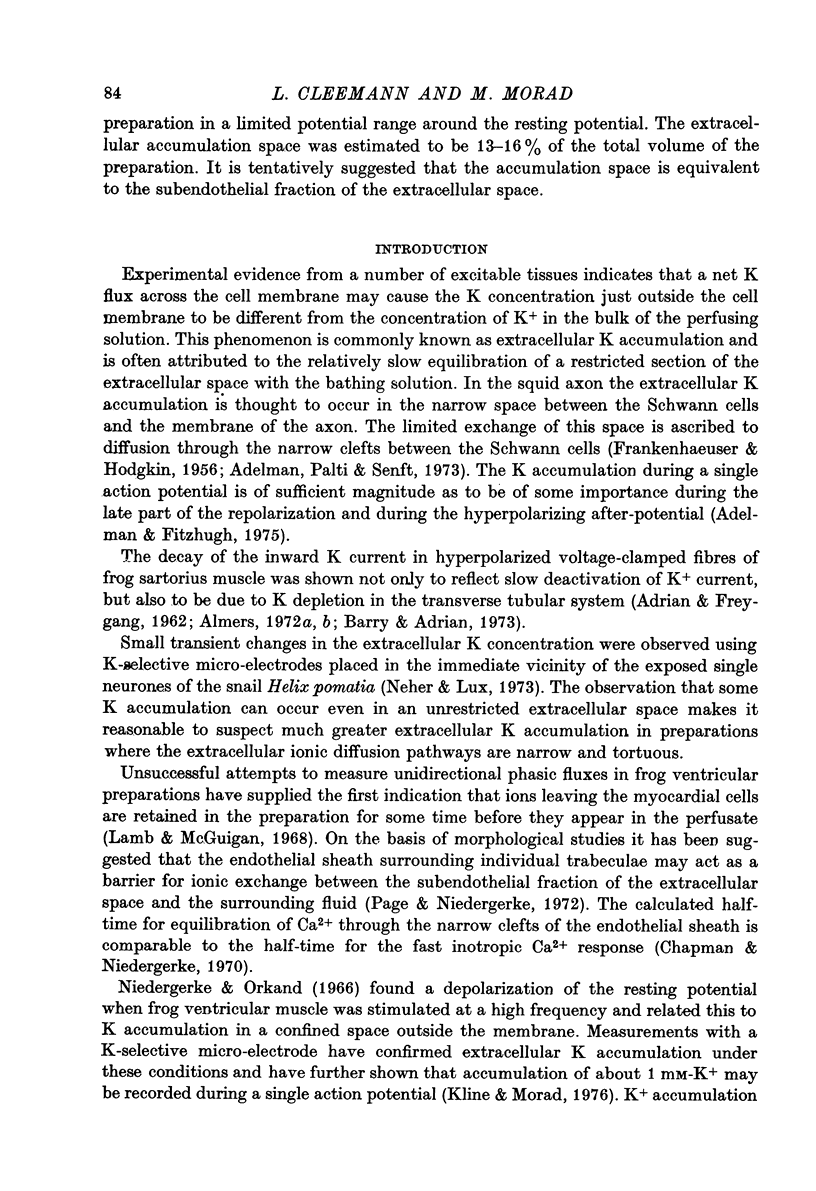
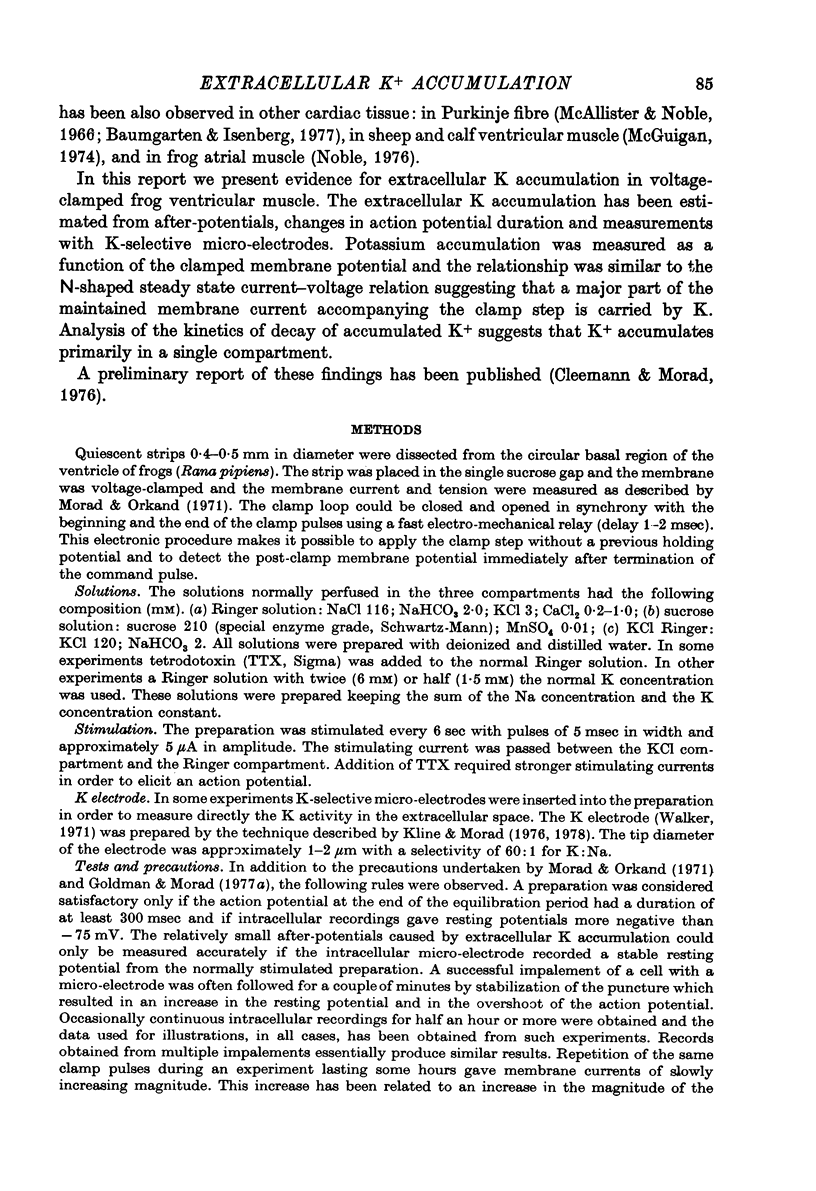
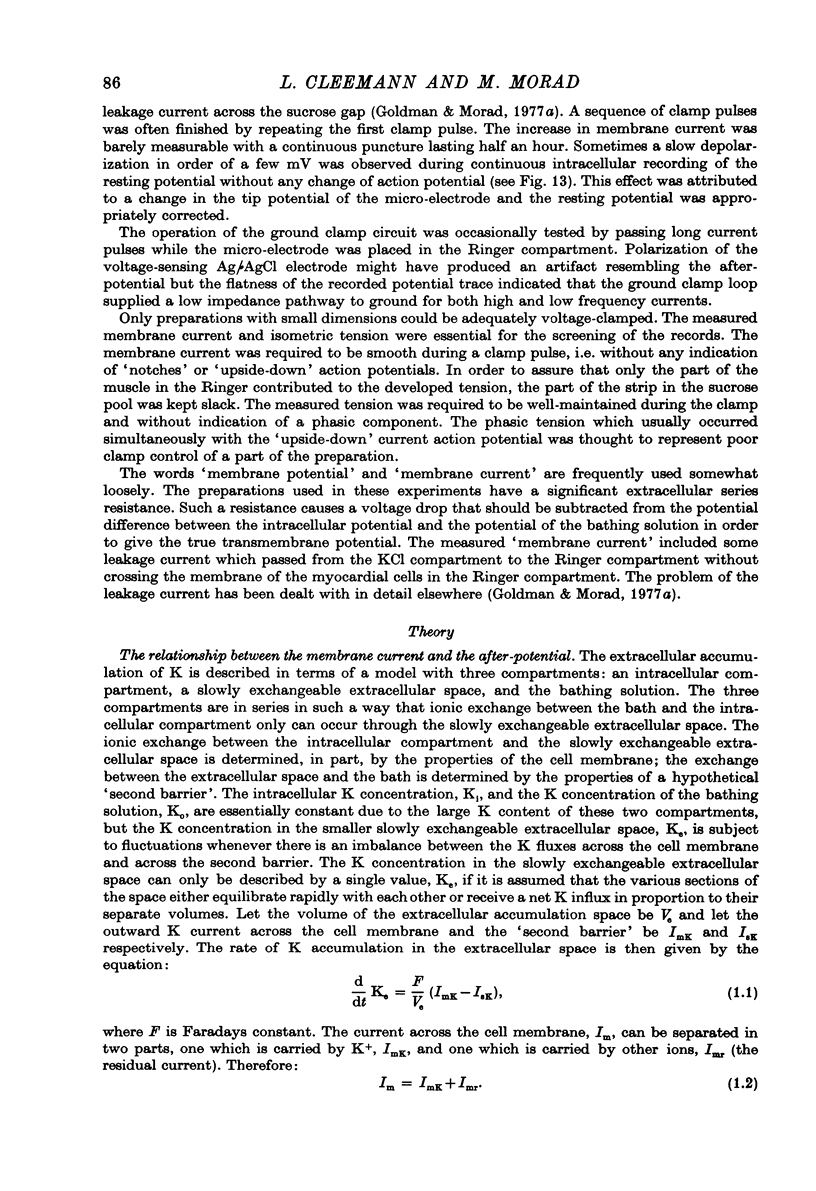
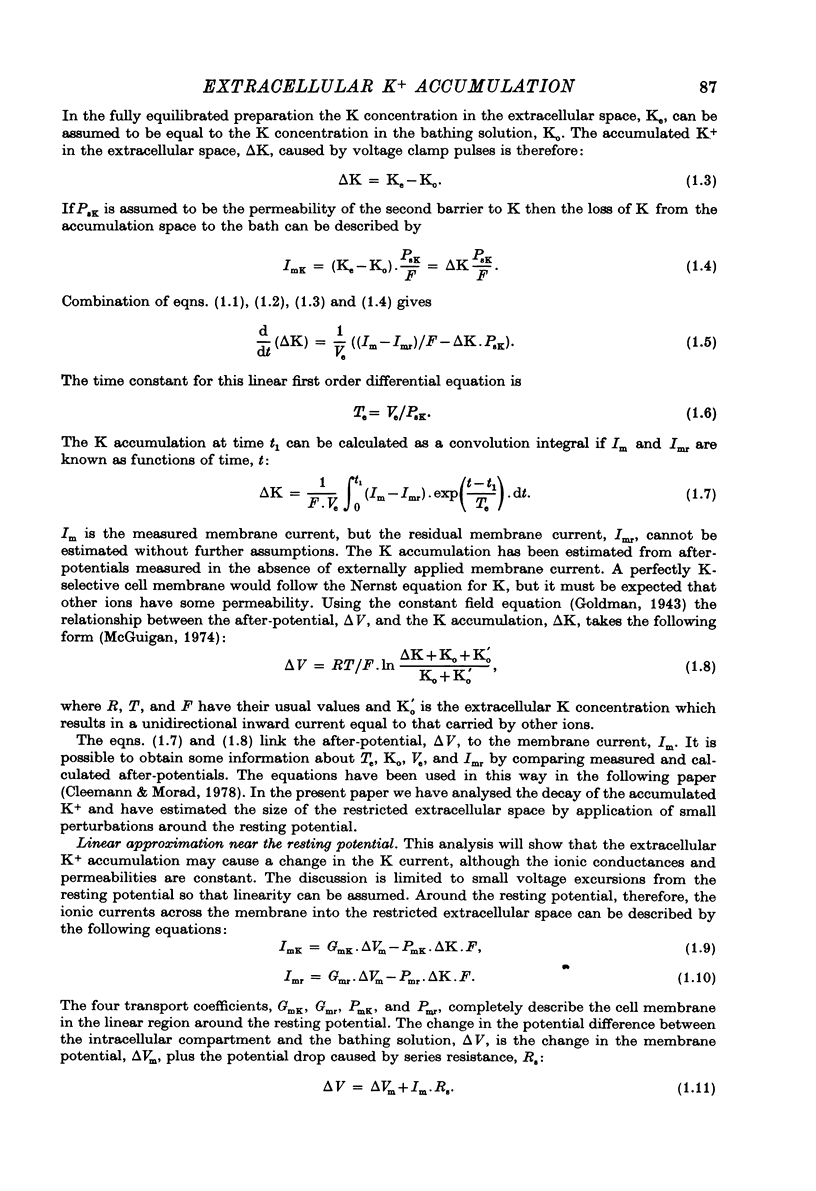
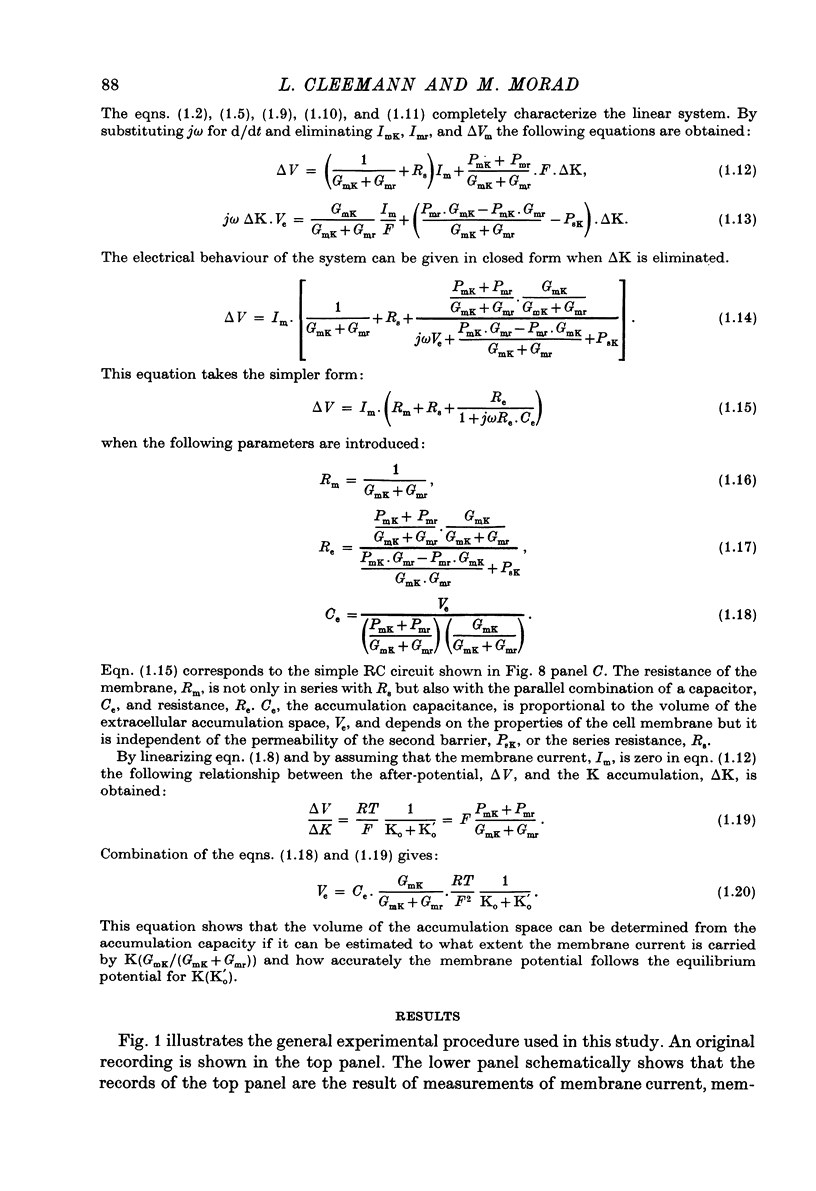
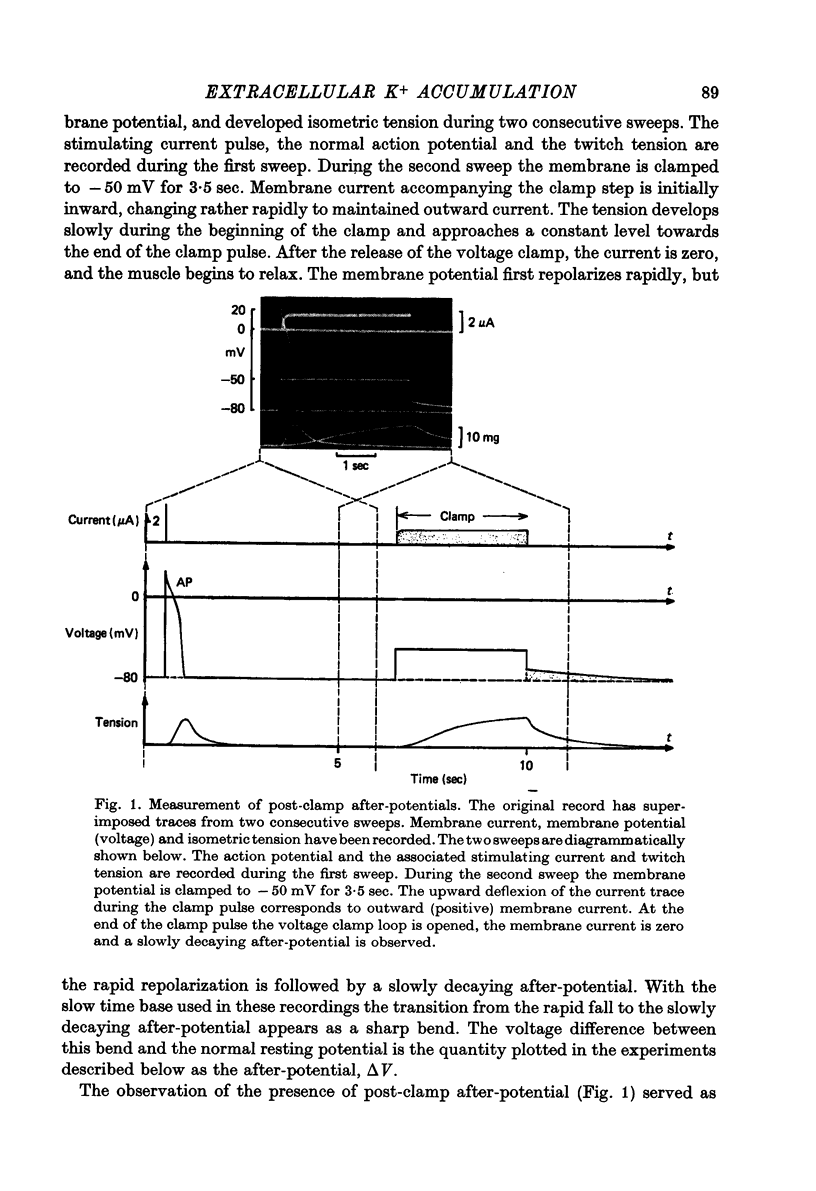
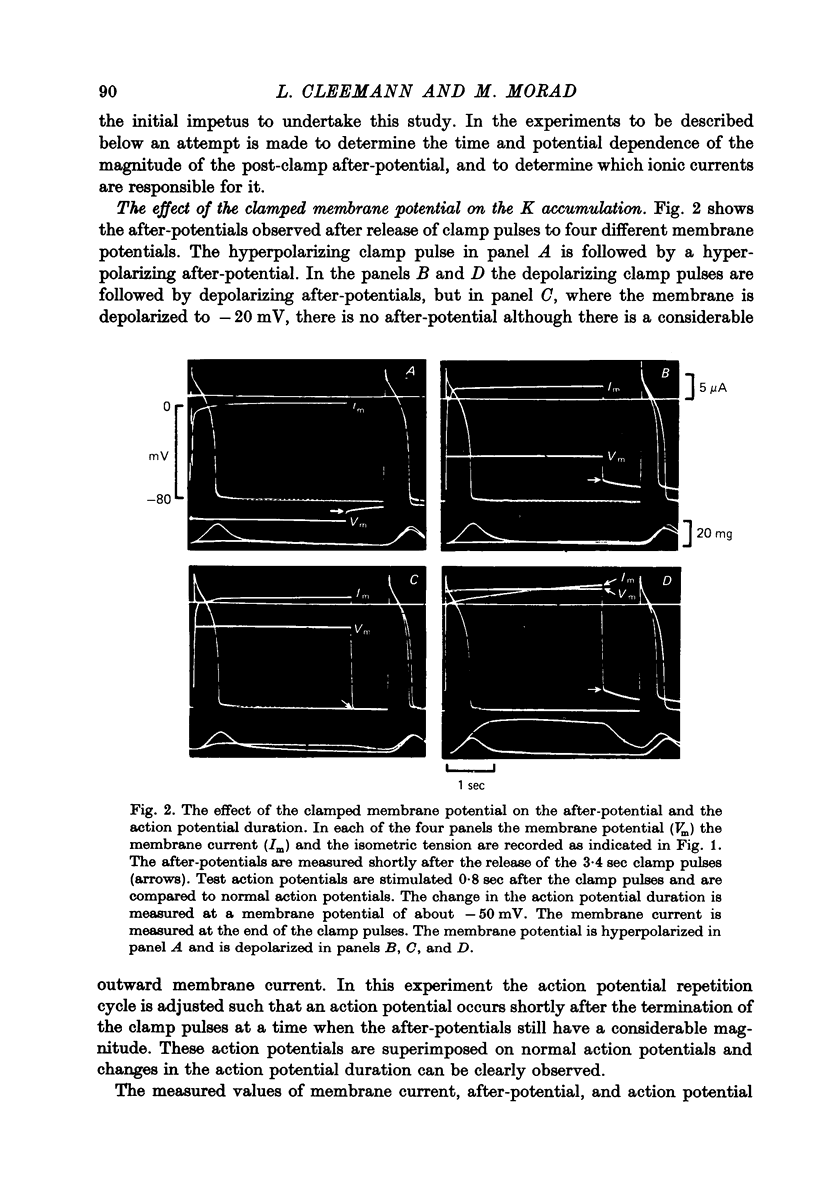
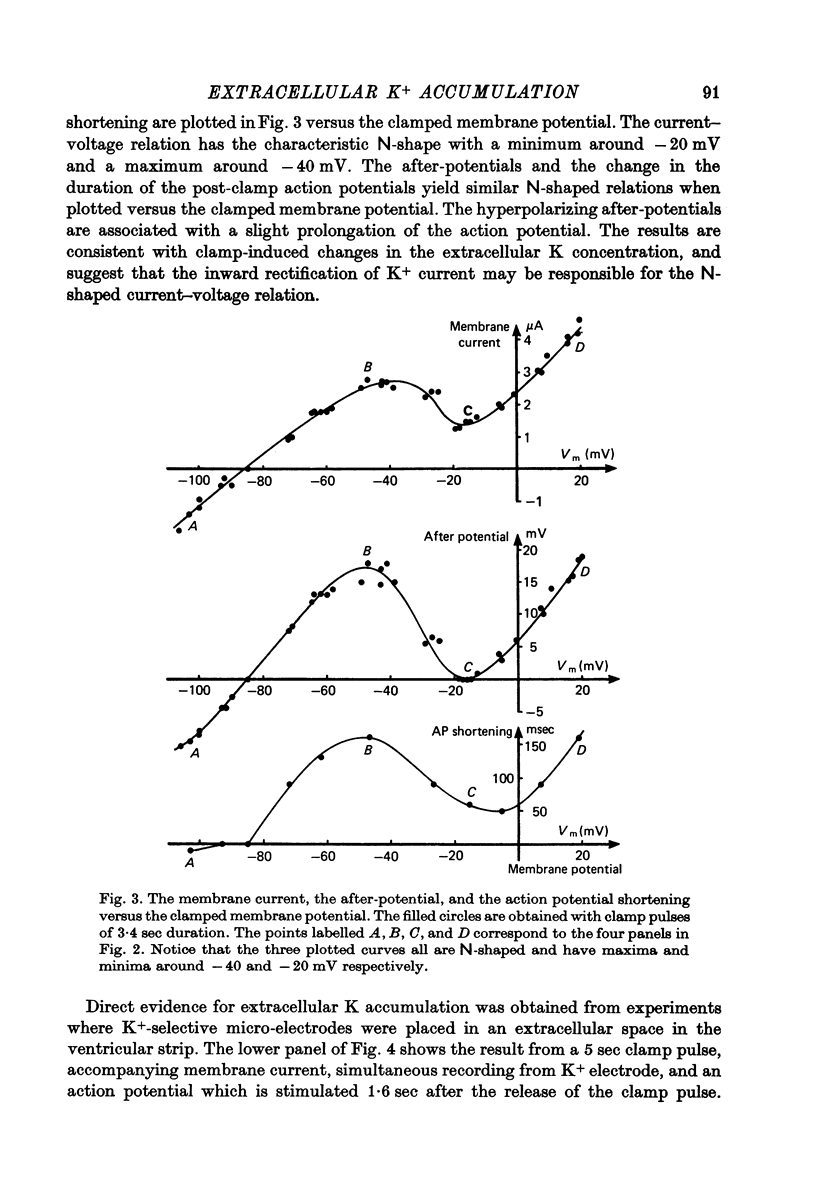
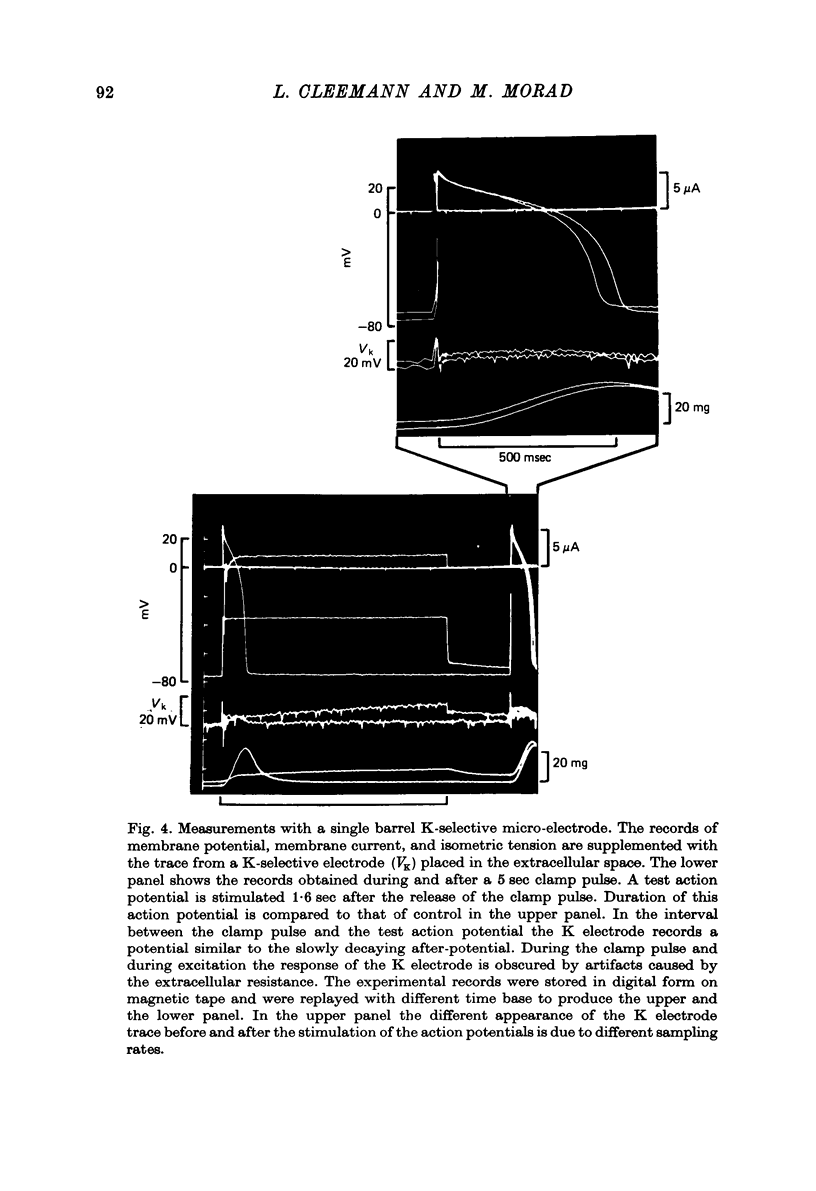
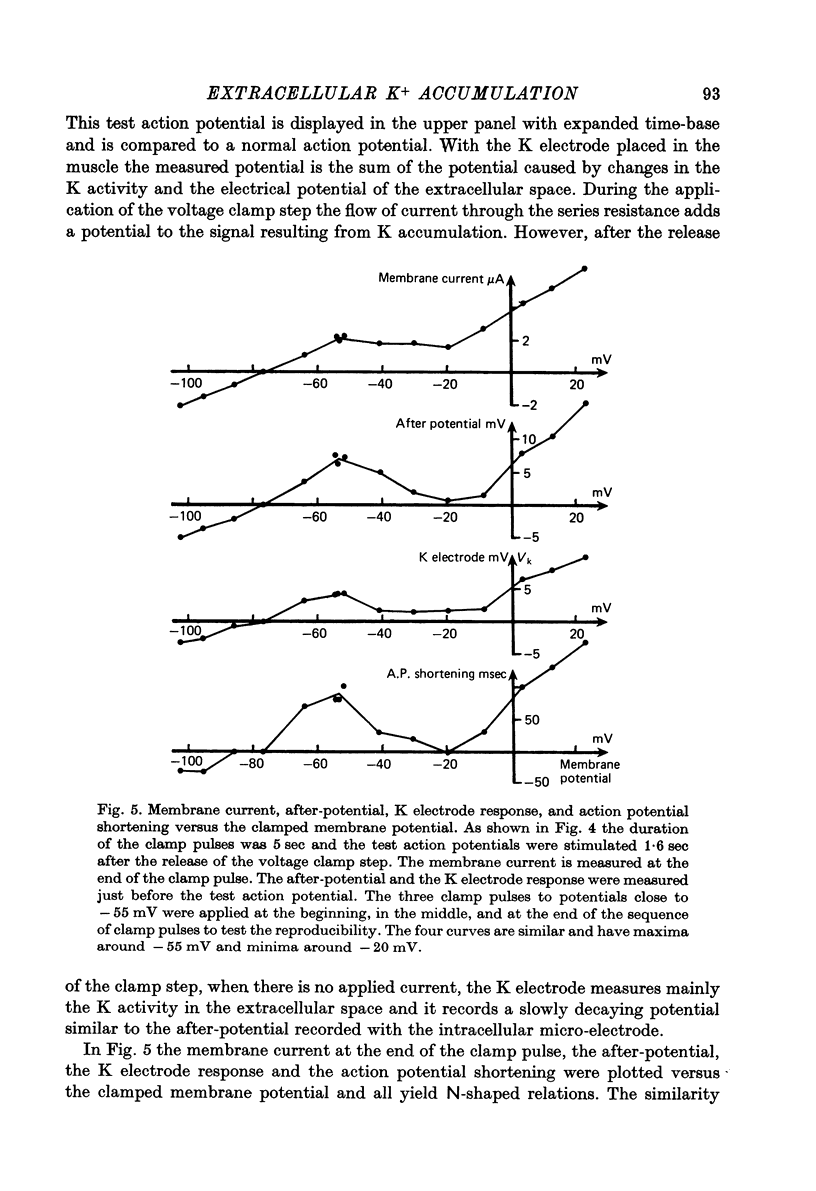
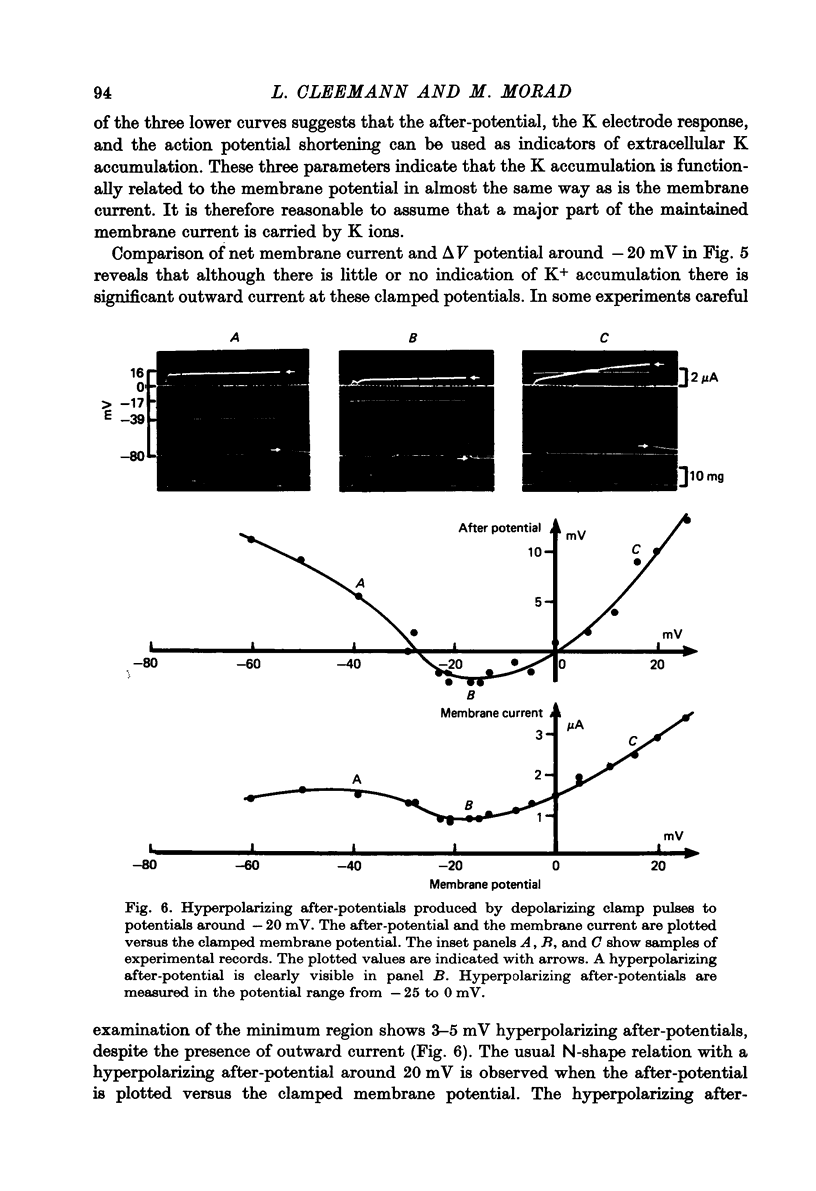
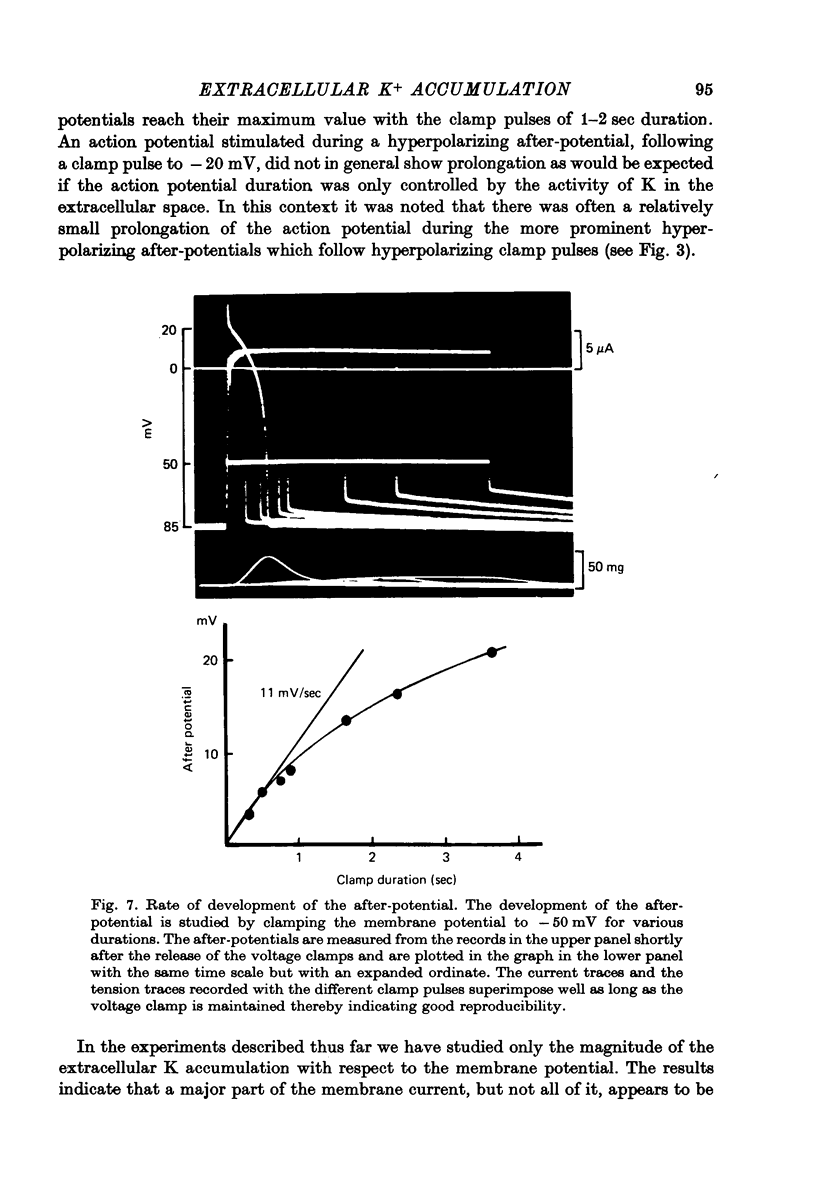
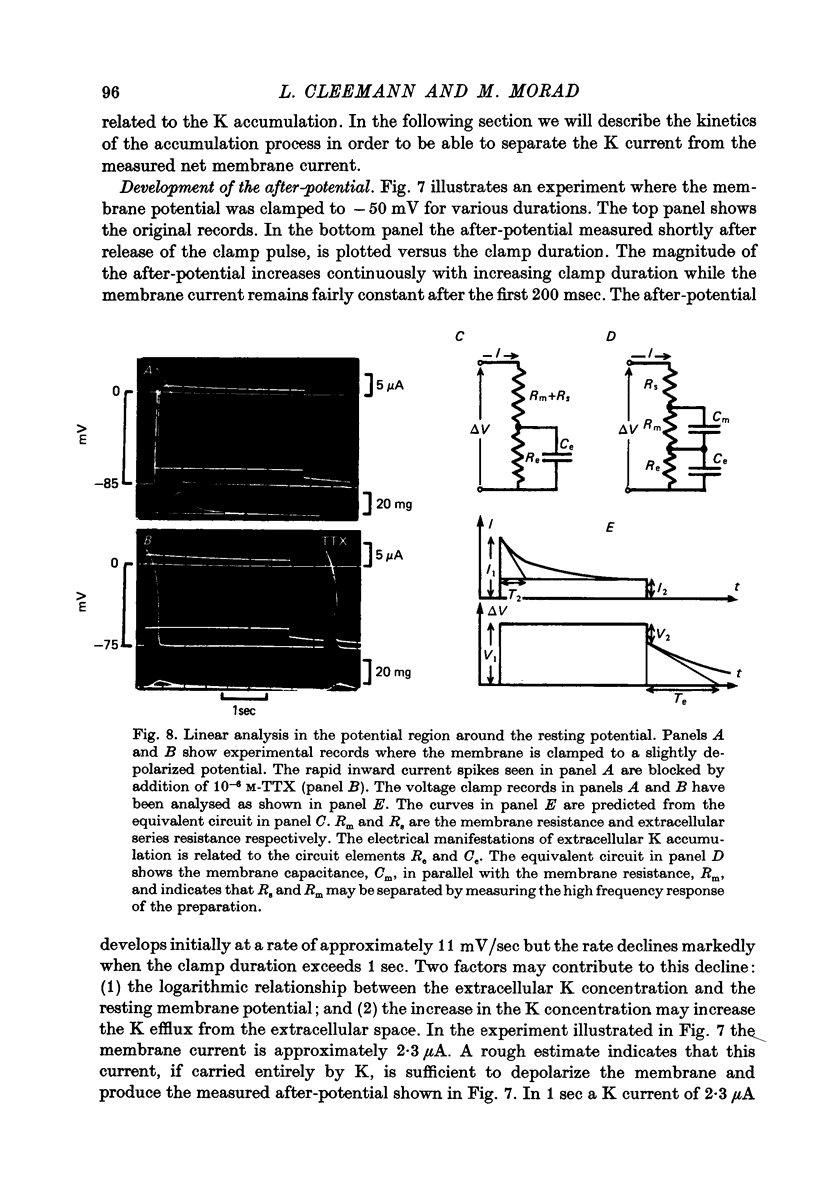
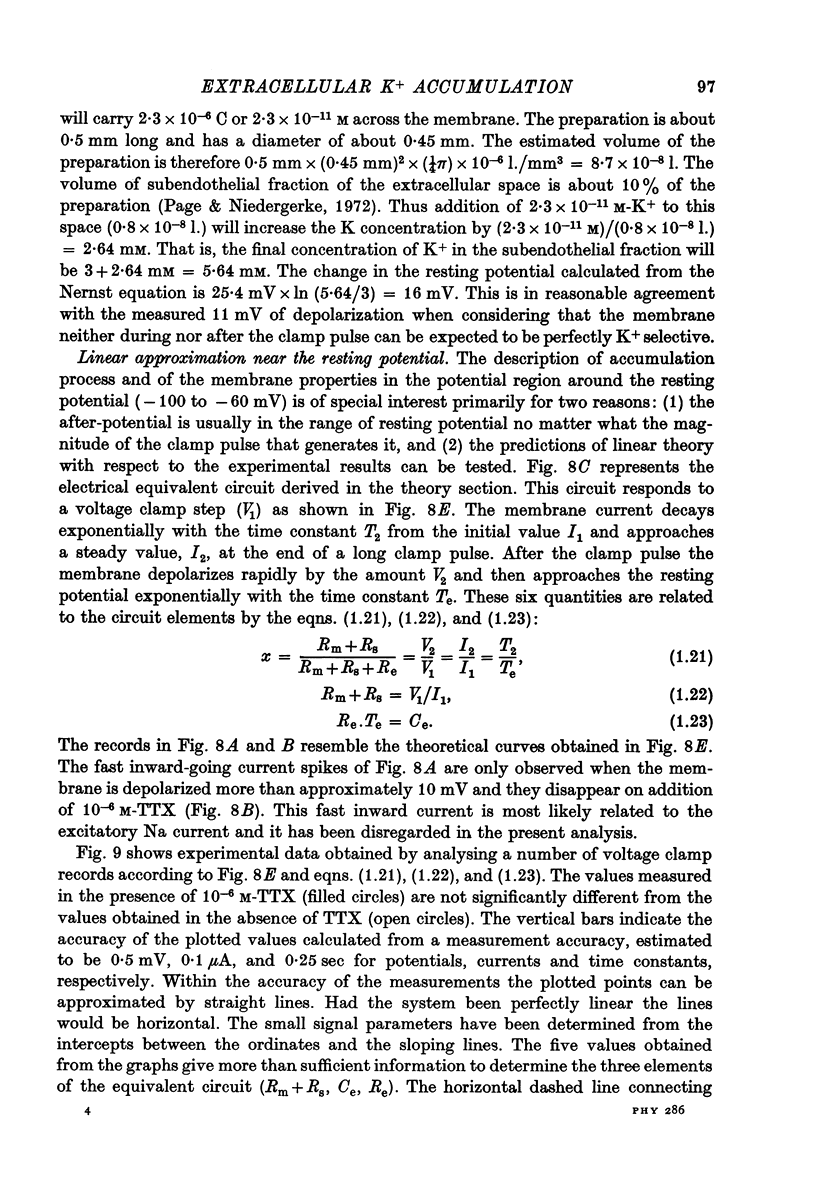
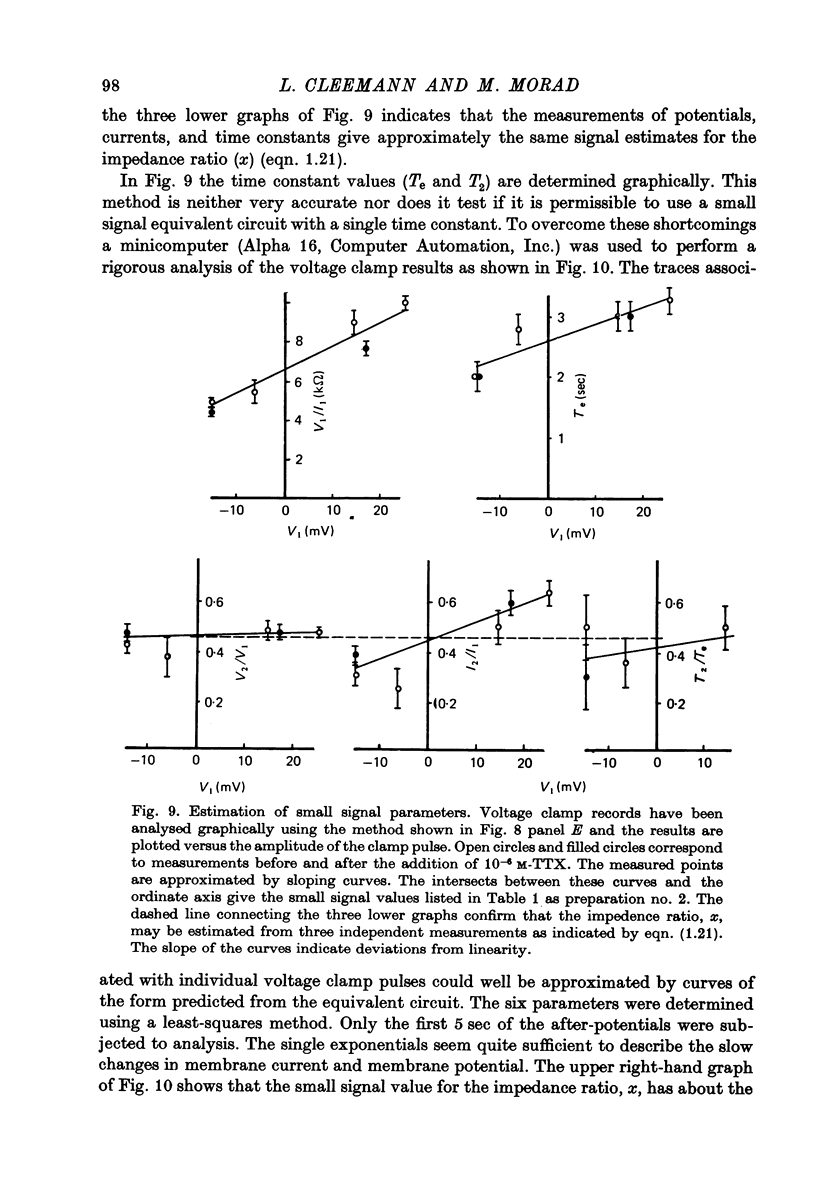
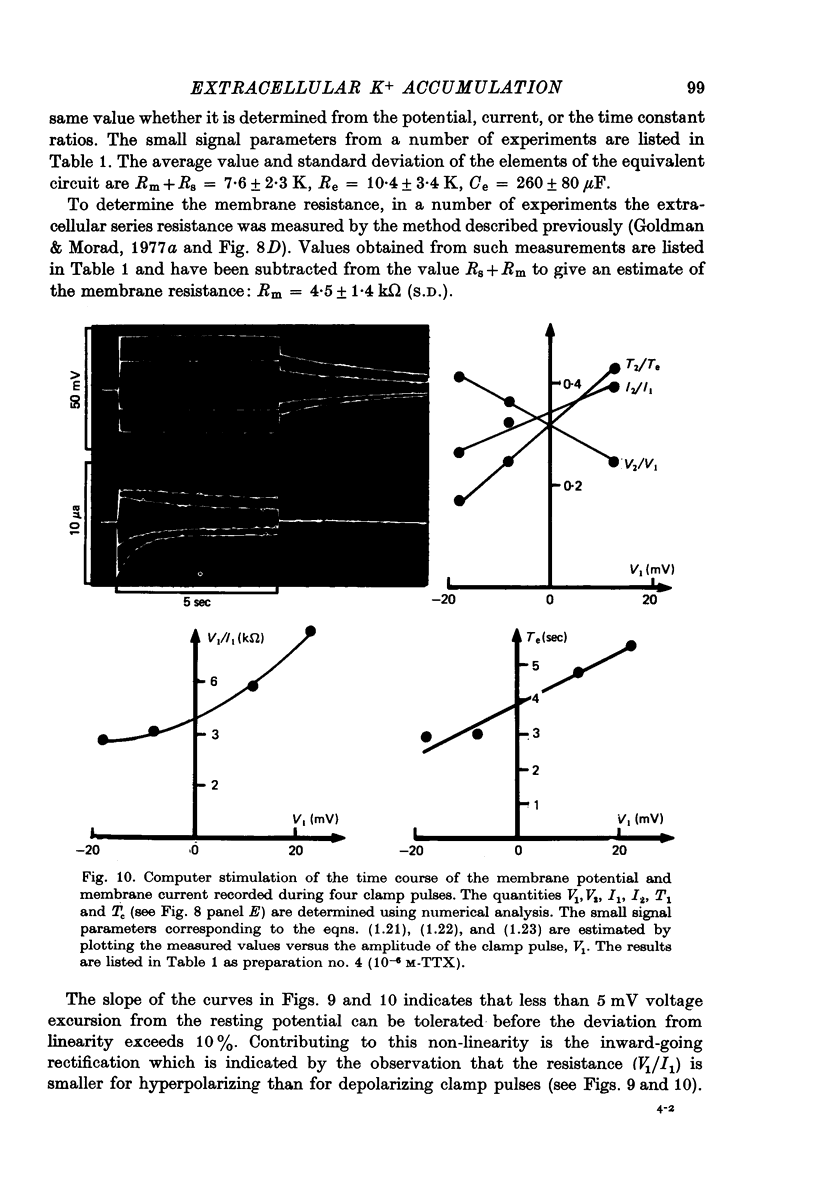
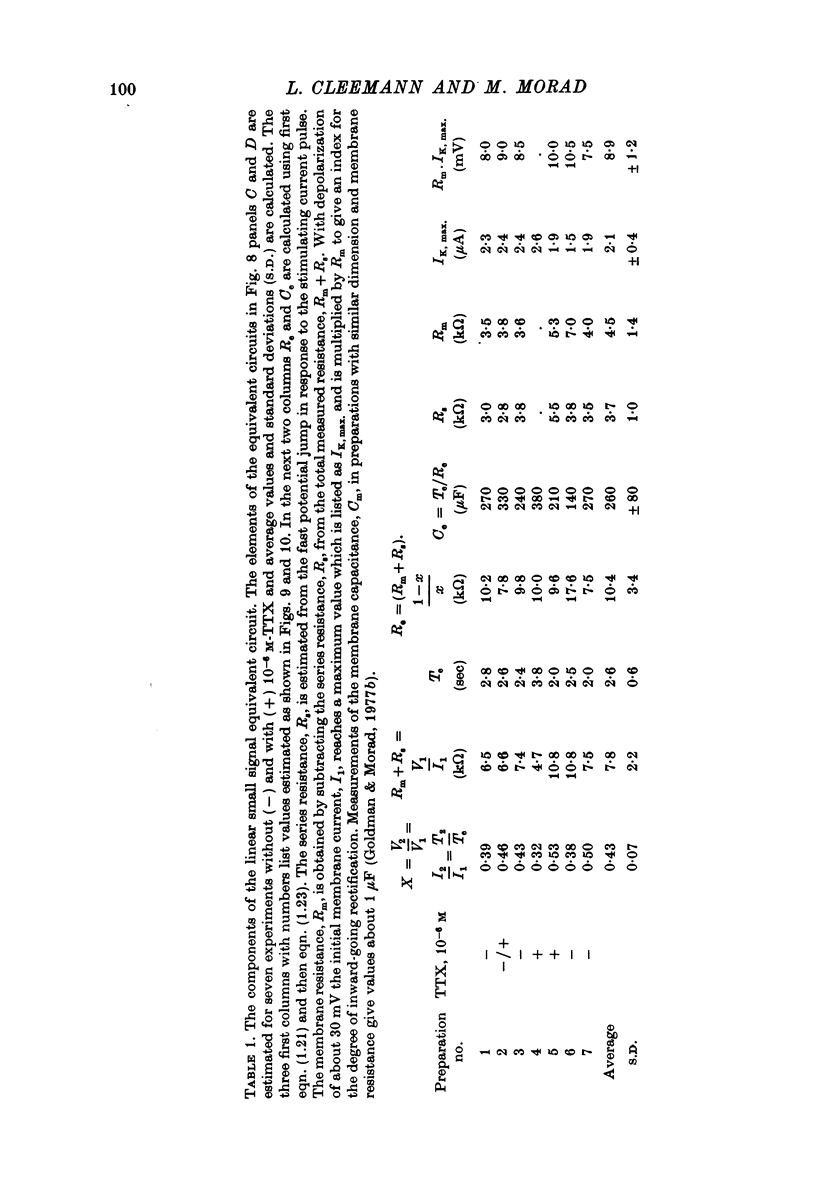
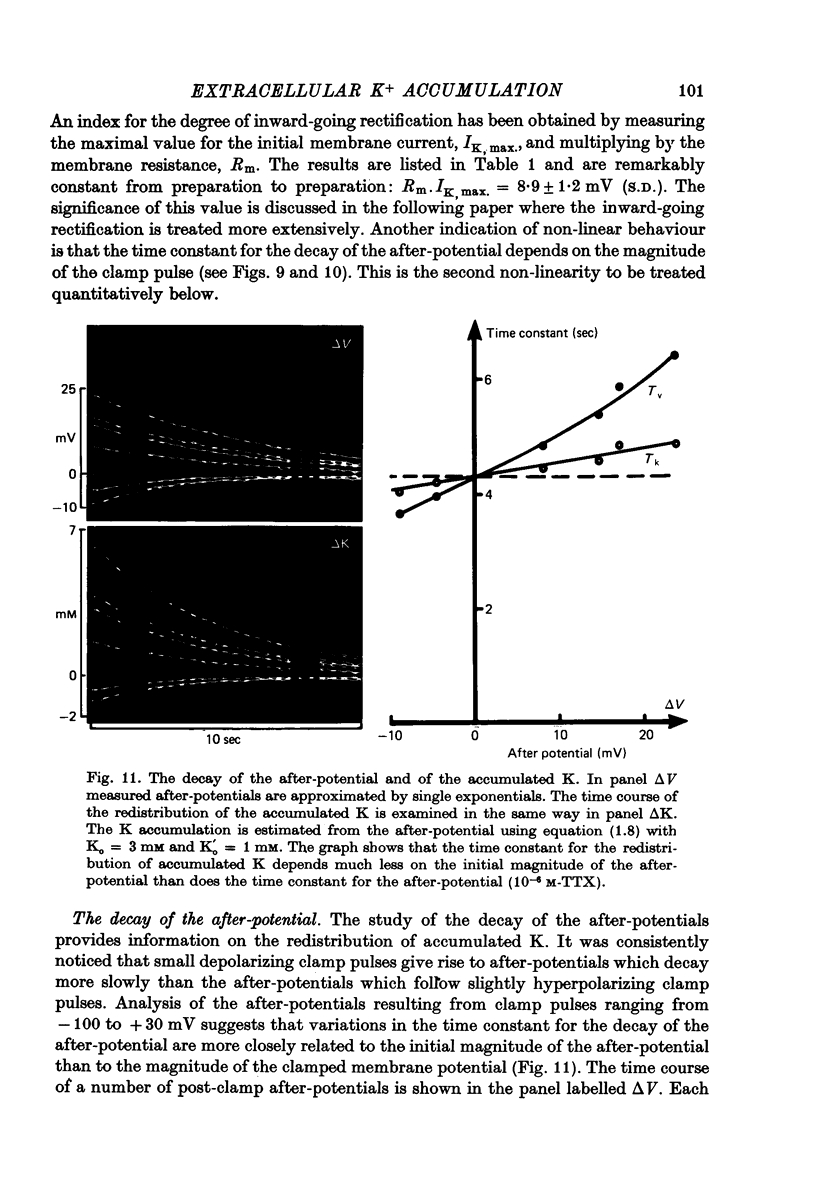
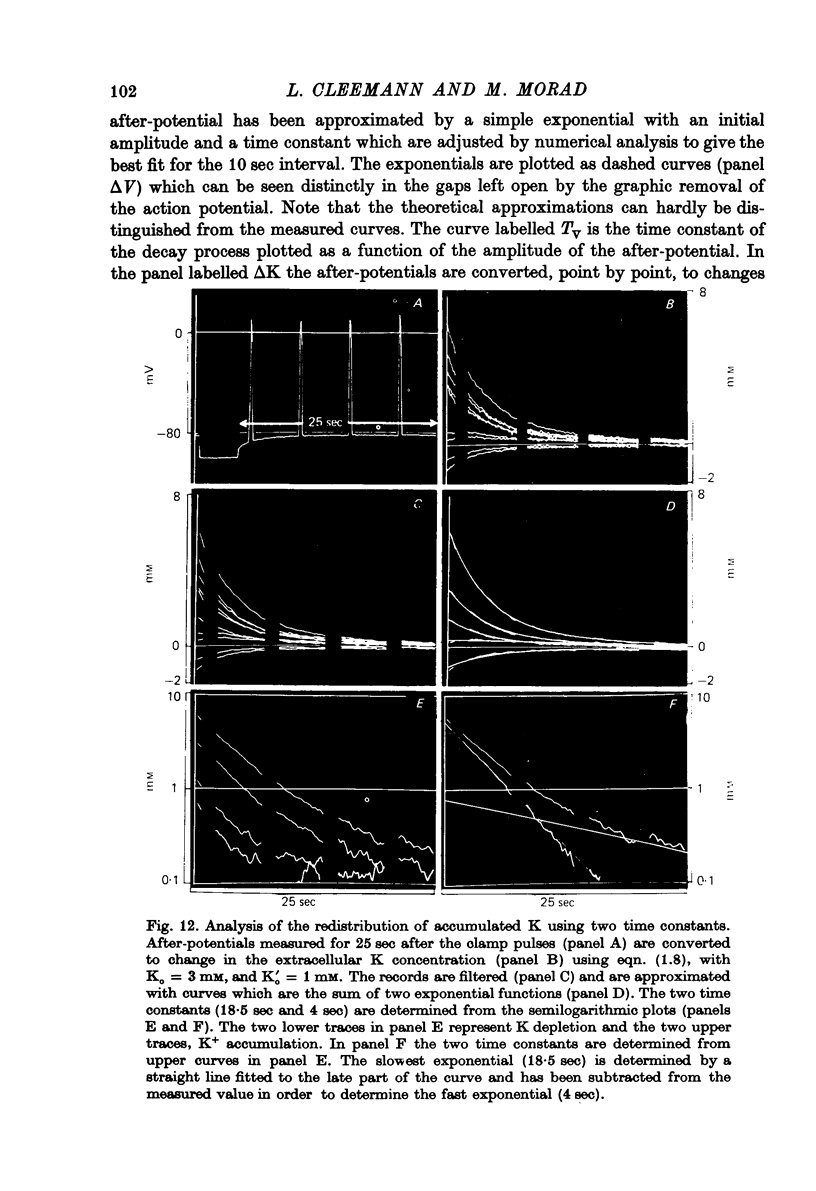
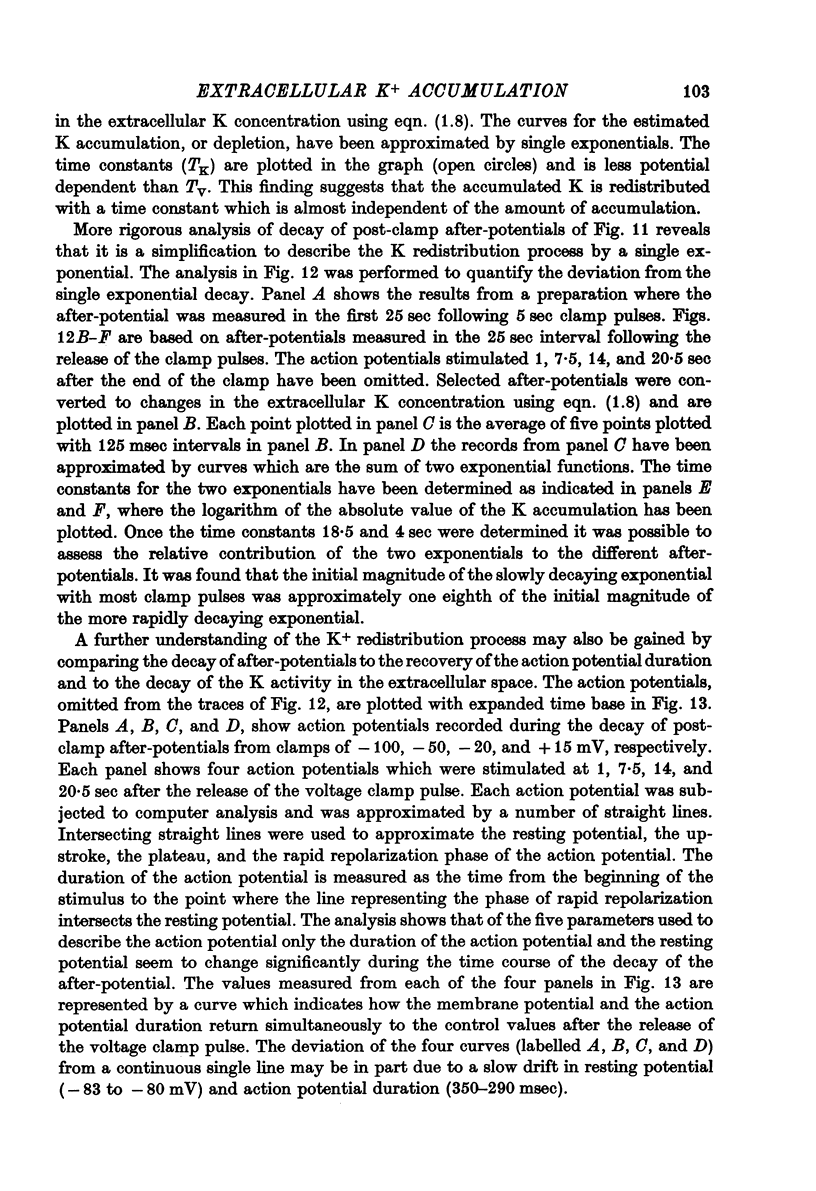
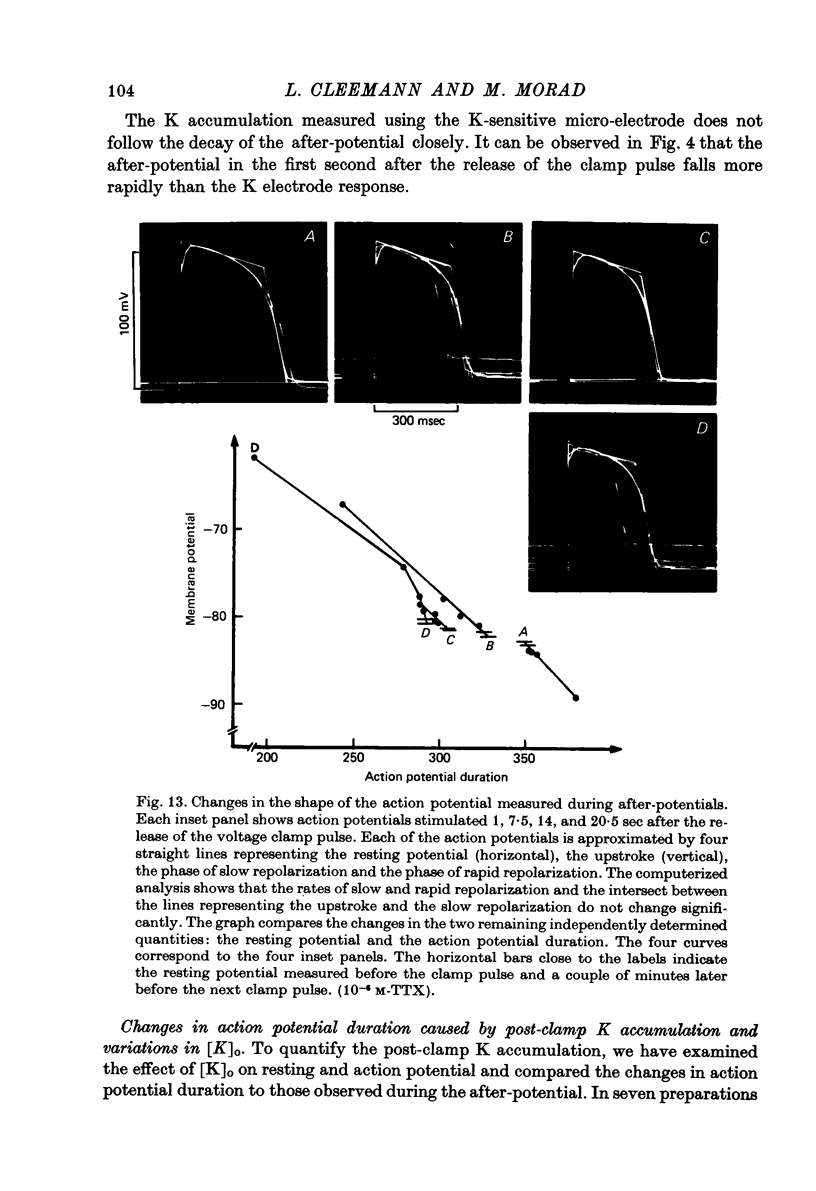
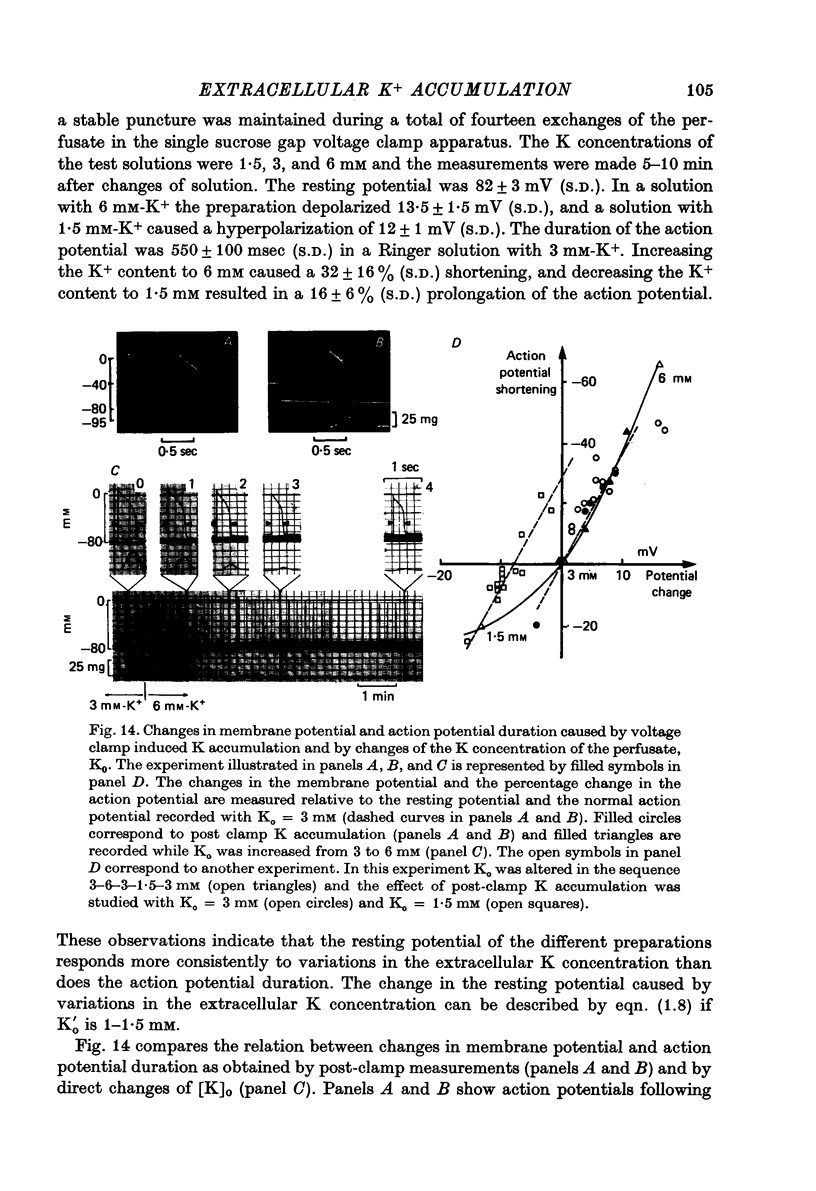
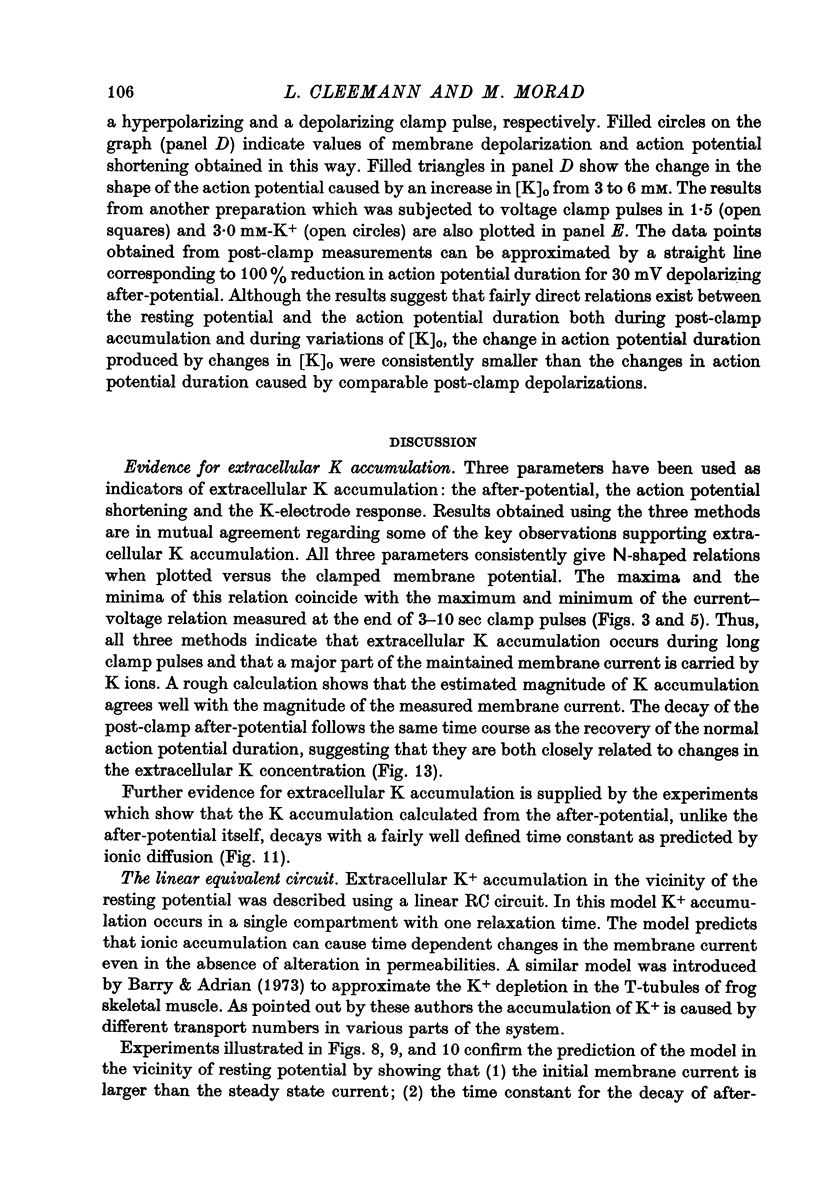
