Abstract
1. Slices of guinea-pig olfactory cortex were maintained in vitro. Electrical properties of neurones in the prepyriform region were studied using single high resistance glass micro-electrodes filled with potassium acetate, connected to a resistance-compensating circuit to allow passage of current through the electrode. 2. Neurones showed a high, stable resting membrane potential (75.4 +/- 2.7 mV, mean +/- S.D.; n = 47). Input resistance measured with small depolarizing currents varied over a range of 9-280 Momega. The time constant for decay of depolarizing potentials was 19.4 +/- 7.5 msec (mean +/- S.D.).. 4. Depolarization produced repetitive action potentials (maximum frequency of 85 Hz) having peak amplitudes of +16 to +47 mV. The action potential was followed by a depolarizing after potential of about 20 mV positive to the membrane potential. 5. In these and other respects, the prepyriform neurones appear to behave like most other neurones in the mammalian brain, after allowing for the more stable recording conditions in this preparation.
Full text
PDF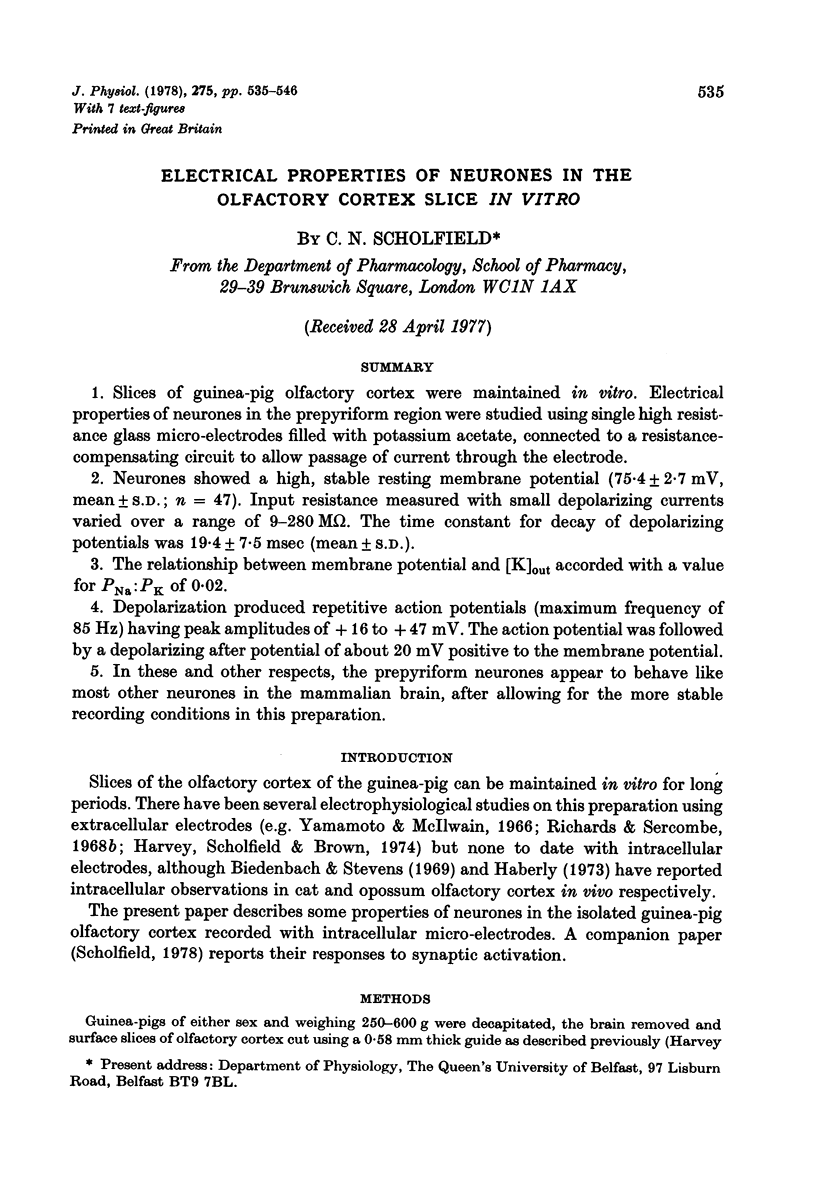
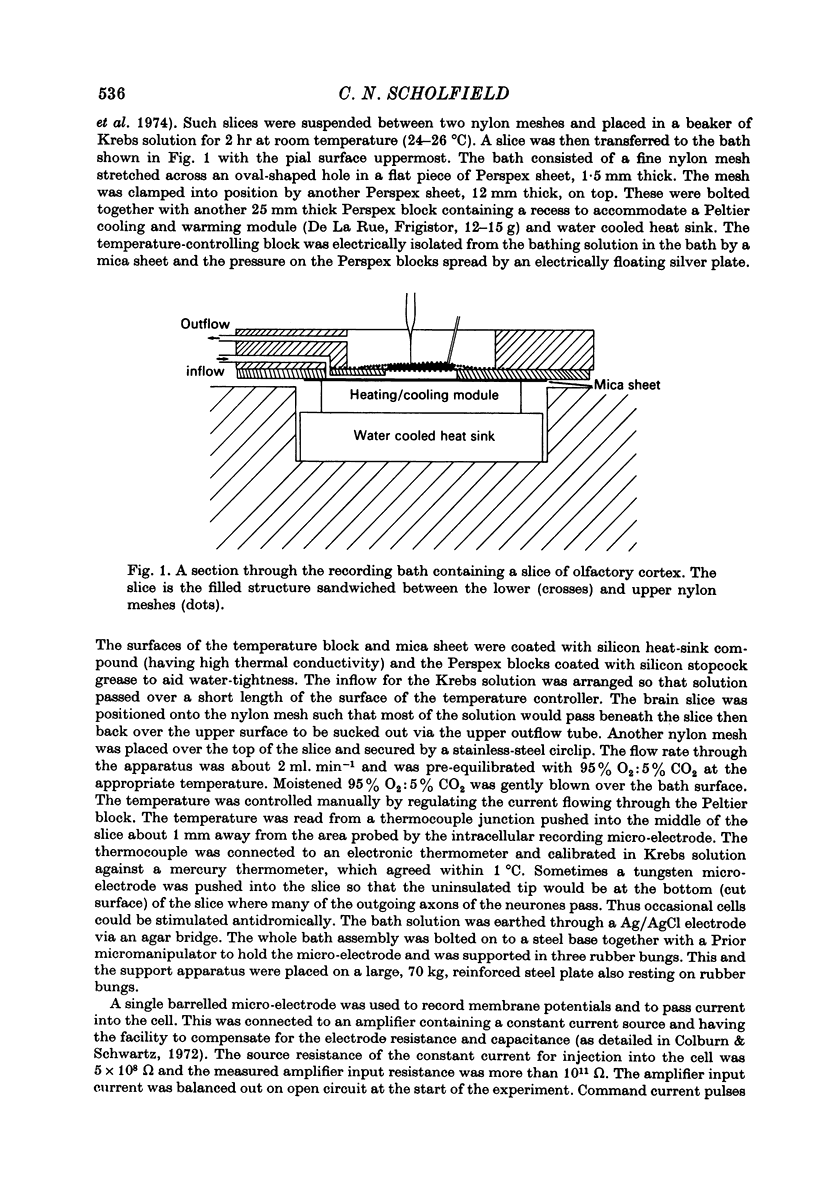
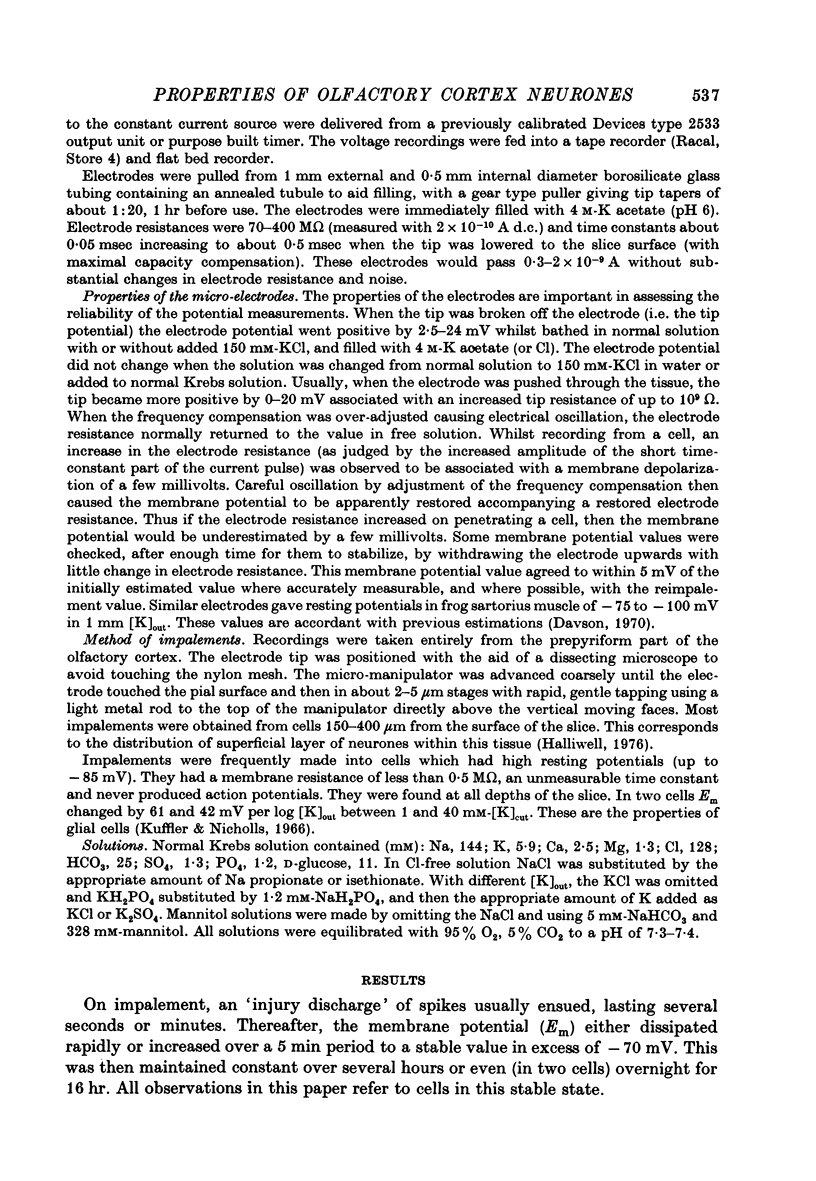
