Abstract
1. 22Na+ and 45Ca2+ fluxes were studied in the clonal nerve cell line PC12. Three distinct types of ion channels were found: (a) voltage-dependent Na+ channels, (b) voltage-dependent Ca2+ channels, and (c) acetylcholine-activated channels permeable to both ions. 2. 22Na+ uptake through voltage-dependent Na+ channels is induced by veratridine and scorpion venom, and is inhibited 50% by 5 X 10(-7) M-tetrodotoxin and greater than 98% by 5 X 10(-6) M-tetrodotoxin. 3. 45Ca2+ uptake through voltage-dependent Ca2+ channels is induced by depolarizing the cells in 50 mM-KCl. This flux is not dependent on the presence of Na+ in the medium and is insensitive to 5 X 10(-6) M-tetrodotoxin. However, 1 mM-Mn2+ causes a 95% inhibition of K+-induced 45Ca2+ uptake. 4. Veratridine and scorpion venom also induce voltage-dependent 45Ca2+ uptake which can be blocked by 1mM-Mn2+. In contrast to KCl-induced 45Ca2+ uptake, this flux is completely blocked by 5 X 10(-6) M-tetrodotoxin and is abolished by removal of Na+ from the medium. Thus the depolarizing stimulus for Ca2+ uptake in this case is Na+ from the medium. Thus the depolarizing stimulus for Ca2+ uptake in this case is Na+ influx through voltage-dependent Na+ channels. 5. Carbamylcholine induces both 22Na+ and 45Ca2+ fluxes which are blocked by nicotinic cholinergic antagonists with the exception of alpha-bungarotoxin. The 22Na+ flux occurs exclusively via acetylcholine receptor channels, as evidenced by the lack of effect of 5 X 10(-6) M-tetrodotoxin. In the presence of Na+, almost all of the 45Ca2+ uptake can be blocked by 1 mM-Mn2+ and thus occurs via voltage-dependent Ca2+ channels which are activated by the depolarizing Na+ influx. 