Abstract
1. A study has been made of the relation between force and speed of shortening, or lengthening, in isolated twitch and slow muscle fibres, dissected from the iliofibularis muscle of Xenopus laevis. Both after-loaded and quick-release contractions were studied. Twitch fibres were stimulated electrically to give tetanic contractions (5-20 °C); slow fibres were activated by a rapid change to solutions with high K concentration (30-75 mM; experiments at 21-24 °C).
2. The velocity of slow fibres was constant during shortening over 10% length change in after-loaded contractions, except at forces exceeding about 0·8 of isometric tension, P0. In quick-release experiments, shortening velocity was found to depend not only on the relative load, P/P0, but also on the instant when the release was made. With increasing time after onset of contraction the initial rate of shortening decreased; also, a progressive fall in speed during shortening became more marked.
3. The fall in initial shortening speed with time of release from the onset of a contracture was more pronounced at high [K]o than at low.
4. The relation between the relative force, P/P0, and shortening velocity, V, in after-loaded contractions (75 mM-K) and quick-release contractions (45 mM-K, early releases) in slow fibres could be represented by a hyperbola with the constants a = 0·10P0, b = 0·11 lengths/sec; extrapolated Vmax. was 1·10 lengths/sec.
5. Isometric tension and maximum shortening velocity in slow fibres were very nearly constant between 32 and 75 mM-K. a/P0, however, was clearly reduced at 32 mM-K, representing a more curved P-V relation.
6. Force—velocity data for twitch fibres (quick-release contractions, 20 °C) were reasonably well fitted by a hyperbola (a = 0·38P0, b = 1·97 lengths/sec, Vmax. = 5·20 lengths/sec), but a systematic deviation was observed for forces exceeding 0·6P0.
7. a/P0 for twitch fibres was found to be independent of temperature in the range 5-20 °C. Q10 for b was 2·24 (10-20 °C), and 2·86 (5-10 °C).
