Abstract
The light-driven proton pump, bacteriorhodopsin (bR) contains a retinal molecule with a Schiff base moiety that can participate in hydrogen-bonding interactions in an internal, water-containing channel. Here we combine quantum chemistry and molecular mechanics techniques to determine the geometries and energetics of retinal Schiff base-water interactions. Ab initio molecular orbital calculations are used to determine potential surfaces for water-Schiff base hydrogen-bonding and to characterize the energetics of rotation of the C-C single bond distal and adjacent to the Schiff base NH group. The ab initio results are combined with semiempirical quantum chemistry calculations to produce a data set used for the parameterization of a molecular mechanics energy function for retinal. Using the molecular mechanics force field the hydrated retinal and associated bR protein environment are energy-minimized and the resulting geometries examined. Two distinct sites are found in which water molecules can have hydrogen-bonding interactions with the Schiff base: one near the NH group of the Schiff base in a polar region directed towards the extracellular side, and the other near a retinal CH group in a relatively nonpolar region, directed towards the cytoplasmic side.
Full text
PDF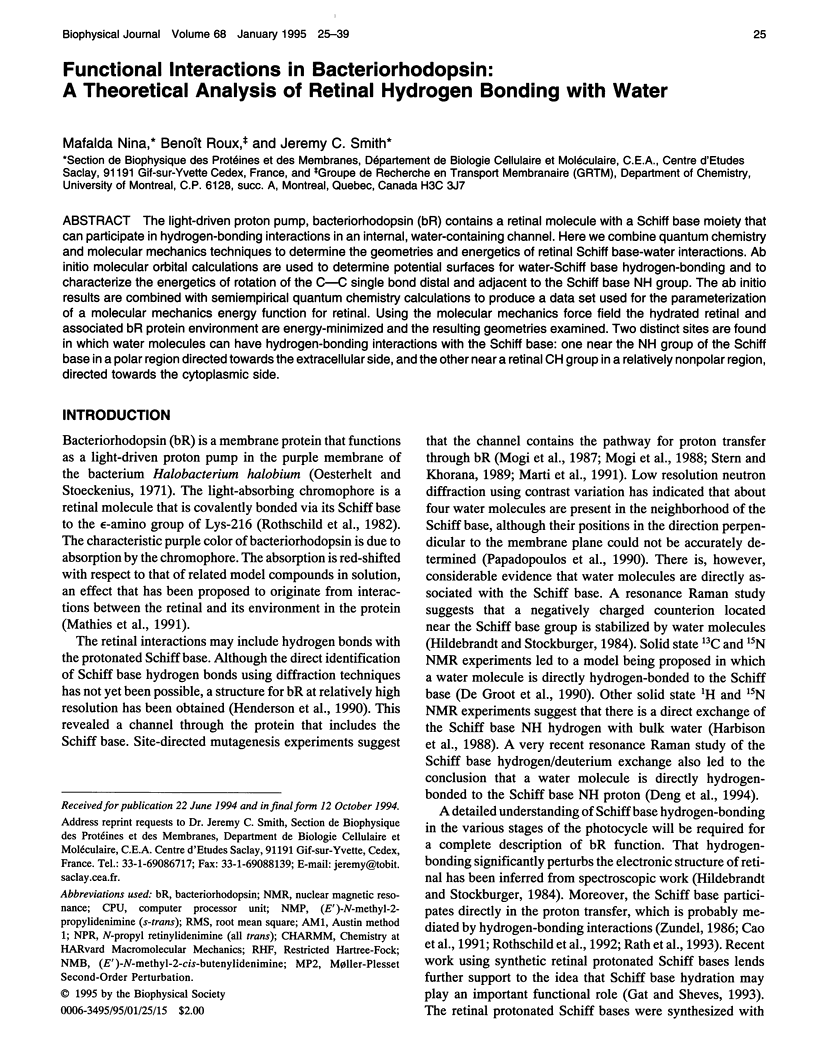
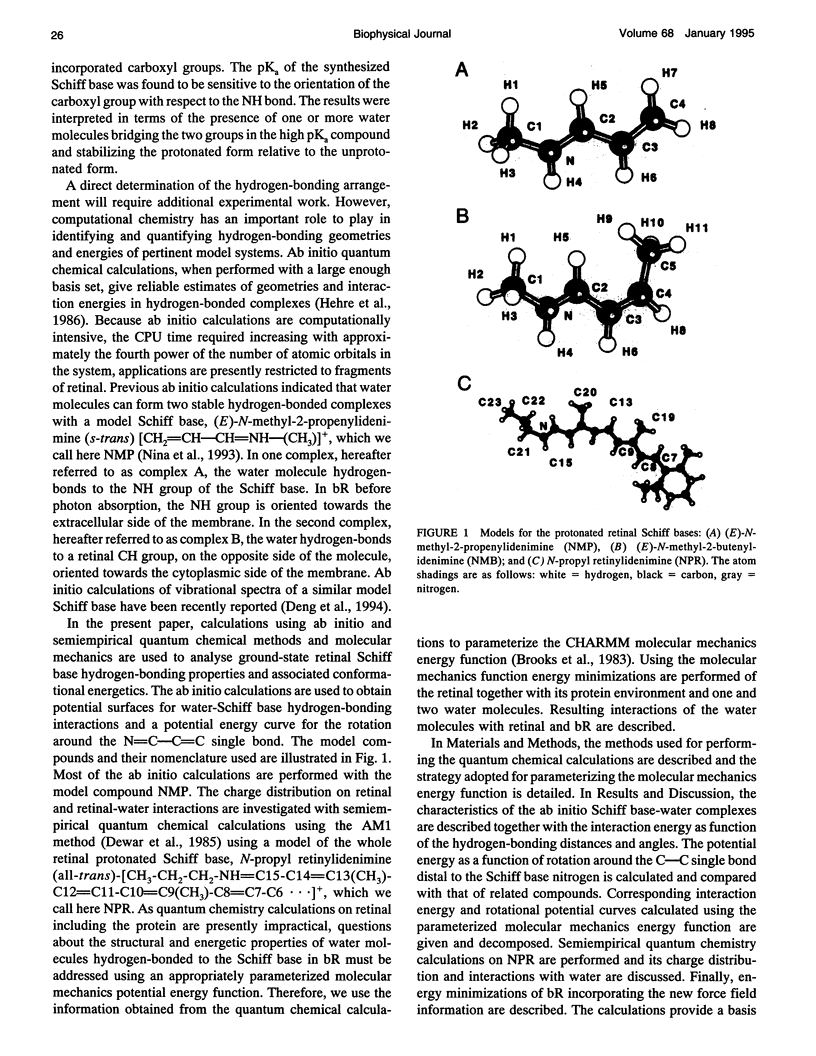