6--8% of the total 45Ca2+ flux, however, is insensitive to 1 mM-Mn2+, suggesting that this portion of the uptake occurs via the acetylcholine receptor channels. In Na+-free medium, the Mn2+-resistant 45Ca2+ component increases to 40% of the total uptake, apparently due to lack of competition from Na+ for the acetylcholine receptor channels. This receptor-linked flux still causes sufficient depolarization to induce the additional 60% of the Ca2+ flux through voltage-dependent, Mn2+ sensitive Ca2+ channels. 6. Mn2+ inhibits Ca2+ flux through voltage-dependent Ca2+ channels by competing for entry through these channels. 50 mM-KCl induces 54Mn2+ fluxes in PC12 cells that are comparable in magnitude to 45Ca2+ fluxes. 7. In normal saline 45Ca2+ efflux from PC12 cells is several times more rapid than in Na+-free medium, indicating the presence of a Ca2+-Na+ exchange mechanism.
Full text
PDF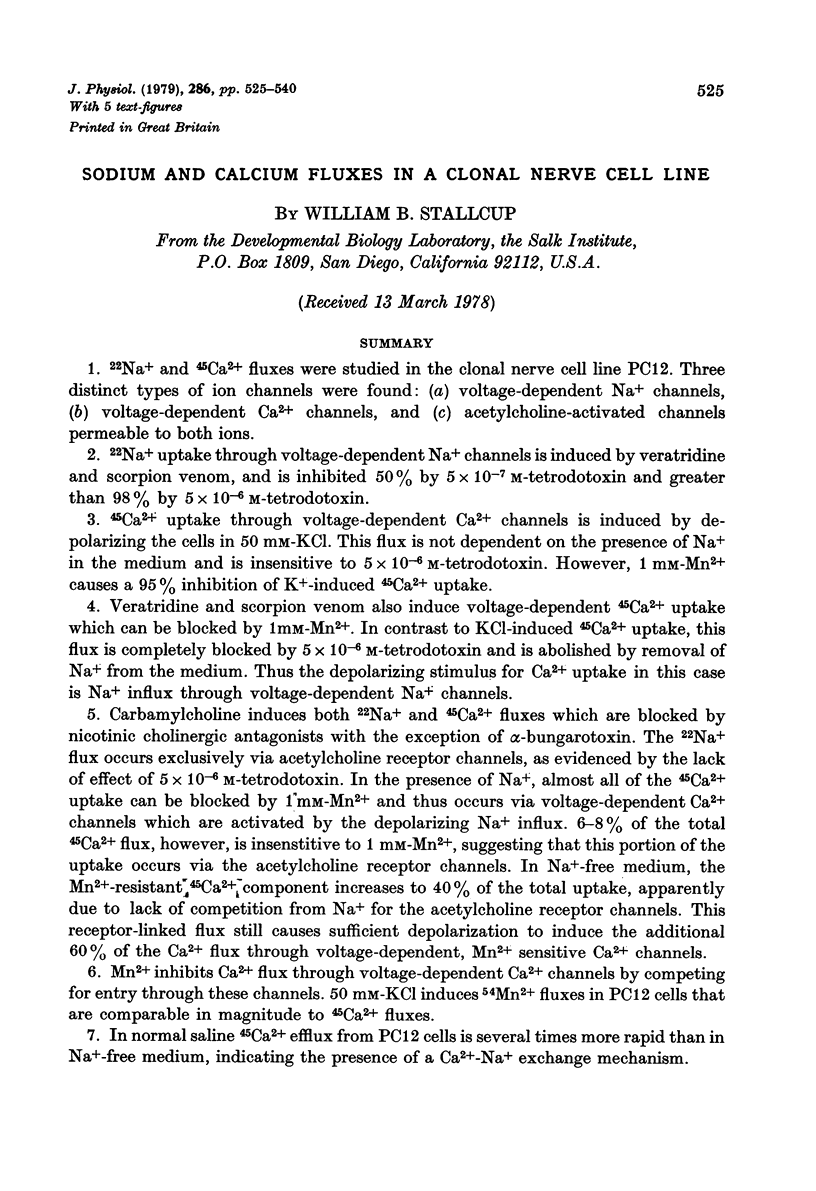
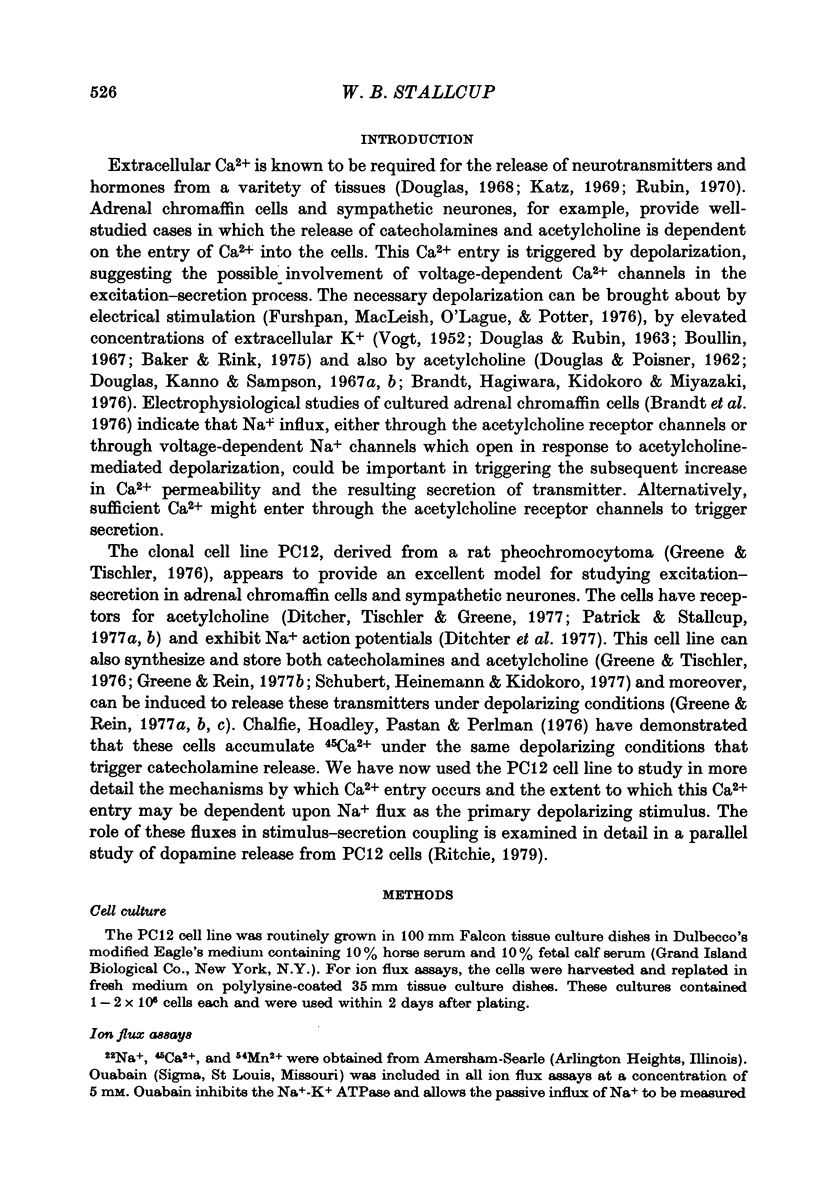
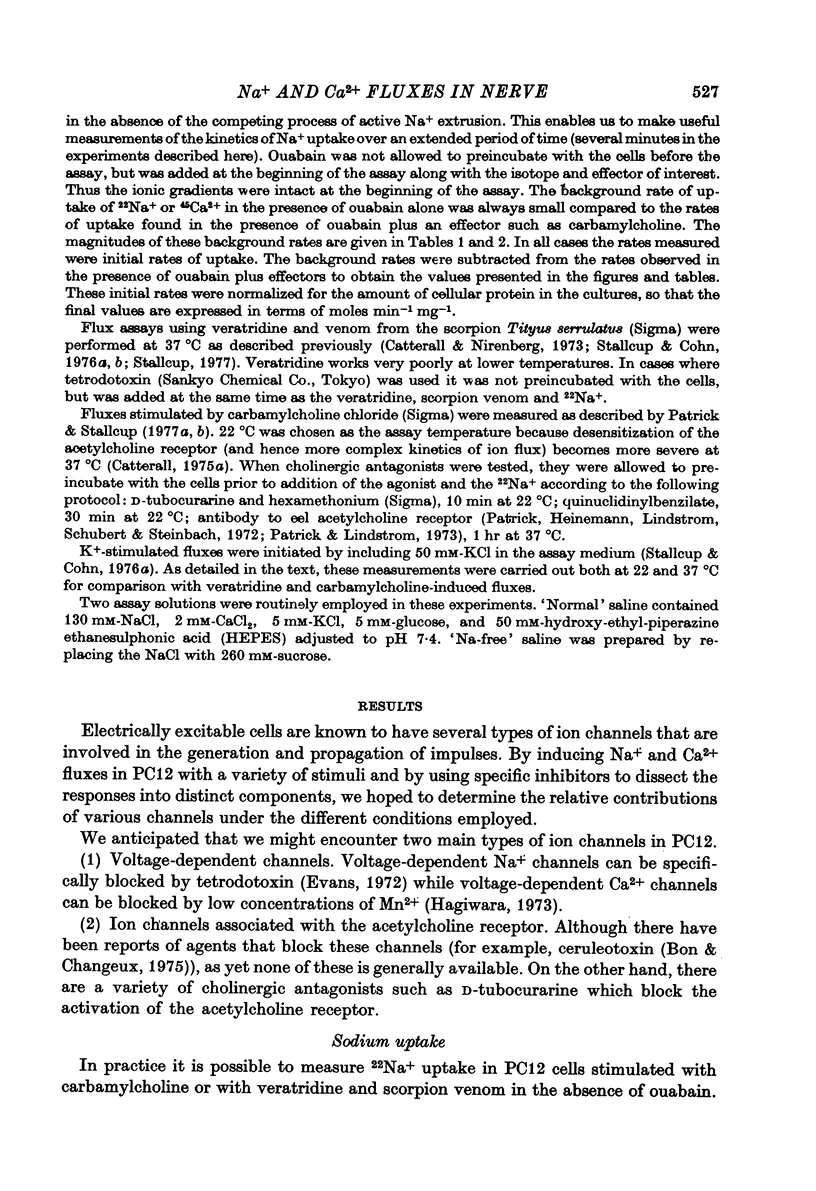
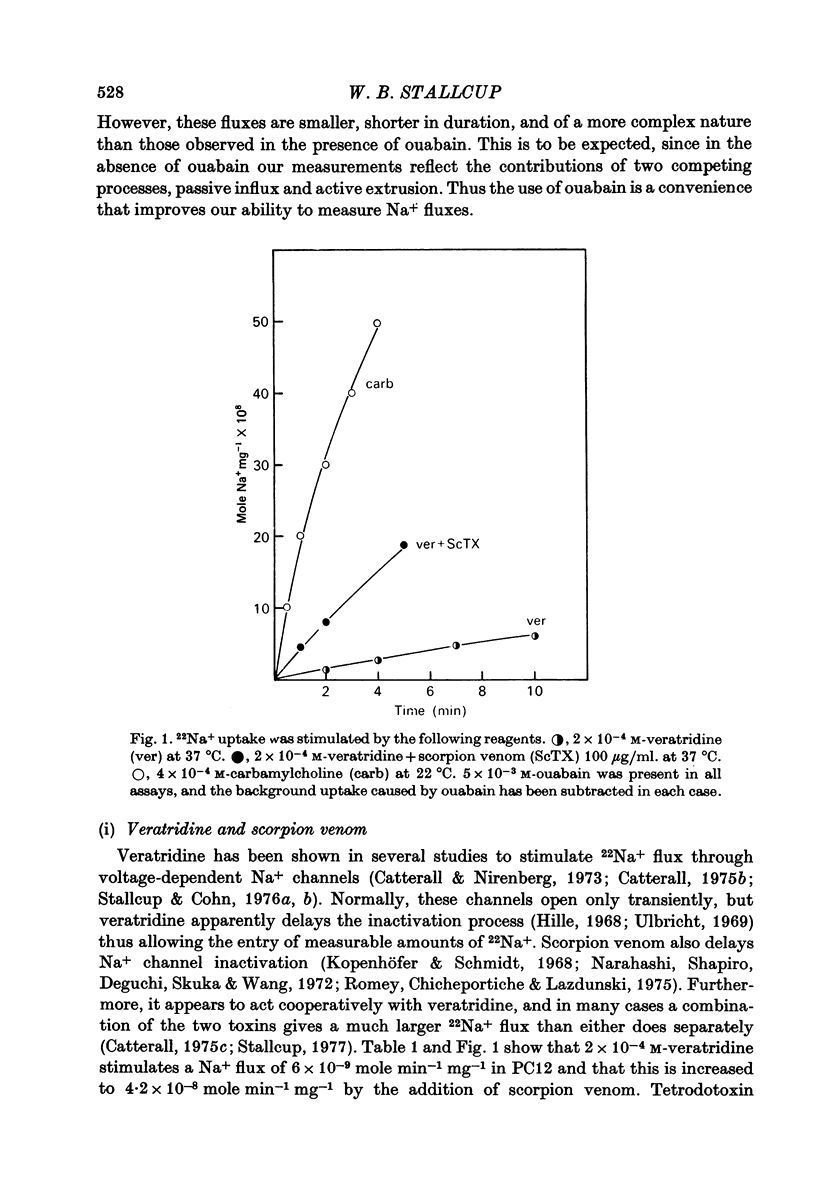
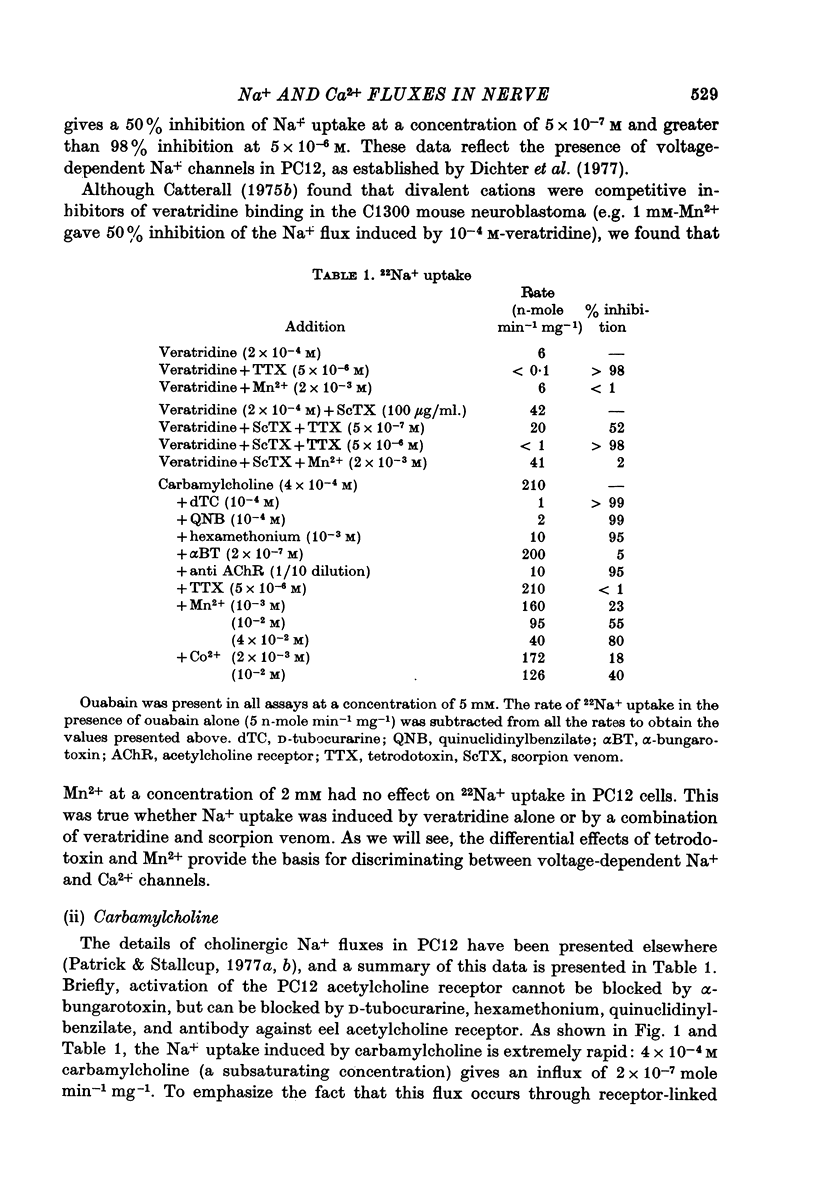
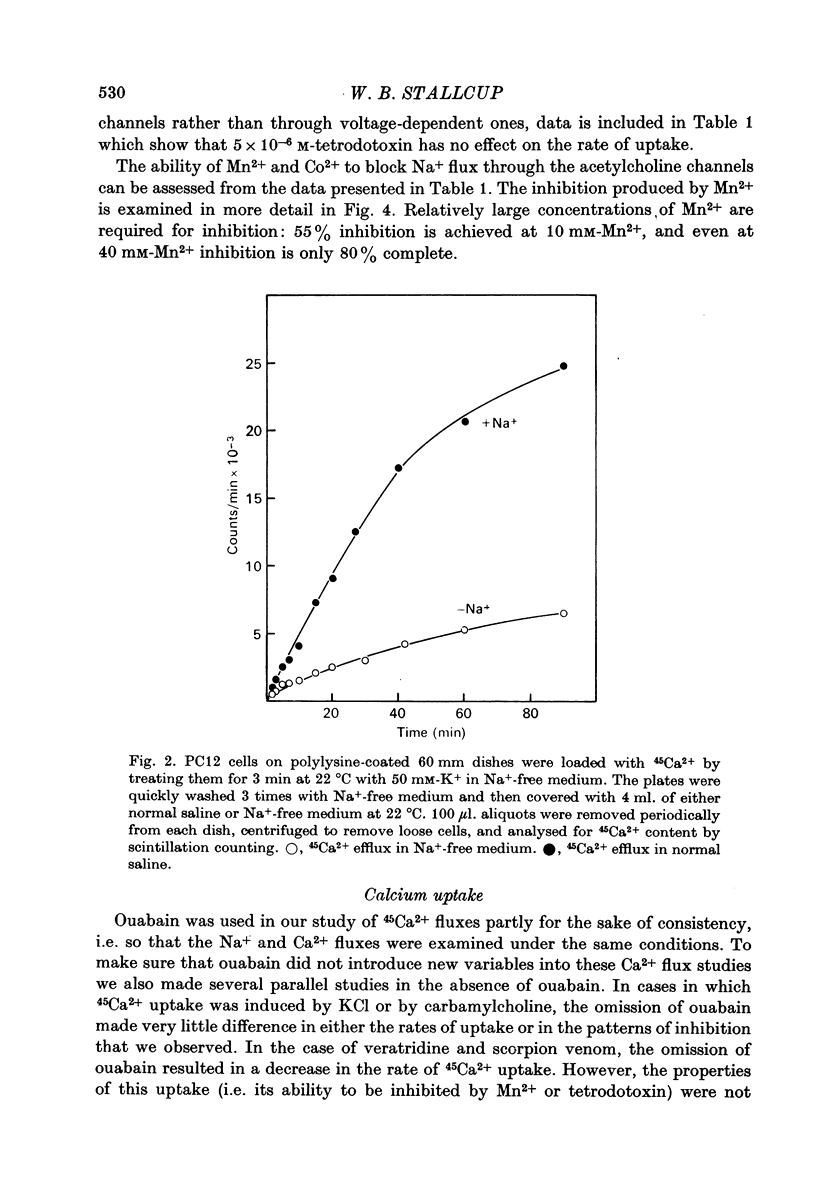
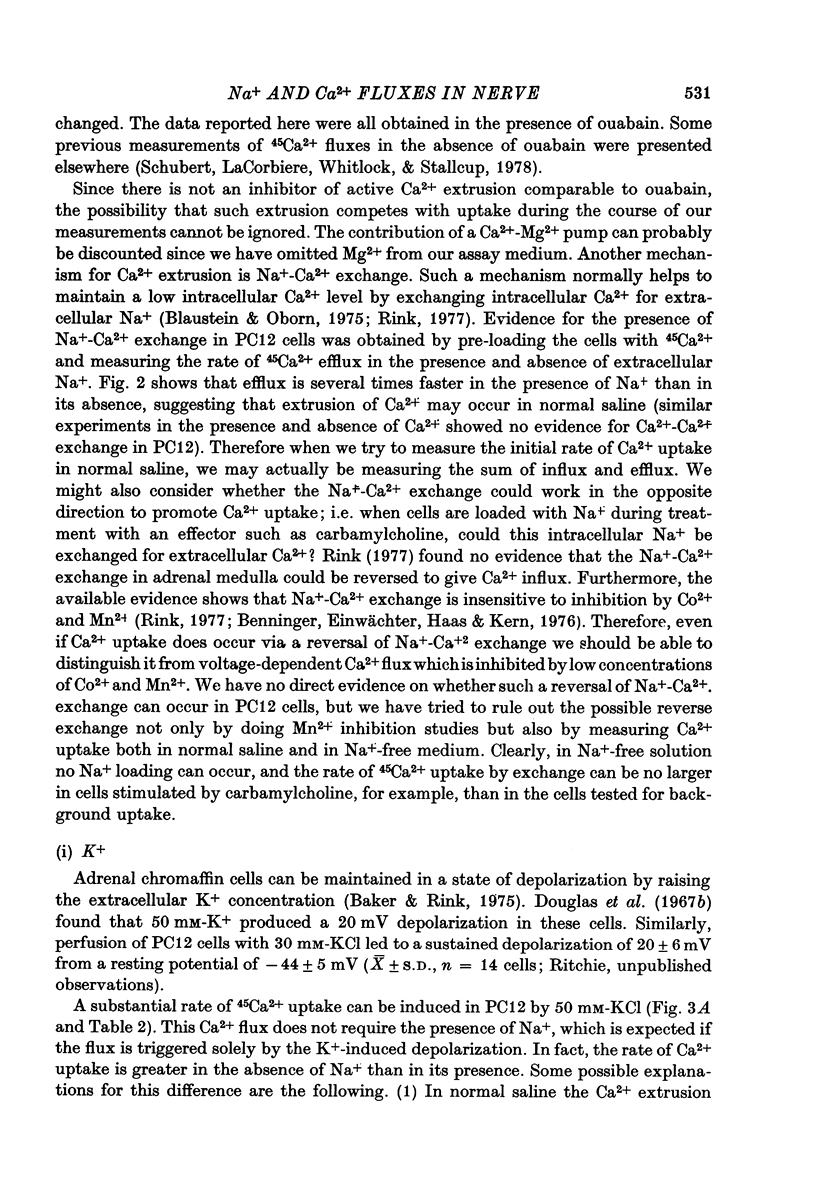
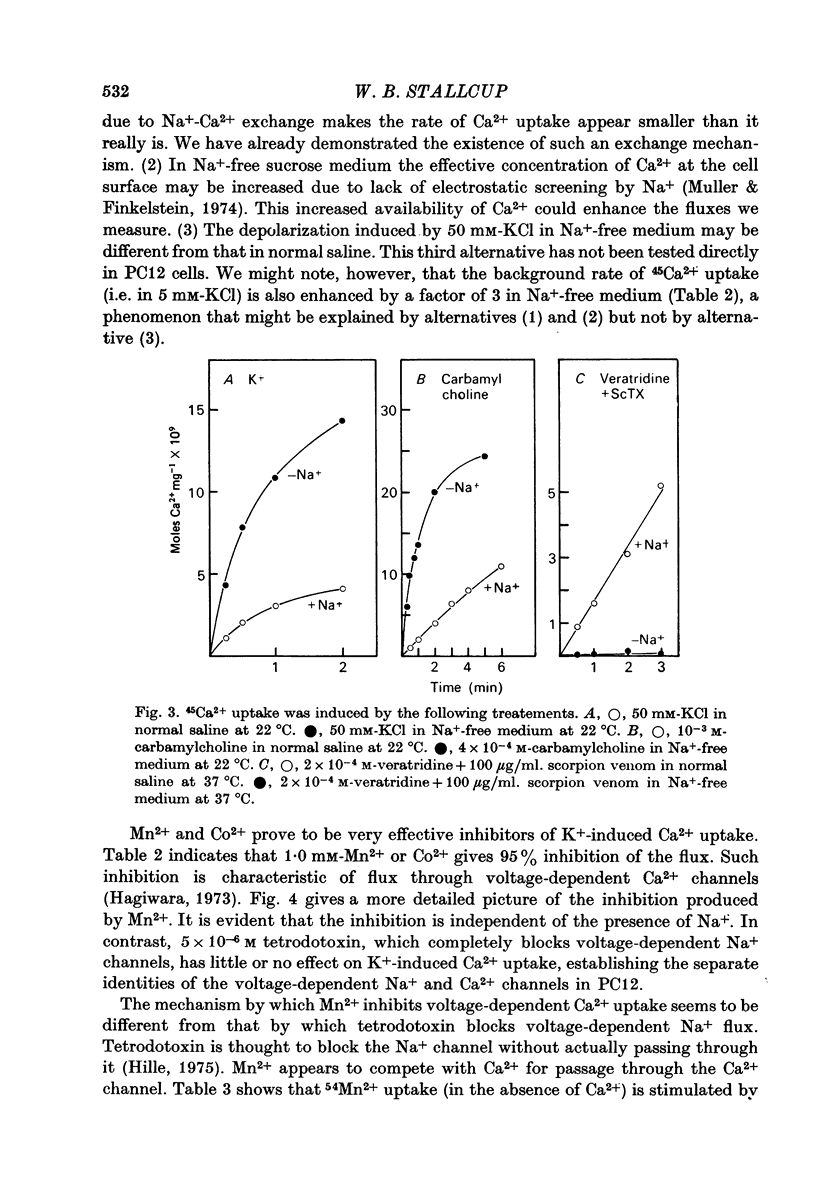
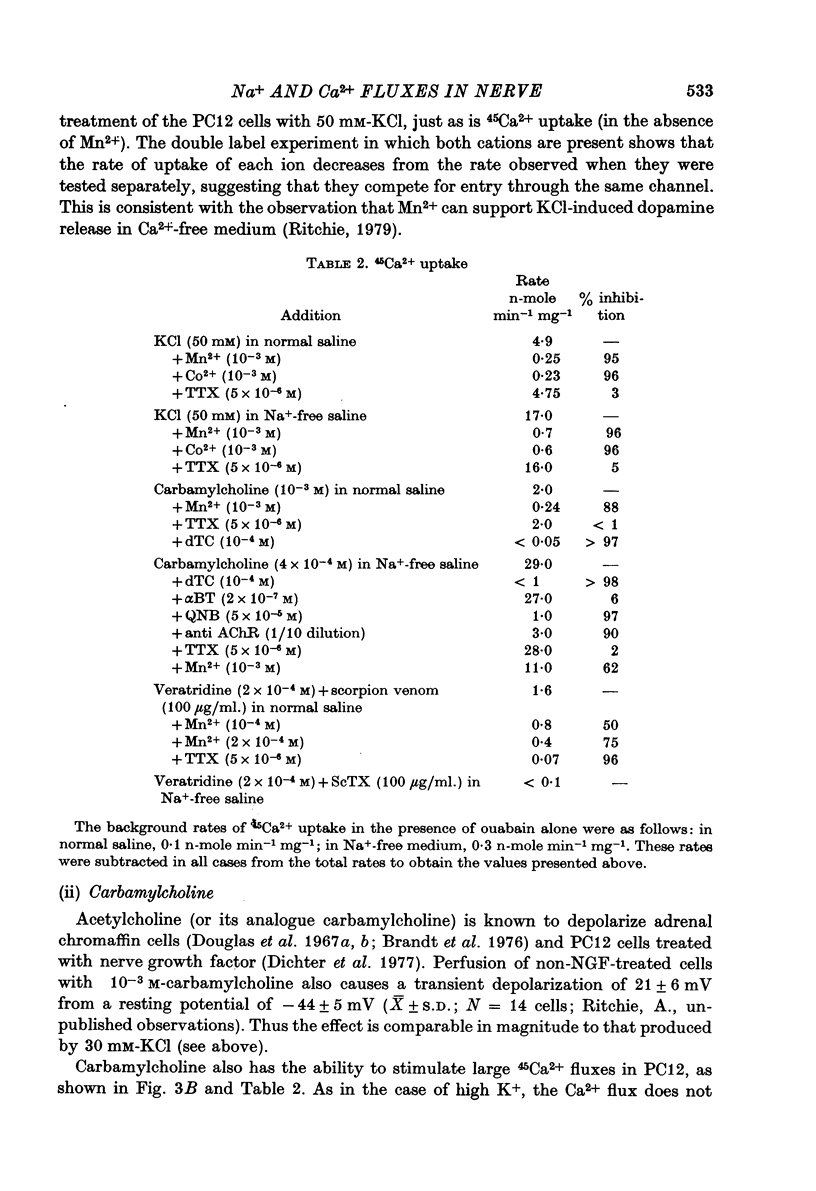
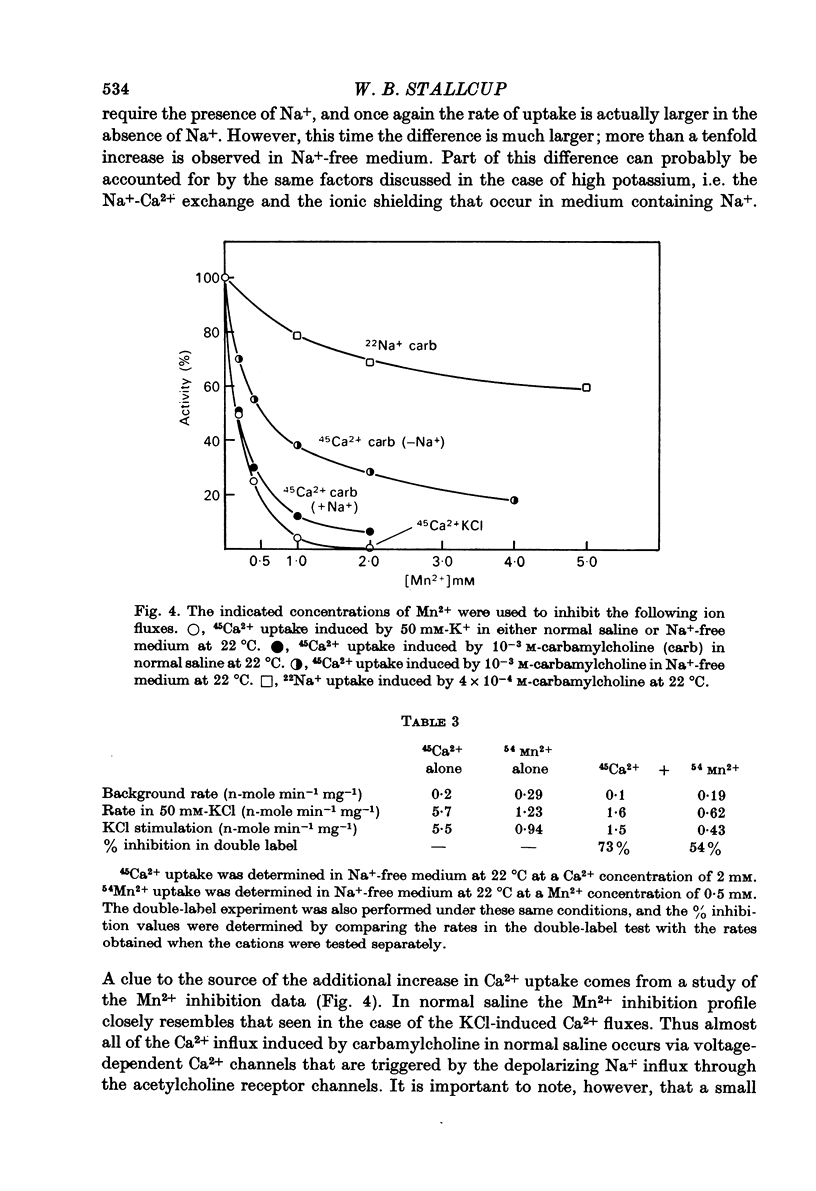
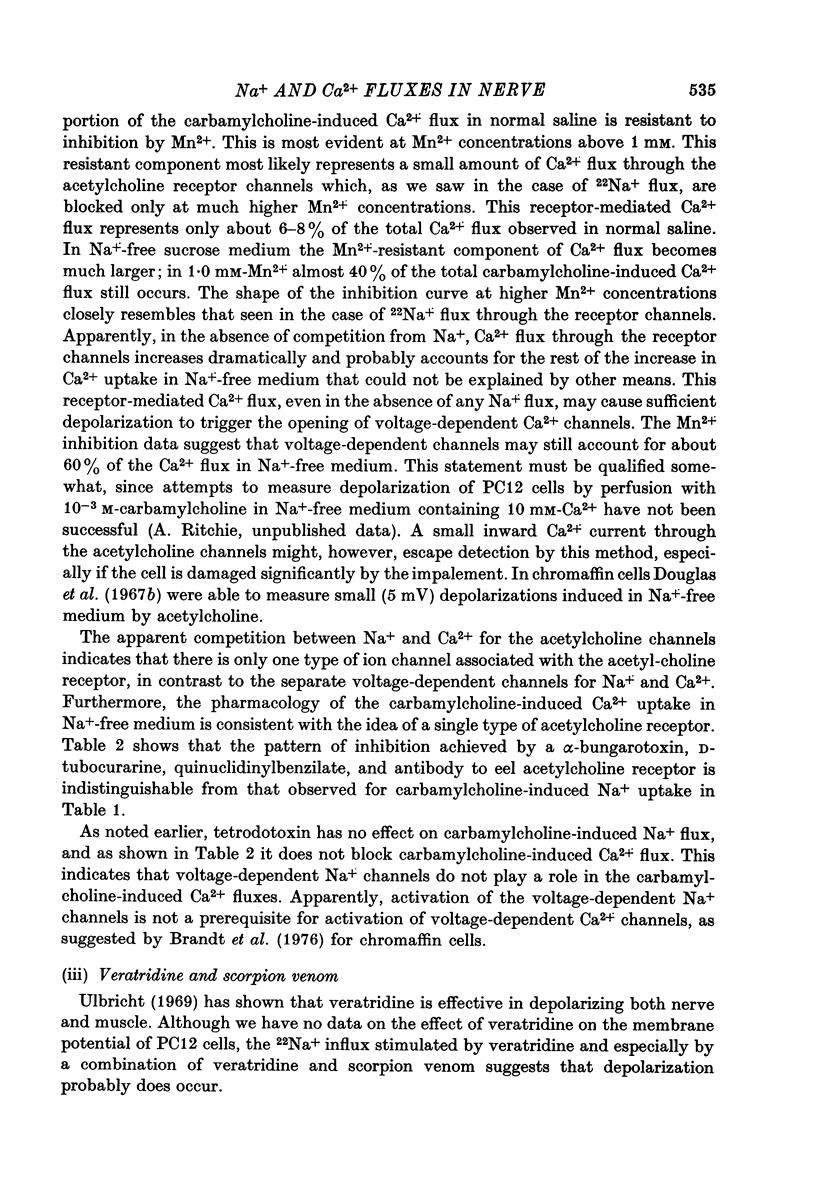
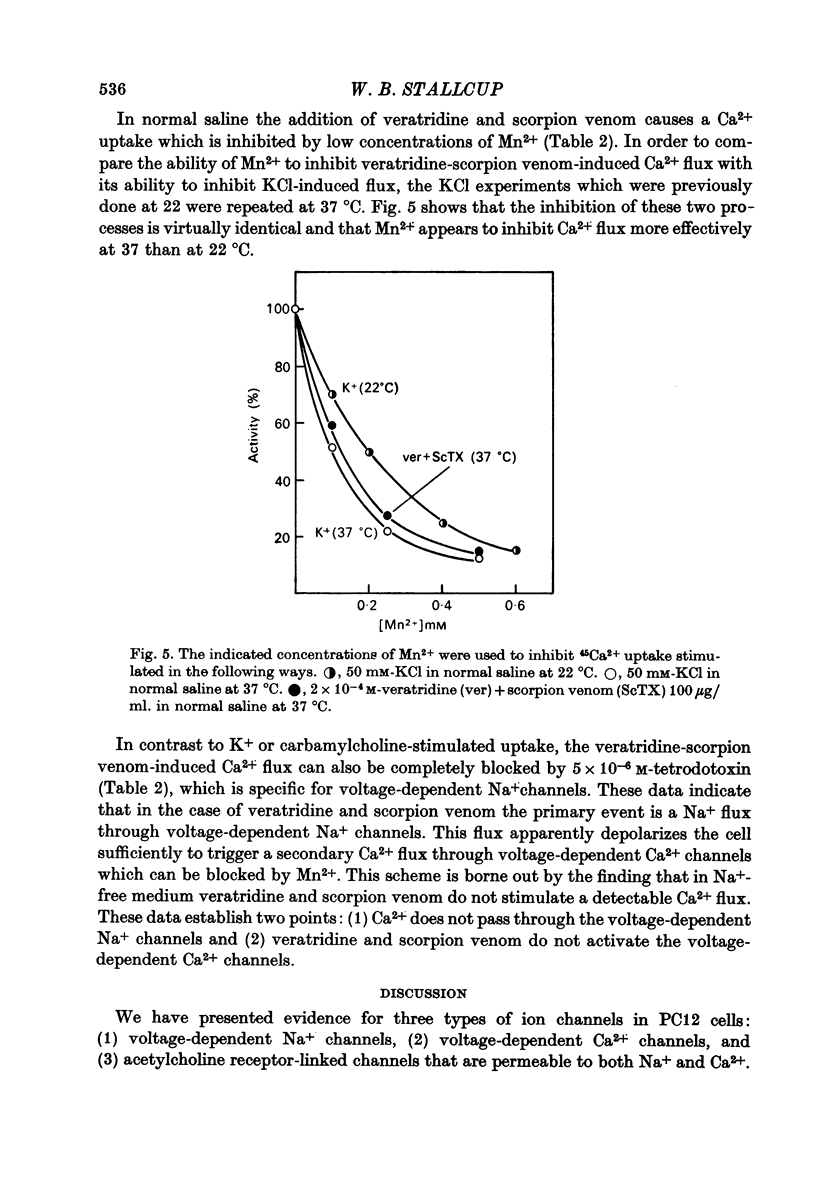
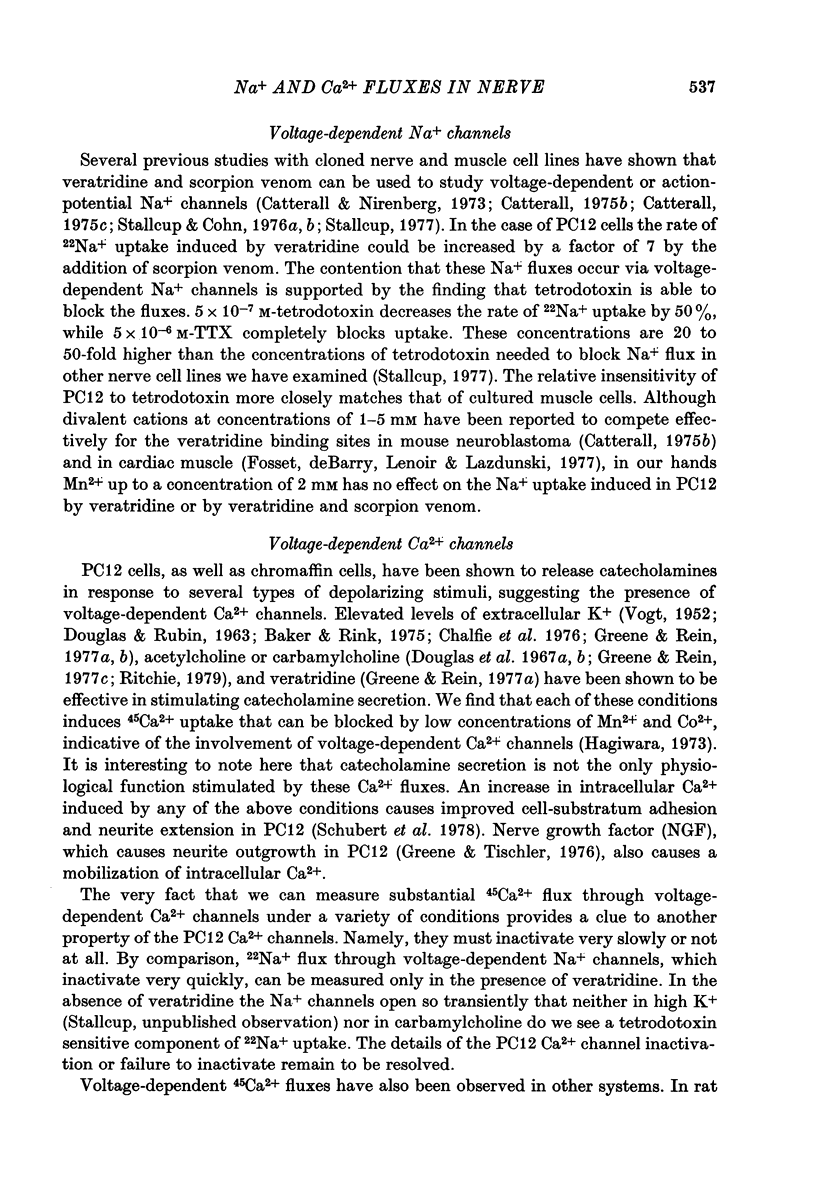
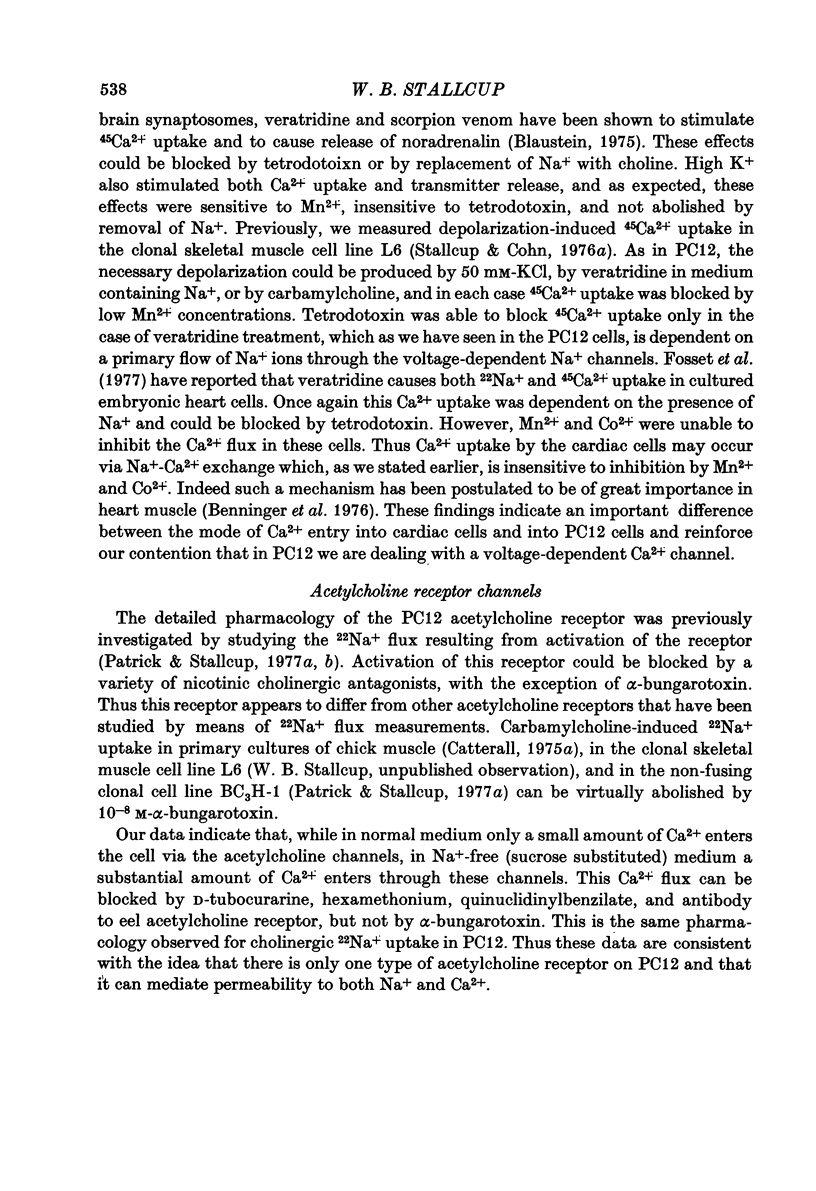
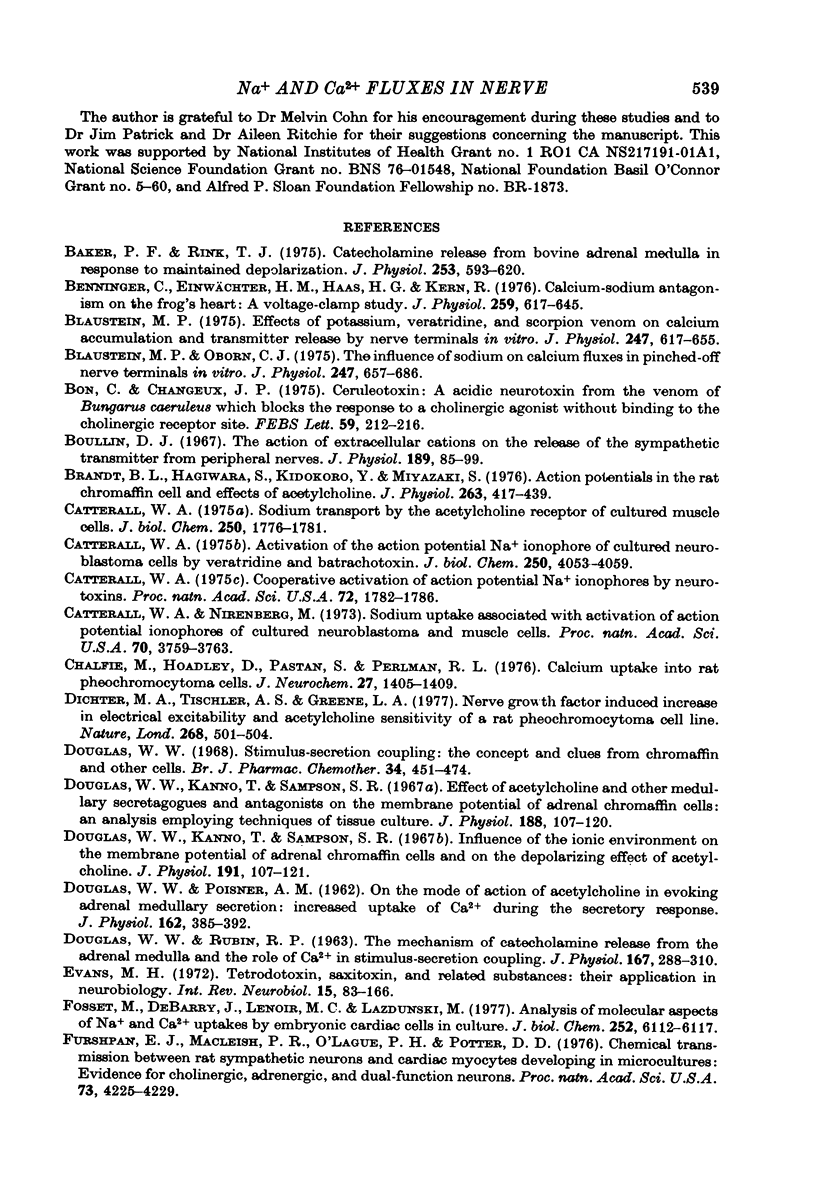
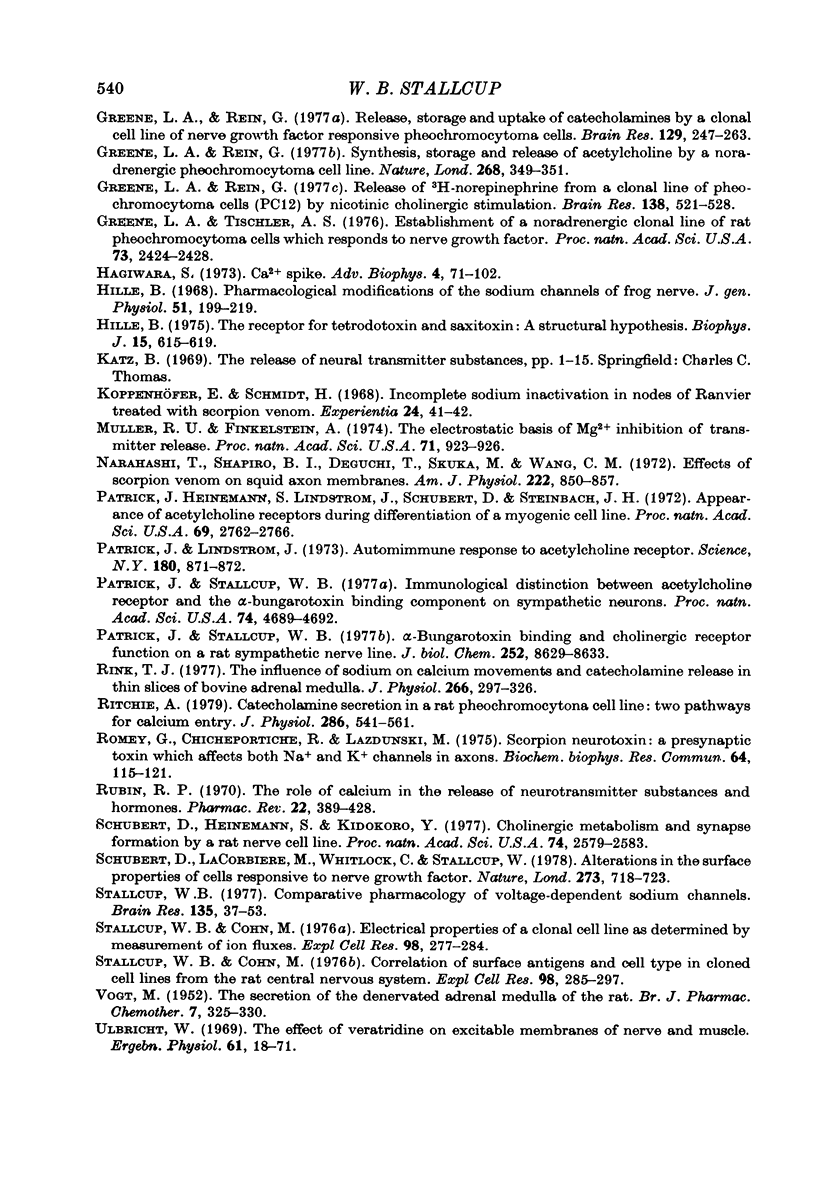
Selected References
These references are in PubMed. This may not be the complete list of references from this article.
- Baker P. F., Rink T. J. Catecholamine release from bovine adrenal medulla in response to maintained depolarization. J Physiol. 1975 Dec;253(2):593–620. doi: 10.1113/jphysiol.1975.sp011209. [DOI] [PMC free article] [PubMed] [Google Scholar]