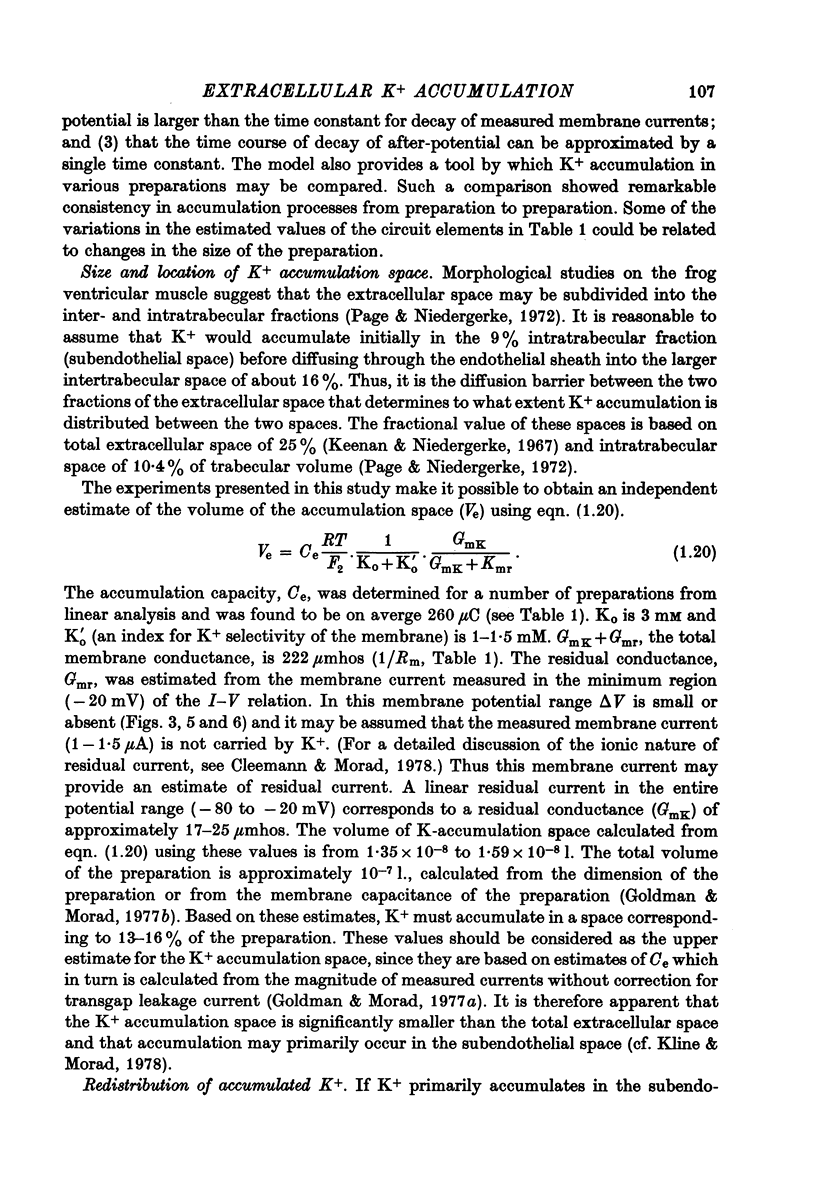
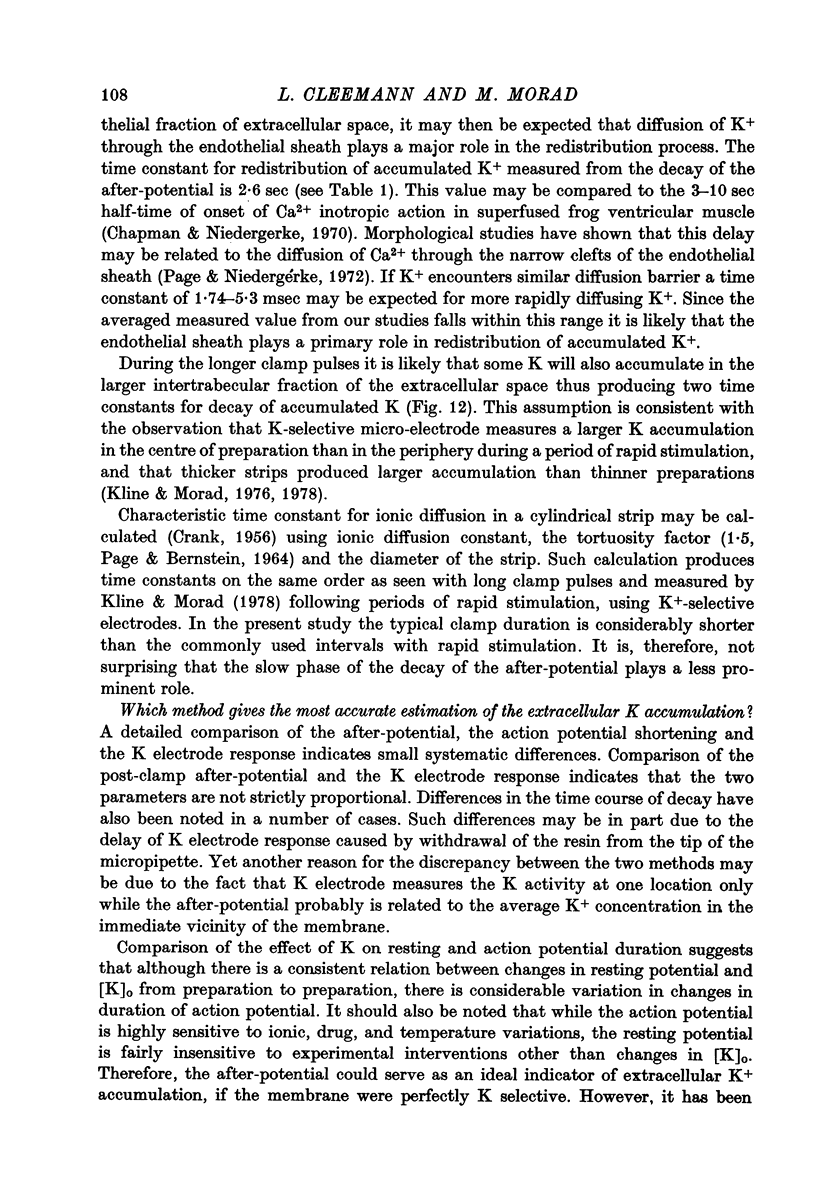
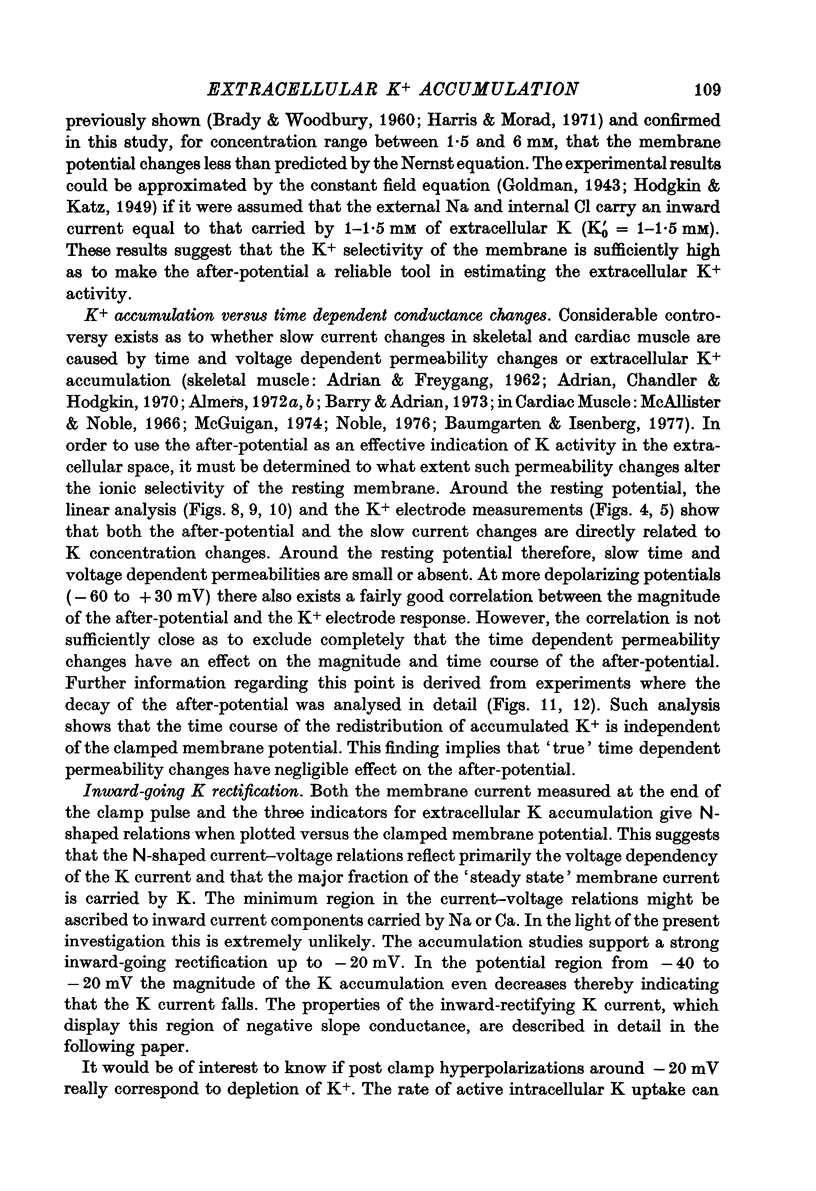
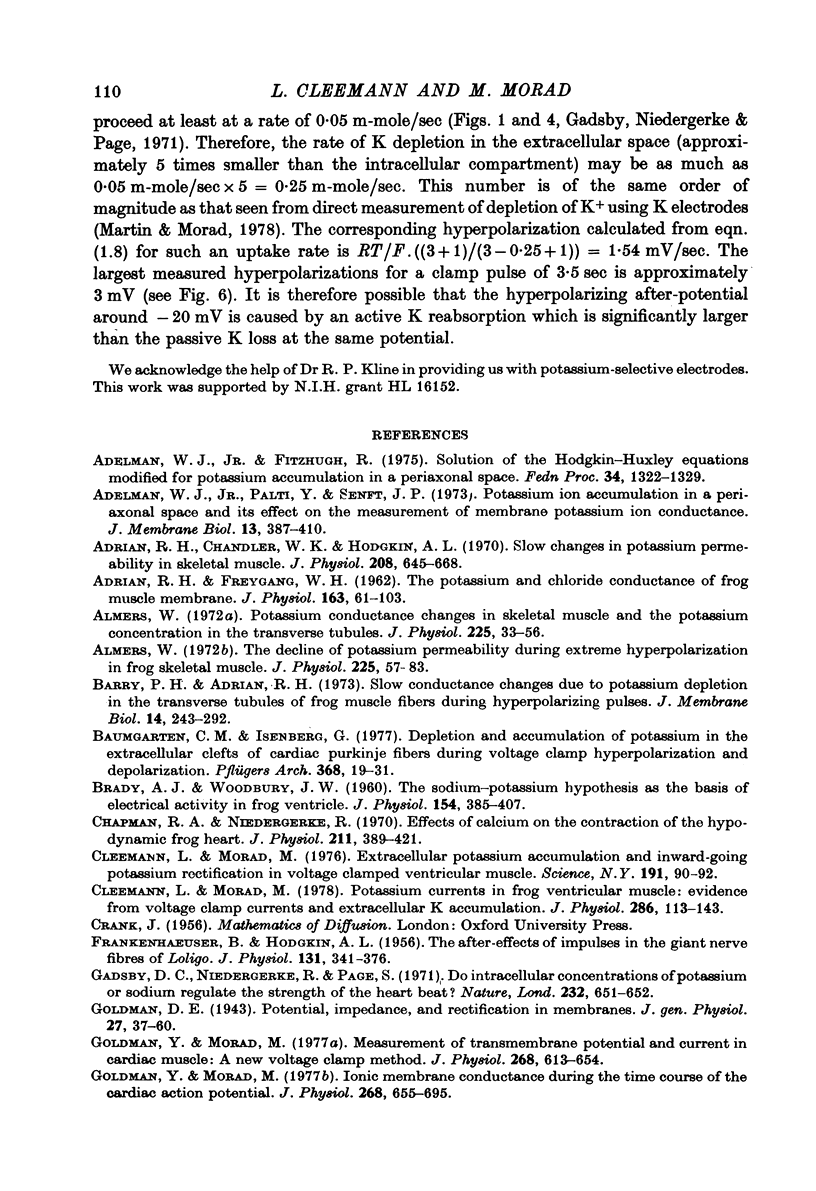
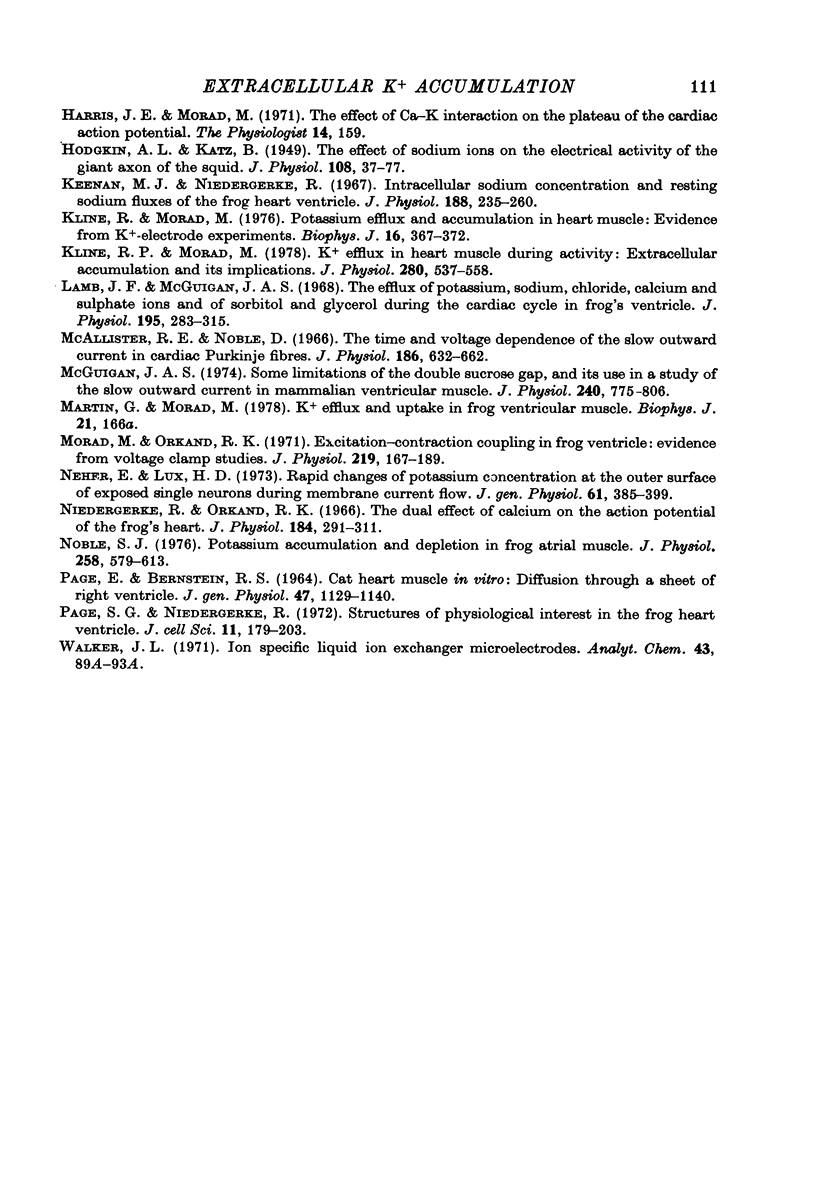
Images in this article
Selected References
These references are in PubMed. This may not be the complete list of references from this article.
- Adelman W. J., Jr, Fitzhugh R. Solutions of the Hodgkin-Huxley equations modified for potassium accumulation in a periaxonal space. Fed Proc. 1975 Apr;34(5):1322–1329. [PubMed] [Google Scholar]
- Adrian R. H., Chandler W. K., Hodgkin A. L. Slow changes in potassium permeability in skeletal muscle. J Physiol. 1970 Jul;208(3):645–668. doi: 10.1113/jphysiol.1970.sp009140. [DOI] [PMC free article] [PubMed] [Google Scholar]
- Adrian R. H., Freygang W. H. The potassium and chloride conductance of frog muscle membrane. J Physiol. 1962 Aug;163(1):61–103. doi: 10.1113/jphysiol.1962.sp006959. [DOI] [PMC free article] [PubMed] [Google Scholar]
