Abstract
The responses of muscle to steady and stepwise shortening are simulated with a model in which actin-myosin cross-bridges cycle through two pathways distinct for the attachment-detachment kinetics and for the proportion of energy converted into work. Small step releases and steady shortening at low velocity (high load) favor the cycle implying approximately 5 nm sliding per cross-bridge interaction and approximately 100/s detachment-reattachment process; large step releases and steady shortening at high velocity (low load) favor the cycle implying approximately 10 nm sliding per cross-bridge interaction and approximately 20/s detachment-reattachment process. The model satisfactorily predicts specific mechanical properties of frog skeletal muscle, such as the rate of regeneration of the working stroke as measured by double-step release experiments and the transition to steady state during multiple step releases (staircase shortening). The rate of energy liberation under different mechanical conditions is correctly reproduced by the model. During steady shortening, the relation of energy liberation rate versus shortening speed attains a maximum (approximately 6 times the isometric rate) for shortening velocities lower than half the maximum velocity of shortening and declines for higher velocities. In addition, the model provides a clue for explaining how, in different muscle types, the higher the isometric maintenance heat, the higher the power output during steady shortening.
Full text
PDF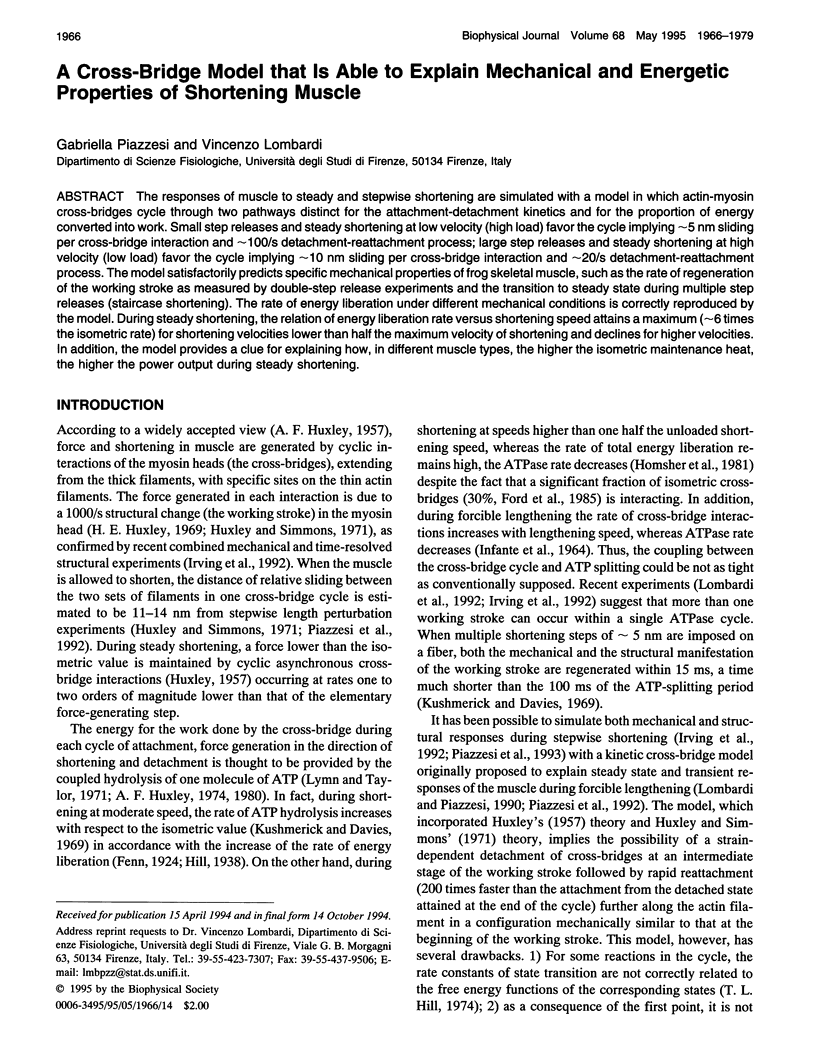
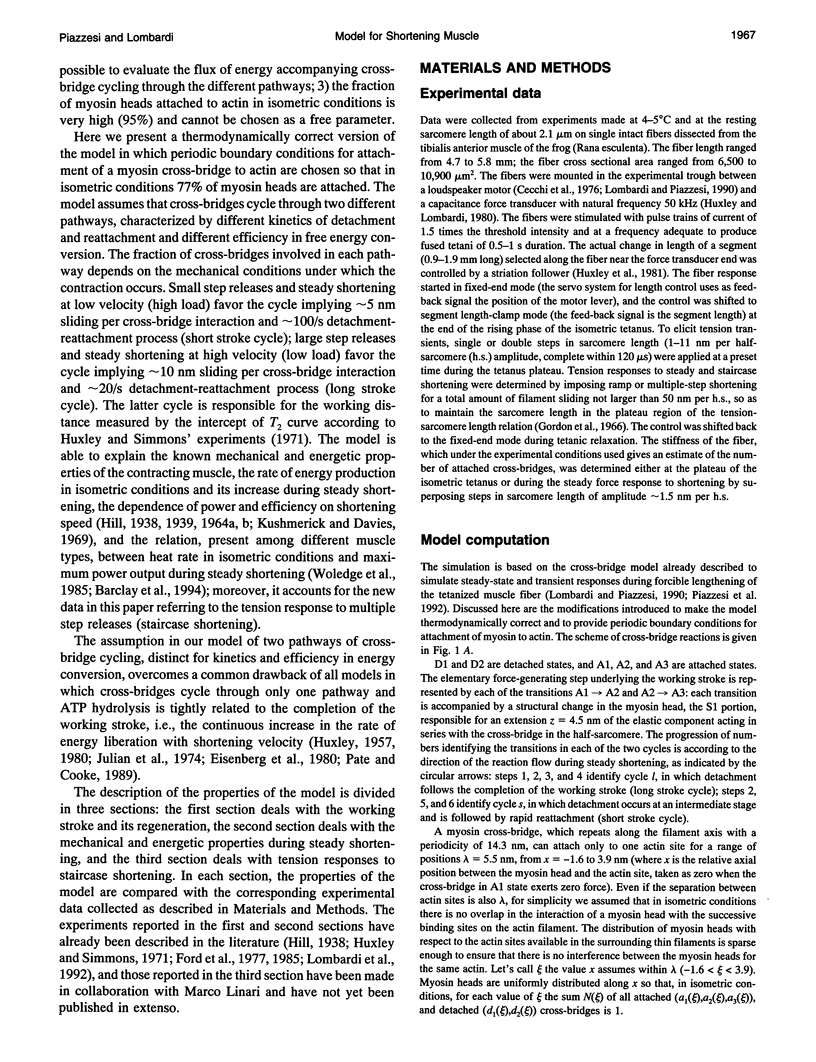
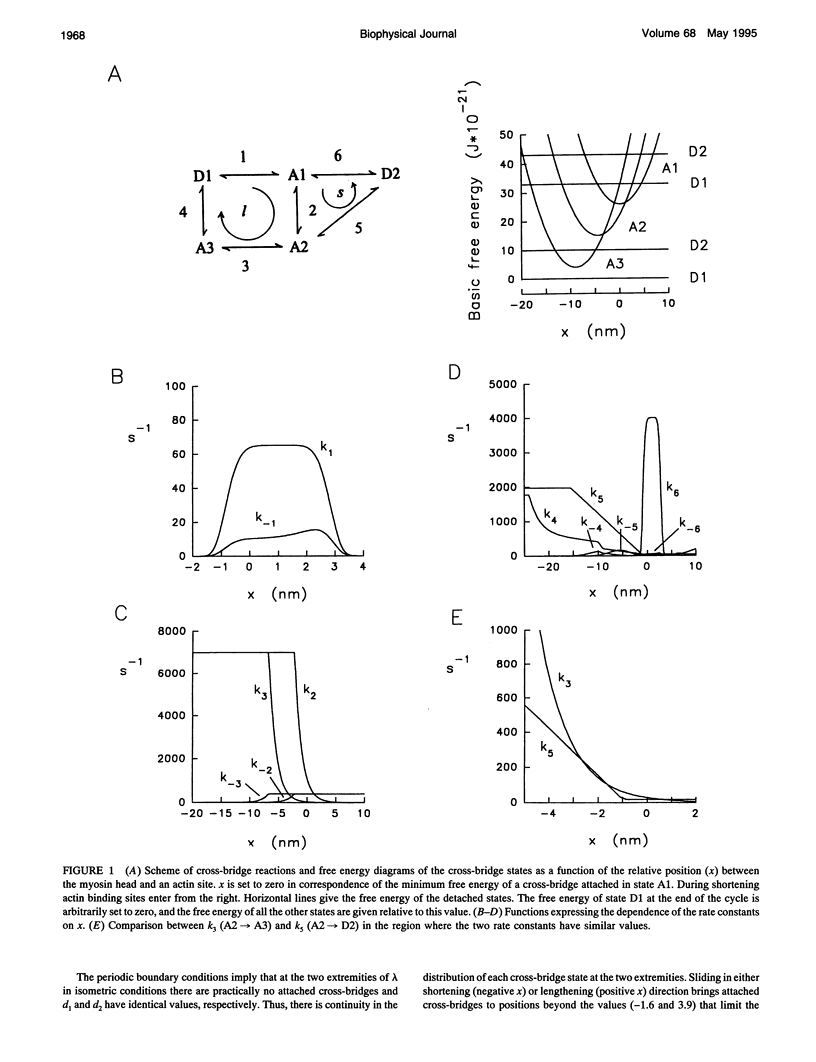
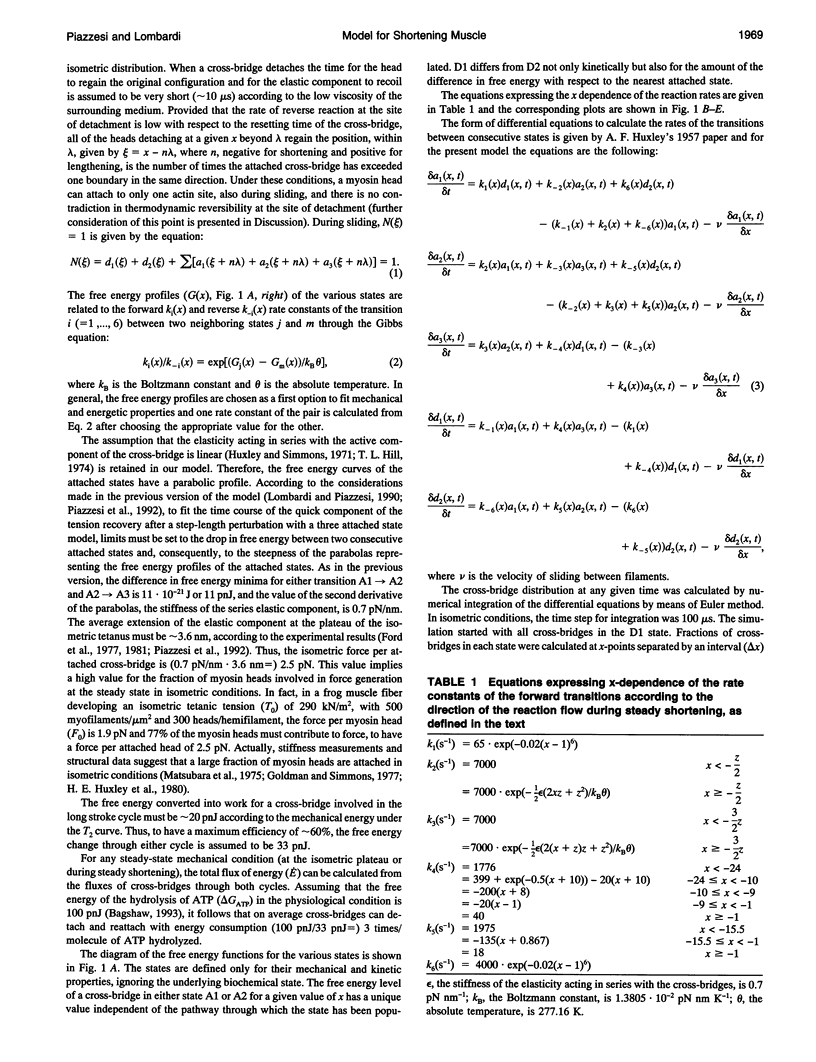
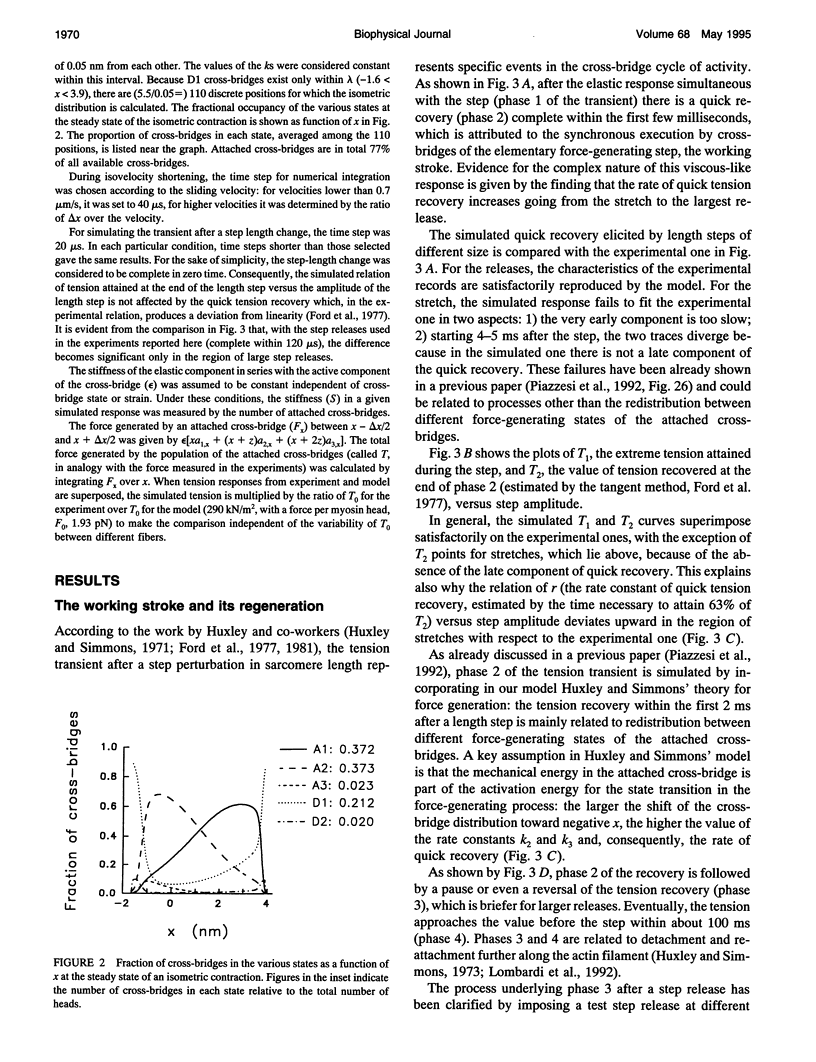
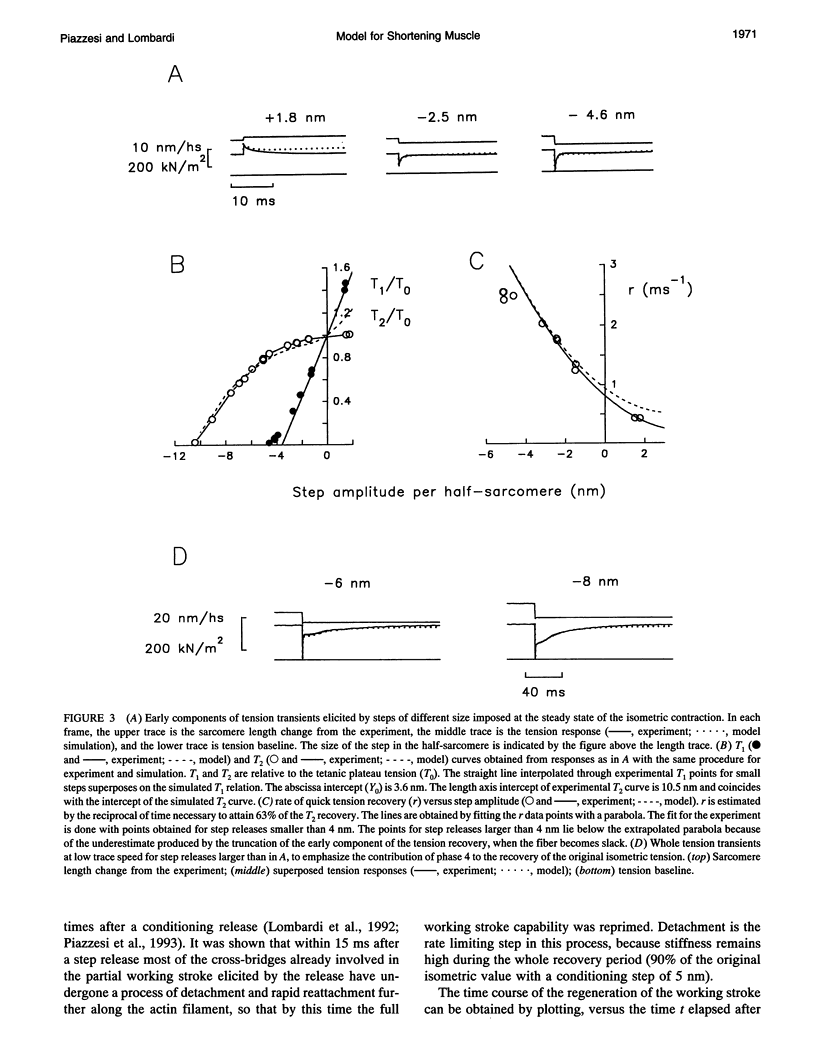
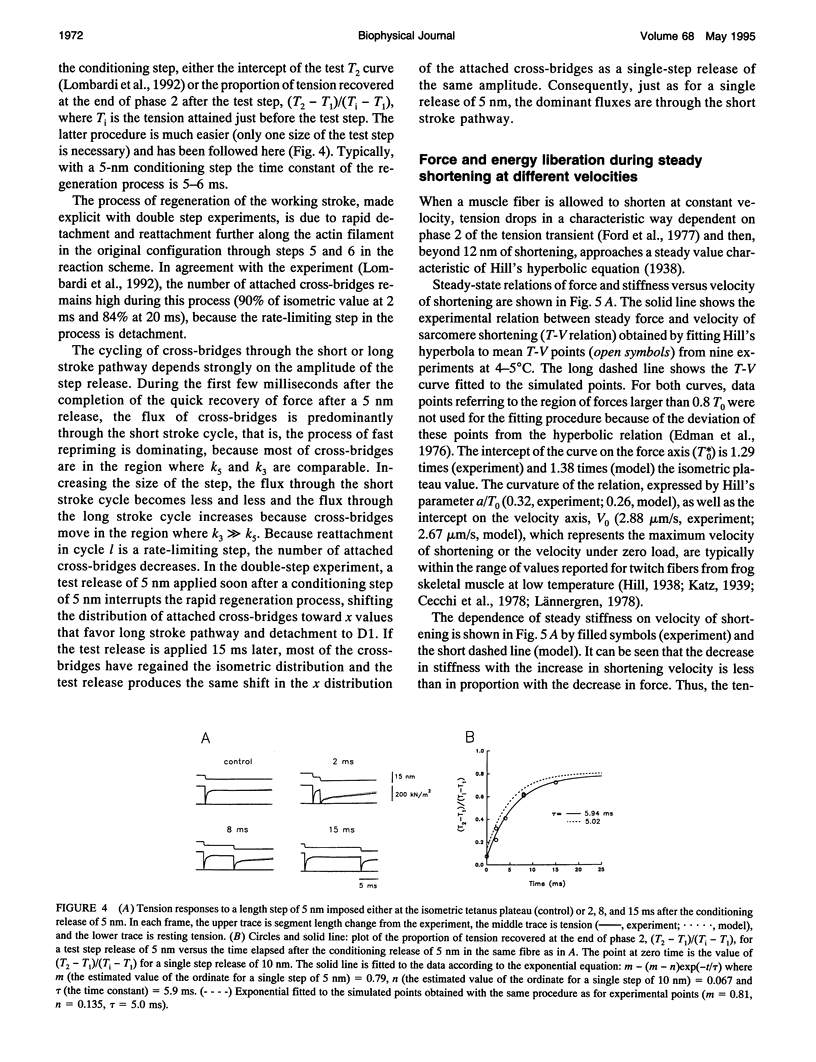
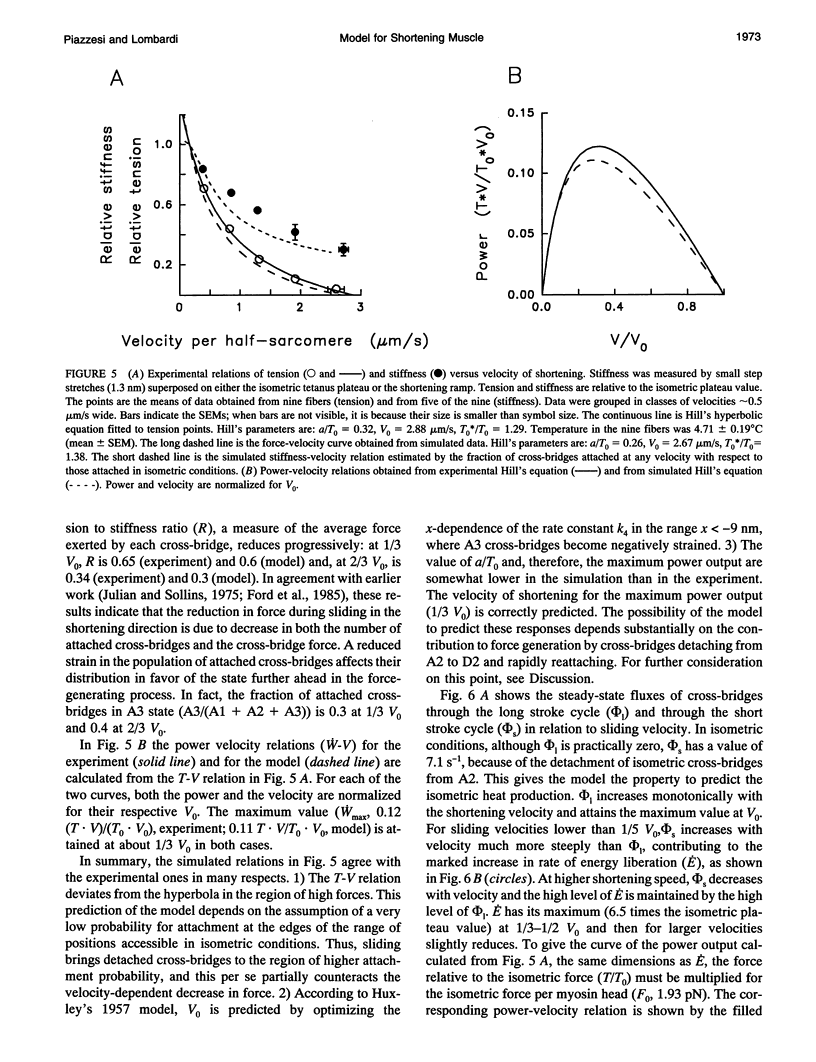