- Benninger C., Einwächter H. M., Haas H. G., Kern R. Calcium-sodium antagonism on the frog's heart: a voltage-clamp study. J Physiol. 1976 Aug;259(3):617–645. doi: 10.1113/jphysiol.1976.sp011486. [DOI] [PMC free article] [PubMed] [Google Scholar]
- Blaustein M. P. Effects of potassium, veratridine, and scorpion venom on calcium accumulation and transmitter release by nerve terminals in vitro. J Physiol. 1975 Jun;247(3):617–655. doi: 10.1113/jphysiol.1975.sp010950. [DOI] [PMC free article] [PubMed] [Google Scholar]
- Blaustein M. P., Oborn C. J. The influence of sodium on calcium fluxes in pinched-off nerve terminals in vitro. J Physiol. 1975 Jun;247(3):657–686. doi: 10.1113/jphysiol.1975.sp010951. [DOI] [PMC free article] [PubMed] [Google Scholar]
- Bon C., Changeux J. P. Ceruleotoxin: an acidic neurotoxin from the venom of Bungarus caeruleus which blocks the response to a cholinergic agonist without binding to the cholinergic receptor site. FEBS Lett. 1975 Nov 15;59(2):212–216. doi: 10.1016/0014-5793(75)80377-2. [DOI] [PubMed] [Google Scholar]
- Boullin D. J. The action of extracellular cations on the release of the sympathetic transmitter from peripheral nerves. J Physiol. 1967 Mar;189(1):85–99. doi: 10.1113/jphysiol.1967.sp008156. [DOI] [PMC free article] [PubMed] [Google Scholar]
- Brandt B. L., Hagiwara S., Kidokoro Y., Miyazaki S. Action potentials in the rat chromaffin cell and effects of acetylcholine. J Physiol. 1976 Dec;263(3):417–439. doi: 10.1113/jphysiol.1976.sp011638. [DOI] [PMC free article] [PubMed] [Google Scholar]