8. Vmax. for twitch fibres was calculated to be 6·34 lengths/sec at 22·5 °C, the average temperature in the slow fibre experiments. The maximum shortening velocity in twitch fibres is thus 6 times higher than in slow fibres.
9. When loads in the range 1·1-1·4P0 were quickly applied to an actively contracting slow fibre, lengthening of the fibre occurred in two phases, an initial rapid phase, followed by a phase of extremely slow lengthening. In corresponding experiments on twitch fibres lengthening was rapid at first and then gradually became slower.
10. Factors affecting the shape of the force—velocity curve are discussed. Calculations based on A. F. Huxley's (1957) model for muscle contraction indicated that cross-bridge turnover rate is about 15 times lower in slow than in twitch fibres.
Full text
PDF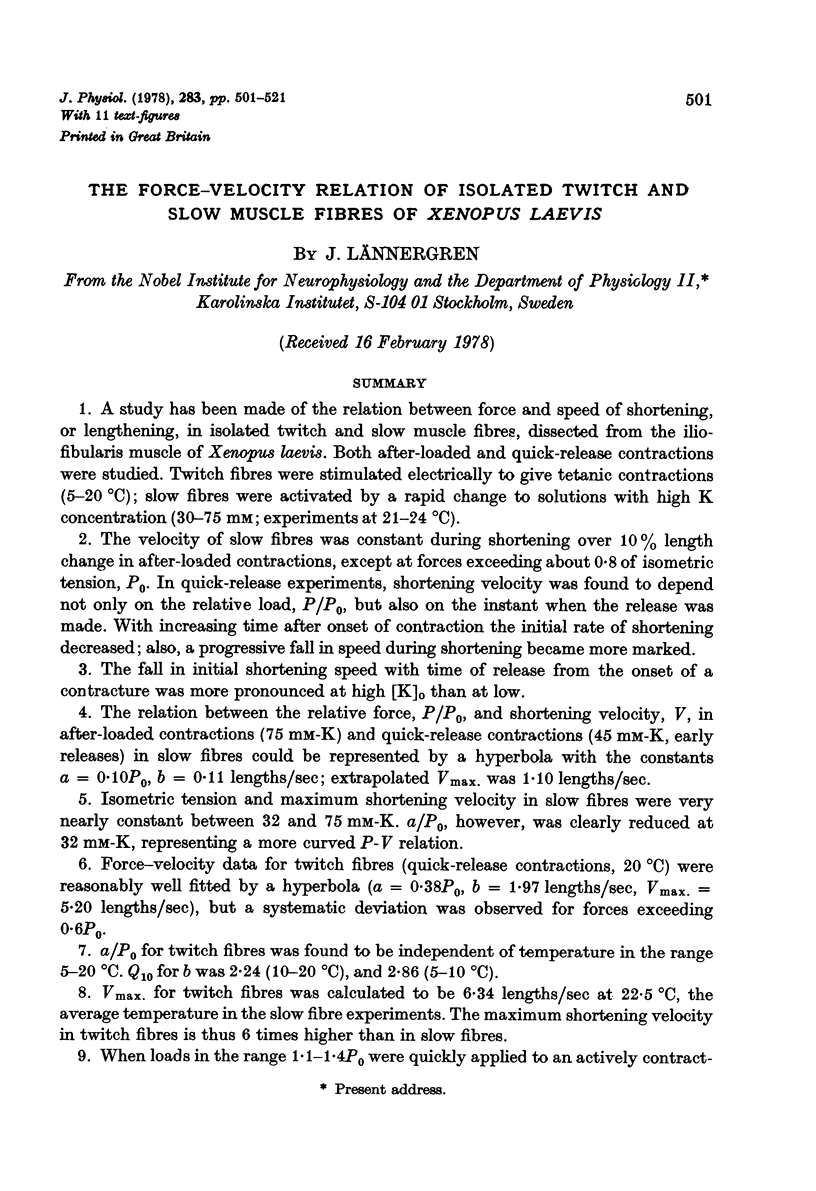
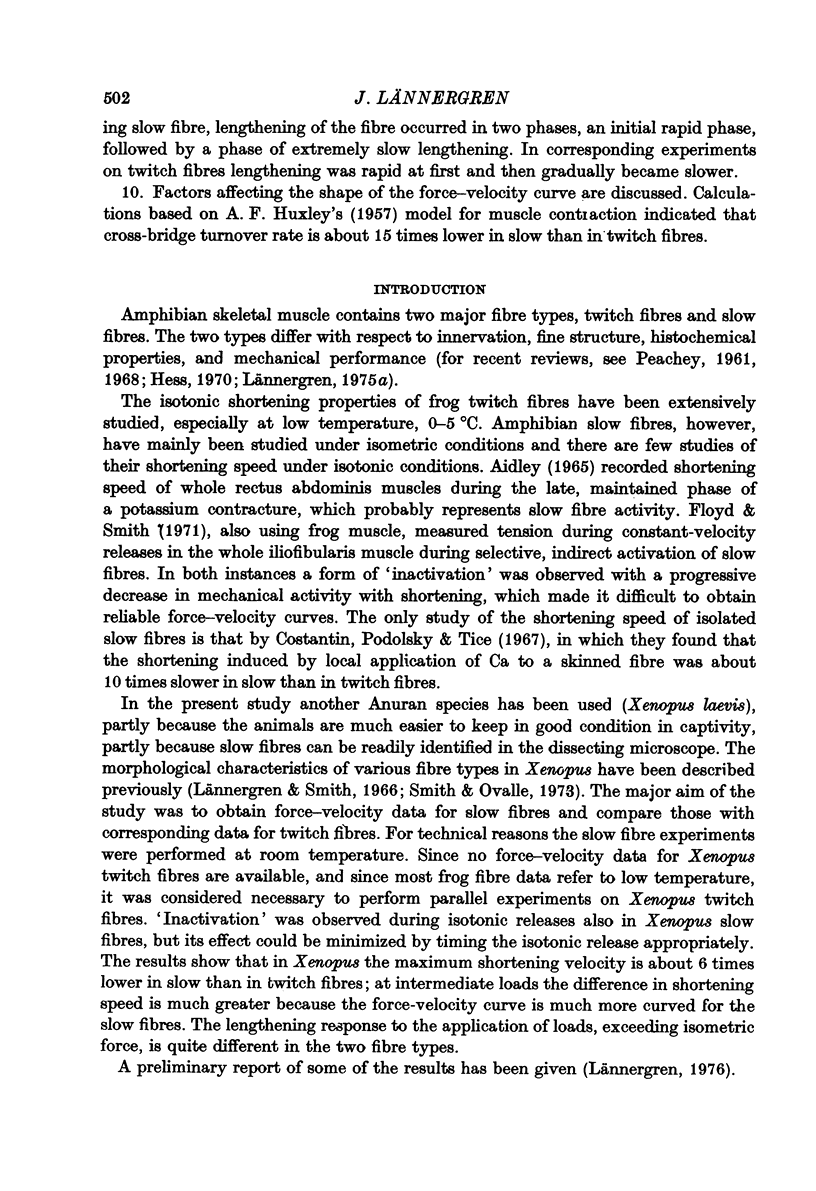
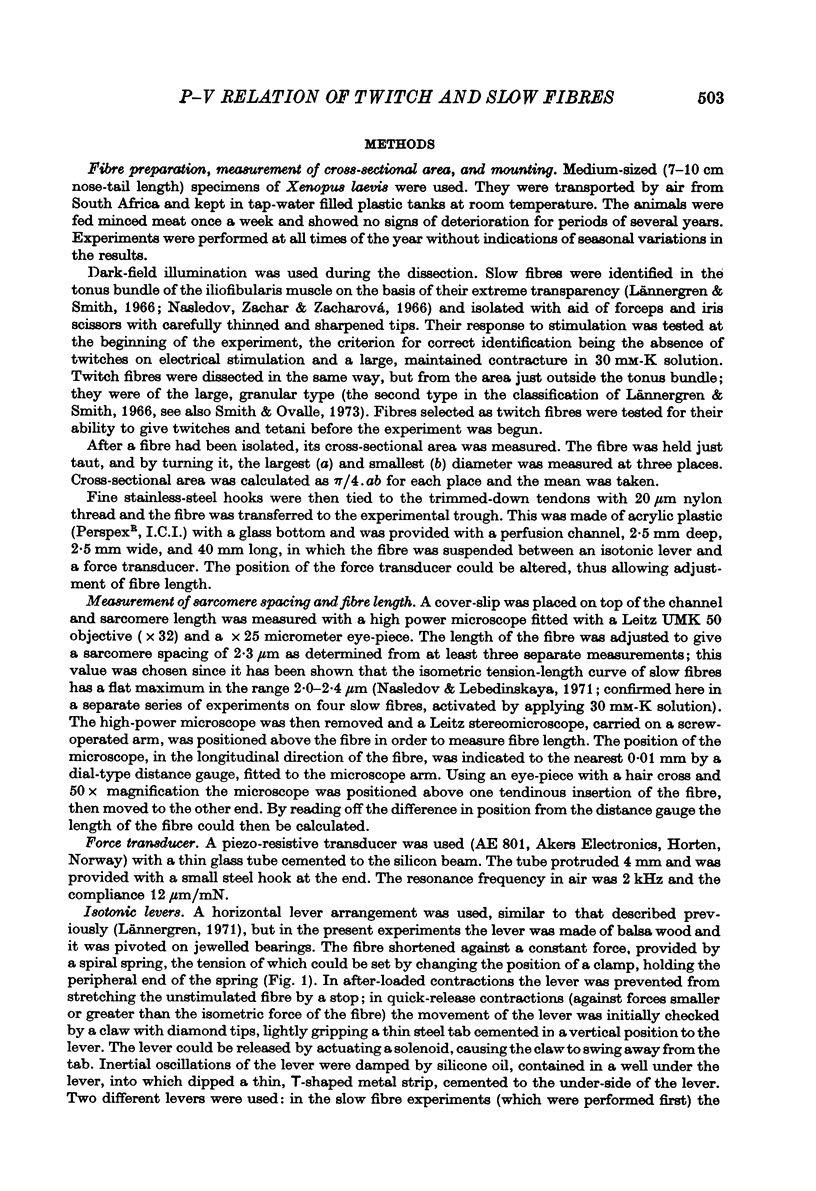
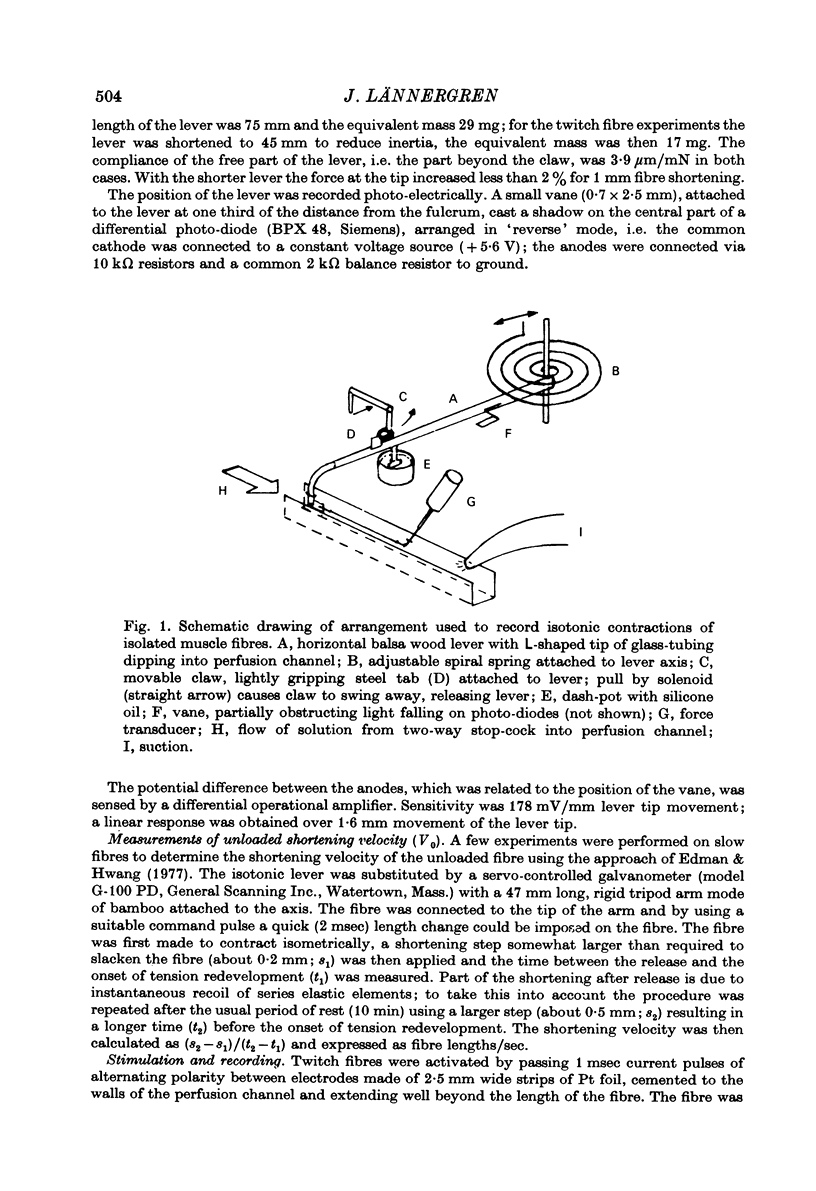
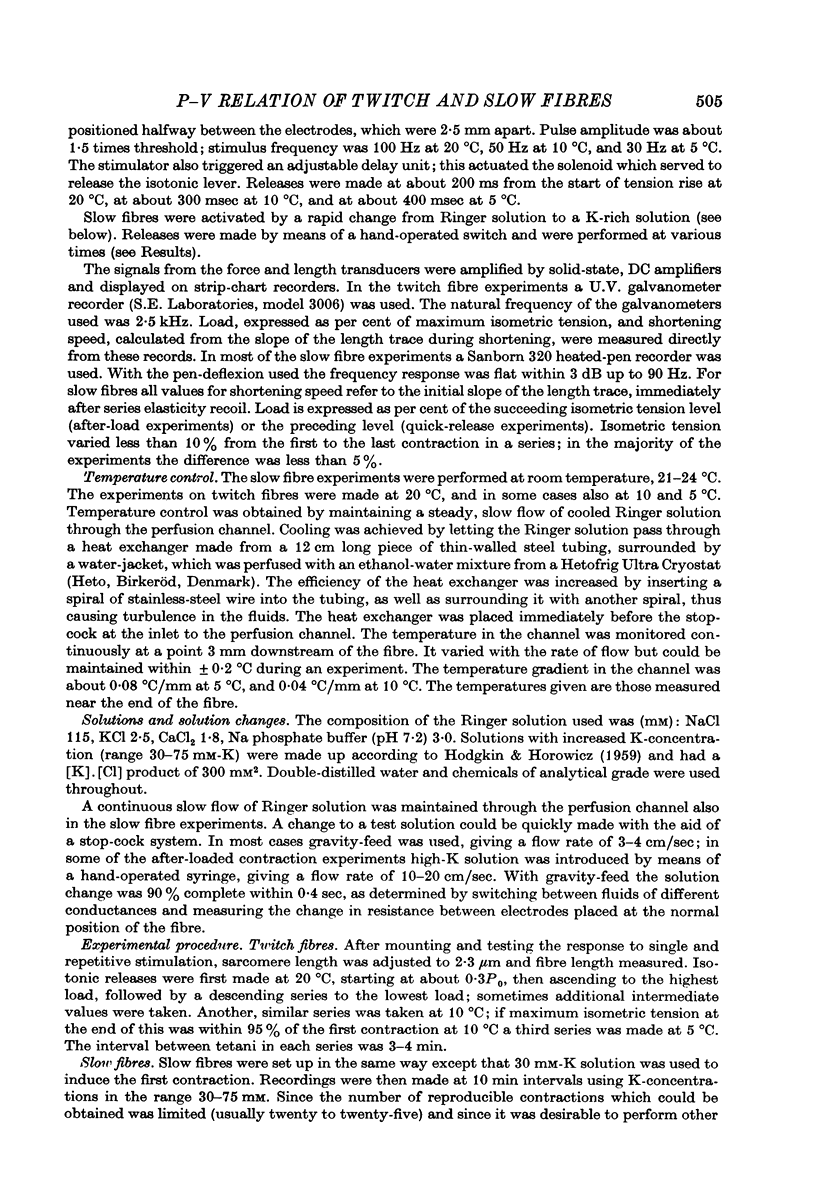
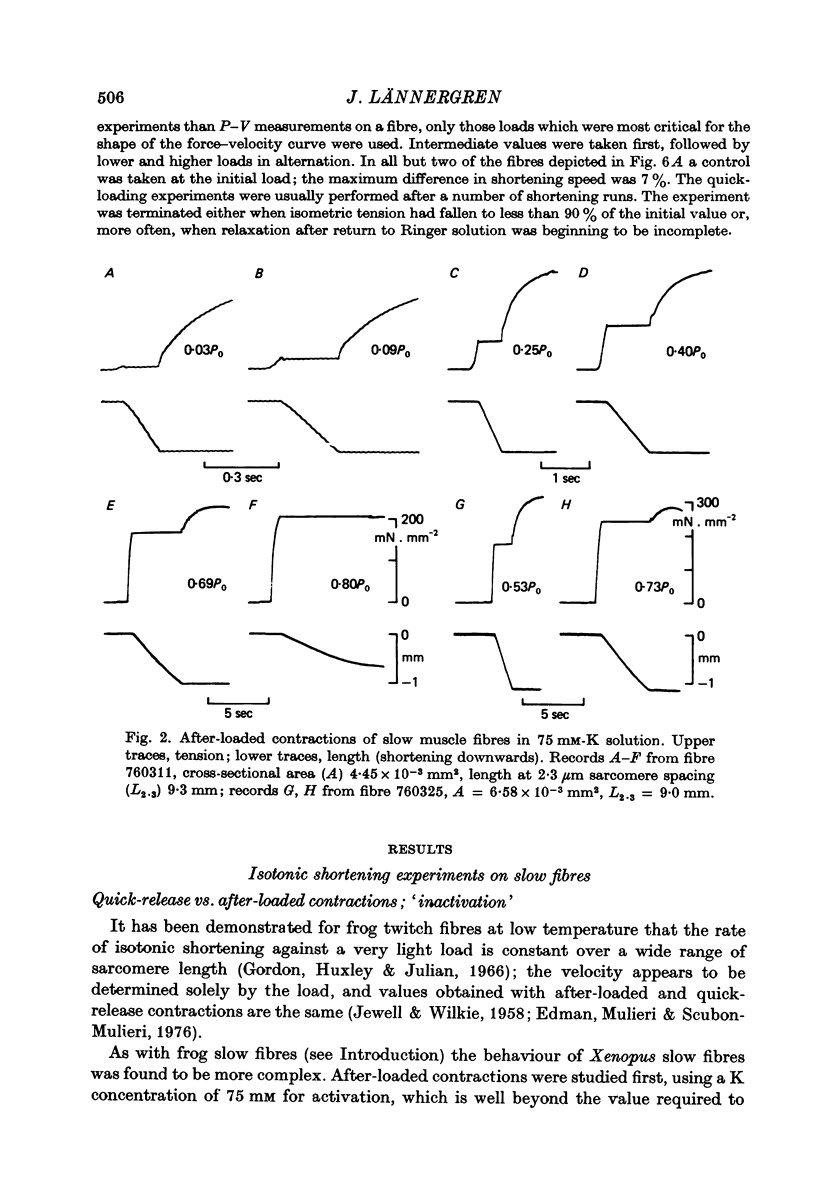
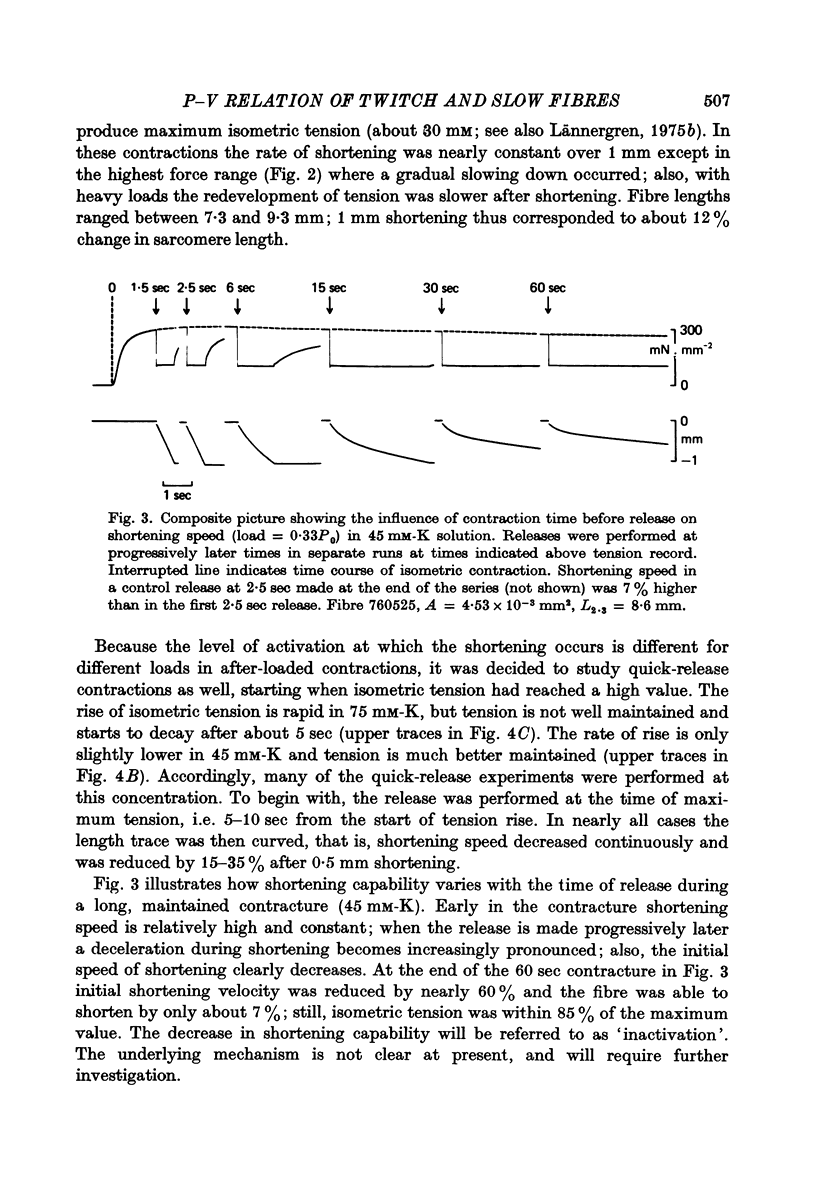