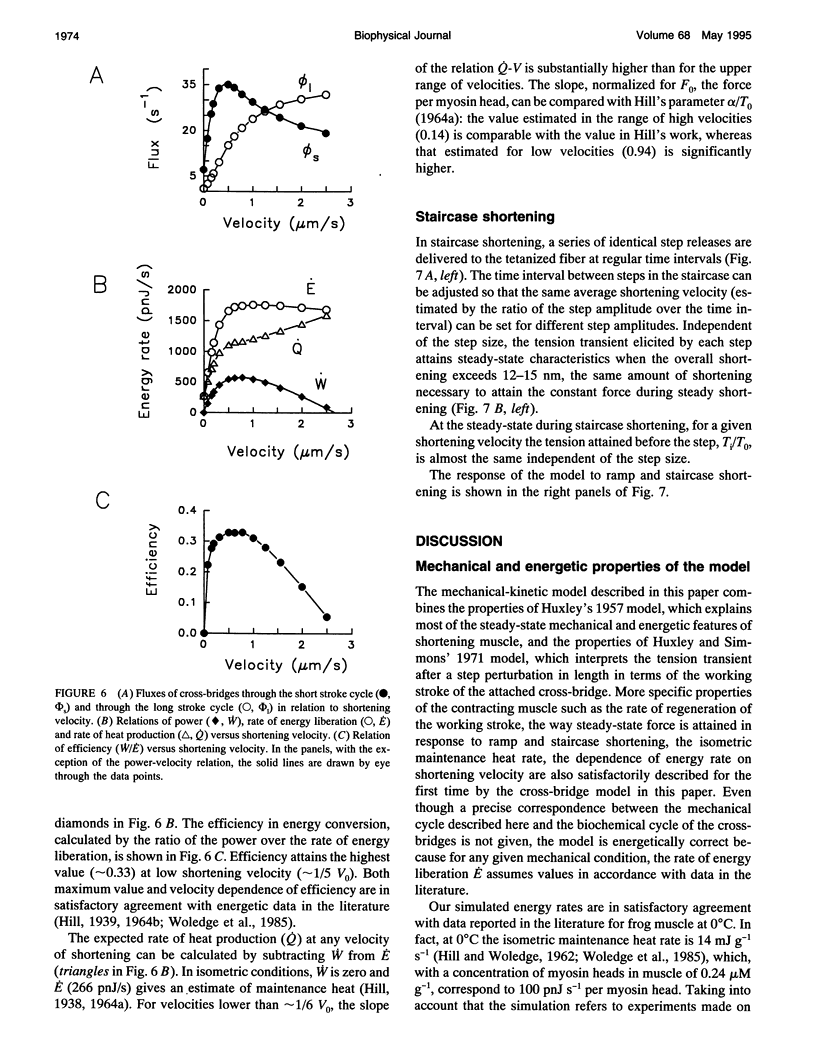
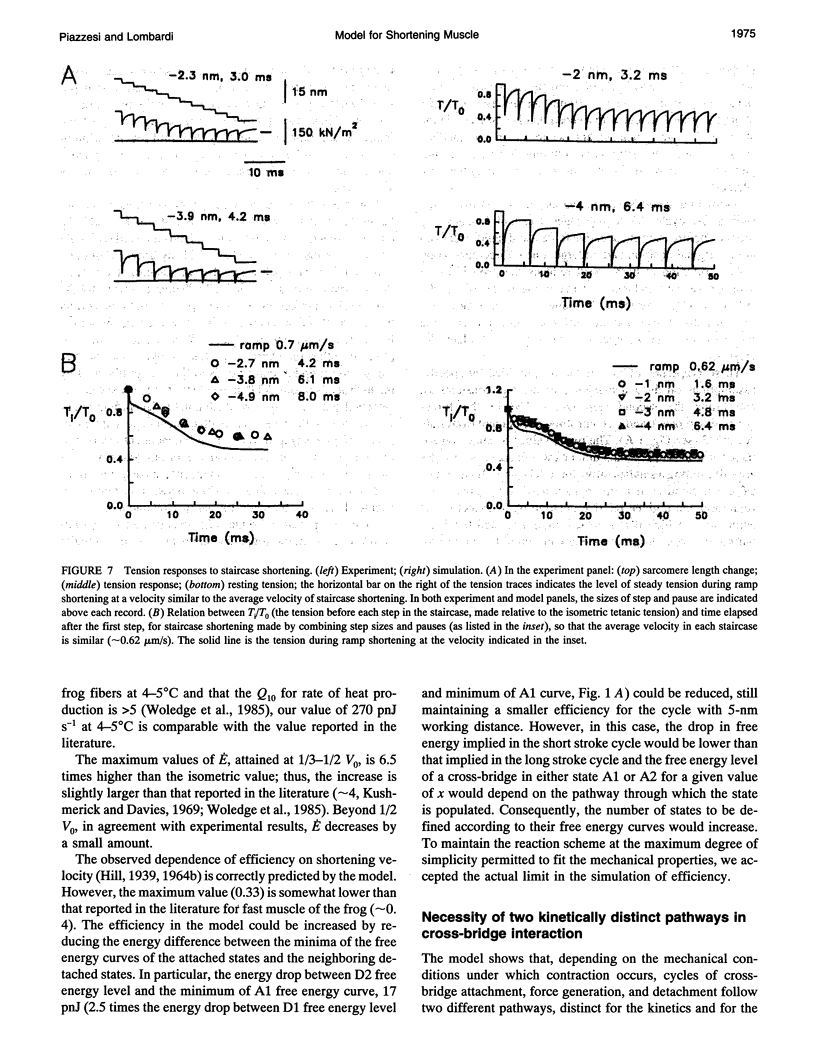
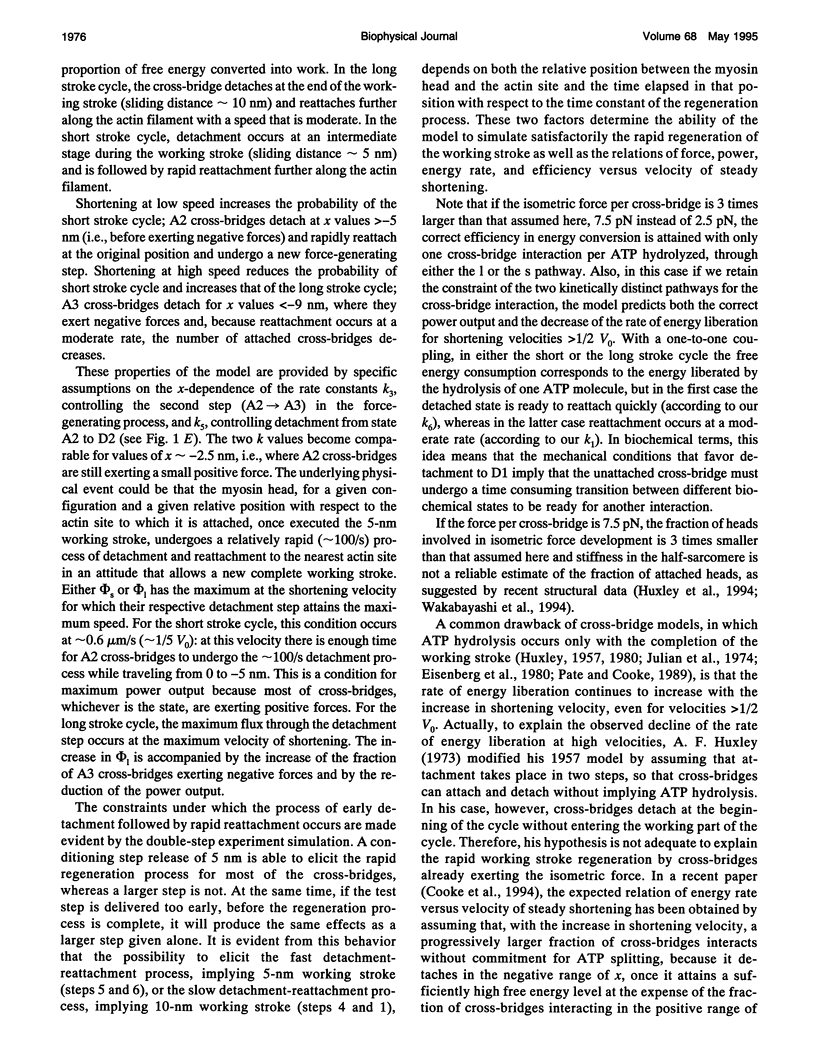
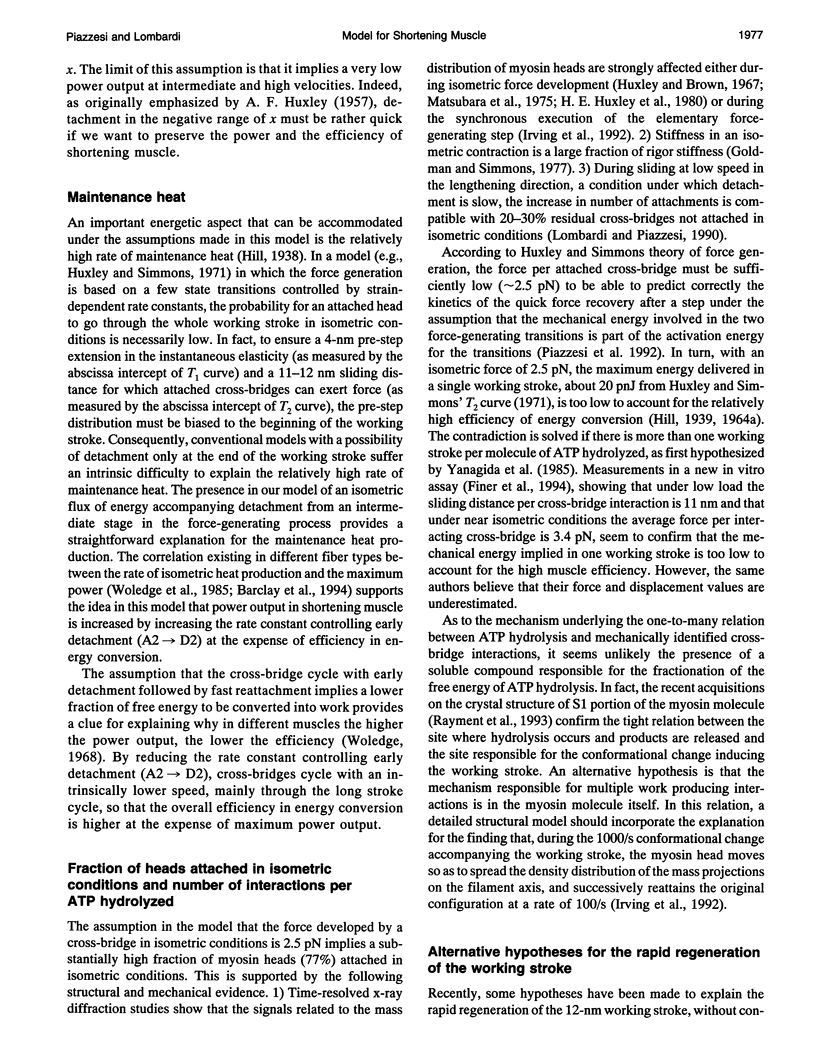
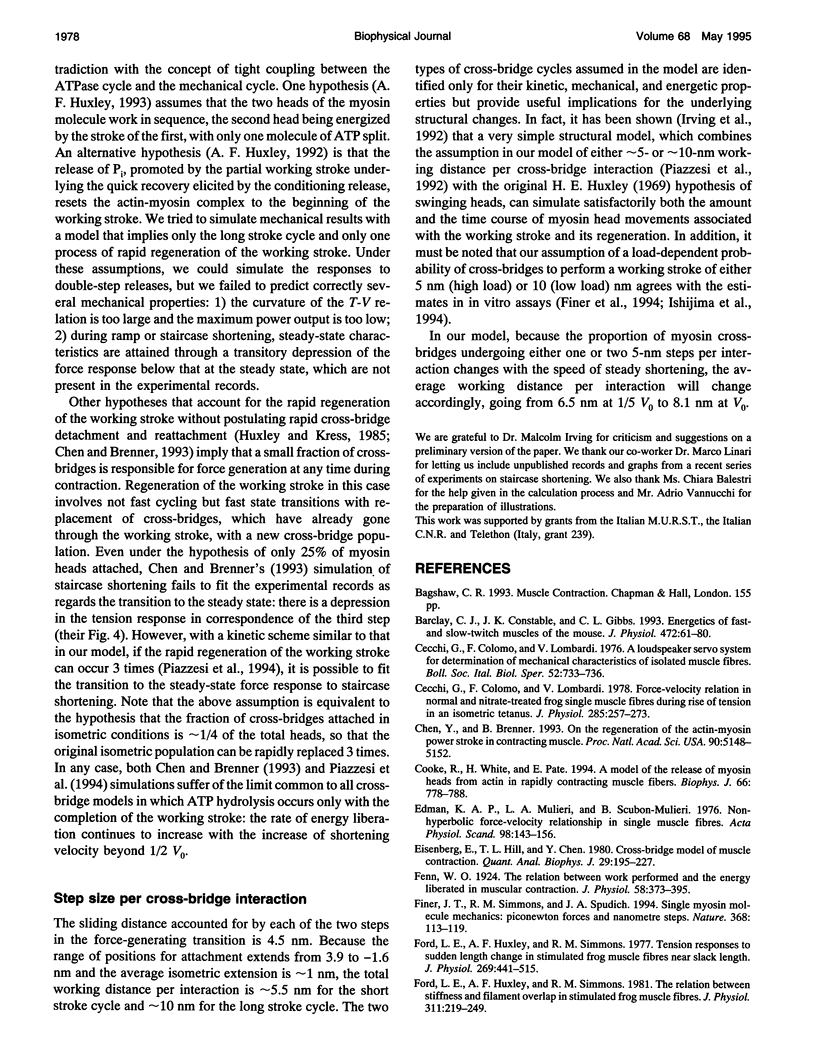
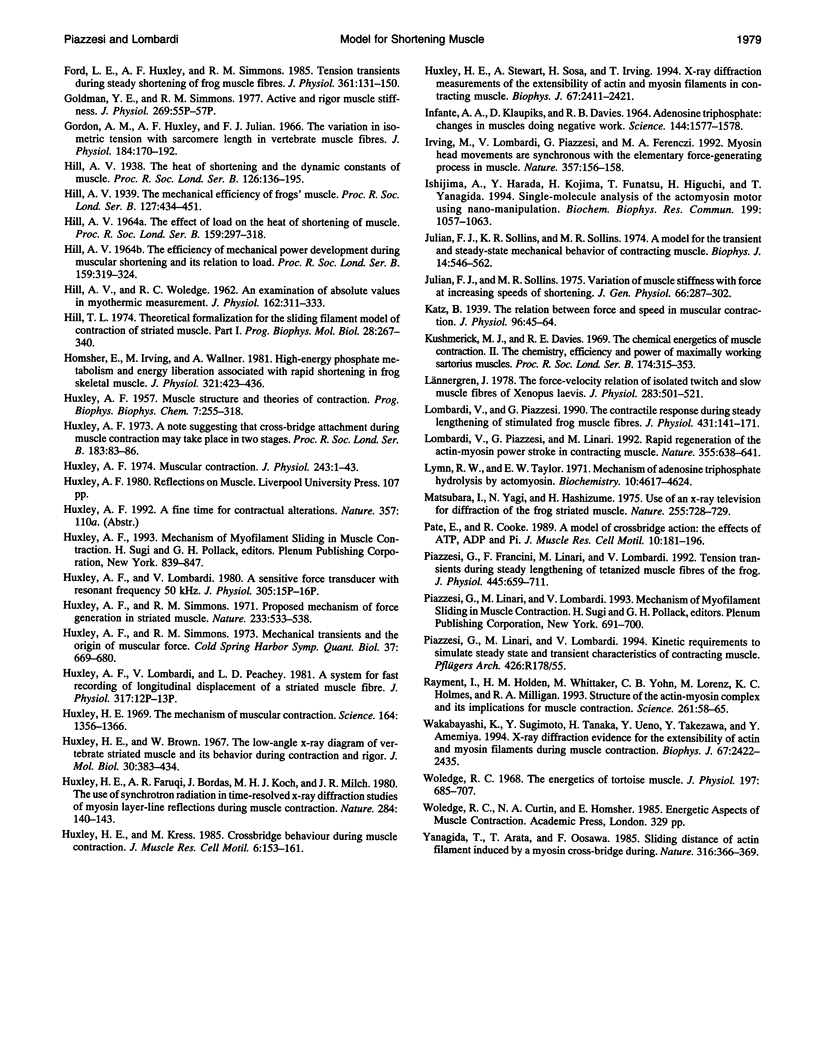
Selected References
These references are in PubMed. This may not be the complete list of references from this article.