- Catterall W. A. Activation of the action potential Na+ ionophore of cultured neuroblastoma cells by veratridine and batrachotoxin. J Biol Chem. 1975 Jun 10;250(11):4053–4059. [PubMed] [Google Scholar]
- Catterall W. A. Cooperative activation of action potential Na+ ionophore by neurotoxins. Proc Natl Acad Sci U S A. 1975 May;72(5):1782–1786. doi: 10.1073/pnas.72.5.1782. [DOI] [PMC free article] [PubMed] [Google Scholar]
- Catterall W. A., Nirenberg M. Sodium uptake associated with activation of action potential ionophores of cultured neuroblastoma and muscle cells. Proc Natl Acad Sci U S A. 1973 Dec;70(12):3759–3763. doi: 10.1073/pnas.70.12.3759. [DOI] [PMC free article] [PubMed] [Google Scholar]
- Catterall W. A. Sodium transport by the acetylcholine receptor of cultured muscle cells. J Biol Chem. 1975 Mar 10;250(5):1776–1781. [PubMed] [Google Scholar]
- Chalfie M., Hoadley D., Pastan S., Perlman R. L. Calcium uptake into rat pheochromocytoma cells. J Neurochem. 1976 Dec;27(6):1405–1409. doi: 10.1111/j.1471-4159.1976.tb02622.x. [DOI] [PubMed] [Google Scholar]
- DOUGLAS W. W., POISNER A. M. On the mode of action of acetylcholine in evoking adrenal medullary secretion: increased uptake of calcium during the secretory response. J Physiol. 1962 Aug;162:385–392. doi: 10.1113/jphysiol.1962.sp006940. [DOI] [PMC free article] [PubMed] [Google Scholar]
- Dichter M. A., Tischler A. S., Greene L. A. Nerve growth factor-induced increase in electrical excitability and acetylcholine sensitivity of a rat pheochromocytoma cell line. Nature. 1977 Aug 11;268(5620):501–504. doi: 10.1038/268501a0. [DOI] [PubMed] [Google Scholar]
- Douglas W. W., Kanno T., Sampson S. R. Effects of acetylcholine and other medullary secretagogues and antagonists on the membrane potential of adrenal chromaffin cells: an analysis employing techniques of tissue culture. J Physiol. 1967 Jan;188(1):107–120. doi: 10.1113/jphysiol.1967.sp008127. [DOI] [PMC free article] [PubMed] [Google Scholar]