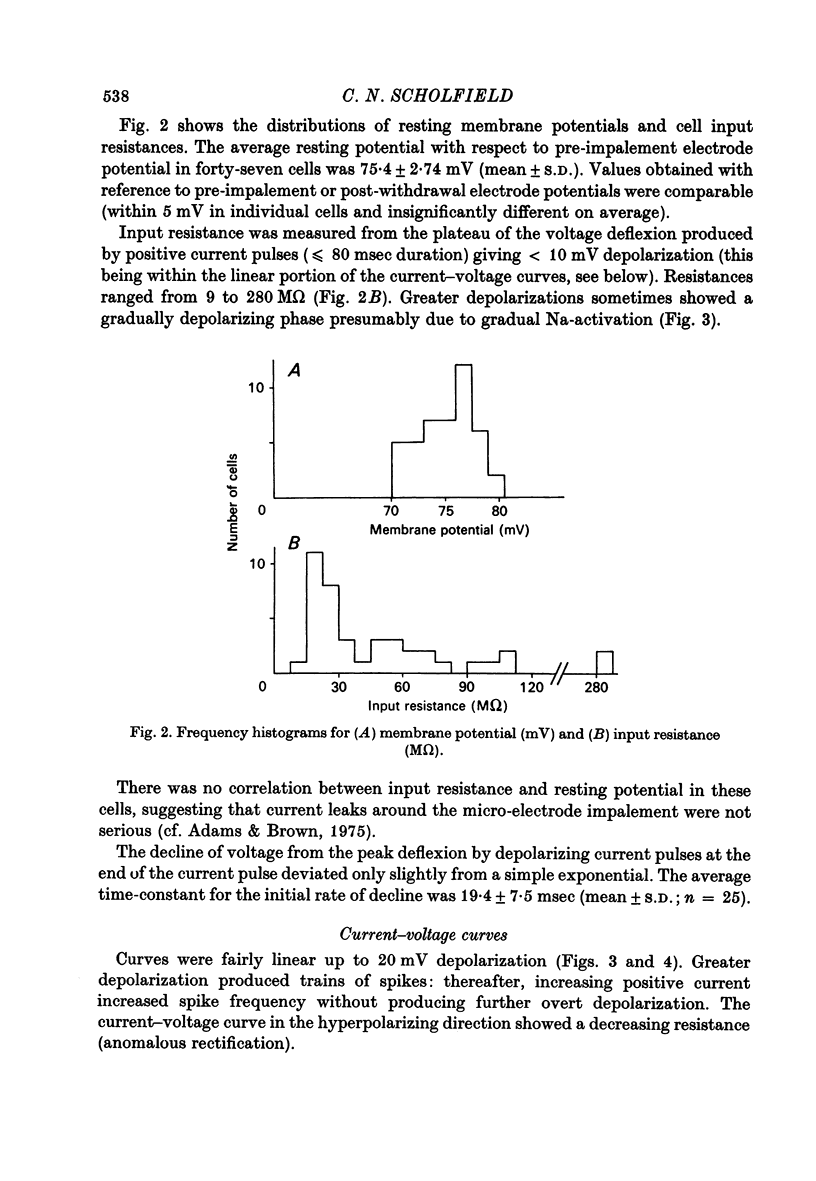
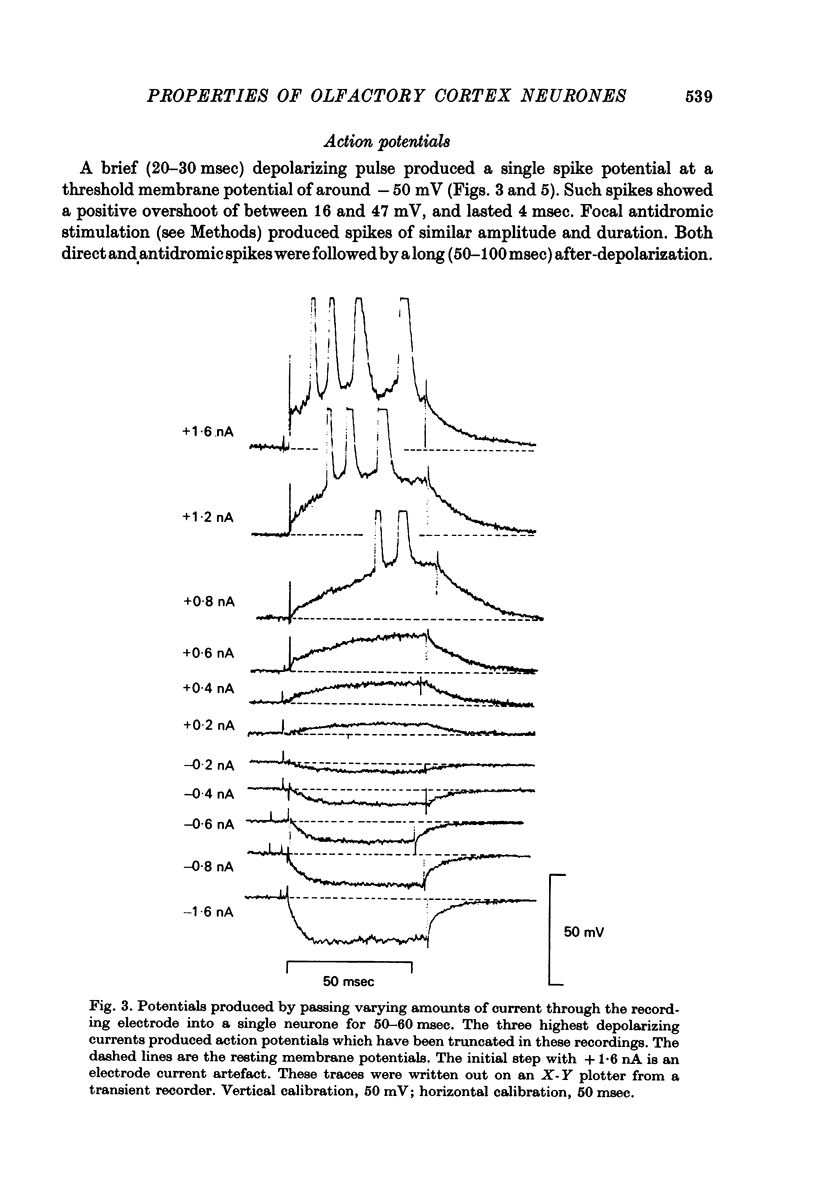
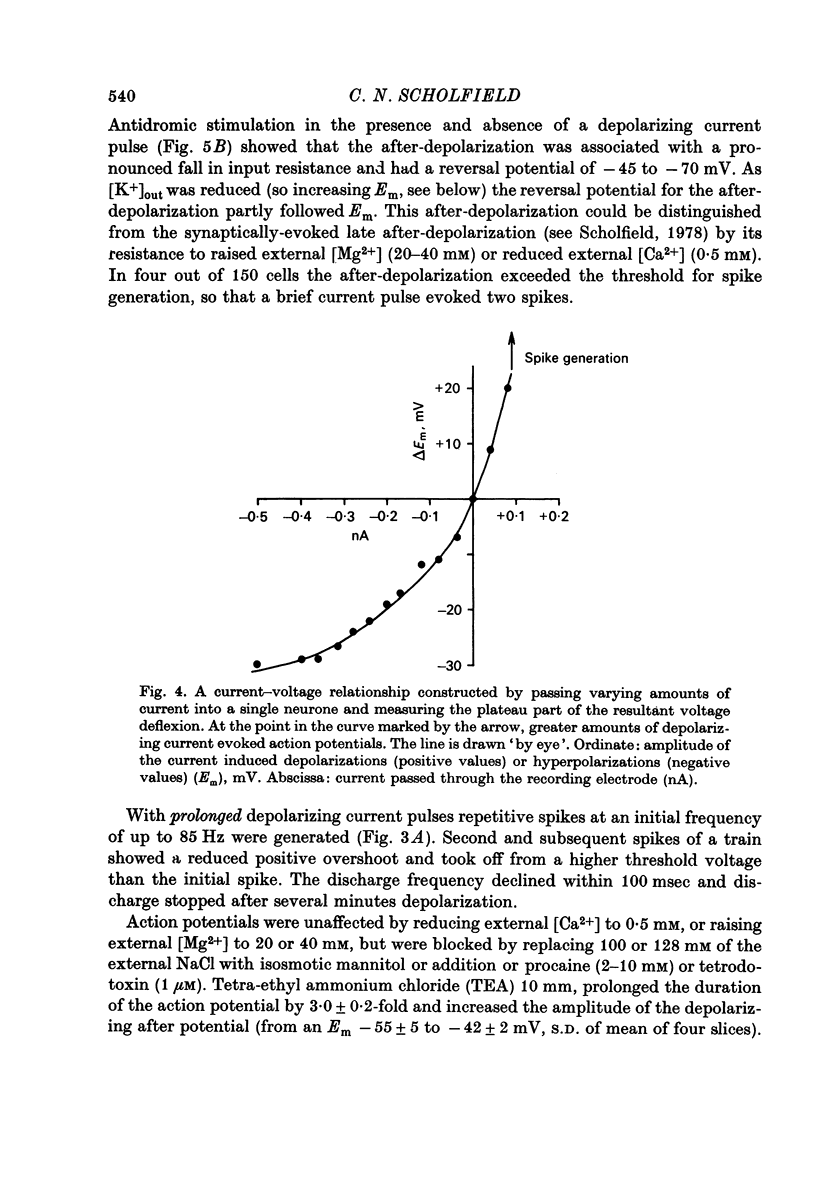
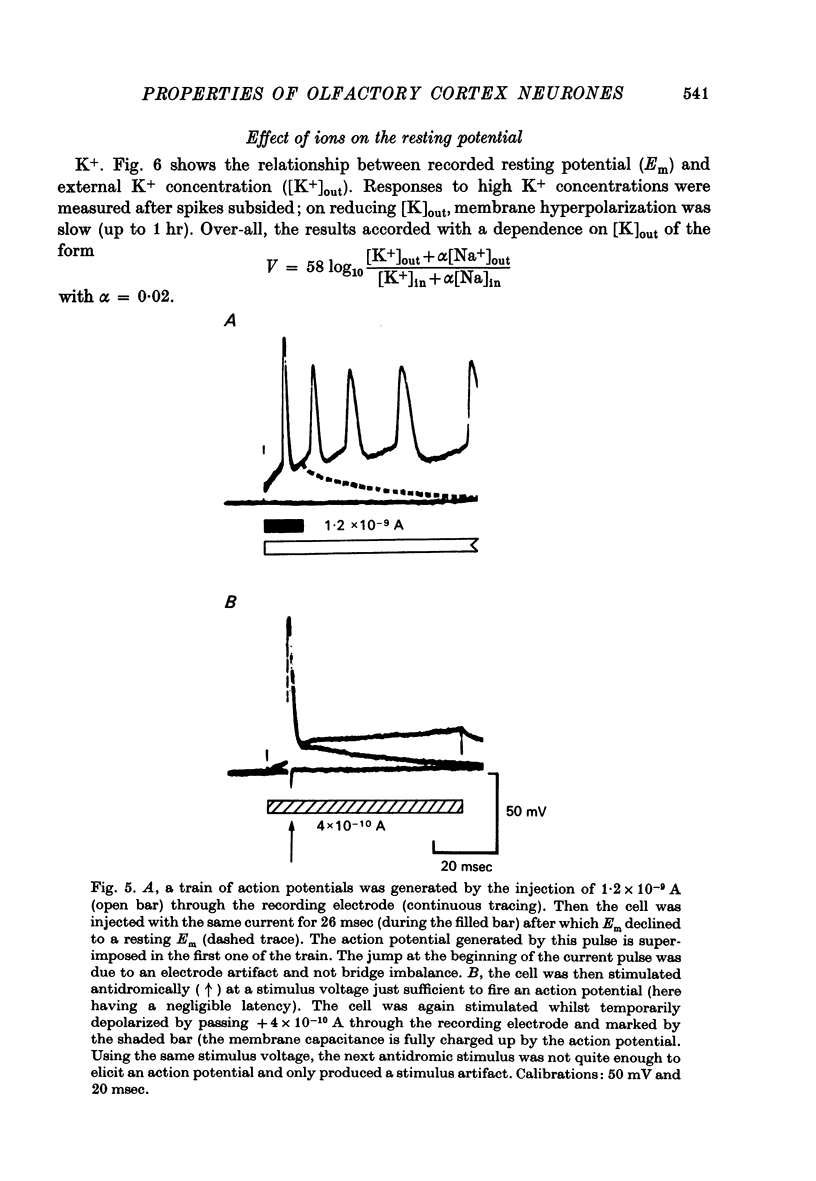
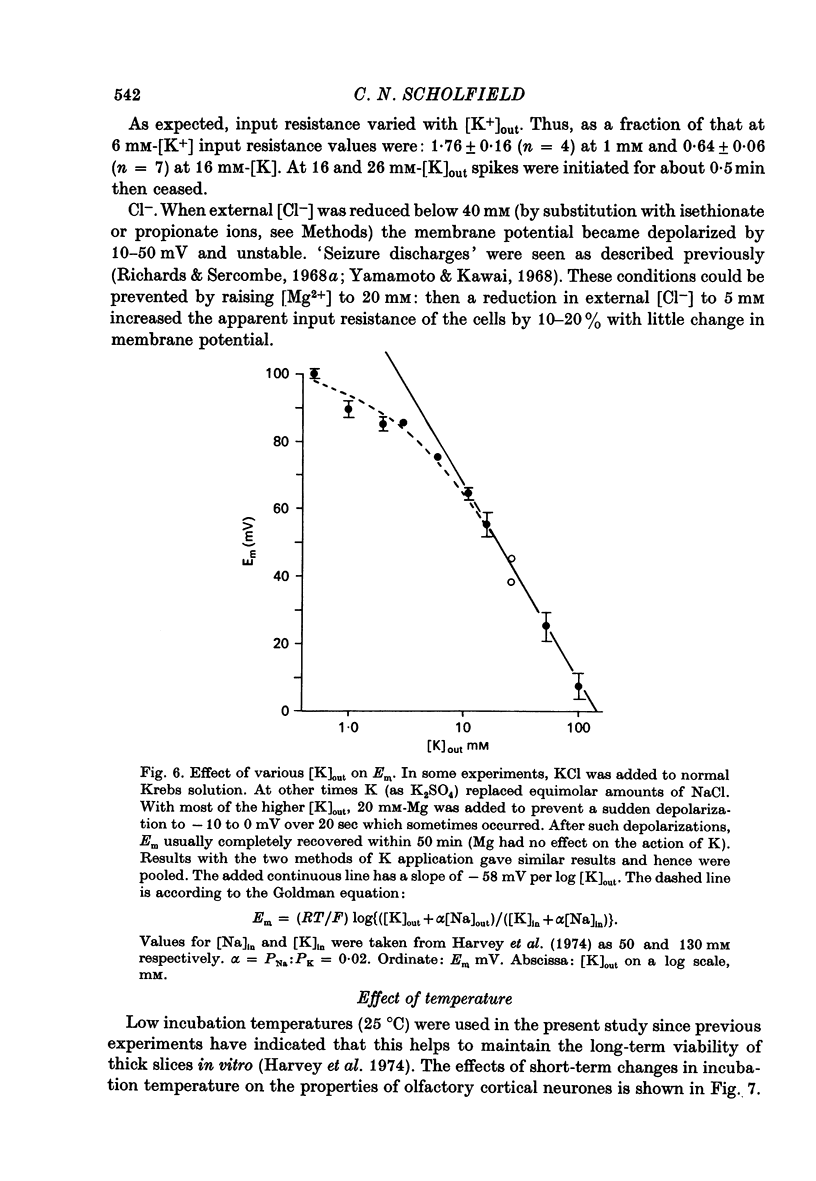
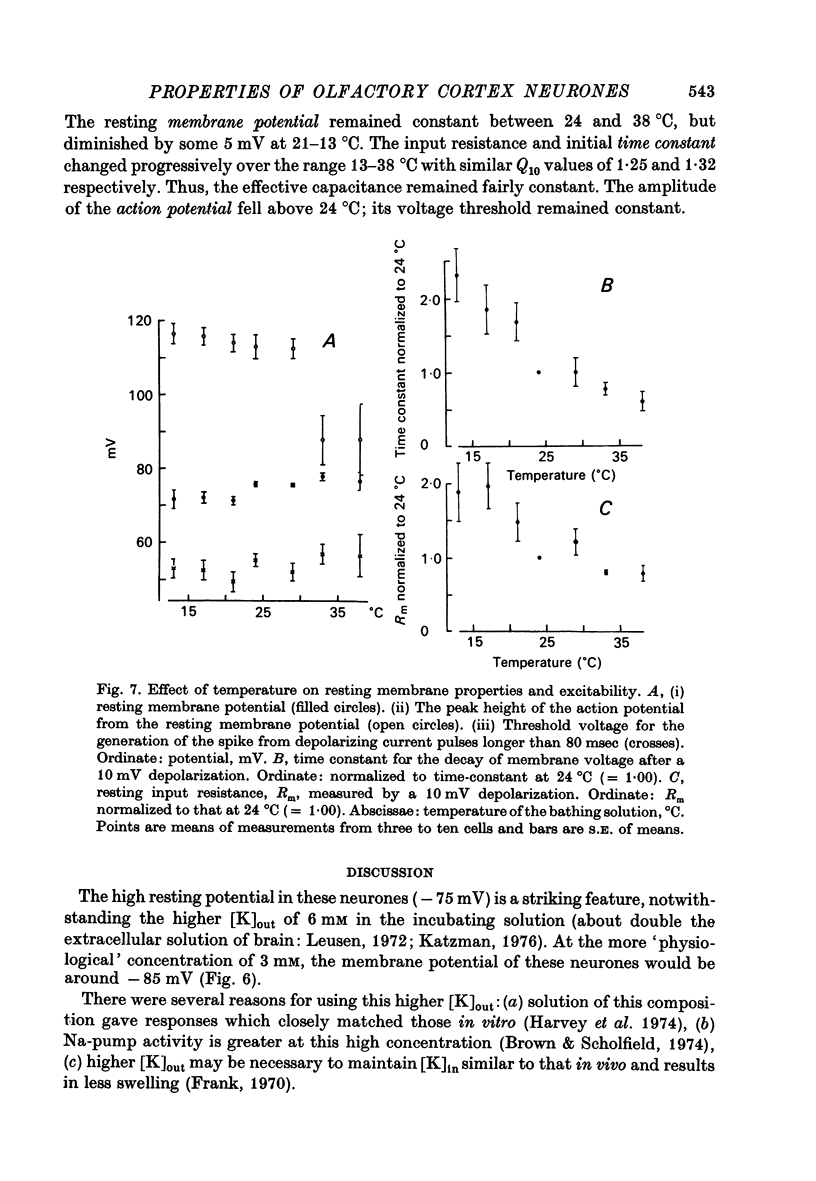