- Barclay C. J., Constable J. K., Gibbs C. L. Energetics of fast- and slow-twitch muscles of the mouse. J Physiol. 1993 Dec;472:61–80. doi: 10.1113/jphysiol.1993.sp019937. [DOI] [PMC free article] [PubMed] [Google Scholar]
- Cecchi G., Colomo F., Lombardi V. A loudspeaker servo system for determination of mechanical characteristics of isolated muscle fibres. Boll Soc Ital Biol Sper. 1976 May 30;52(10):733–736. [PubMed] [Google Scholar]
- Cecchi G., Colomo F., Lombardi V. Force-velocity relation in normal and nitrate-treated frog single muscle fibres during rise of tension in an isometric tetanus. J Physiol. 1978 Dec;285:257–273. doi: 10.1113/jphysiol.1978.sp012570. [DOI] [PMC free article] [PubMed] [Google Scholar]
- Chen Y. D., Brenner B. On the regeneration of the actin-myosin power stroke in contracting muscle. Proc Natl Acad Sci U S A. 1993 Jun 1;90(11):5148–5152. doi: 10.1073/pnas.90.11.5148. [DOI] [PMC free article] [PubMed] [Google Scholar]
- Cooke R., White H., Pate E. A model of the release of myosin heads from actin in rapidly contracting muscle fibers. Biophys J. 1994 Mar;66(3 Pt 1):778–788. doi: 10.1016/s0006-3495(94)80854-9. [DOI] [PMC free article] [PubMed] [Google Scholar]
- Edman K. A., Mulieri L. A., Scubon-Mulieri B. Non-hyperbolic force-velocity relationship in single muscle fibres. Acta Physiol Scand. 1976 Oct;98(2):143–156. doi: 10.1111/j.1748-1716.1976.tb00234.x. [DOI] [PubMed] [Google Scholar]
- Eisenberg E., Hill T. L., Chen Y. Cross-bridge model of muscle contraction. Quantitative analysis. Biophys J. 1980 Feb;29(2):195–227. doi: 10.1016/S0006-3495(80)85126-5. [DOI] [PMC free article] [PubMed] [Google Scholar]
- Fenn W. O. The relation between the work performed and the energy liberated in muscular contraction. J Physiol. 1924 May 23;58(6):373–395. doi: 10.1113/jphysiol.1924.sp002141. [DOI] [PMC free article] [PubMed] [Google Scholar]
- Finer J. T., Simmons R. M., Spudich J. A. Single myosin molecule mechanics: piconewton forces and nanometre steps. Nature. 1994 Mar 10;368(6467):113–119. doi: 10.1038/368113a0. [DOI] [PubMed] [Google Scholar]
- Ford L. E., Huxley A. F., Simmons R. M. Tension responses to sudden length change in stimulated frog muscle fibres near slack length. J Physiol. 1977 Jul;269(2):441–515. doi: 10.1113/jphysiol.1977.sp011911. [DOI] [PMC free article] [PubMed] [Google Scholar]
- Ford L. E., Huxley A. F., Simmons R. M. Tension transients during steady shortening of frog muscle fibres. J Physiol. 1985 Apr;361:131–150. doi: 10.1113/jphysiol.1985.sp015637. [DOI] [PMC free article] [PubMed] [Google Scholar]
- Ford L. E., Huxley A. F., Simmons R. M. The relation between stiffness and filament overlap in stimulated frog muscle fibres. J Physiol. 1981 Feb;311:219–249. doi: 10.1113/jphysiol.1981.sp013582. [DOI] [PMC free article] [PubMed] [Google Scholar]
- Goldman Y. E., Simmons R. M. Active and rigor muscle stiffness [proceedings]. J Physiol. 1977 Jul;269(1):55P–57P. [PubMed] [Google Scholar]
- Gordon A. M., Huxley A. F., Julian F. J. The variation in isometric tension with sarcomere length in vertebrate muscle fibres. J Physiol. 1966 May;184(1):170–192. doi: 10.1113/jphysiol.1966.sp007909. [DOI] [PMC free article] [PubMed] [Google Scholar]
- HILL A. V. THE EFFECT OF LOAD ON THE HEAT OF SHORTENING OF MUSCLE. Proc R Soc Lond B Biol Sci. 1964 Jan 14;159:297–318. doi: 10.1098/rspb.1964.0004. [DOI] [PubMed] [Google Scholar]
- HILL A. V. THE EFFICIENCY OF MECHANICAL POWER DEVELOPMENT DURING MUSCULAR SHORTENING AND ITS RELATION TO LOAD. Proc R Soc Lond B Biol Sci. 1964 Jan 14;159:319–324. doi: 10.1098/rspb.1964.0005. [DOI] [PubMed] [Google Scholar]
- HILL A. V., WOLEDGE R. C. An examination of absolute values in myothermic measurements. J Physiol. 1962 Jul;162:311–333. doi: 10.1113/jphysiol.1962.sp006935. [DOI] [PMC free article] [PubMed] [Google Scholar]
- HUXLEY A. F. Muscle structure and theories of contraction. Prog Biophys Biophys Chem. 1957;7:255–318. [PubMed] [Google Scholar]
- Hill T. L. Theoretical formalism for the sliding filament model of contraction of striated muscle. Part I. Prog Biophys Mol Biol. 1974;28:267–340. doi: 10.1016/0079-6107(74)90020-0. [DOI] [PubMed] [Google Scholar]
- Homsher E., Irving M., Wallner A. High-energy phosphate metabolism and energy liberation associated with rapid shortening in frog skeletal muscle. J Physiol. 1981 Dec;321:423–436. doi: 10.1113/jphysiol.1981.sp013994. [DOI] [PMC free article] [PubMed] [Google Scholar]
- Huxley A. F. A note suggesting that the cross-bridge attachment during muscle contraction may take place in two stages. Proc R Soc Lond B Biol Sci. 1973 Feb 27;183(1070):83–86. doi: 10.1098/rspb.1973.0006. [DOI] [PubMed] [Google Scholar]
- Huxley A. F. Muscular contraction. J Physiol. 1974 Nov;243(1):1–43. [PMC free article] [PubMed] [Google Scholar]
- Huxley A. F., Simmons R. M. Proposed mechanism of force generation in striated muscle. Nature. 1971 Oct 22;233(5321):533–538. doi: 10.1038/233533a0. [DOI] [PubMed] [Google Scholar]
