Abstract
A persistent problem with the rotating cross-bridge model for muscle contraction has been the inability to detect any large conformational changes within the myosin molecule to account for a working stroke of 5-10 nm. The recent crystal structure of myosin subfragment-1 suggests a solution to this problem by showing the presence of two distinct domains: a catalytic or motor domain, from which extends a long, 8.5-nm alpha-helix that is stabilized by the regulatory and essential light chains. Rayment et al. (1993) proposed that closure of a cleft in the motor domain could rotate the light chain-binding domain by a sufficient distance to account for the power stroke. With the development of new in vitro motility assays, and the ability to prepare unusual myosins by biochemical and molecular biological methods, we can now examine this hypothesis and explore the role of the light chains in generating force and movement. Here we will review some of these recent data and outline a possible mechanism for how light chains regulate contractile properties.
Full text
PDF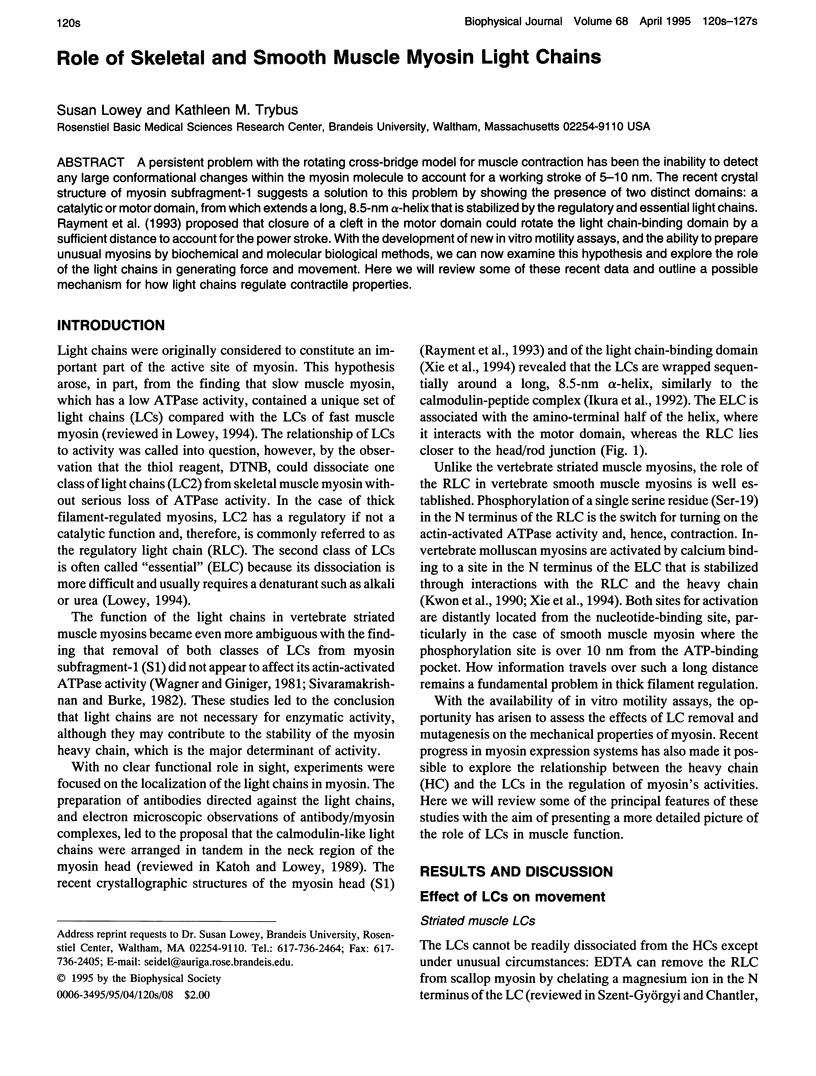
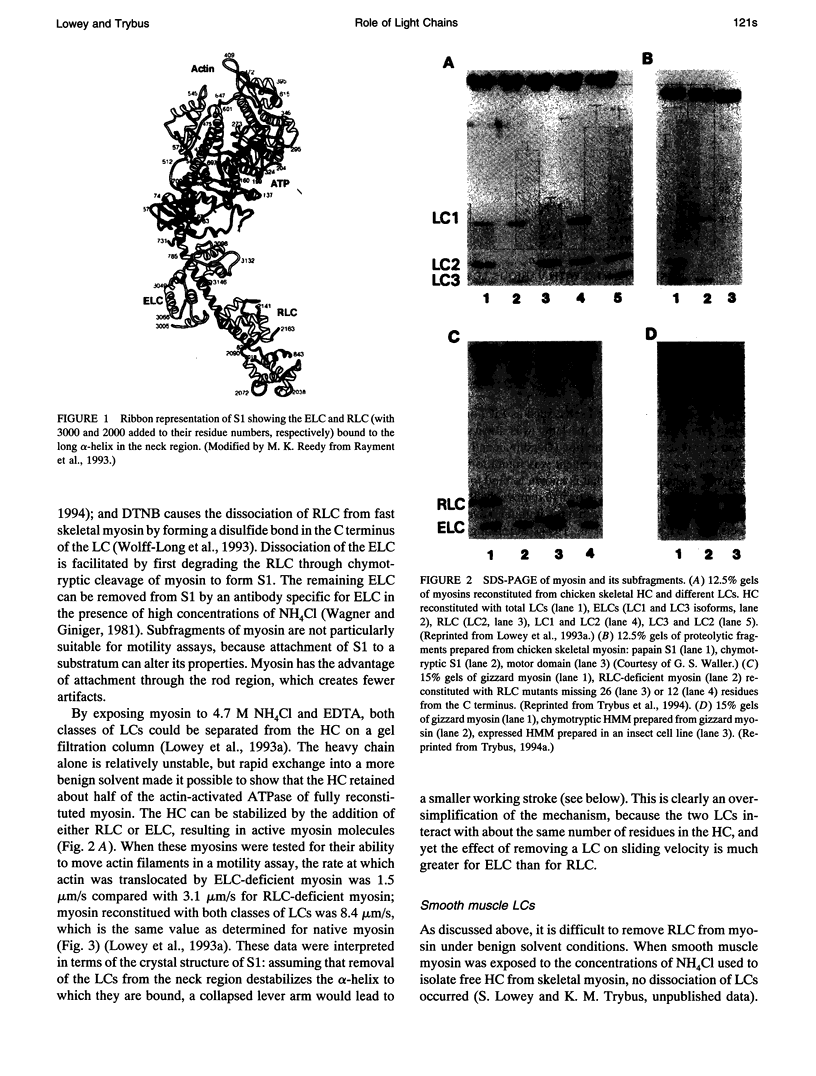
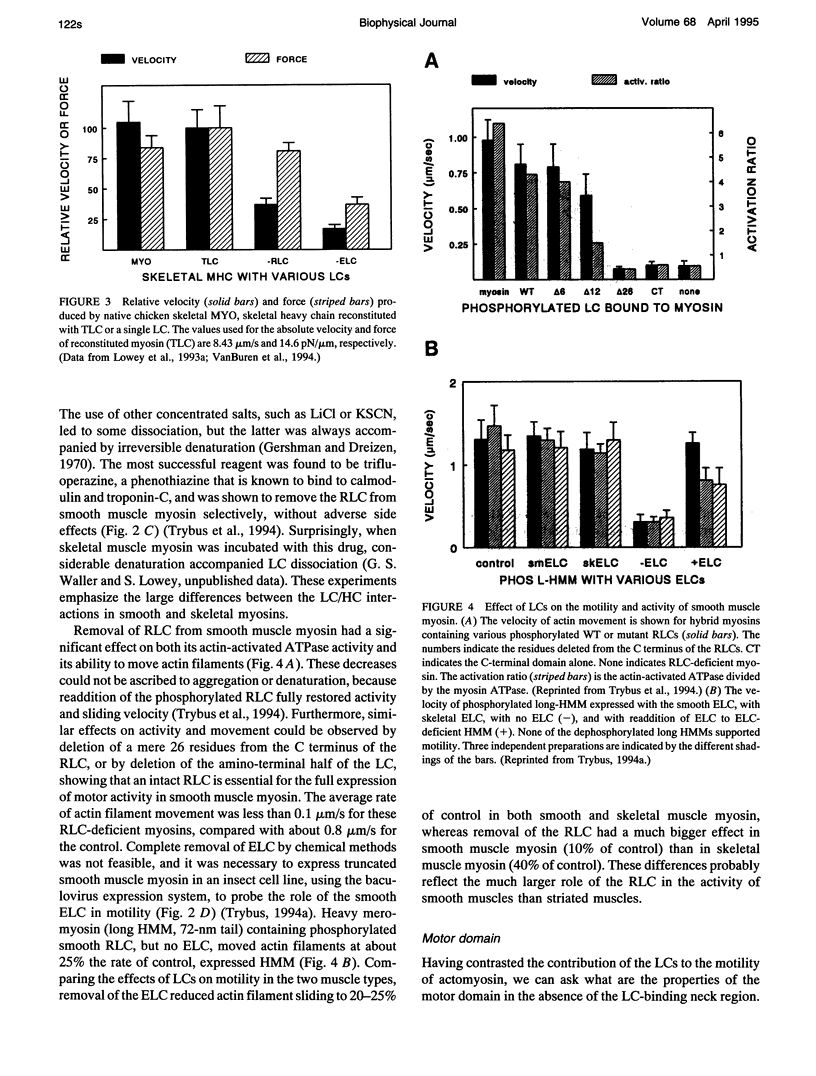