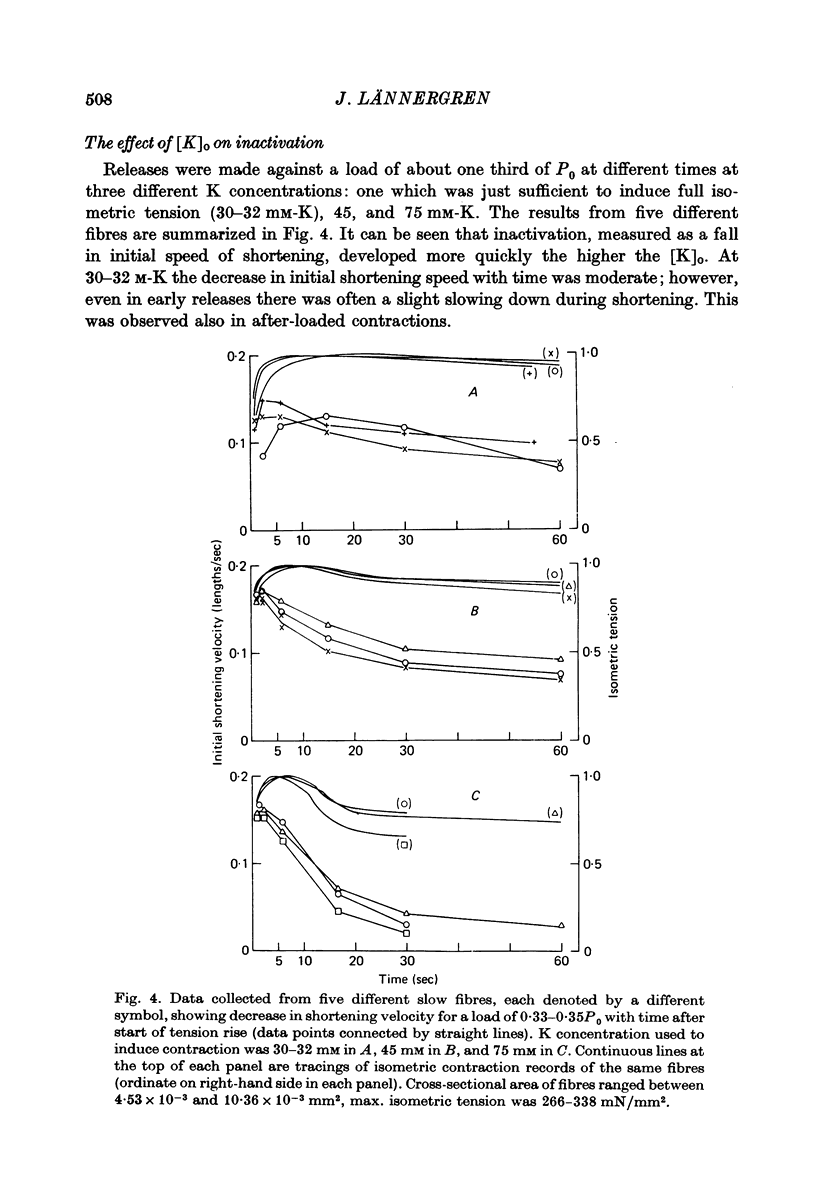
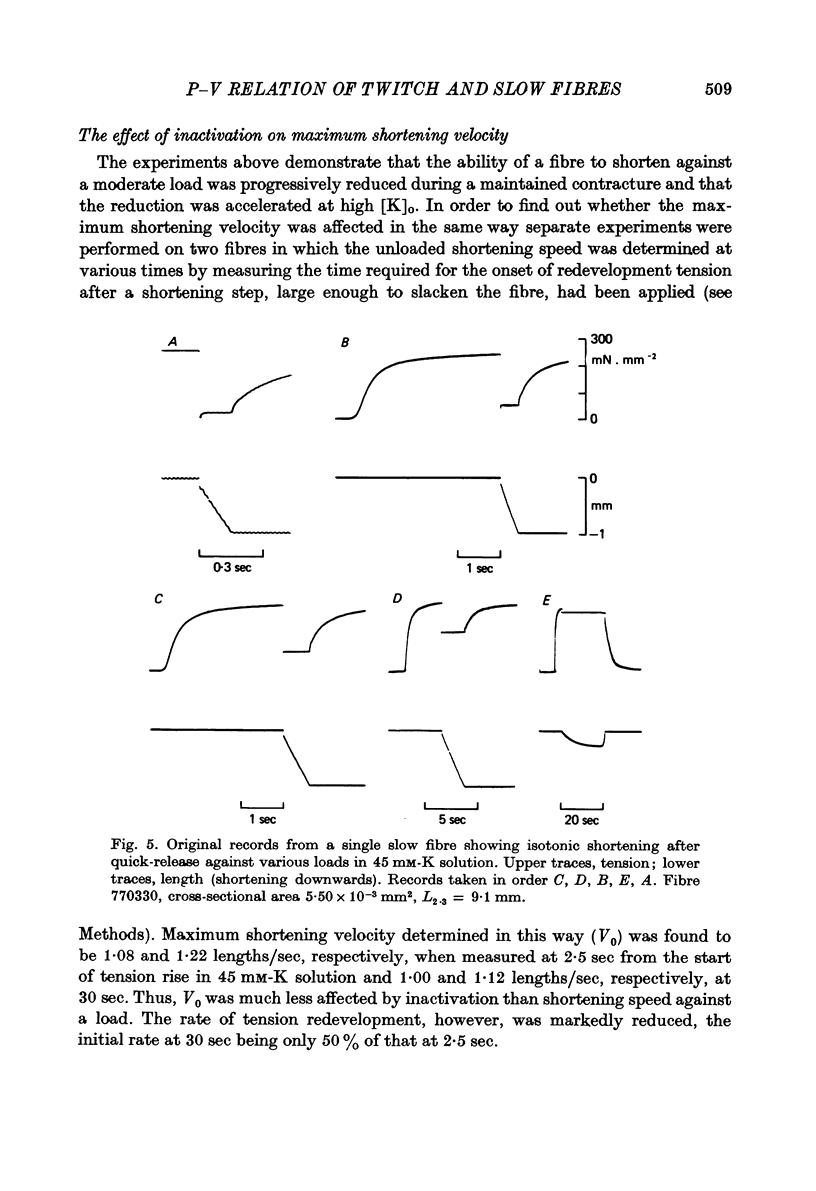
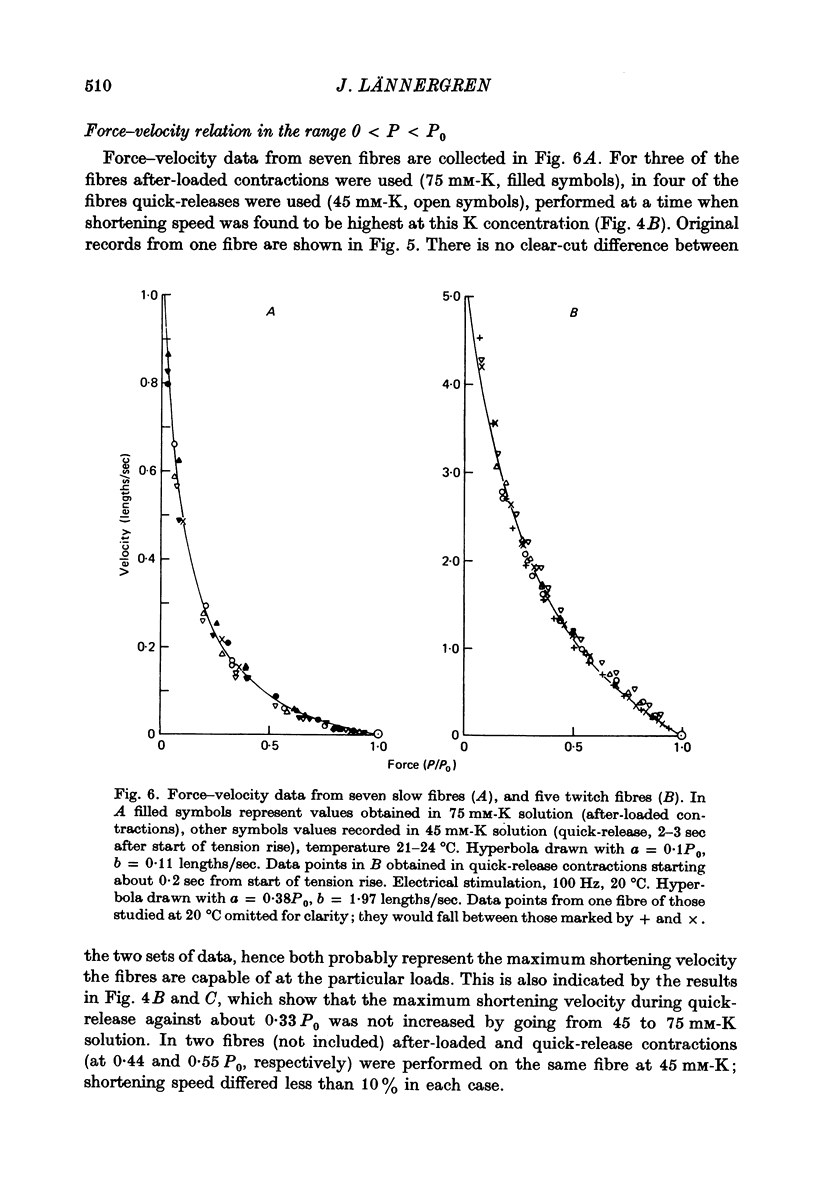
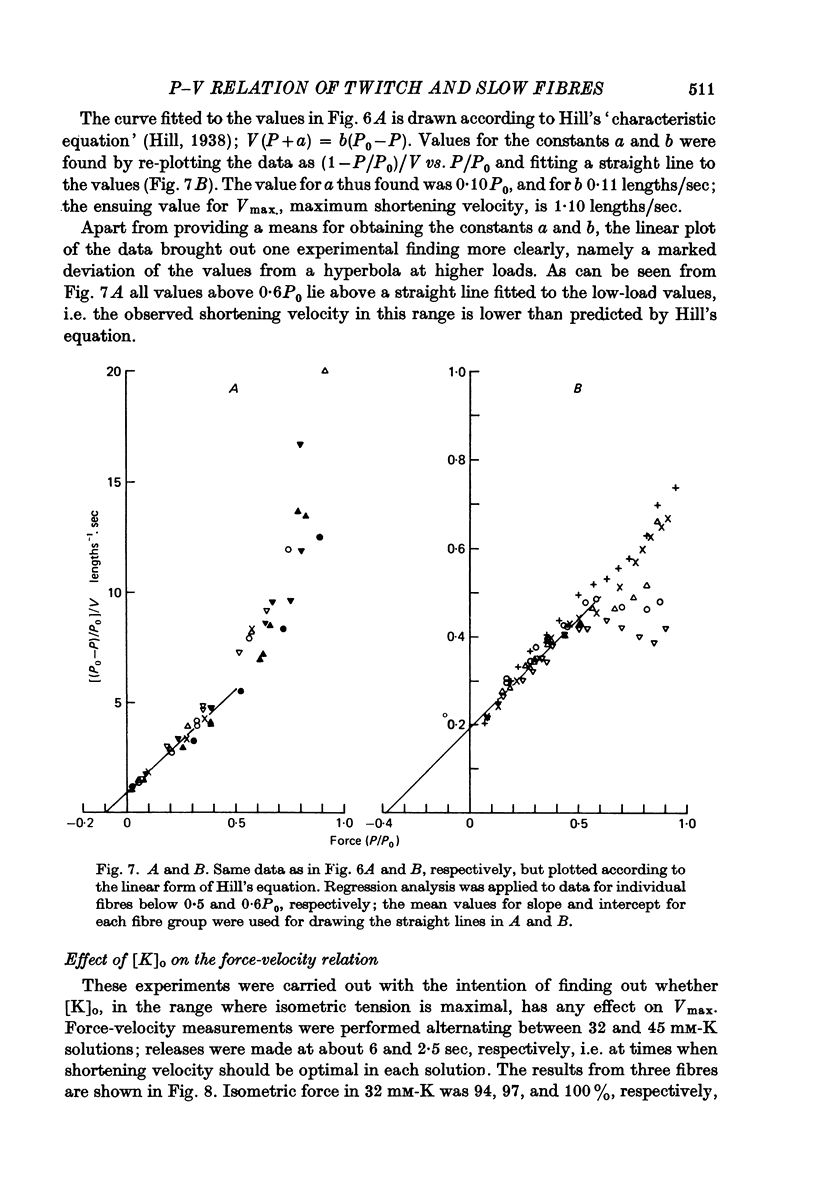
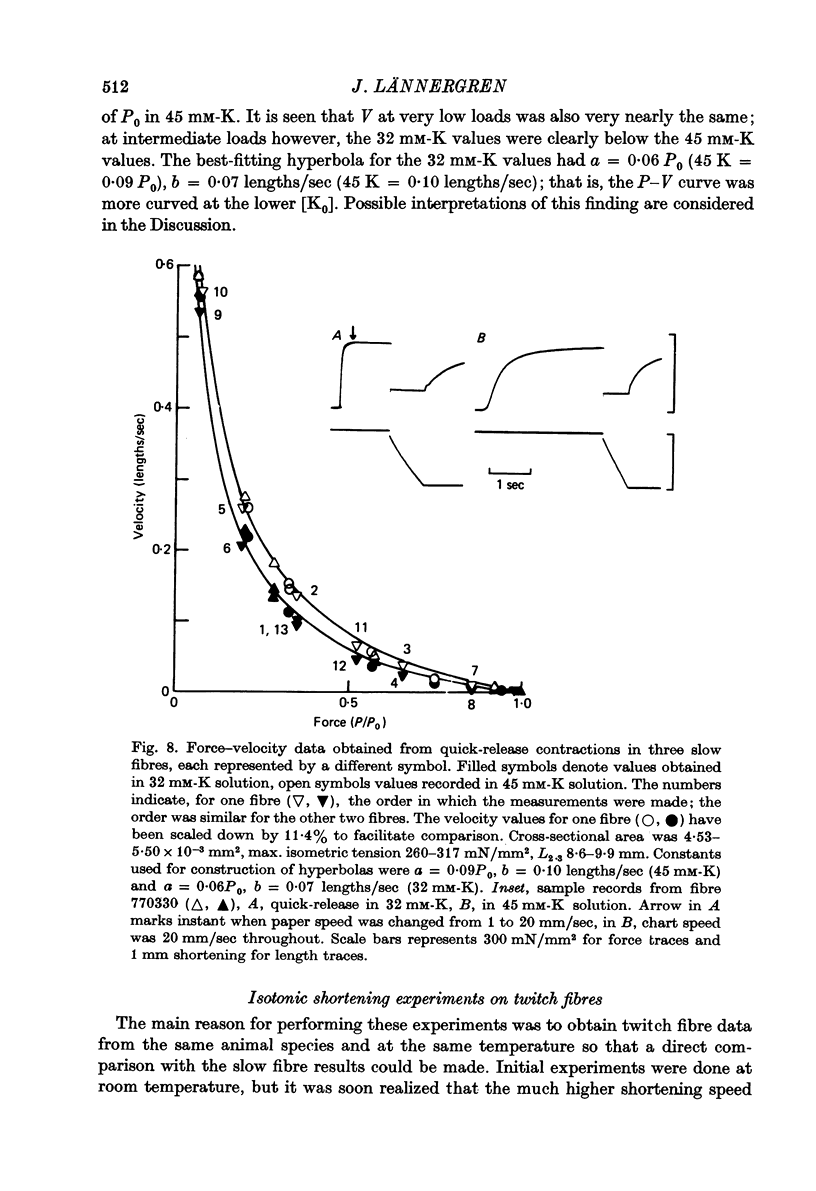
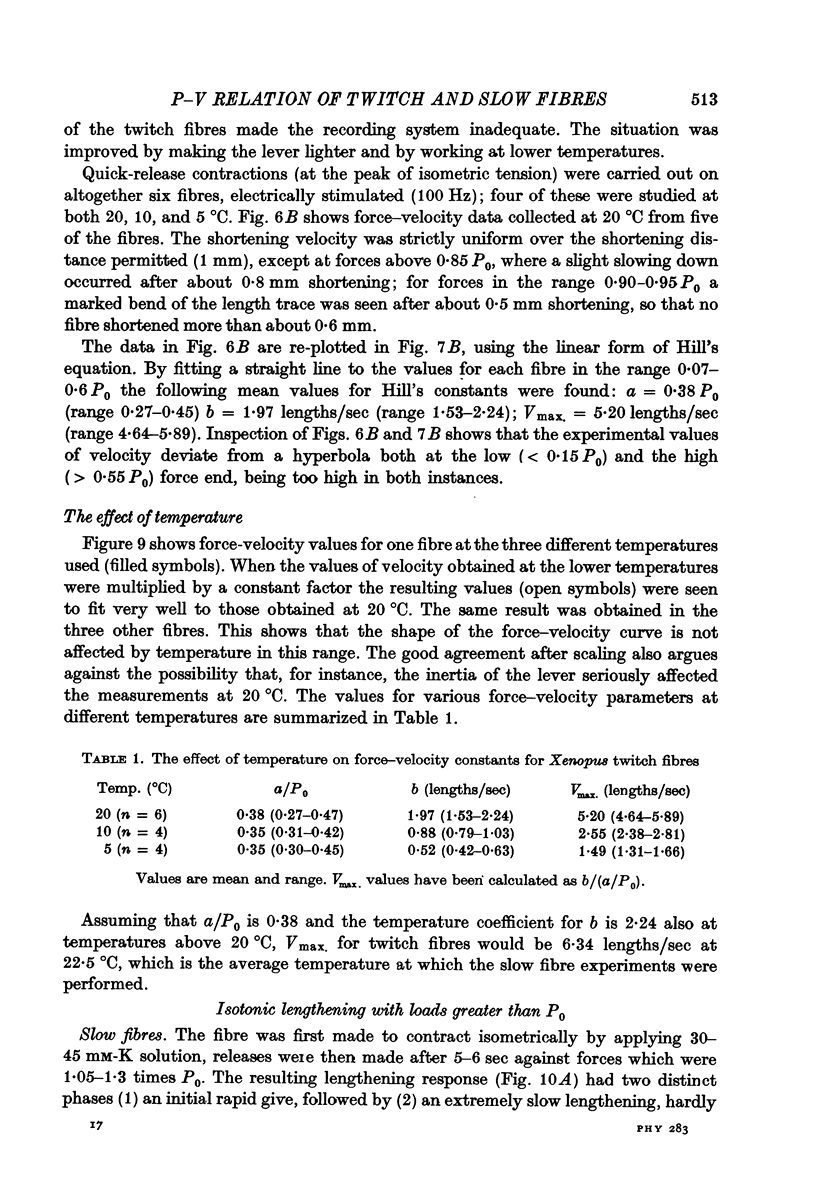
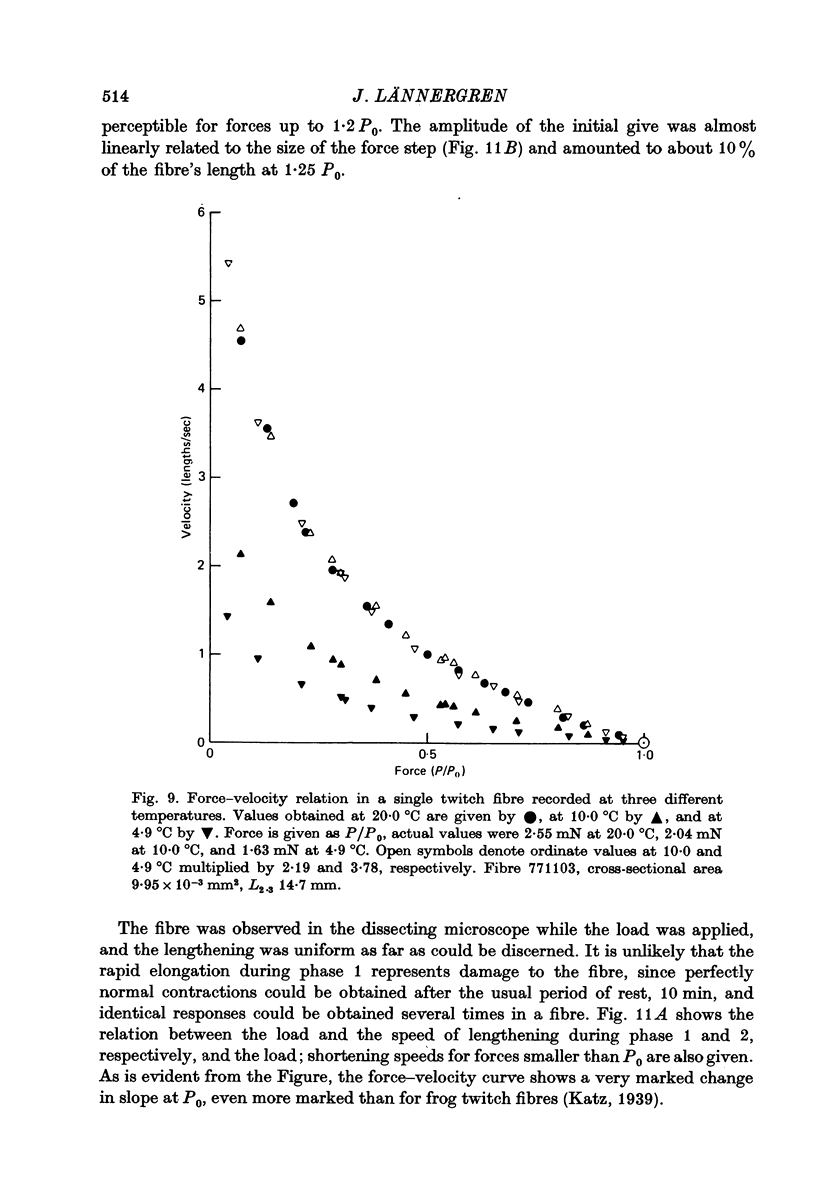
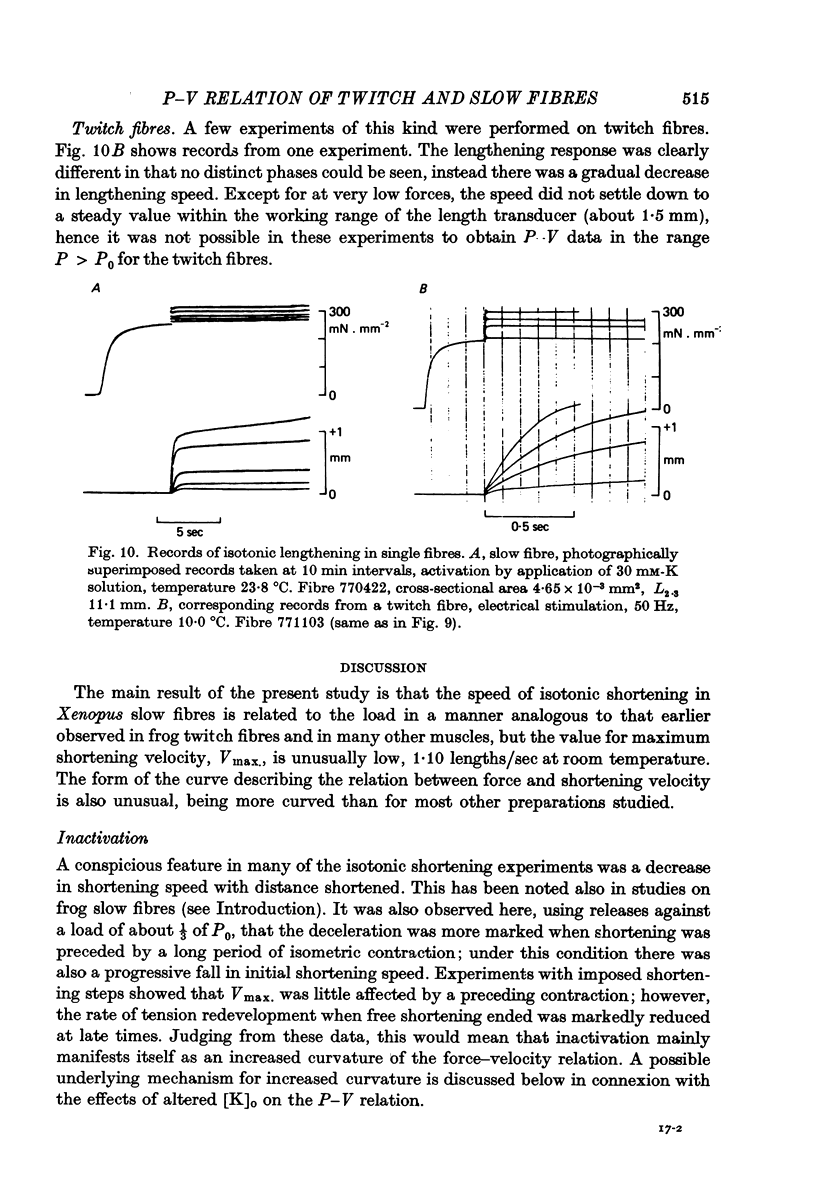
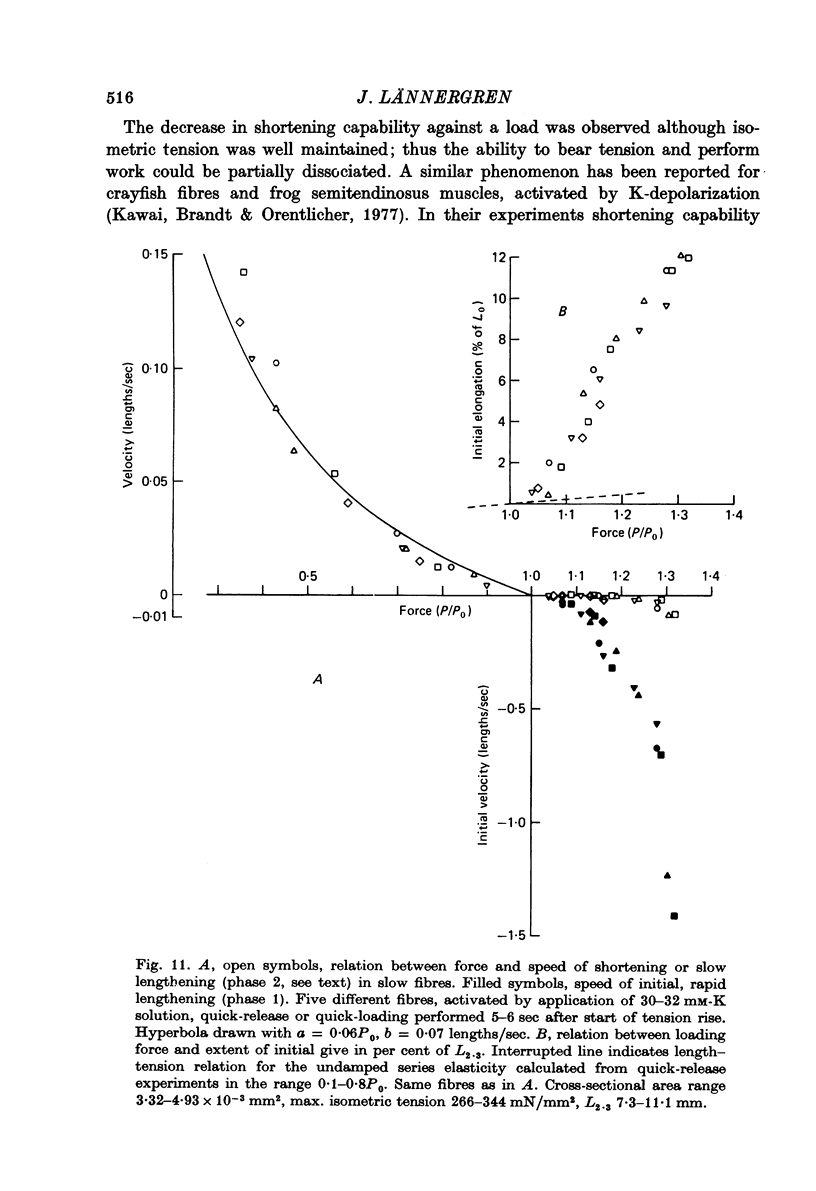
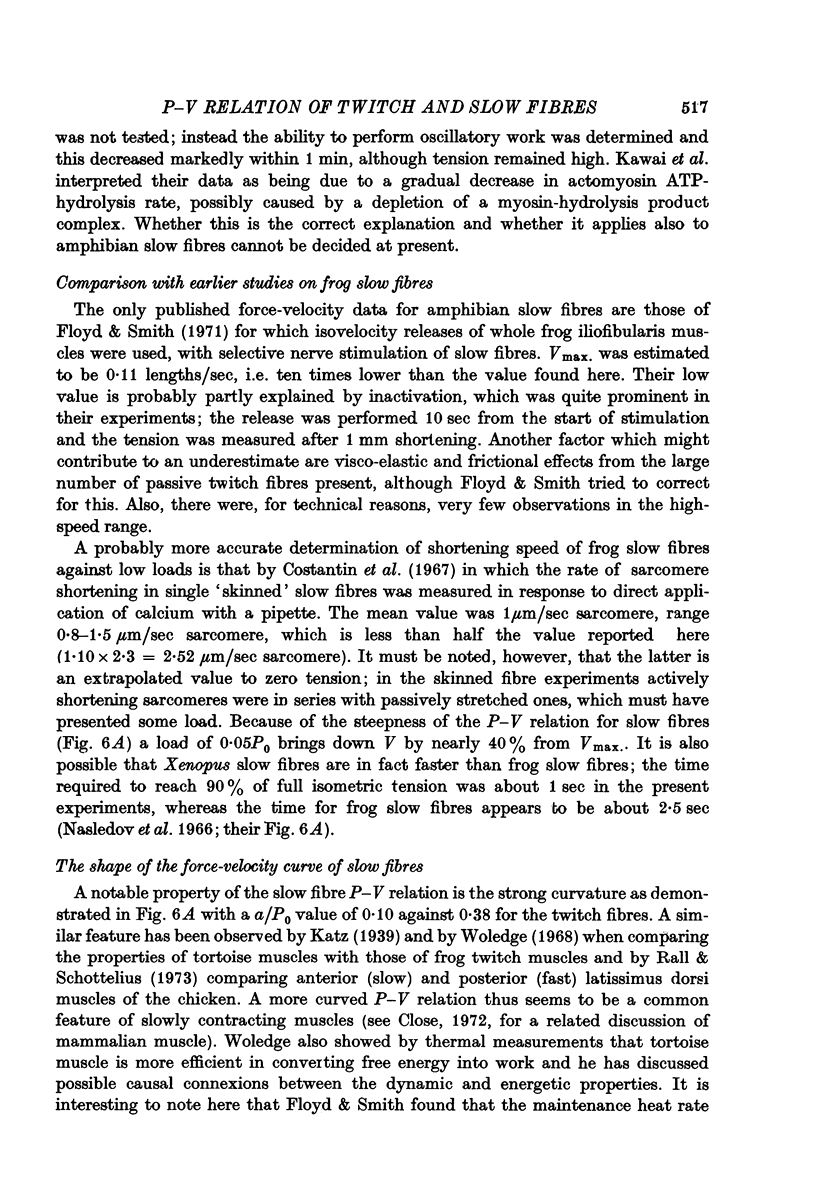
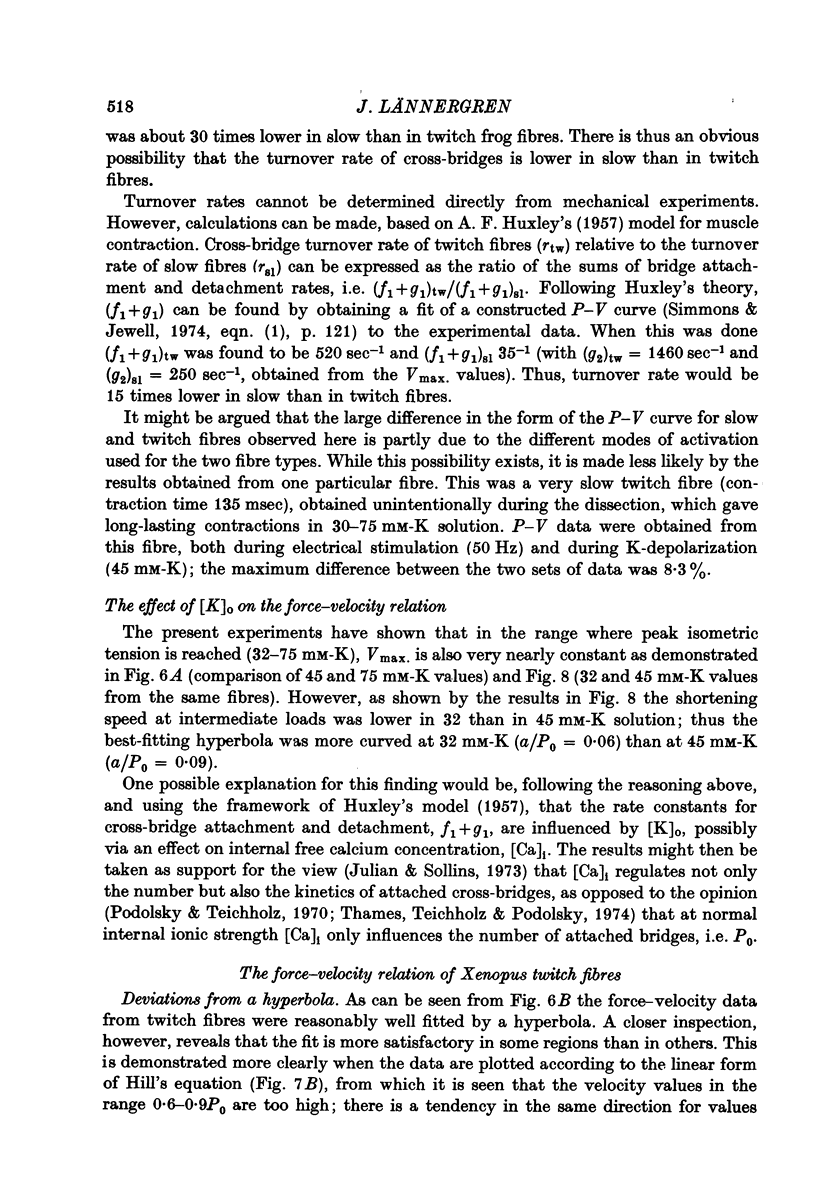
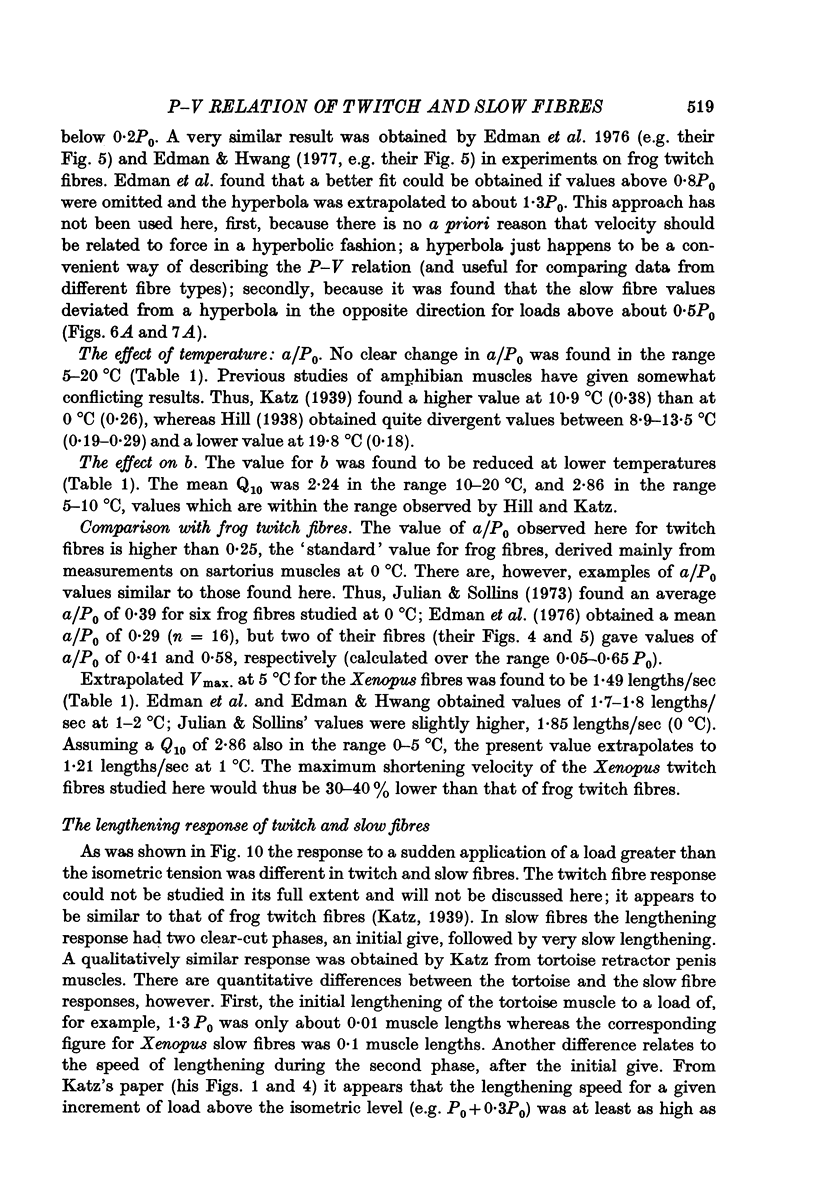
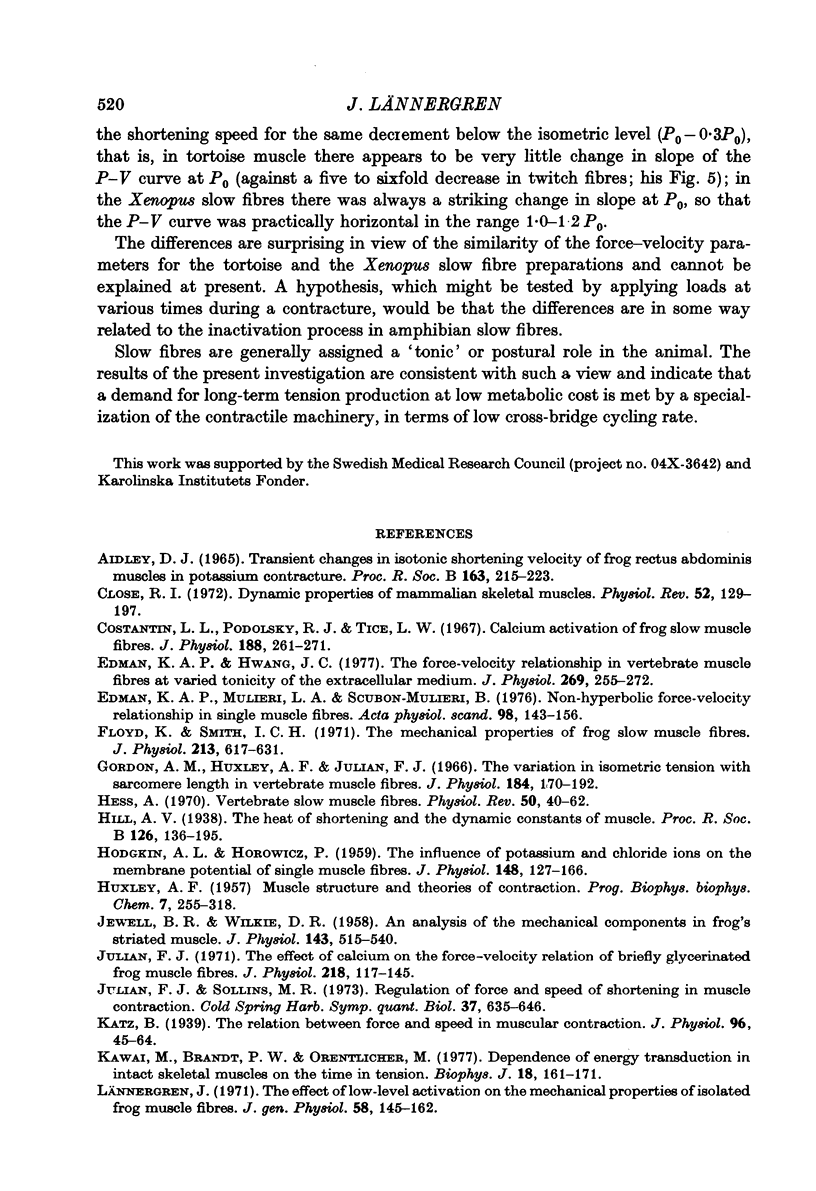
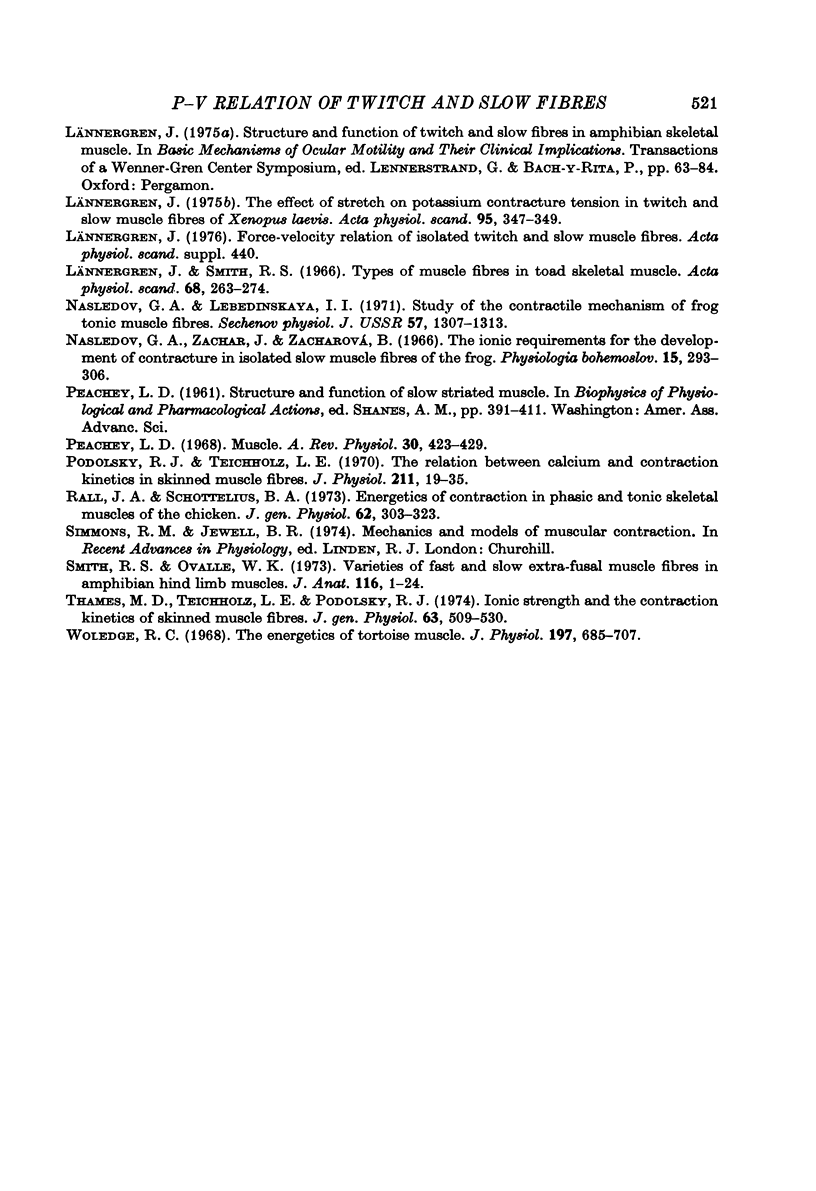
Selected References
These references are in PubMed. This may not be the complete list of references from this article.
- Close R. I. Dynamic properties of mammalian skeletal muscles. Physiol Rev. 1972 Jan;52(1):129–197. doi: 10.1152/physrev.1972.52.1.129. [DOI] [PubMed] [Google Scholar]
- Costantin L. L., Podolsky R. J., Tice L. W. Calcium activation of frog slow muscle fibres. J Physiol. 1967 Jan;188(2):261–271. doi: 10.1113/jphysiol.1967.sp008137. [DOI] [PMC free article] [PubMed] [Google Scholar]