- Almers W. Potassium conductance changes in skeletal muscle and the potassium concentration in the transverse tubules. J Physiol. 1972 Aug;225(1):33–56. doi: 10.1113/jphysiol.1972.sp009928. [DOI] [PMC free article] [PubMed] [Google Scholar]
- Almers W. The decline of potassium permeability during extreme hyperpolarization in frog skeletal muscle. J Physiol. 1972 Aug;225(1):57–83. doi: 10.1113/jphysiol.1972.sp009929. [DOI] [PMC free article] [PubMed] [Google Scholar]
- Barry P. H., Adrian R. H. Slow conductance changes due to potassium depletion in the transverse tubules of frog muscle fibers during hyperpolarizing pulses. J Membr Biol. 1973;14(3):243–292. doi: 10.1007/BF01868081. [DOI] [PubMed] [Google Scholar]
- Baumgarten C. M., Isenberg G. Depletion and accumulation of potassium in the extracellular clefts of cardiac Purkinje fibers during voltage clamp hyperpolarization and depolarization. Pflugers Arch. 1977 Mar 11;368(1-2):19–31. doi: 10.1007/BF01063450. [DOI] [PubMed] [Google Scholar]
- Brady A. J., Woodbury J. W. The sodium-potassium hypothesis as the basis of electrical activity in frog ventricle. J Physiol. 1960 Dec;154(2):385–407. doi: 10.1113/jphysiol.1960.sp006586. [DOI] [PMC free article] [PubMed] [Google Scholar]
- Chapman R. A., Niedergerke R. Effects of calcium on the contraction of the hypodynamic frog heart. J Physiol. 1970 Dec;211(2):389–421. doi: 10.1113/jphysiol.1970.sp009284. [DOI] [PMC free article] [PubMed] [Google Scholar]
- Cleemann L., Morad M. Extracellular potassium accumulation and inward-going potassium rectification in voltage clamped ventricular muscle. Science. 1976 Jan 9;191(4222):90–92. doi: 10.1126/science.1246599. [DOI] [PubMed] [Google Scholar]
- Cleemann L., Morad M. Potassium currents in frog ventricular muscle: evidence from voltage clamp currents and extracellular K accumulation. J Physiol. 1979 Jan;286:113–143. doi: 10.1113/jphysiol.1979.sp012609. [DOI] [PMC free article] [PubMed] [Google Scholar]