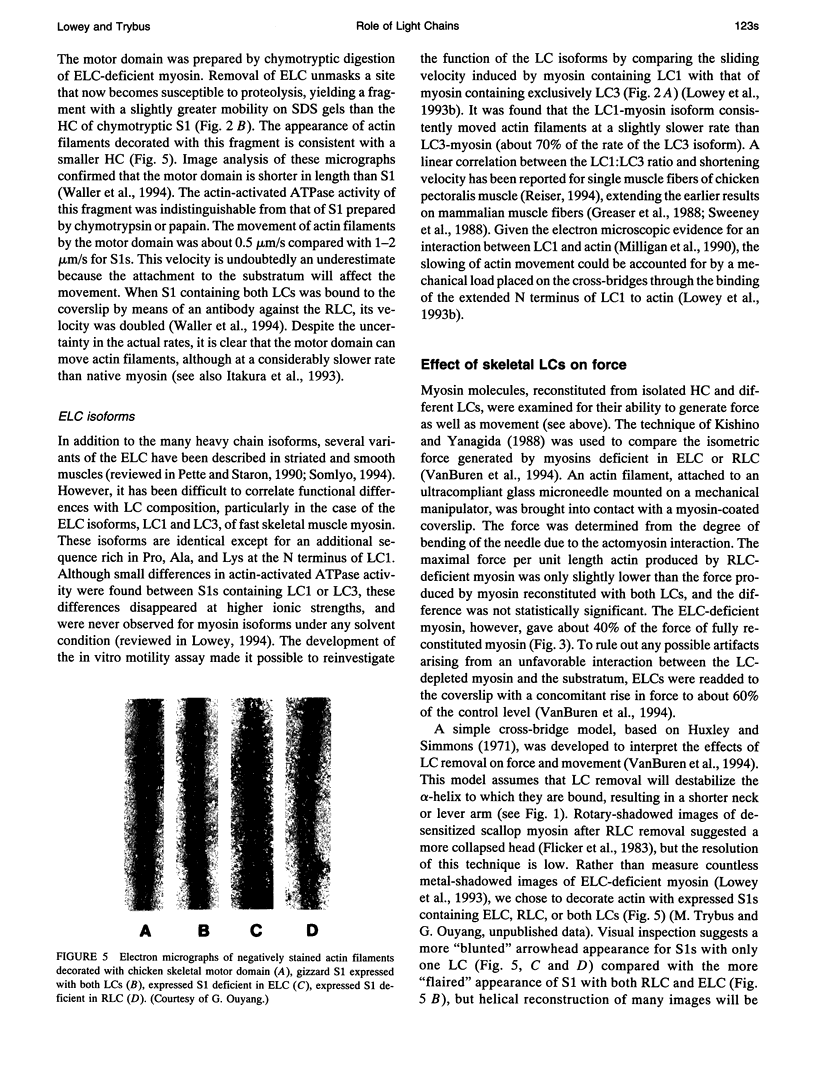
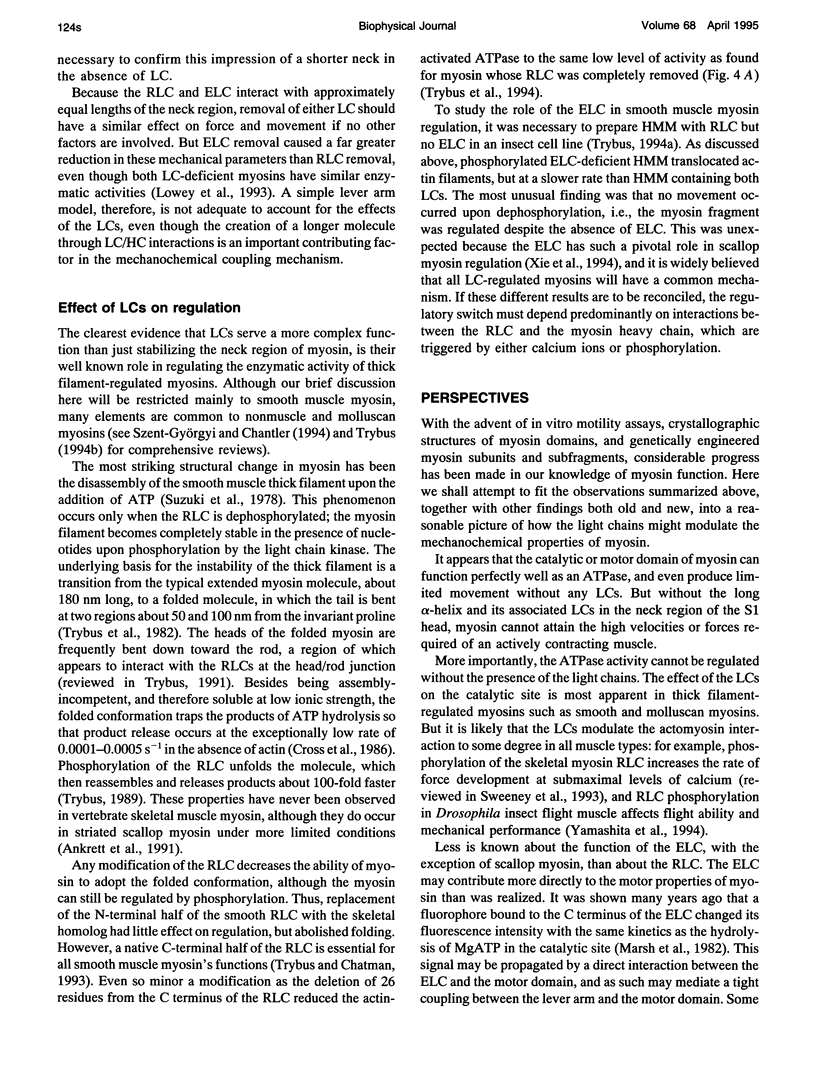
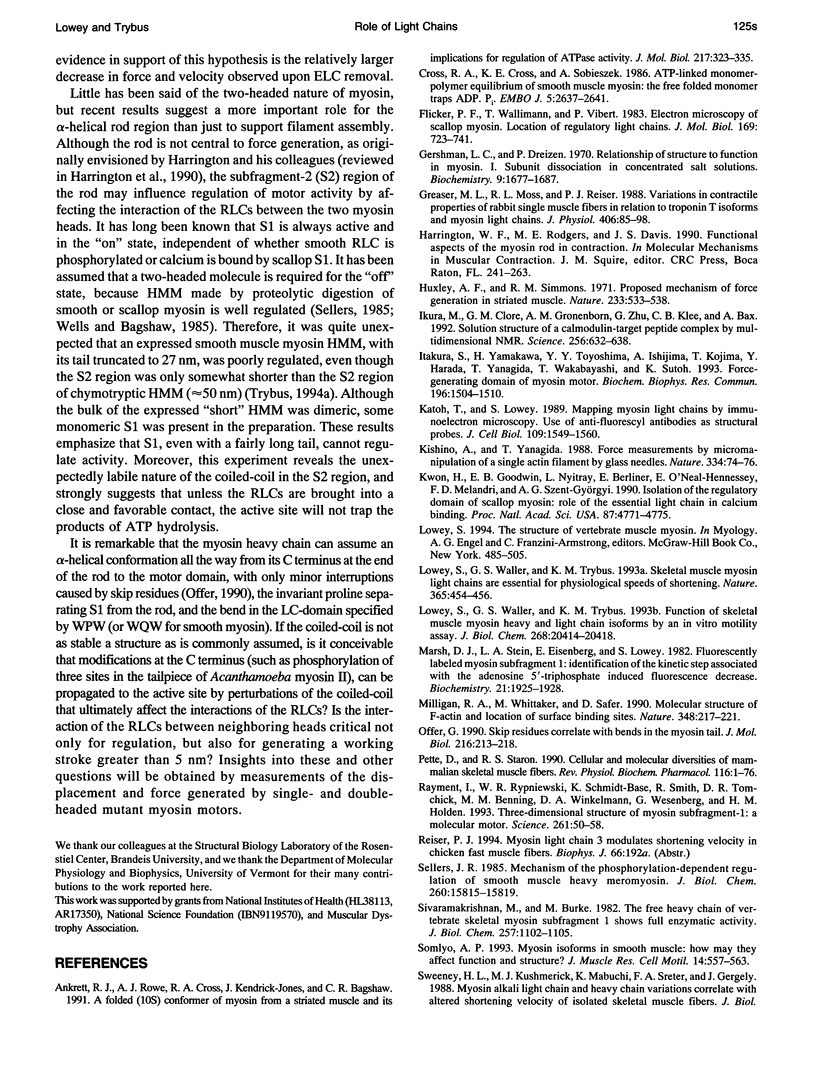
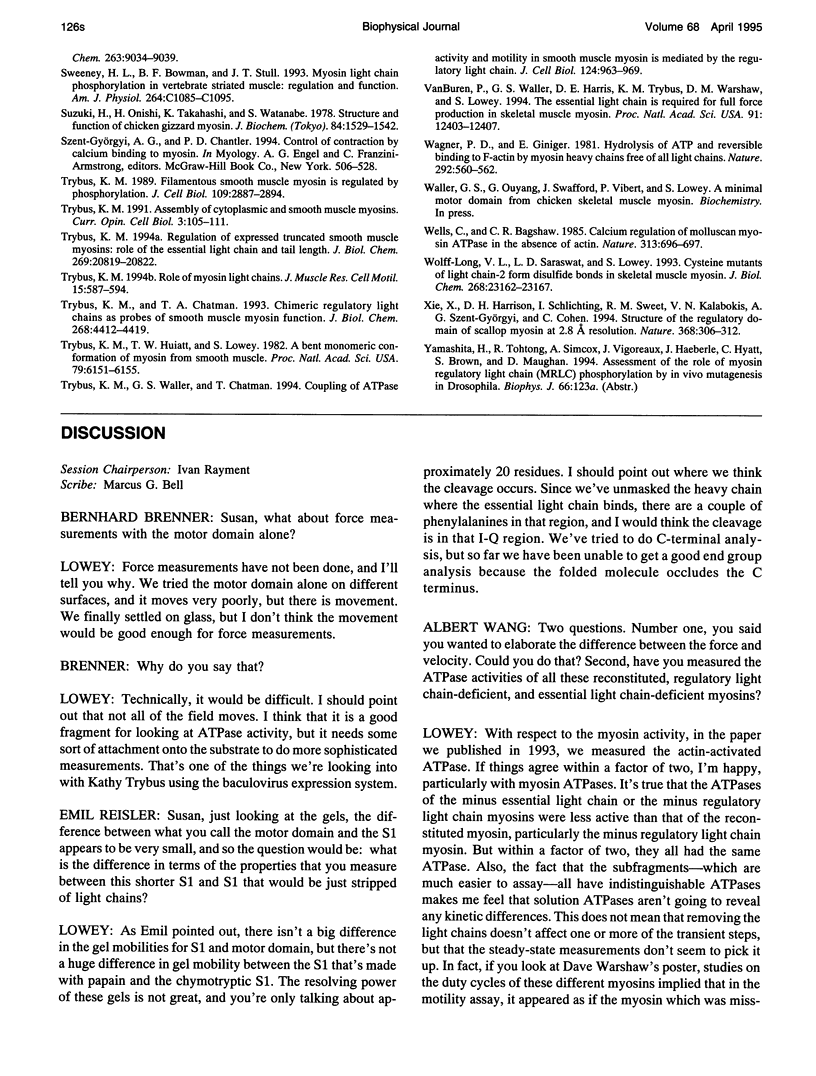
Images in this article
Selected References
These references are in PubMed. This may not be the complete list of references from this article.
- Ankrett R. J., Rowe A. J., Cross R. A., Kendrick-Jones J., Bagshaw C. R. A folded (10 S) conformer of myosin from a striated muscle and its implications for regulation of ATPase activity. J Mol Biol. 1991 Jan 20;217(2):323–335. doi: 10.1016/0022-2836(91)90546-i. [DOI] [PubMed] [Google Scholar]
- Cross R. A., Cross K. E., Sobieszek A. ATP-linked monomer-polymer equilibrium of smooth muscle myosin: the free folded monomer traps ADP.Pi. EMBO J. 1986 Oct;5(10):2637–2641. doi: 10.1002/j.1460-2075.1986.tb04545.x. [DOI] [PMC free article] [PubMed] [Google Scholar]
- Flicker P. F., Wallimann T., Vibert P. Electron microscopy of scallop myosin. Location of regulatory light chains. J Mol Biol. 1983 Sep 25;169(3):723–741. doi: 10.1016/s0022-2836(83)80167-3. [DOI] [PubMed] [Google Scholar]