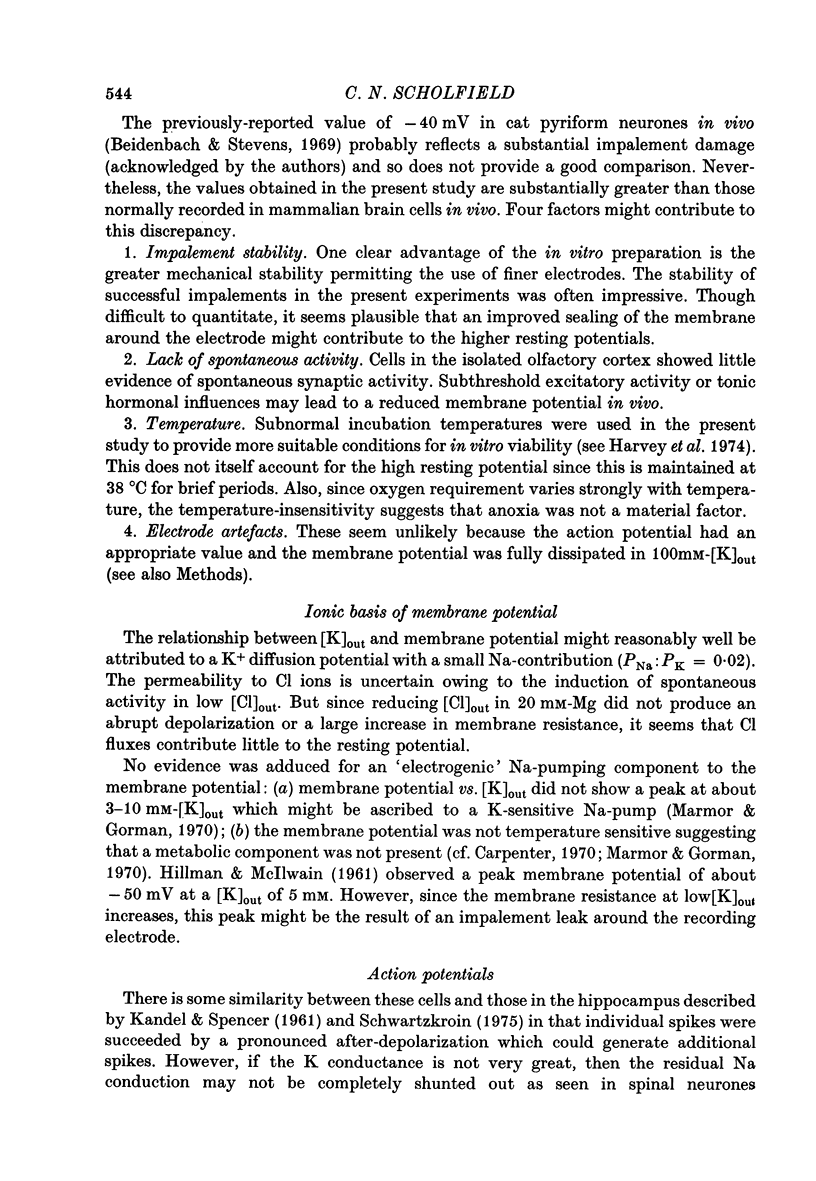
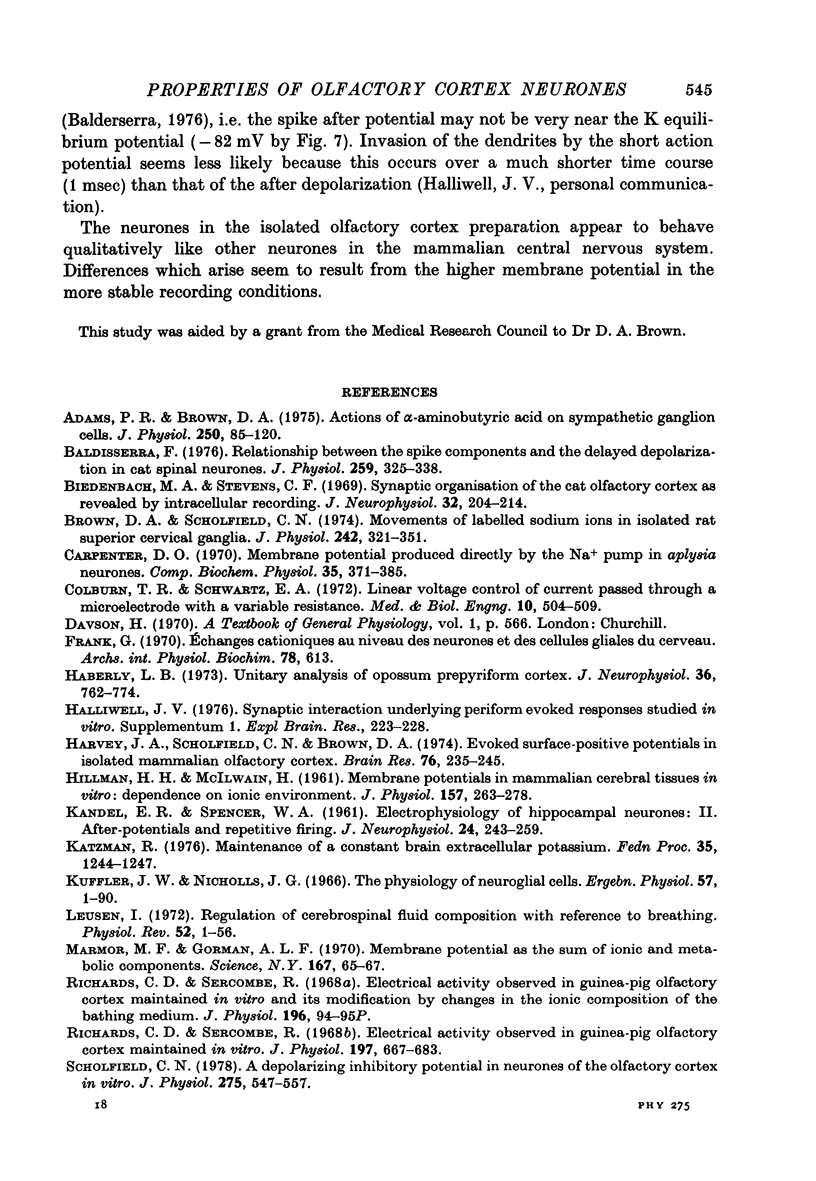
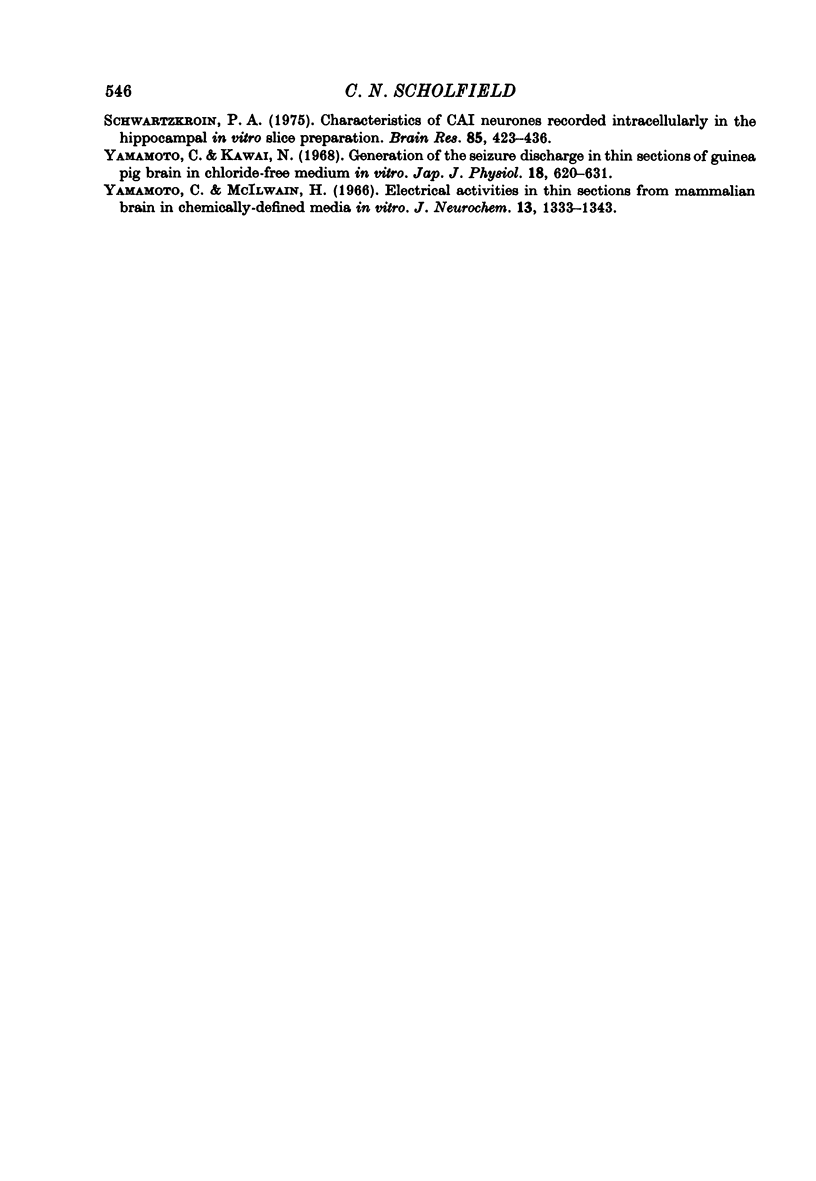
Selected References
These references are in PubMed. This may not be the complete list of references from this article.