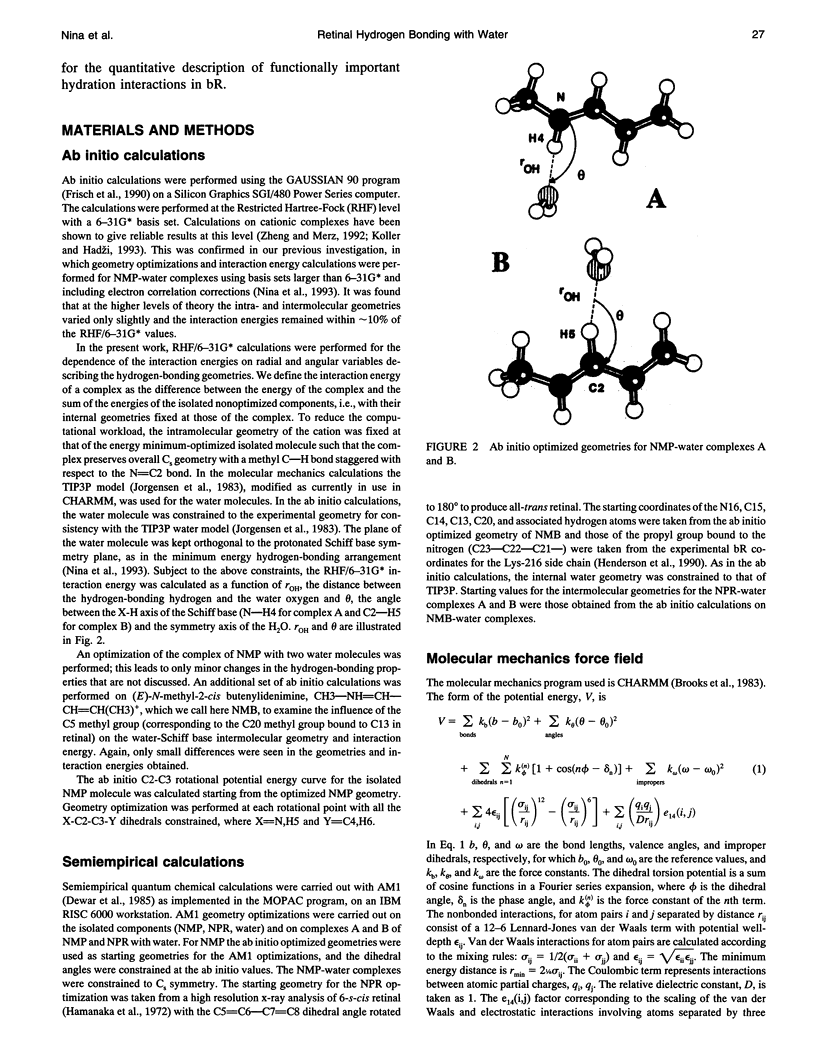
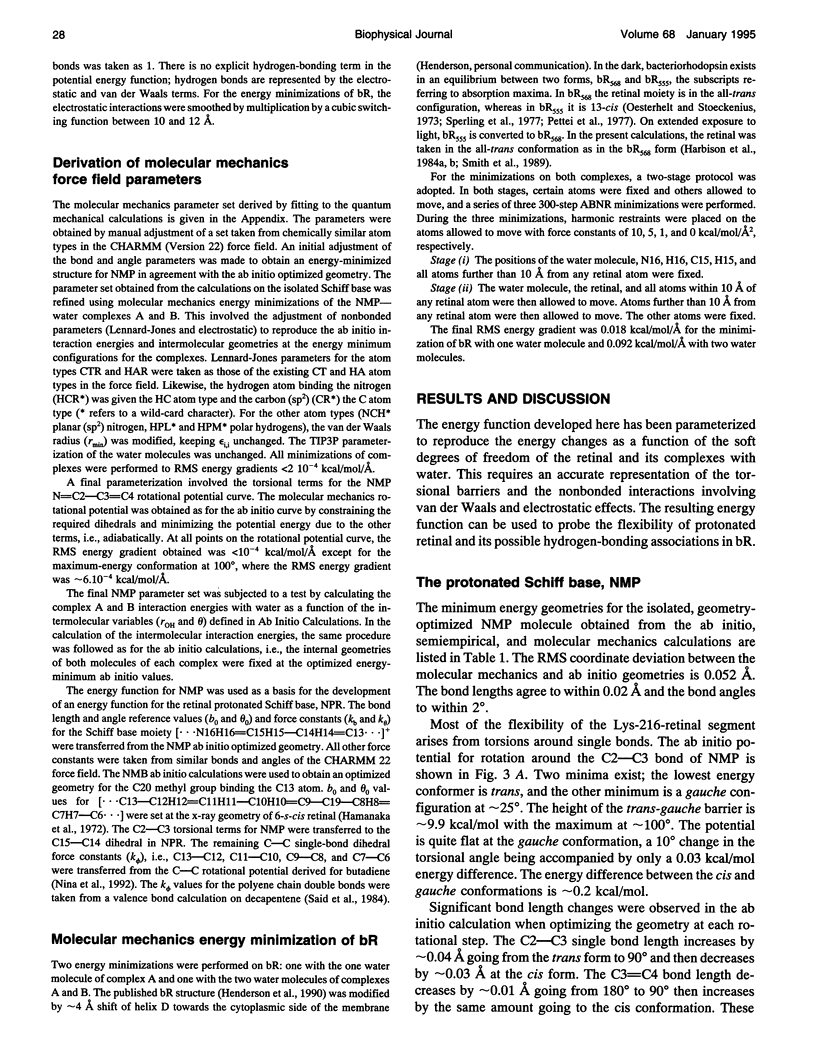
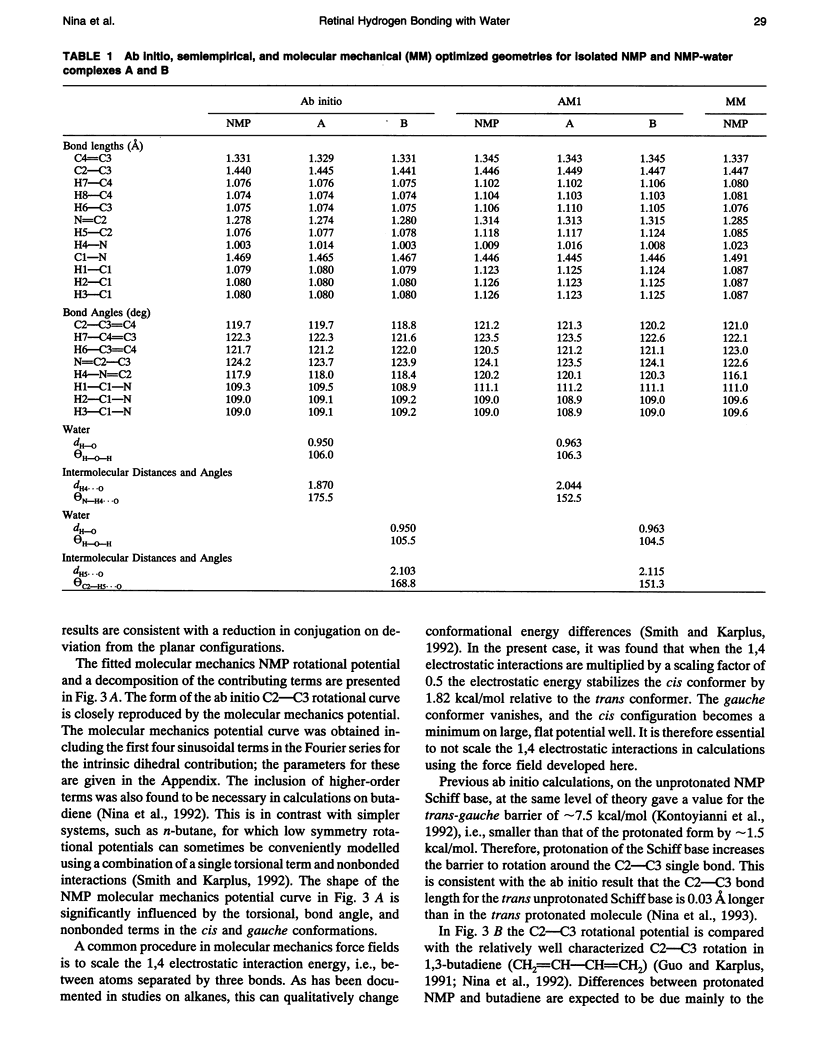
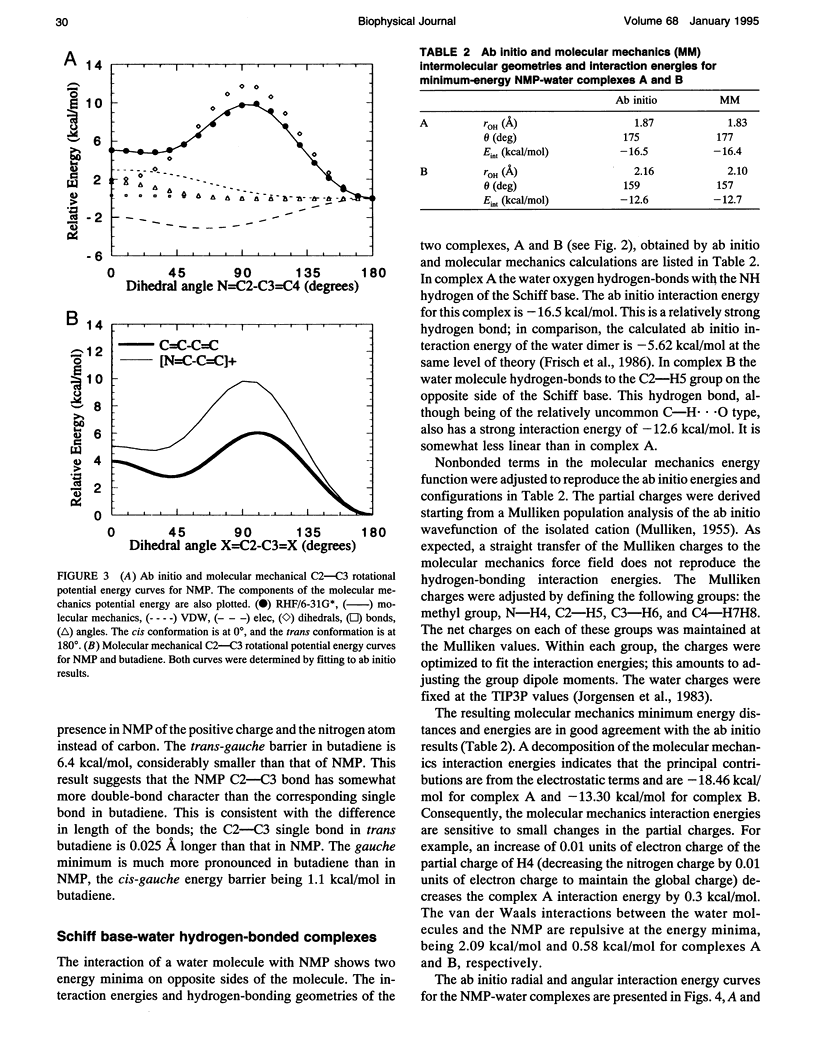
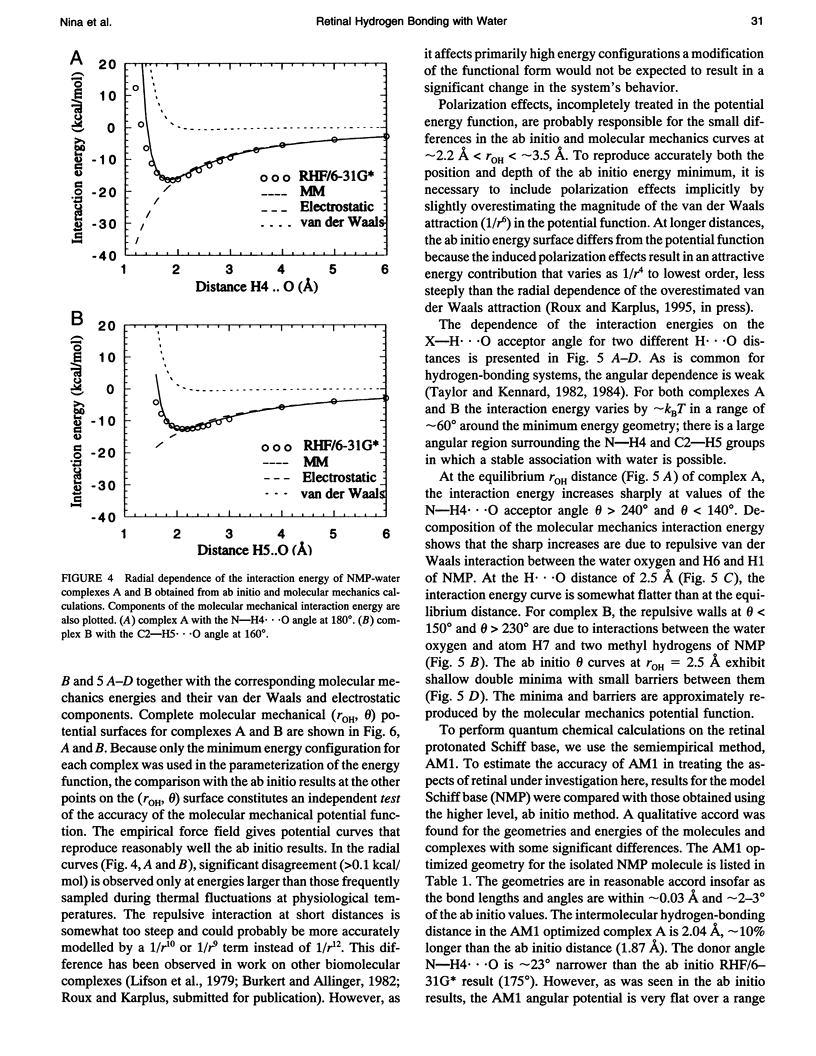
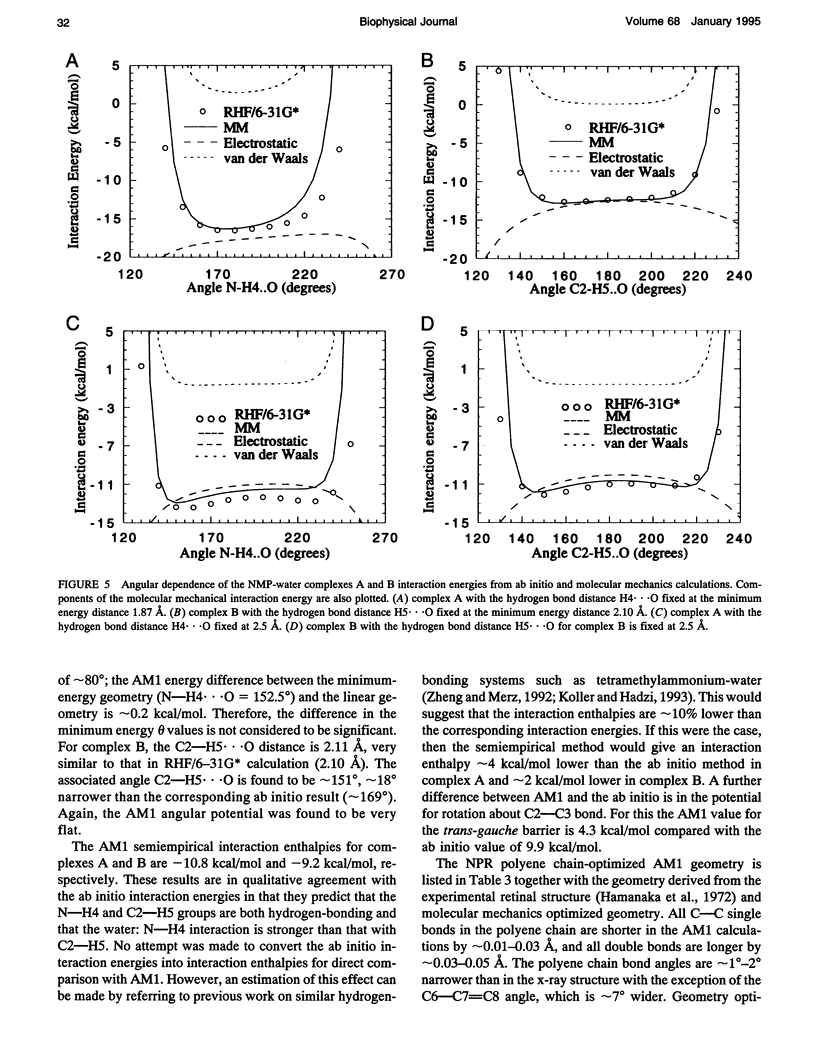
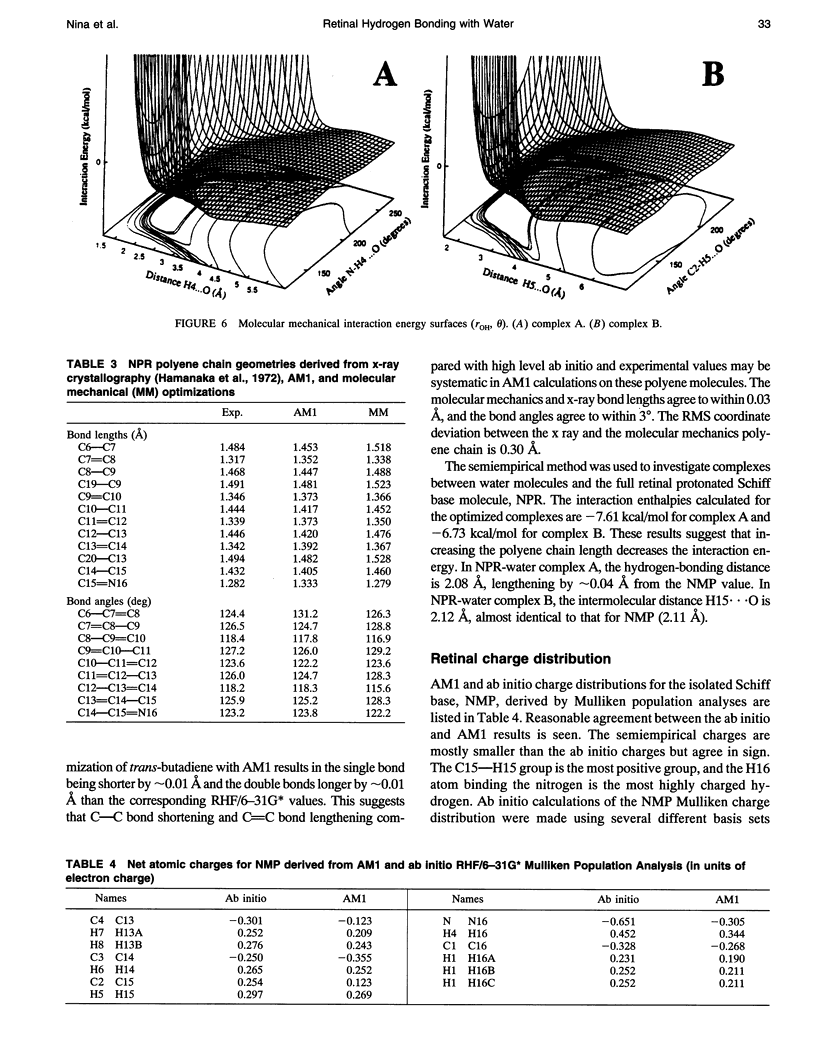
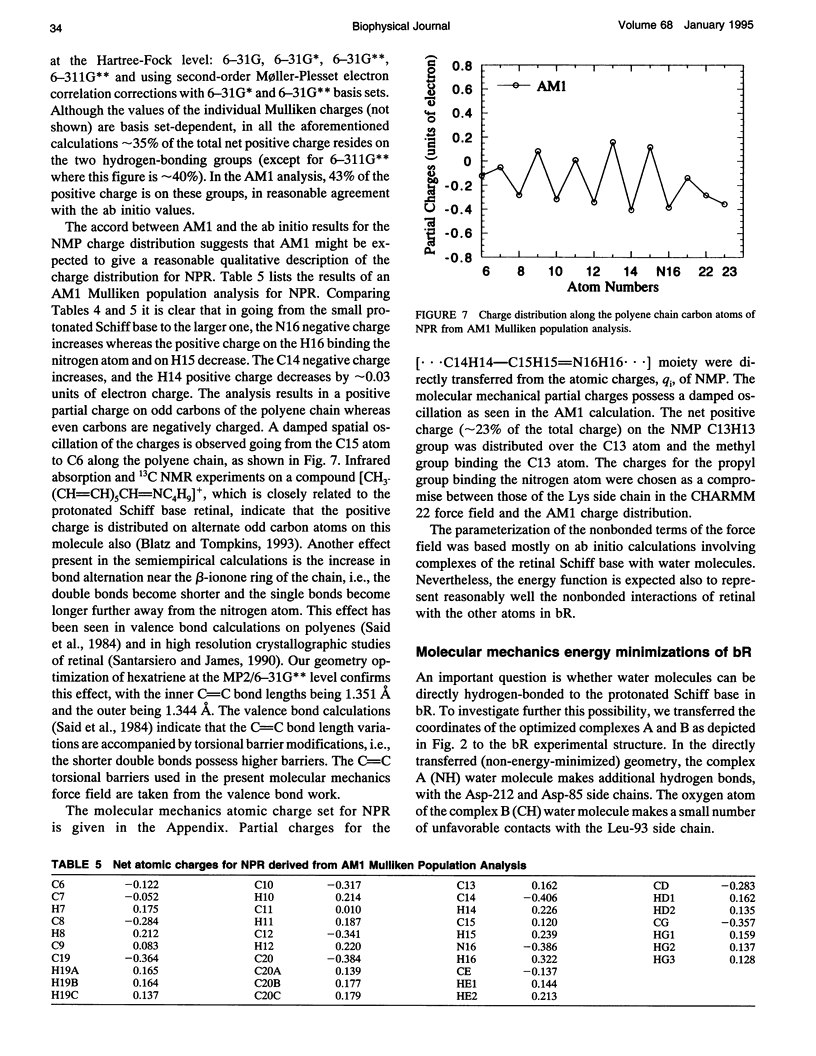