- Gershman L. C., Dreizen P. Relationship of structure to function in myosin. I. Subunit dissociation in concentrated salt solutions. Biochemistry. 1970 Apr 14;9(8):1677–1687. doi: 10.1021/bi00810a005. [DOI] [PubMed] [Google Scholar]
- Greaser M. L., Moss R. L., Reiser P. J. Variations in contractile properties of rabbit single muscle fibres in relation to troponin T isoforms and myosin light chains. J Physiol. 1988 Dec;406:85–98. doi: 10.1113/jphysiol.1988.sp017370. [DOI] [PMC free article] [PubMed] [Google Scholar]
- Huxley A. F., Simmons R. M. Proposed mechanism of force generation in striated muscle. Nature. 1971 Oct 22;233(5321):533–538. doi: 10.1038/233533a0. [DOI] [PubMed] [Google Scholar]
- Ikura M., Clore G. M., Gronenborn A. M., Zhu G., Klee C. B., Bax A. Solution structure of a calmodulin-target peptide complex by multidimensional NMR. Science. 1992 May 1;256(5057):632–638. doi: 10.1126/science.1585175. [DOI] [PubMed] [Google Scholar]
- Itakura S., Yamakawa H., Toyoshima Y. Y., Ishijima A., Kojima T., Harada Y., Yanagida T., Wakabayashi T., Sutoh K. Force-generating domain of myosin motor. Biochem Biophys Res Commun. 1993 Nov 15;196(3):1504–1510. doi: 10.1006/bbrc.1993.2422. [DOI] [PubMed] [Google Scholar]