- Edman K. A., Hwang J. C. The force-velocity relationship in vertebrate muscle fibres at varied tonicity of the extracellular medium. J Physiol. 1977 Jul;269(2):255–272. doi: 10.1113/jphysiol.1977.sp011901. [DOI] [PMC free article] [PubMed] [Google Scholar]
- Edman K. A., Mulieri L. A., Scubon-Mulieri B. Non-hyperbolic force-velocity relationship in single muscle fibres. Acta Physiol Scand. 1976 Oct;98(2):143–156. doi: 10.1111/j.1748-1716.1976.tb00234.x. [DOI] [PubMed] [Google Scholar]
- Floyd K., Smith I. C. The mechanical and thermal properties of frog slow muscle fibres. J Physiol. 1971 Mar;213(3):617–631. doi: 10.1113/jphysiol.1971.sp009404. [DOI] [PMC free article] [PubMed] [Google Scholar]
- Gordon A. M., Huxley A. F., Julian F. J. The variation in isometric tension with sarcomere length in vertebrate muscle fibres. J Physiol. 1966 May;184(1):170–192. doi: 10.1113/jphysiol.1966.sp007909. [DOI] [PMC free article] [PubMed] [Google Scholar]
- HODGKIN A. L., HOROWICZ P. The influence of potassium and chloride ions on the membrane potential of single muscle fibres. J Physiol. 1959 Oct;148:127–160. doi: 10.1113/jphysiol.1959.sp006278. [DOI] [PMC free article] [PubMed] [Google Scholar]
- HUXLEY A. F. Muscle structure and theories of contraction. Prog Biophys Biophys Chem. 1957;7:255–318. [PubMed] [Google Scholar]
- Hess A. Vertebrate slow muscle fibers. Physiol Rev. 1970 Jan;50(1):40–62. doi: 10.1152/physrev.1970.50.1.40. [DOI] [PubMed] [Google Scholar]
- JEWELL B. R., WILKIE D. R. An analysis of the mechanical components in frog's striated muscle. J Physiol. 1958 Oct 31;143(3):515–540. doi: 10.1113/jphysiol.1958.sp006075. [DOI] [PMC free article] [PubMed] [Google Scholar]
- Julian F. J. The effect of calcium on the force-velocity relation of briefly glycerinated frog muscle fibres. J Physiol. 1971 Oct;218(1):117–145. doi: 10.1113/jphysiol.1971.sp009607. [DOI] [PMC free article] [PubMed] [Google Scholar]