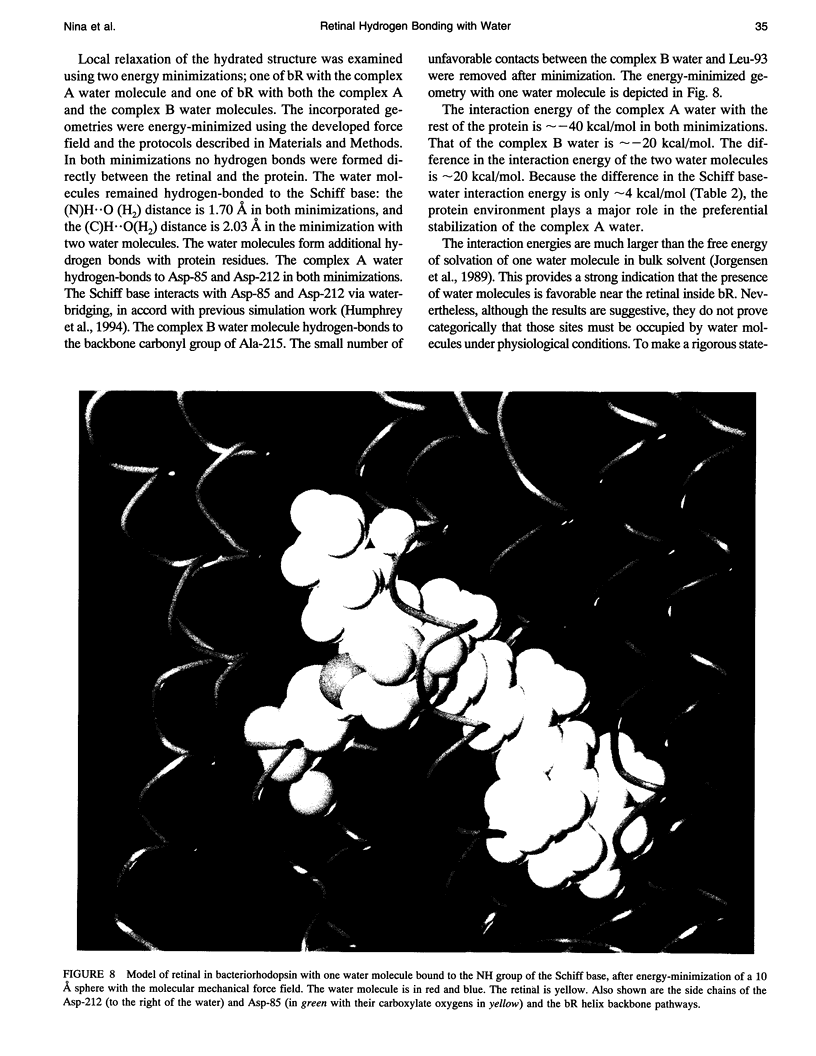
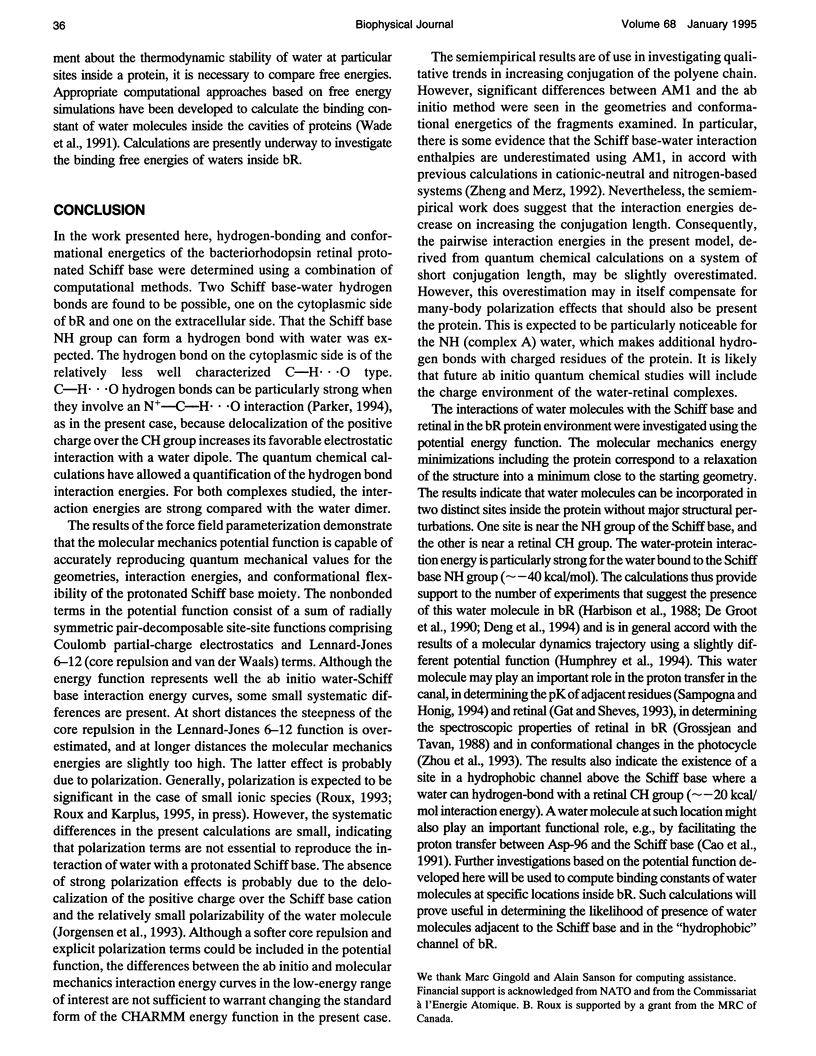
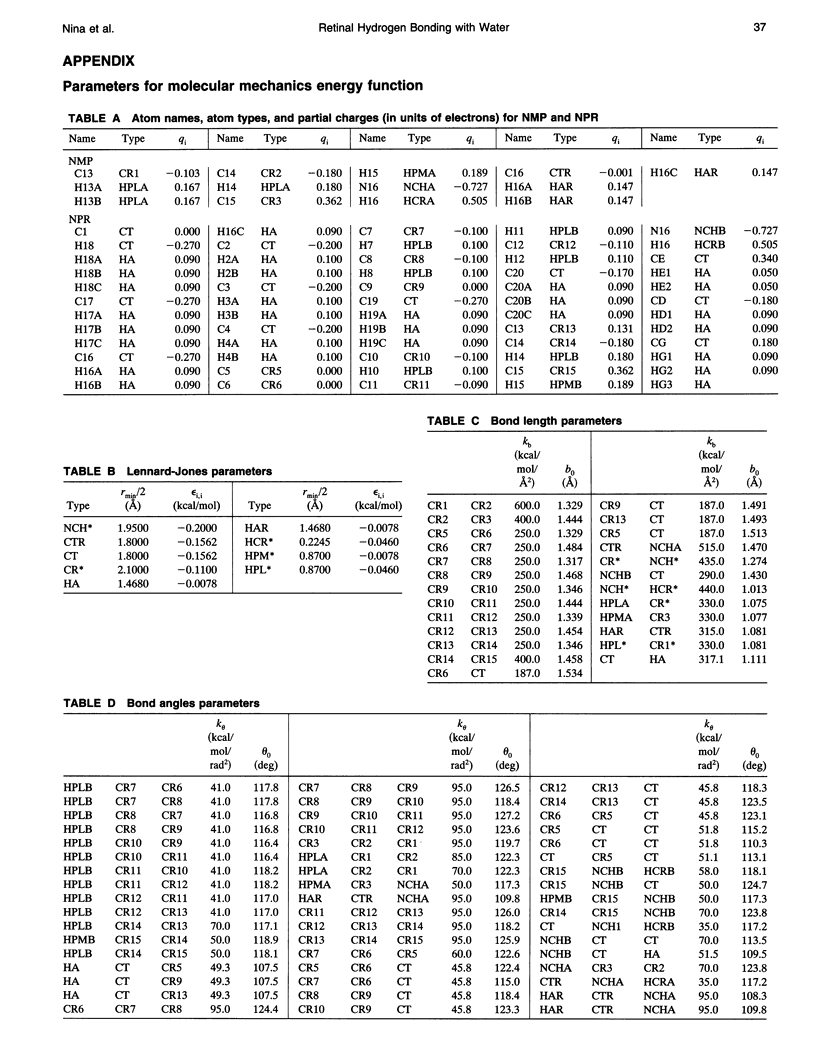
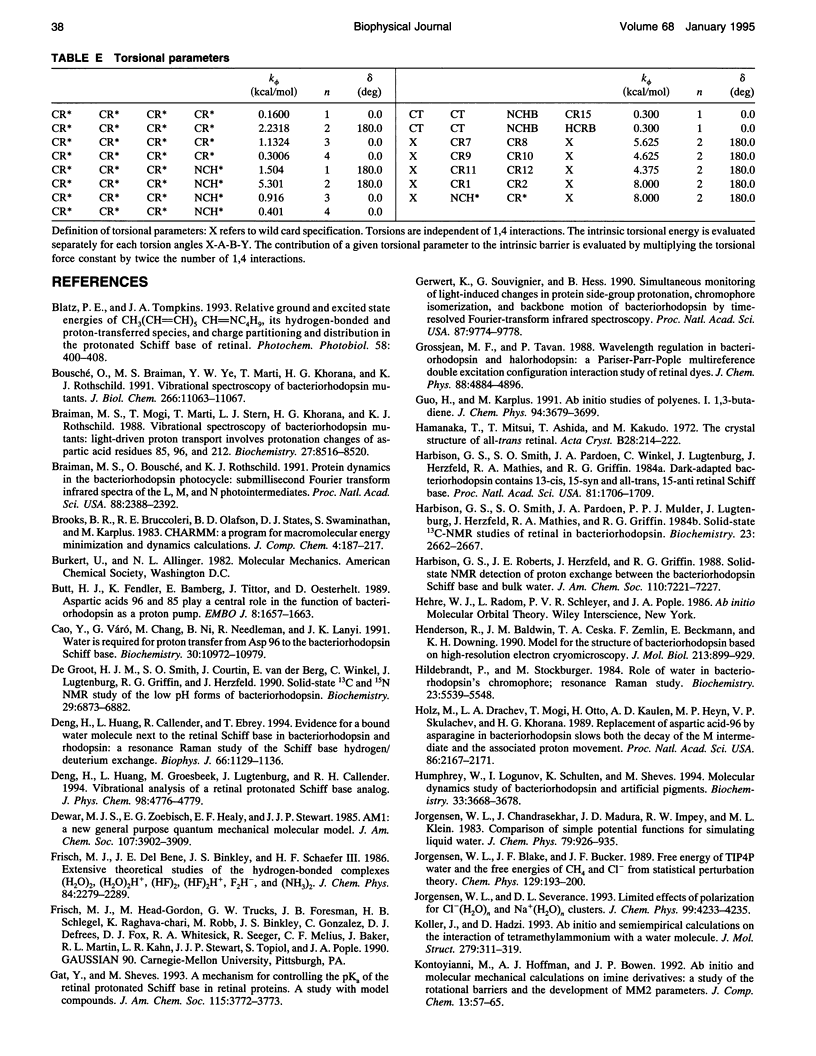
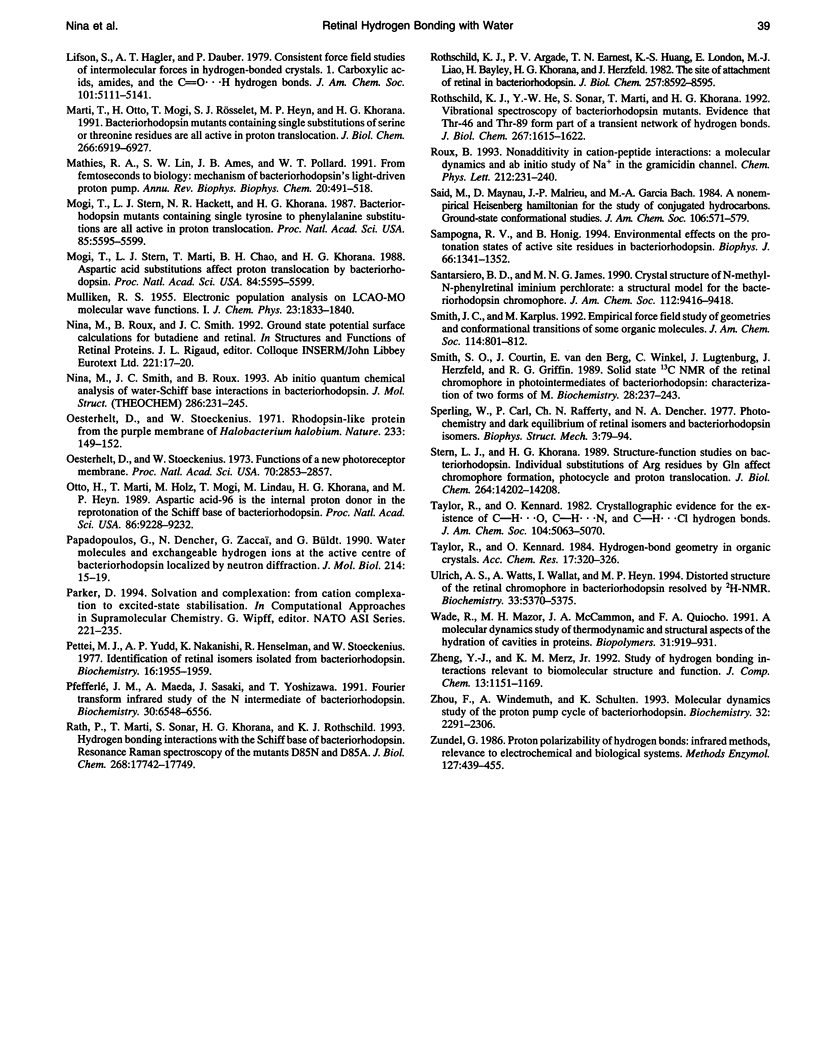
Images in this article
Selected References
These references are in PubMed. This may not be the complete list of references from this article.