- Katoh T., Lowey S. Mapping myosin light chains by immunoelectron microscopy. Use of anti-fluorescyl antibodies as structural probes. J Cell Biol. 1989 Oct;109(4 Pt 1):1549–1560. doi: 10.1083/jcb.109.4.1549. [DOI] [PMC free article] [PubMed] [Google Scholar]
- Kishino A., Yanagida T. Force measurements by micromanipulation of a single actin filament by glass needles. Nature. 1988 Jul 7;334(6177):74–76. doi: 10.1038/334074a0. [DOI] [PubMed] [Google Scholar]
- Kwon H., Goodwin E. B., Nyitray L., Berliner E., O'Neall-Hennessey E., Melandri F. D., Szent-Györgyi A. G. Isolation of the regulatory domain of scallop myosin: role of the essential light chain in calcium binding. Proc Natl Acad Sci U S A. 1990 Jun;87(12):4771–4775. doi: 10.1073/pnas.87.12.4771. [DOI] [PMC free article] [PubMed] [Google Scholar]
- Lowey S., Waller G. S., Trybus K. M. Function of skeletal muscle myosin heavy and light chain isoforms by an in vitro motility assay. J Biol Chem. 1993 Sep 25;268(27):20414–20418. [PubMed] [Google Scholar]
- Lowey S., Waller G. S., Trybus K. M. Skeletal muscle myosin light chains are essential for physiological speeds of shortening. Nature. 1993 Sep 30;365(6445):454–456. doi: 10.1038/365454a0. [DOI] [PubMed] [Google Scholar]