- Katz B. The relation between force and speed in muscular contraction. J Physiol. 1939 Jun 14;96(1):45–64. doi: 10.1113/jphysiol.1939.sp003756. [DOI] [PMC free article] [PubMed] [Google Scholar]
- Kawai M., Brandt P., Orentlicher M. Dependence of energy transduction in intact skeletal muscles on the time in tension. Biophys J. 1977 May;18(2):161–172. doi: 10.1016/S0006-3495(77)85605-1. [DOI] [PMC free article] [PubMed] [Google Scholar]
- Lännergren J. The effect of stretch on potassium contracture tension in twitch and slow muscle fibres of Xenopus laevis. Acta Physiol Scand. 1975 Nov;95(3):347–349. doi: 10.1111/j.1748-1716.1975.tb10060.x. [DOI] [PubMed] [Google Scholar]
- Nasledov G. A., Lebedinskaia I. I. Issledovaniia sokratitel'nogo mekhanizma tonicheskikh myshechnykh volokon liagushki. Fiziol Zh SSSR Im I M Sechenova. 1971 Sep;57(9):1307–1312. [PubMed] [Google Scholar]
- Nasledov G. A., Zachar J., Zacharová D. The ionic requirements for the development of contracture in isolated slow muscle fibres of the frog. Physiol Bohemoslov. 1966;15(4):293–306. [PubMed] [Google Scholar]
