Abstract
1. Characteristics of glycyl-L-leucine influx across the mucosal border of isolated guinea-pig ileum have been investigated. The influx of the peptide was measured with glycine-labelled or leucine-labelled compounds (Gly*-Leu or Gly-Leu*) and compared with that of a constituent amino acids under various experimental conditions 2. Gly-Leu* influx over a wide range of peptide concentrations. The latter obeyed simple Michaelis--Menten kinetics whereas the former could be described in terms of two saturable components. 3. Total replacement of medium Na with mannitol had no effect on Gly*-Leu influx, while it markedly reduced Gly-Leu* influx to a level slightly greater than Gly*-Leu influx. L-Leucine influx was partially dependent on Na in contrast to glycine influx which was absolutely dependent on Na. 4. Gly*-Leu influx was not inhibited by the simultaneous presence of glycine or L-isoleucine, while Gly-Leu* influx was strongly inhibited by L-leucine and L-isoleucine. Gly-Leu* influx under submaximal inhibition by L-isoleucine was about the same as Gly*-Leu influx. Di- or tri-glycine did not inhibit glycyl-L-leucine influx, while glycyl-L-leucine markedly inhibited diglycine influx, the inhibition being not competitive but of the mixed type. 5. A Michaelis--Menten type relation was observed for the increment in the transmural potential induced by glycyl-L-leucine, L-leucine or the mixture of the dipeptide and L-leucine. In all cases, the values of the maximum potential change were identical, suggesting that a single electrogenic transfer mechanism was operating in these cases. 