- Marsh D. J., Stein L. A., Eisenberg E., Lowey S. Fluorescently labeled myosin subfragment 1: identification of the kinetic step associated with the adenosine 5'-triphosphate induced fluorescence decrease. Biochemistry. 1982 Apr 13;21(8):1925–1928. doi: 10.1021/bi00537a035. [DOI] [PubMed] [Google Scholar]
- Milligan R. A., Whittaker M., Safer D. Molecular structure of F-actin and location of surface binding sites. Nature. 1990 Nov 15;348(6298):217–221. doi: 10.1038/348217a0. [DOI] [PubMed] [Google Scholar]
- Offer G. Skip residues correlate with bends in the myosin tail. J Mol Biol. 1990 Nov 20;216(2):213–218. doi: 10.1016/S0022-2836(05)80309-2. [DOI] [PubMed] [Google Scholar]
- Pette D., Staron R. S. Cellular and molecular diversities of mammalian skeletal muscle fibers. Rev Physiol Biochem Pharmacol. 1990;116:1–76. doi: 10.1007/3540528806_3. [DOI] [PubMed] [Google Scholar]
- Rayment I., Rypniewski W. R., Schmidt-Bäse K., Smith R., Tomchick D. R., Benning M. M., Winkelmann D. A., Wesenberg G., Holden H. M. Three-dimensional structure of myosin subfragment-1: a molecular motor. Science. 1993 Jul 2;261(5117):50–58. doi: 10.1126/science.8316857. [DOI] [PubMed] [Google Scholar]