- Douglas W. W., Kanno T., Sampson S. R. Influence of the ionic environment on the membrane potential of adrenal chromaffin cells and on the depolarizing effect of acetylcholine. J Physiol. 1967 Jul;191(1):107–121. doi: 10.1113/jphysiol.1967.sp008239. [DOI] [PMC free article] [PubMed] [Google Scholar]
- Douglas W. W., Rubin R. P. The mechanism of catecholamine release from the adrenal medulla and the role of calcium in stimulus-secretion coupling. J Physiol. 1963 Jul;167(2):288–310. doi: 10.1113/jphysiol.1963.sp007150. [DOI] [PMC free article] [PubMed] [Google Scholar]
- Douglas W. W. Stimulus-secretion coupling: the concept and clues from chromaffin and other cells. Br J Pharmacol. 1968 Nov;34(3):451–474. doi: 10.1111/j.1476-5381.1968.tb08474.x. [DOI] [PMC free article] [PubMed] [Google Scholar]
- Evans M. H. Tetrodotoxin, saxitoxin, and related substances: their applications in neurobiology. Int Rev Neurobiol. 1972;15:83–166. doi: 10.1016/s0074-7742(08)60329-3. [DOI] [PubMed] [Google Scholar]
- Fosset M., De Barry J., Lenoir M. C., Lazdunski M. Analysis of molecular aspects of Na+ and Ca2+ uptakes by embryonic cardiac cells in culture. J Biol Chem. 1977 Sep 10;252(17):6112–6117. [PubMed] [Google Scholar]
- Furshpan E. J., MacLeish P. R., O'Lague P. H., Potter D. D. Chemical transmission between rat sympathetic neurons and cardiac myocytes developing in microcultures: evidence for cholinergic, adrenergic, and dual-function neurons. Proc Natl Acad Sci U S A. 1976 Nov;73(11):4225–4229. doi: 10.1073/pnas.73.11.4225. [DOI] [PMC free article] [PubMed] [Google Scholar]