- Huxley H. E., Brown W. The low-angle x-ray diagram of vertebrate striated muscle and its behaviour during contraction and rigor. J Mol Biol. 1967 Dec 14;30(2):383–434. doi: 10.1016/s0022-2836(67)80046-9. [DOI] [PubMed] [Google Scholar]
- Huxley H. E., Faruqi A. R., Bordas J., Koch M. H., Milch J. R. The use of synchrotron radiation in time-resolved X-ray diffraction studies of myosin layer-line reflections during muscle contraction. Nature. 1980 Mar 13;284(5752):140–143. doi: 10.1038/284140a0. [DOI] [PubMed] [Google Scholar]
- Huxley H. E., Kress M. Crossbridge behaviour during muscle contraction. J Muscle Res Cell Motil. 1985 Apr;6(2):153–161. doi: 10.1007/BF00713057. [DOI] [PubMed] [Google Scholar]
- Huxley H. E., Stewart A., Sosa H., Irving T. X-ray diffraction measurements of the extensibility of actin and myosin filaments in contracting muscle. Biophys J. 1994 Dec;67(6):2411–2421. doi: 10.1016/S0006-3495(94)80728-3. [DOI] [PMC free article] [PubMed] [Google Scholar]
- Huxley H. E. The mechanism of muscular contraction. Science. 1969 Jun 20;164(3886):1356–1365. doi: 10.1126/science.164.3886.1356. [DOI] [PubMed] [Google Scholar]
- INFANTE A. A., KLAUPIKS D., DAVIES R. E. ADENOSINE TRIPHOSPHATE: CHANGES IN MUSCLES DOING NEGATIVE WORK. Science. 1964 Jun 26;144(3626):1577–1578. doi: 10.1126/science.144.3626.1577. [DOI] [PubMed] [Google Scholar]
- Irving M., Lombardi V., Piazzesi G., Ferenczi M. A. Myosin head movements are synchronous with the elementary force-generating process in muscle. Nature. 1992 May 14;357(6374):156–158. doi: 10.1038/357156a0. [DOI] [PubMed] [Google Scholar]
- Ishijima A., Harada Y., Kojima H., Funatsu T., Higuchi H., Yanagida T. Single-molecule analysis of the actomyosin motor using nano-manipulation. Biochem Biophys Res Commun. 1994 Mar 15;199(2):1057–1063. doi: 10.1006/bbrc.1994.1336. [DOI] [PubMed] [Google Scholar]
- Julian F. J., Sollins K. R., Sollins M. R. A model for the transient and steady-state mechanical behavior of contracting muscle. Biophys J. 1974 Jul;14(7):546–562. doi: 10.1016/S0006-3495(74)85934-5. [DOI] [PMC free article] [PubMed] [Google Scholar]
- Katz B. The relation between force and speed in muscular contraction. J Physiol. 1939 Jun 14;96(1):45–64. doi: 10.1113/jphysiol.1939.sp003756. [DOI] [PMC free article] [PubMed] [Google Scholar]
- Kushmerick M. J., Davies R. E. The chemical energetics of muscle contraction. II. The chemistry, efficiency and power of maximally working sartorius muscles. Appendix. Free energy and enthalpy of atp hydrolysis in the sarcoplasm. Proc R Soc Lond B Biol Sci. 1969 Dec 23;174(1036):315–353. doi: 10.1098/rspb.1969.0096. [DOI] [PubMed] [Google Scholar]
- Lombardi V., Piazzesi G., Linari M. Rapid regeneration of the actin-myosin power stroke in contracting muscle. Nature. 1992 Feb 13;355(6361):638–641. doi: 10.1038/355638a0. [DOI] [PubMed] [Google Scholar]
- Lombardi V., Piazzesi G. The contractile response during steady lengthening of stimulated frog muscle fibres. J Physiol. 1990 Dec;431:141–171. doi: 10.1113/jphysiol.1990.sp018324. [DOI] [PMC free article] [PubMed] [Google Scholar]
- Lymn R. W., Taylor E. W. Mechanism of adenosine triphosphate hydrolysis by actomyosin. Biochemistry. 1971 Dec 7;10(25):4617–4624. doi: 10.1021/bi00801a004. [DOI] [PubMed] [Google Scholar]
- Lännergren J. The force-velocity relation of isolated twitch and slow muscle fibres of Xenopus laevis. J Physiol. 1978 Oct;283:501–521. doi: 10.1113/jphysiol.1978.sp012516. [DOI] [PMC free article] [PubMed] [Google Scholar]
- Matsubara I., Yagi N., Hashizume H. Use of an X-ray television for diffraction of the frog striated muscle. Nature. 1975 Jun 26;255(5511):728–729. doi: 10.1038/255728a0. [DOI] [PubMed] [Google Scholar]
- Pate E., Cooke R. A model of crossbridge action: the effects of ATP, ADP and Pi. J Muscle Res Cell Motil. 1989 Jun;10(3):181–196. doi: 10.1007/BF01739809. [DOI] [PubMed] [Google Scholar]
- Piazzesi G., Francini F., Linari M., Lombardi V. Tension transients during steady lengthening of tetanized muscle fibres of the frog. J Physiol. 1992 Jan;445:659–711. doi: 10.1113/jphysiol.1992.sp018945. [DOI] [PMC free article] [PubMed] [Google Scholar]
- Rayment I., Holden H. M., Whittaker M., Yohn C. B., Lorenz M., Holmes K. C., Milligan R. A. Structure of the actin-myosin complex and its implications for muscle contraction. Science. 1993 Jul 2;261(5117):58–65. doi: 10.1126/science.8316858. [DOI] [PubMed] [Google Scholar]
- Wakabayashi K., Sugimoto Y., Tanaka H., Ueno Y., Takezawa Y., Amemiya Y. X-ray diffraction evidence for the extensibility of actin and myosin filaments during muscle contraction. Biophys J. 1994 Dec;67(6):2422–2435. doi: 10.1016/S0006-3495(94)80729-5. [DOI] [PMC free article] [PubMed] [Google Scholar]
- Woledge R. C. The energetics of tortoise muscle. J Physiol. 1968 Aug;197(3):685–707. doi: 10.1113/jphysiol.1968.sp008582. [DOI] [PMC free article] [PubMed] [Google Scholar]
- Yanagida T., Arata T., Oosawa F. Sliding distance of actin filament induced by a myosin crossbridge during one ATP hydrolysis cycle. Nature. 1985 Jul 25;316(6026):366–369. doi: 10.1038/316366a0. [DOI] [PubMed] [Google Scholar]