- Sellers J. R. Mechanism of the phosphorylation-dependent regulation of smooth muscle heavy meromyosin. J Biol Chem. 1985 Dec 15;260(29):15815–15819. [PubMed] [Google Scholar]
- Sivaramakrishnan M., Burke M. The free heavy chain of vertebrate skeletal myosin subfragment 1 shows full enzymatic activity. J Biol Chem. 1982 Jan 25;257(2):1102–1105. [PubMed] [Google Scholar]
- Somlyo A. P. Myosin isoforms in smooth muscle: how may they affect function and structure? J Muscle Res Cell Motil. 1993 Dec;14(6):557–563. doi: 10.1007/BF00141552. [DOI] [PubMed] [Google Scholar]
- Suzuki H., Onishi H., Takahashi K., Watanabe S. Structure and function of chicken gizzard myosin. J Biochem. 1978 Dec;84(6):1529–1542. doi: 10.1093/oxfordjournals.jbchem.a132278. [DOI] [PubMed] [Google Scholar]
- Sweeney H. L., Bowman B. F., Stull J. T. Myosin light chain phosphorylation in vertebrate striated muscle: regulation and function. Am J Physiol. 1993 May;264(5 Pt 1):C1085–C1095. doi: 10.1152/ajpcell.1993.264.5.C1085. [DOI] [PubMed] [Google Scholar]
- Trybus K. M. Assembly of cytoplasmic and smooth muscle myosins. Curr Opin Cell Biol. 1991 Feb;3(1):105–111. doi: 10.1016/0955-0674(91)90172-u. [DOI] [PubMed] [Google Scholar]