- Greene L. A., Rein G. Release of (3H)norepinephrine from a clonal line of pheochromocytoma cells (PC12) by nicotinic cholinergic stimulation. Brain Res. 1977 Dec 23;138(3):521–528. doi: 10.1016/0006-8993(77)90687-4. [DOI] [PubMed] [Google Scholar]
- Greene L. A., Rein G. Release, storage and uptake of catecholamines by a clonal cell line of nerve growth factor (NGF) responsive pheo-chromocytoma cells. Brain Res. 1977 Jul 1;129(2):247–263. doi: 10.1016/0006-8993(77)90005-1. [DOI] [PubMed] [Google Scholar]
- Greene L. A., Rein G. Synthesis, storage and release of acetylcholine by a noradrenergic pheochromocytoma cell line. Nature. 1977 Jul 28;268(5618):349–351. doi: 10.1038/268349a0. [DOI] [PubMed] [Google Scholar]
- Greene L. A., Tischler A. S. Establishment of a noradrenergic clonal line of rat adrenal pheochromocytoma cells which respond to nerve growth factor. Proc Natl Acad Sci U S A. 1976 Jul;73(7):2424–2428. doi: 10.1073/pnas.73.7.2424. [DOI] [PMC free article] [PubMed] [Google Scholar]
- Hagiwara S. Ca spike. Adv Biophys. 1973;4:71–102. [PubMed] [Google Scholar]
- Hille B. Pharmacological modifications of the sodium channels of frog nerve. J Gen Physiol. 1968 Feb;51(2):199–219. doi: 10.1085/jgp.51.2.199. [DOI] [PMC free article] [PubMed] [Google Scholar]
- Hille B. The receptor for tetrodotoxin and saxitoxin. A structural hypothesis. Biophys J. 1975 Jun;15(6):615–619. doi: 10.1016/S0006-3495(75)85842-5. [DOI] [PMC free article] [PubMed] [Google Scholar]
- Koppenhöfer E., Schmidt H. Incomplete sodium inactivation in nodes of Ranvier treated with scorpion venom. Experientia. 1968 Jan 15;24(1):41–42. doi: 10.1007/BF02136780. [DOI] [PubMed] [Google Scholar]