- Podolsky R. J., Teichholz L. E. The relation between calcium and contraction kinetics in skinned muscle fibres. J Physiol. 1970 Nov;211(1):19–35. doi: 10.1113/jphysiol.1970.sp009263. [DOI] [PMC free article] [PubMed] [Google Scholar]
- Rall J. A., Schottelius B. A. Energetics of contraction in phasic and tonic skeletal muscles of the chicken. J Gen Physiol. 1973 Sep;62(3):303–323. doi: 10.1085/jgp.62.3.303. [DOI] [PMC free article] [PubMed] [Google Scholar]
- Smith R. S., Ovalle W. K., Jr Varieties of fast and slow extrafusal muscle fibres in amphibian hind limb muscles. J Anat. 1973 Oct;116(Pt 1):1–24. [PMC free article] [PubMed] [Google Scholar]
- Thames M. D., Teichholz L. E., Podolsky R. J. Ionic strength and the contraction kinetics of skinned muscle fibers. J Gen Physiol. 1974 Apr;63(4):509–530. doi: 10.1085/jgp.63.4.509. [DOI] [PMC free article] [PubMed] [Google Scholar]
- Woledge R. C. The energetics of tortoise muscle. J Physiol. 1968 Aug;197(3):685–707. doi: 10.1113/jphysiol.1968.sp008582. [DOI] [PMC free article] [PubMed] [Google Scholar]