- FRANKENHAEUSER B., HODGKIN A. L. The after-effects of impulses in the giant nerve fibres of Loligo. J Physiol. 1956 Feb 28;131(2):341–376. doi: 10.1113/jphysiol.1956.sp005467. [DOI] [PMC free article] [PubMed] [Google Scholar]
- Goldman Y., Morad M. Ionic membrane conductance during the time course of the cardiac action potential. J Physiol. 1977 Jul;268(3):655–695. doi: 10.1113/jphysiol.1977.sp011876. [DOI] [PMC free article] [PubMed] [Google Scholar]
- Goldman Y., Morad M. Measurement of transmembrane potential and current in cardiac muscle: a new voltage clamp method. J Physiol. 1977 Jul;268(3):613–654. doi: 10.1113/jphysiol.1977.sp011875. [DOI] [PMC free article] [PubMed] [Google Scholar]
- HODGKIN A. L., KATZ B. The effect of sodium ions on the electrical activity of giant axon of the squid. J Physiol. 1949 Mar 1;108(1):37–77. doi: 10.1113/jphysiol.1949.sp004310. [DOI] [PMC free article] [PubMed] [Google Scholar]
- Keenan M. J., Niedergerke R. Intracellular sodium concentration and resting sodium fluxes of the frog heart ventricle. J Physiol. 1967 Jan;188(2):235–260. doi: 10.1113/jphysiol.1967.sp008136. [DOI] [PMC free article] [PubMed] [Google Scholar]
- Kline R. P., Morad M. Potassium efflux in heart muscle during activity: extracellular accumulation and its implications. J Physiol. 1978 Jul;280:537–558. doi: 10.1113/jphysiol.1978.sp012400. [DOI] [PMC free article] [PubMed] [Google Scholar]
- Kline R., Morad M. Potassium efflux and accumulation in heart muscle. Evidence from K +/- electrode experiments. Biophys J. 1976 Apr;16(4):367–372. doi: 10.1016/S0006-3495(76)85694-9. [DOI] [PMC free article] [PubMed] [Google Scholar]
- Lamb J. F., McGuigan J. A. The efflux of potassium, sodium, chloride, calcium and sulphate ions and of sorbitol and glycerol during the cardiac cycle in frog's ventricle. J Physiol. 1968 Mar;195(2):283–315. doi: 10.1113/jphysiol.1968.sp008459. [DOI] [PMC free article] [PubMed] [Google Scholar]