- Muller R. U., Finkelstein A. The electrostatic basis of Mg++ inhibition of transmitter release. Proc Natl Acad Sci U S A. 1974 Mar;71(3):923–926. doi: 10.1073/pnas.71.3.923. [DOI] [PMC free article] [PubMed] [Google Scholar]
- Narahashi T., Shapiro B. I., Deguchi T., Scuka M., Wang C. M. Effects of scorpion venom on squid axon membranes. Am J Physiol. 1972 Apr;222(4):850–857. doi: 10.1152/ajplegacy.1972.222.4.850. [DOI] [PubMed] [Google Scholar]
- Patrick J., Heinemann S. F., Lindstrom J., Schubert D., Steinbach J. H. Appearance of acetylcholine receptors during differentiation of a myogenic cell line. Proc Natl Acad Sci U S A. 1972 Oct;69(10):2762–2766. doi: 10.1073/pnas.69.10.2762. [DOI] [PMC free article] [PubMed] [Google Scholar]
- Patrick J., Lindstrom J. Autoimmune response to acetylcholine receptor. Science. 1973 May 25;180(4088):871–872. doi: 10.1126/science.180.4088.871. [DOI] [PubMed] [Google Scholar]
- Patrick J., Stallcup B. alpha-Bungarotoxin binding and cholinergic receptor function on a rat sympathetic nerve line. J Biol Chem. 1977 Dec 10;252(23):8629–8633. [PubMed] [Google Scholar]
- Patrick J., Stallcup W. B. Immunological distinction between acetylcholine receptor and the alpha-bungarotoxin-binding component on sympathetic neurons. Proc Natl Acad Sci U S A. 1977 Oct;74(10):4689–4692. doi: 10.1073/pnas.74.10.4689. [DOI] [PMC free article] [PubMed] [Google Scholar]
- Rink T. J. The influence of sodium on calcium movements and catecholamine release in thin slices of bovine adrenal medulla. J Physiol. 1977 Apr;266(2):297–325. doi: 10.1113/jphysiol.1977.sp011769. [DOI] [PMC free article] [PubMed] [Google Scholar]
- Ritchie A. K. Catecholamine secretion in a rat pheochromocytoma cell line: two pathways for calcium entry. J Physiol. 1979 Jan;286:541–561. doi: 10.1113/jphysiol.1979.sp012636. [DOI] [PMC free article] [PubMed] [Google Scholar]