- Trybus K. M., Chatman T. A. Chimeric regulatory light chains as probes of smooth muscle myosin function. J Biol Chem. 1993 Feb 25;268(6):4412–4419. [PubMed] [Google Scholar]
- Trybus K. M. Filamentous smooth muscle myosin is regulated by phosphorylation. J Cell Biol. 1989 Dec;109(6 Pt 1):2887–2894. doi: 10.1083/jcb.109.6.2887. [DOI] [PMC free article] [PubMed] [Google Scholar]
- Trybus K. M., Huiatt T. W., Lowey S. A bent monomeric conformation of myosin from smooth muscle. Proc Natl Acad Sci U S A. 1982 Oct;79(20):6151–6155. doi: 10.1073/pnas.79.20.6151. [DOI] [PMC free article] [PubMed] [Google Scholar]
- Trybus K. M. Regulation of expressed truncated smooth muscle myosins. Role of the essential light chain and tail length. J Biol Chem. 1994 Aug 19;269(33):20819–20822. [PubMed] [Google Scholar]
- Trybus K. M. Role of myosin light chains. J Muscle Res Cell Motil. 1994 Dec;15(6):587–594. doi: 10.1007/BF00121066. [DOI] [PubMed] [Google Scholar]
- Trybus K. M., Waller G. S., Chatman T. A. Coupling of ATPase activity and motility in smooth muscle myosin is mediated by the regulatory light chain. J Cell Biol. 1994 Mar;124(6):963–969. doi: 10.1083/jcb.124.6.963. [DOI] [PMC free article] [PubMed] [Google Scholar]