- McAllister R. E., Noble D. The time and voltage dependence of the slow outward current in cardiac Purkinje fibres. J Physiol. 1966 Oct;186(3):632–662. doi: 10.1113/jphysiol.1966.sp008060. [DOI] [PMC free article] [PubMed] [Google Scholar]
- McGuigan J. A. Some limitations of the double sucrose gap, and its use in a study of the slow outward current in mammalian ventricular muscle. J Physiol. 1974 Aug;240(3):775–806. doi: 10.1113/jphysiol.1974.sp010634. [DOI] [PMC free article] [PubMed] [Google Scholar]
- Morad M., Orkand R. K. Excitation-concentration coupling in frog ventricle: evidence from voltage clamp studies. J Physiol. 1971 Dec;219(1):167–189. doi: 10.1113/jphysiol.1971.sp009656. [DOI] [PMC free article] [PubMed] [Google Scholar]
- Neher E., Lux H. D. Rapid changes of potassium concentration at the outer surface of exposed single neurons during membrane current flow. J Gen Physiol. 1973 Mar;61(3):385–399. doi: 10.1085/jgp.61.3.385. [DOI] [PMC free article] [PubMed] [Google Scholar]
- Niedergerke R., Orkand R. K. The dual effect of calcium on the action potential of the frog's heart. J Physiol. 1966 May;184(2):291–311. doi: 10.1113/jphysiol.1966.sp007916. [DOI] [PMC free article] [PubMed] [Google Scholar]
- Noble S. J. Potassium accumulation and depletion in frog atrial muscle. J Physiol. 1976 Jul;258(3):579–613. doi: 10.1113/jphysiol.1976.sp011436. [DOI] [PMC free article] [PubMed] [Google Scholar]
- PAGE E., BERNSTEIN R. S. CAT HEART MUSCLE IN VITRO. V. DIFFUSION THROUGH A SHEET OF RIGHT VENTRICLE. J Gen Physiol. 1964 Jul;47:1129–1140. doi: 10.1085/jgp.47.6.1129. [DOI] [PMC free article] [PubMed] [Google Scholar]
- Page S. G., Niedergerke R. Structures of physiological interest in the frog heart ventricle. J Cell Sci. 1972 Jul;11(1):179–203. doi: 10.1242/jcs.11.1.179. [DOI] [PubMed] [Google Scholar]