- Blatz P. E., Tompkins J. A. Relative ground and excited state energies of CH3(CH = CH)5CH = NC4H9, its hydrogen-bonded and proton-transferred species, and charge partitioning and distribution in the protonated Schiff base of retinal. Photochem Photobiol. 1993 Sep;58(3):400–408. doi: 10.1111/j.1751-1097.1993.tb09581.x. [DOI] [PubMed] [Google Scholar]
- Bousché O., Braiman M., He Y. W., Marti T., Khorana H. G., Rothschild K. J. Vibrational spectroscopy of bacteriorhodopsin mutants. Evidence that ASP-96 deprotonates during the M----N transition. J Biol Chem. 1991 Jun 15;266(17):11063–11067. [PubMed] [Google Scholar]
- Braiman M. S., Bousché O., Rothschild K. J. Protein dynamics in the bacteriorhodopsin photocycle: submillisecond Fourier transform infrared spectra of the L, M, and N photointermediates. Proc Natl Acad Sci U S A. 1991 Mar 15;88(6):2388–2392. doi: 10.1073/pnas.88.6.2388. [DOI] [PMC free article] [PubMed] [Google Scholar]
- Braiman M. S., Mogi T., Marti T., Stern L. J., Khorana H. G., Rothschild K. J. Vibrational spectroscopy of bacteriorhodopsin mutants: light-driven proton transport involves protonation changes of aspartic acid residues 85, 96, and 212. Biochemistry. 1988 Nov 15;27(23):8516–8520. doi: 10.1021/bi00423a002. [DOI] [PubMed] [Google Scholar]
- Butt H. J., Fendler K., Bamberg E., Tittor J., Oesterhelt D. Aspartic acids 96 and 85 play a central role in the function of bacteriorhodopsin as a proton pump. EMBO J. 1989 Jun;8(6):1657–1663. doi: 10.1002/j.1460-2075.1989.tb03556.x. [DOI] [PMC free article] [PubMed] [Google Scholar]
- Cao Y., Váró G., Chang M., Ni B. F., Needleman R., Lanyi J. K. Water is required for proton transfer from aspartate-96 to the bacteriorhodopsin Schiff base. Biochemistry. 1991 Nov 12;30(45):10972–10979. doi: 10.1021/bi00109a023. [DOI] [PubMed] [Google Scholar]
- Deng H., Huang L., Callender R., Ebrey T. Evidence for a bound water molecule next to the retinal Schiff base in bacteriorhodopsin and rhodopsin: a resonance Raman study of the Schiff base hydrogen/deuterium exchange. Biophys J. 1994 Apr;66(4):1129–1136. doi: 10.1016/S0006-3495(94)80893-8. [DOI] [PMC free article] [PubMed] [Google Scholar]
- Gerwert K., Souvignier G., Hess B. Simultaneous monitoring of light-induced changes in protein side-group protonation, chromophore isomerization, and backbone motion of bacteriorhodopsin by time-resolved Fourier-transform infrared spectroscopy. Proc Natl Acad Sci U S A. 1990 Dec 15;87(24):9774–9778. doi: 10.1073/pnas.87.24.9774. [DOI] [PMC free article] [PubMed] [Google Scholar]
- Harbison G. S., Smith S. O., Pardoen J. A., Mulder P. P., Lugtenburg J., Herzfeld J., Mathies R., Griffin R. G. Solid-state 13C NMR studies of retinal in bacteriorhodopsin. Biochemistry. 1984 Jun 5;23(12):2662–2667. doi: 10.1021/bi00307a019. [DOI] [PubMed] [Google Scholar]
- Harbison G. S., Smith S. O., Pardoen J. A., Winkel C., Lugtenburg J., Herzfeld J., Mathies R., Griffin R. G. Dark-adapted bacteriorhodopsin contains 13-cis, 15-syn and all-trans, 15-anti retinal Schiff bases. Proc Natl Acad Sci U S A. 1984 Mar;81(6):1706–1709. doi: 10.1073/pnas.81.6.1706. [DOI] [PMC free article] [PubMed] [Google Scholar]
- Henderson R., Baldwin J. M., Ceska T. A., Zemlin F., Beckmann E., Downing K. H. Model for the structure of bacteriorhodopsin based on high-resolution electron cryo-microscopy. J Mol Biol. 1990 Jun 20;213(4):899–929. doi: 10.1016/S0022-2836(05)80271-2. [DOI] [PubMed] [Google Scholar]
- Holz M., Drachev L. A., Mogi T., Otto H., Kaulen A. D., Heyn M. P., Skulachev V. P., Khorana H. G. Replacement of aspartic acid-96 by asparagine in bacteriorhodopsin slows both the decay of the M intermediate and the associated proton movement. Proc Natl Acad Sci U S A. 1989 Apr;86(7):2167–2171. doi: 10.1073/pnas.86.7.2167. [DOI] [PMC free article] [PubMed] [Google Scholar]
- Humphrey W., Logunov I., Schulten K., Sheves M. Molecular dynamics study of bacteriorhodopsin and artificial pigments. Biochemistry. 1994 Mar 29;33(12):3668–3678. doi: 10.1021/bi00178a025. [DOI] [PubMed] [Google Scholar]
- Marti T., Otto H., Mogi T., Rösselet S. J., Heyn M. P., Khorana H. G. Bacteriorhodopsin mutants containing single substitutions of serine or threonine residues are all active in proton translocation. J Biol Chem. 1991 Apr 15;266(11):6919–6927. [PubMed] [Google Scholar]
- Mathies R. A., Lin S. W., Ames J. B., Pollard W. T. From femtoseconds to biology: mechanism of bacteriorhodopsin's light-driven proton pump. Annu Rev Biophys Biophys Chem. 1991;20:491–518. doi: 10.1146/annurev.bb.20.060191.002423. [DOI] [PubMed] [Google Scholar]
- Mogi T., Stern L. J., Hackett N. R., Khorana H. G. Bacteriorhodopsin mutants containing single tyrosine to phenylalanine substitutions are all active in proton translocation. Proc Natl Acad Sci U S A. 1987 Aug;84(16):5595–5599. doi: 10.1073/pnas.84.16.5595. [DOI] [PMC free article] [PubMed] [Google Scholar]