- VanBuren P., Waller G. S., Harris D. E., Trybus K. M., Warshaw D. M., Lowey S. The essential light chain is required for full force production by skeletal muscle myosin. Proc Natl Acad Sci U S A. 1994 Dec 20;91(26):12403–12407. doi: 10.1073/pnas.91.26.12403. [DOI] [PMC free article] [PubMed] [Google Scholar]
- Wagner P. D., Giniger E. Hydrolysis of ATP and reversible binding to F-actin by myosin heavy chains free of all light chains. Nature. 1981 Aug 6;292(5823):560–562. doi: 10.1038/292560a0. [DOI] [PubMed] [Google Scholar]
- Wells C., Bagshaw C. R. Calcium regulation of molluscan myosin ATPase in the absence of actin. Nature. 1985 Feb 21;313(6004):696–697. doi: 10.1038/313696a0. [DOI] [PubMed] [Google Scholar]
- Wolff-Long V. L., Saraswat L. D., Lowey S. Cysteine mutants of light chain-2 form disulfide bonds in skeletal muscle myosin. J Biol Chem. 1993 Nov 5;268(31):23162–23167. [PubMed] [Google Scholar]
- Xie X., Harrison D. H., Schlichting I., Sweet R. M., Kalabokis V. N., Szent-Györgyi A. G., Cohen C. Structure of the regulatory domain of scallop myosin at 2.8 A resolution. Nature. 1994 Mar 24;368(6469):306–312. doi: 10.1038/368306a0. [DOI] [PubMed] [Google Scholar]