- Romey G., Chicheportiche R., Lazdunski M., Rochat H., Miranda F., Lissitzky S. Scorpion neurotoxin - a presynaptic toxin which affects both Na+ and K+ channels in axons. Biochem Biophys Res Commun. 1975 May 5;64(1):115–121. doi: 10.1016/0006-291x(75)90226-0. [DOI] [PubMed] [Google Scholar]
- Rubin R. P. The role of calcium in the release of neurotransmitter substances and hormones. Pharmacol Rev. 1970 Sep;22(3):389–428. [PubMed] [Google Scholar]
- Schubert D., Heinemann S., Kidokoro Y. Cholinergic metabolism and synapse formation by a rat nerve cell line. Proc Natl Acad Sci U S A. 1977 Jun;74(6):2579–2583. doi: 10.1073/pnas.74.6.2579. [DOI] [PMC free article] [PubMed] [Google Scholar]
- Schubert D., LaCorbiere M., Whitlock C., Stallcup W. Alterations in the surface properties of cells responsive to nerve growth factor. Nature. 1978 Jun 29;273(5665):718–723. doi: 10.1038/273718a0. [DOI] [PubMed] [Google Scholar]
- Stallcup W. B., Cohn M. Correlation of surface antigens and cell type in cloned cell lines from the rat central nervous system. Exp Cell Res. 1976 Mar 15;98(2):285–297. doi: 10.1016/0014-4827(76)90440-7. [DOI] [PubMed] [Google Scholar]
- Stallcup W. B. Comparative pharmacology of voltage-dependent sodium channels. Brain Res. 1977 Oct 21;135(1):37–53. doi: 10.1016/0006-8993(77)91050-2. [DOI] [PubMed] [Google Scholar]
- Ulbricht W. The effect of veratridine on excitable membranes of nerve and muscle. Ergeb Physiol. 1969;61:18–71. doi: 10.1007/BFb0111446. [DOI] [PubMed] [Google Scholar]
- VOGT M. The secretion of the denervated adrenal medulla of the cat. Br J Pharmacol Chemother. 1952 Jun;7(2):325–330. doi: 10.1111/j.1476-5381.1952.tb01329.x. [DOI] [PMC free article] [PubMed] [Google Scholar]