- Adams P. R., Brown D. A. Actions of gamma-aminobutyric acid on sympathetic ganglion cells. J Physiol. 1975 Aug;250(1):85–120. doi: 10.1113/jphysiol.1975.sp011044. [DOI] [PMC free article] [PubMed] [Google Scholar]
- Baldissera F. Relationships between the spike components and the delayed depolarization in cat spinal neurones. J Physiol. 1976 Jul;259(2):325–338. doi: 10.1113/jphysiol.1976.sp011468. [DOI] [PMC free article] [PubMed] [Google Scholar]
- Biedenbach M. A., Stevens C. F. Synaptic organization of cat olfactory cortex as revealed by intracellular recording. J Neurophysiol. 1969 Mar;32(2):204–214. doi: 10.1152/jn.1969.32.2.204. [DOI] [PubMed] [Google Scholar]
- Brown D. A., Scholfield C. N. Movements of labelled sodium ions in isolated rat superior cervical ganglia. J Physiol. 1974 Oct;242(2):321–351. doi: 10.1113/jphysiol.1974.sp010710. [DOI] [PMC free article] [PubMed] [Google Scholar]
- Colburn T. R., Schwartz E. A. Linear voltage control of current passed through a micropipette with variable resistance. Med Biol Eng. 1972 Jul;10(4):504–509. doi: 10.1007/BF02474198. [DOI] [PubMed] [Google Scholar]
- HILLMAN H. H., McILWAIN H. Membrane potentials in mammalian cerebral tissues in vitro: dependence on ionic environment. J Physiol. 1961 Jul;157:263–278. doi: 10.1113/jphysiol.1961.sp006720. [DOI] [PMC free article] [PubMed] [Google Scholar]
- Haberly L. B. Unitary analysis of opossum prepyriform cortex. J Neurophysiol. 1973 Jul;36(4):762–774. doi: 10.1152/jn.1973.36.4.762. [DOI] [PubMed] [Google Scholar]
- Harvey J. A., Scholfield C. N., Brown D. A. Evoked surface-positive potentials in isolated mammalian olfactory cortex. Brain Res. 1974 Aug 16;76(2):235–245. doi: 10.1016/0006-8993(74)90457-0. [DOI] [PubMed] [Google Scholar]
- KANDEL E. R., SPENCER W. A. Electrophysiology of hippocampal neurons. II. After-potentials and repetitive firing. J Neurophysiol. 1961 May;24:243–259. doi: 10.1152/jn.1961.24.3.243. [DOI] [PubMed] [Google Scholar]
- Katzman R. Maintenance of a constant brain extracellular potassium. Fed Proc. 1976 May 1;35(6):1244–1247. [PubMed] [Google Scholar]
- Kuffler S. W., Nicholls J. G. The physiology of neuroglial cells. Ergeb Physiol. 1966;57:1–90. [PubMed] [Google Scholar]
- Leusen I. Regulation of cerebrospinal fluid composition with reference to breathing. Physiol Rev. 1972 Jan;52(1):1–56. doi: 10.1152/physrev.1972.52.1.1. [DOI] [PubMed] [Google Scholar]
- Marmor M. F., Gorman A. L. Membrane potential as the sum of ionic and metabolic components. Science. 1970 Jan 2;167(3914):65–67. doi: 10.1126/science.167.3914.65. [DOI] [PubMed] [Google Scholar]
- Richards C. D., Sercombe R. Electrical activity observed in guinea-pig olfactory cortex maintained in vitro and its modification by changes in the ionic composition of the bathing medium. J Physiol. 1968 May;196(2):94P–95P. [PubMed] [Google Scholar]
- Richards C. D., Sercombe R. Electrical activity observed in guinea-pig olfactory cortex maintained in vitro. J Physiol. 1968 Aug;197(3):667–683. doi: 10.1113/jphysiol.1968.sp008581. [DOI] [PMC free article] [PubMed] [Google Scholar]
- Scholfield C. N. A depolarizing inhibitory potential in neurones of the olfactory cortex in vitro. J Physiol. 1978 Feb;275:547–557. doi: 10.1113/jphysiol.1978.sp012207. [DOI] [PMC free article] [PubMed] [Google Scholar]
- Schwartzkroin P. A. Characteristics of CA1 neurons recorded intracellularly in the hippocampal in vitro slice preparation. Brain Res. 1975 Mar 7;85(3):423–436. doi: 10.1016/0006-8993(75)90817-3. [DOI] [PubMed] [Google Scholar]
- Yamamoto C., Kawai N. Generation of the seizure discharge in thin sections from the guinea pig brain in chloride-free medium in vitro. Jpn J Physiol. 1968 Oct 15;18(5):620–631. doi: 10.2170/jjphysiol.18.620. [DOI] [PubMed] [Google Scholar]
- Yamamoto C., McIlwain H. Electrical activities in thin sections from the mammalian brain maintained in chemically-defined media in vitro. J Neurochem. 1966 Dec;13(12):1333–1343. doi: 10.1111/j.1471-4159.1966.tb04296.x. [DOI] [PubMed] [Google Scholar]