- Mogi T., Stern L. J., Hackett N. R., Khorana H. G. Bacteriorhodopsin mutants containing single tyrosine to phenylalanine substitutions are all active in proton translocation. Proc Natl Acad Sci U S A. 1987 Aug;84(16):5595–5599. doi: 10.1073/pnas.84.16.5595. [DOI] [PMC free article] [PubMed] [Google Scholar]
- Oesterhelt D., Stoeckenius W. Functions of a new photoreceptor membrane. Proc Natl Acad Sci U S A. 1973 Oct;70(10):2853–2857. doi: 10.1073/pnas.70.10.2853. [DOI] [PMC free article] [PubMed] [Google Scholar]
- Oesterhelt D., Stoeckenius W. Rhodopsin-like protein from the purple membrane of Halobacterium halobium. Nat New Biol. 1971 Sep 29;233(39):149–152. doi: 10.1038/newbio233149a0. [DOI] [PubMed] [Google Scholar]
- Otto H., Marti T., Holz M., Mogi T., Lindau M., Khorana H. G., Heyn M. P. Aspartic acid-96 is the internal proton donor in the reprotonation of the Schiff base of bacteriorhodopsin. Proc Natl Acad Sci U S A. 1989 Dec;86(23):9228–9232. doi: 10.1073/pnas.86.23.9228. [DOI] [PMC free article] [PubMed] [Google Scholar]
- Papadopoulos G., Dencher N. A., Zaccai G., Büldt G. Water molecules and exchangeable hydrogen ions at the active centre of bacteriorhodopsin localized by neutron diffraction. Elements of the proton pathway? J Mol Biol. 1990 Jul 5;214(1):15–19. doi: 10.1016/0022-2836(90)90140-h. [DOI] [PubMed] [Google Scholar]
- Pettei M. J., Yudd A. P., Nakanishi K., Henselman R., Stoeckenius W. Identification of retinal isomers isolated from bacteriorhodopsin. Biochemistry. 1977 May 3;16(9):1955–1959. doi: 10.1021/bi00628a031. [DOI] [PubMed] [Google Scholar]
- Pfefferlé J. M., Maeda A., Sasaki J., Yoshizawa T. Fourier transform infrared study of the N intermediate of bacteriorhodopsin. Biochemistry. 1991 Jul 2;30(26):6548–6556. doi: 10.1021/bi00240a027. [DOI] [PubMed] [Google Scholar]
- Rath P., Marti T., Sonar S., Khorana H. G., Rothschild K. J. Hydrogen bonding interactions with the Schiff base of bacteriorhodopsin. Resonance Raman spectroscopy of the mutants D85N and D85A. J Biol Chem. 1993 Aug 25;268(24):17742–17749. [PubMed] [Google Scholar]
- Rothschild K. J., Argade P. V., Earnest T. N., Huang K. S., London E., Liao M. J., Bayley H., Khorana H. G., Herzfeld J. The site of attachment of retinal in bacteriorhodopsin. A resonance Raman study. J Biol Chem. 1982 Aug 10;257(15):8592–8595. [PubMed] [Google Scholar]
- Rothschild K. J., He Y. W., Sonar S., Marti T., Khorana H. G. Vibrational spectroscopy of bacteriorhodopsin mutants. Evidence that Thr-46 and Thr-89 form part of a transient network of hydrogen bonds. J Biol Chem. 1992 Jan 25;267(3):1615–1622. [PubMed] [Google Scholar]
- Sampogna R. V., Honig B. Environmental effects on the protonation states of active site residues in bacteriorhodopsin. Biophys J. 1994 May;66(5):1341–1352. doi: 10.1016/S0006-3495(94)80925-7. [DOI] [PMC free article] [PubMed] [Google Scholar]
- Smith S. O., Courtin J., van den Berg E., Winkel C., Lugtenburg J., Herzfeld J., Griffin R. G. Solid-state 13C NMR of the retinal chromophore in photointermediates of bacteriorhodopsin: characterization of two forms of M. Biochemistry. 1989 Jan 10;28(1):237–243. doi: 10.1021/bi00427a033. [DOI] [PubMed] [Google Scholar]
- Sperling W., Carl P., Rafferty Ch, Dencher N. A. Photochemistry and dark equilibrium of retinal isomers and bacteriorhodopsin isomers. Biophys Struct Mech. 1977 Jun 29;3(2):79–94. doi: 10.1007/BF00535798. [DOI] [PubMed] [Google Scholar]
- Stern L. J., Khorana H. G. Structure-function studies on bacteriorhodopsin. X. Individual substitutions of arginine residues by glutamine affect chromophore formation, photocycle, and proton translocation. J Biol Chem. 1989 Aug 25;264(24):14202–14208. [PubMed] [Google Scholar]
- Ulrich A. S., Watts A., Wallat I., Heyn M. P. Distorted structure of the retinal chromophore in bacteriorhodopsin resolved by 2H-NMR. Biochemistry. 1994 May 10;33(18):5370–5375. doi: 10.1021/bi00184a003. [DOI] [PubMed] [Google Scholar]
- Wade R. C., Mazor M. H., McCammon J. A., Quiocho F. A. A molecular dynamics study of thermodynamic and structural aspects of the hydration of cavities in proteins. Biopolymers. 1991 Jul;31(8):919–931. doi: 10.1002/bip.360310802. [DOI] [PubMed] [Google Scholar]
- Zhou F., Windemuth A., Schulten K. Molecular dynamics study of the proton pump cycle of bacteriorhodopsin. Biochemistry. 1993 Mar 9;32(9):2291–2306. doi: 10.1021/bi00060a022. [DOI] [PubMed] [Google Scholar]
- Zundel G. Proton polarizability of hydrogen bonds: infrared methods, relevance to electrochemical and biological systems. Methods Enzymol. 1986;127:439–455. doi: 10.1016/0076-6879(86)27034-2. [DOI] [PubMed] [Google Scholar]
- de Groot H. J., Smith S. O., Courtin J., van den Berg E., Winkel C., Lugtenburg J., Griffin R. G., Herzfeld J. Solid-state 13C and 15N NMR study of the low pH forms of bacteriorhodopsin. Biochemistry. 1990 Jul 24;29(29):6873–6883. doi: 10.1021/bi00481a017. [DOI] [PubMed] [Google Scholar]