6. It is concluded that about a half of glycyl-L-leucine influx is mediated by a carrier system for intact glycyl-L-leucine which is independent of sodium, and the other half is transported as L-leucine after membrane surface hydrolysis, part of this component being sodium-dependent and electrogenic. It is also suggested that the carrier sites for glycyl-L-leucine and glycylglycine are very closely located but separated.
Full text
PDF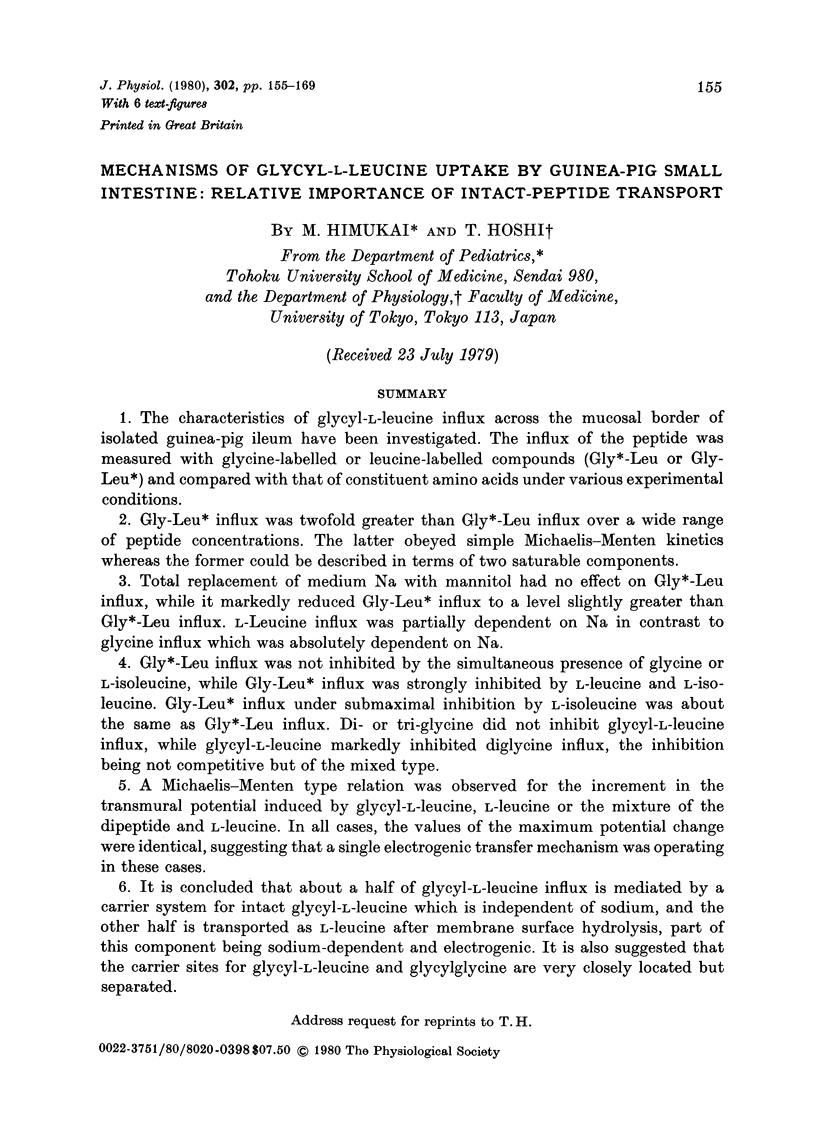
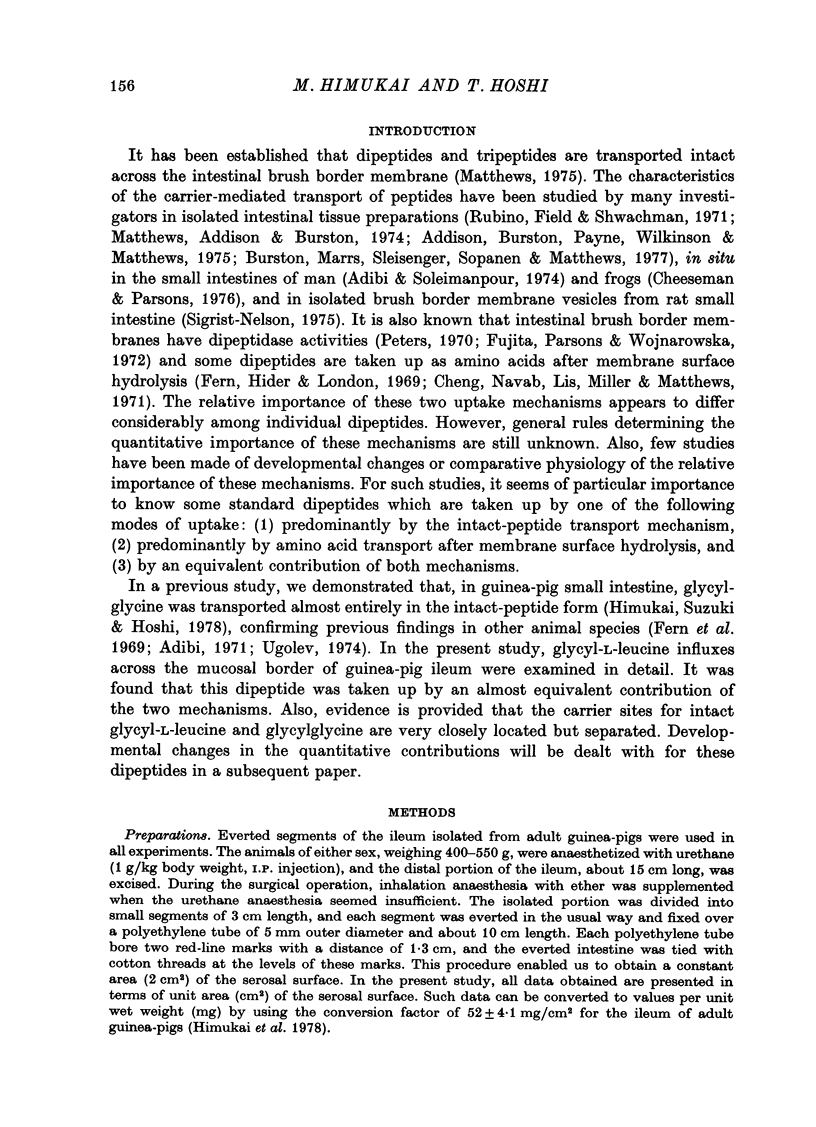
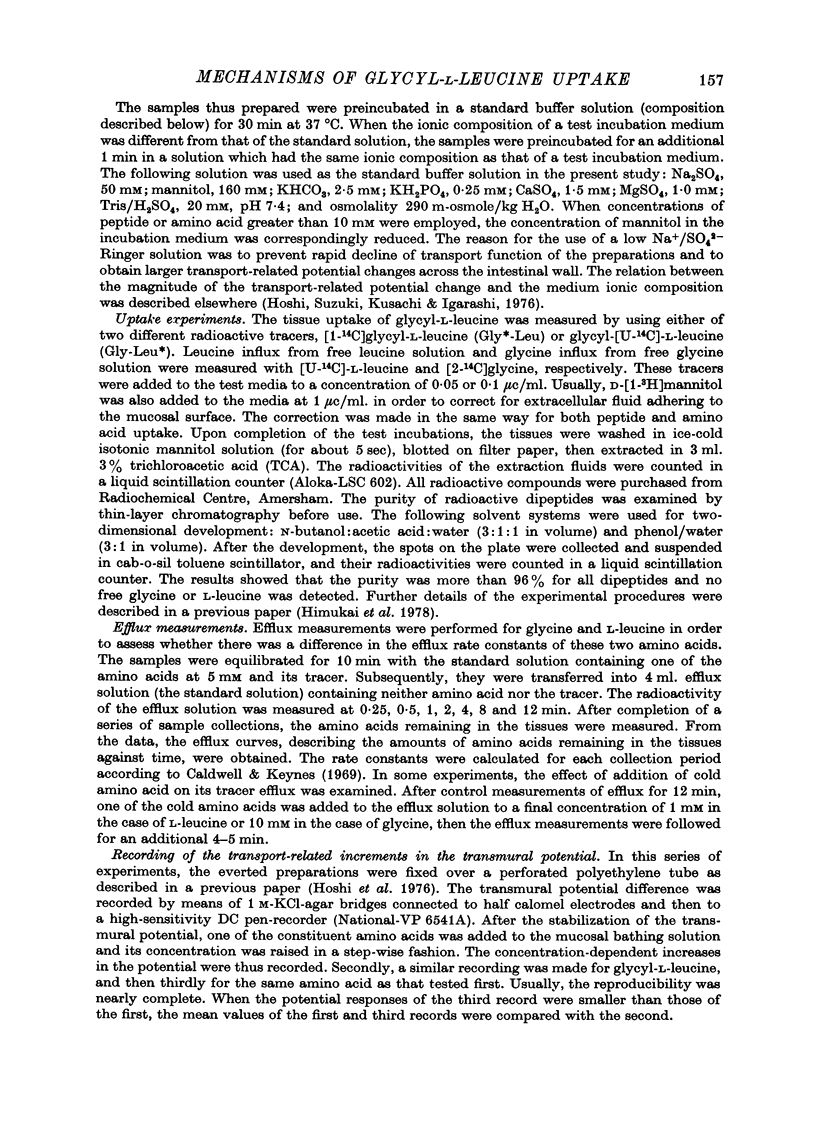
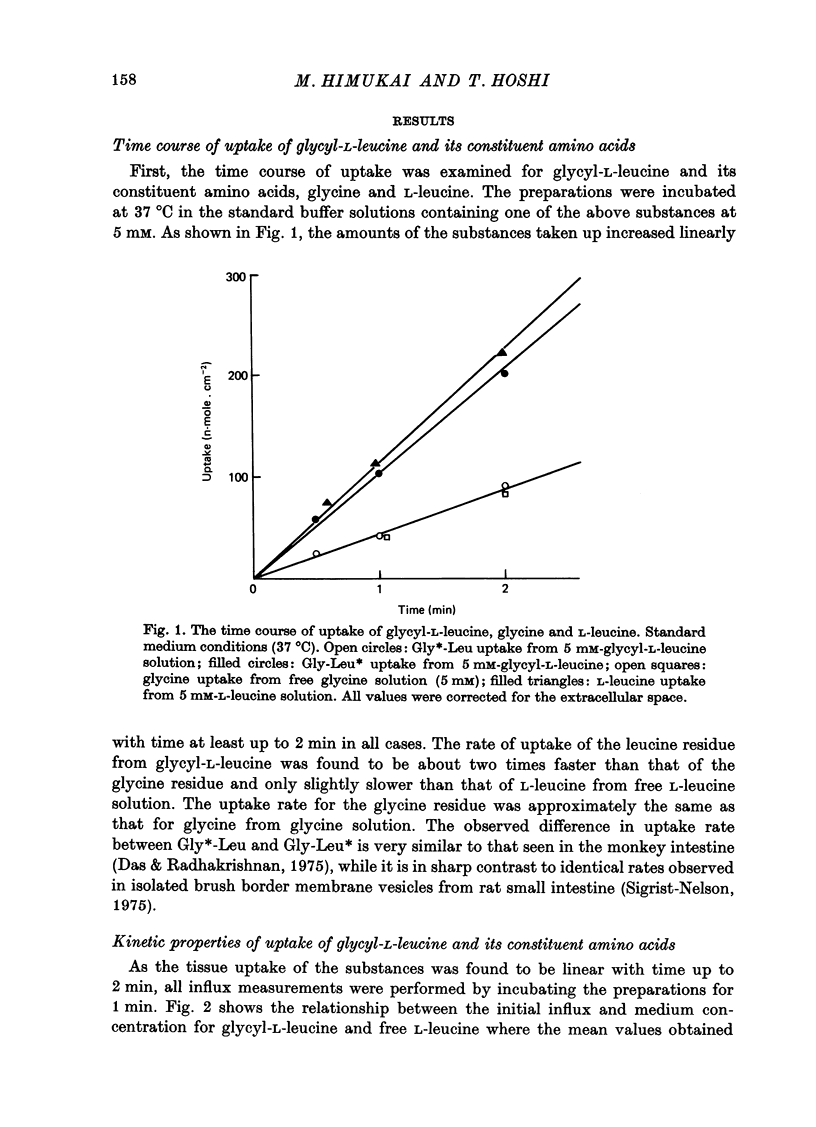
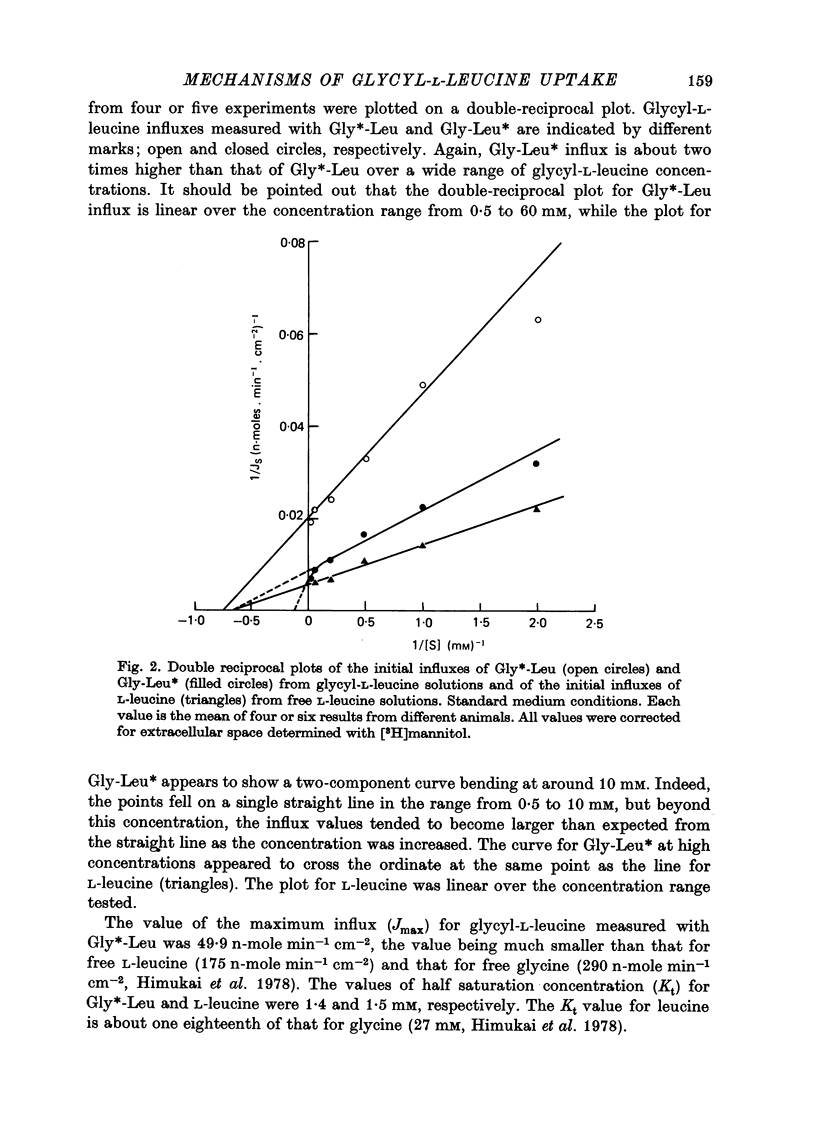
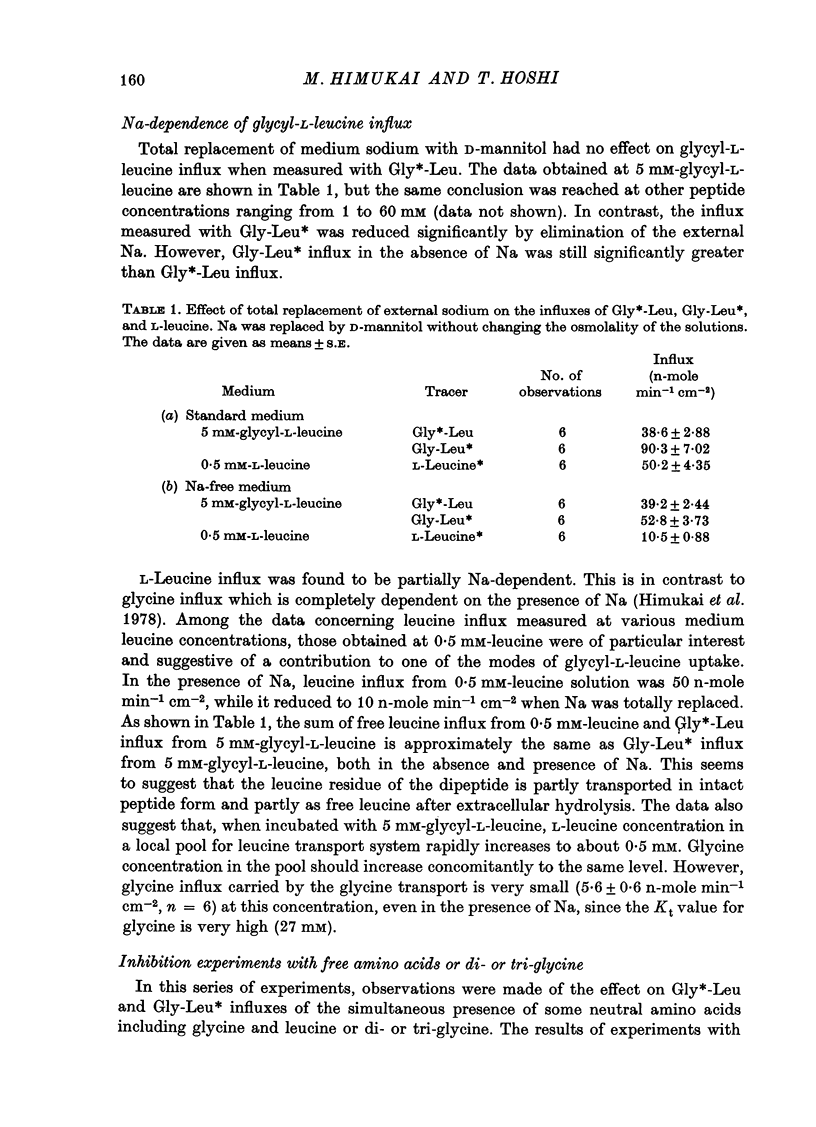
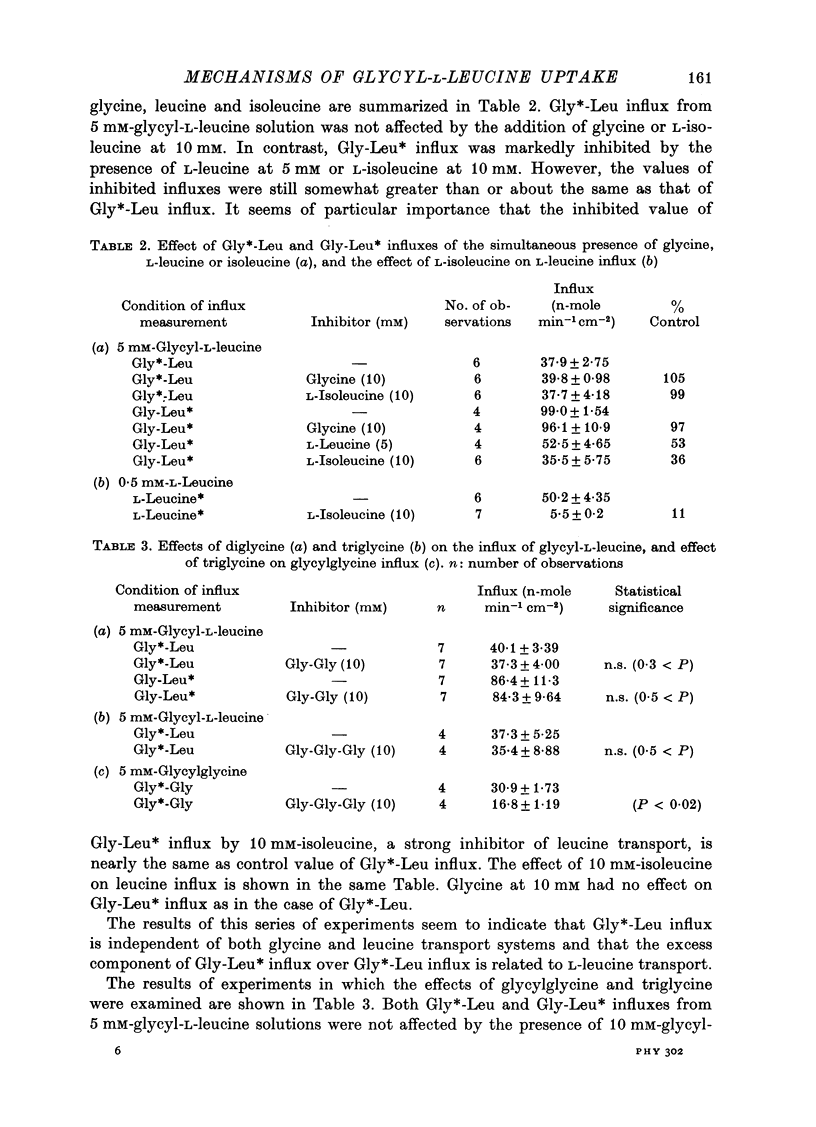
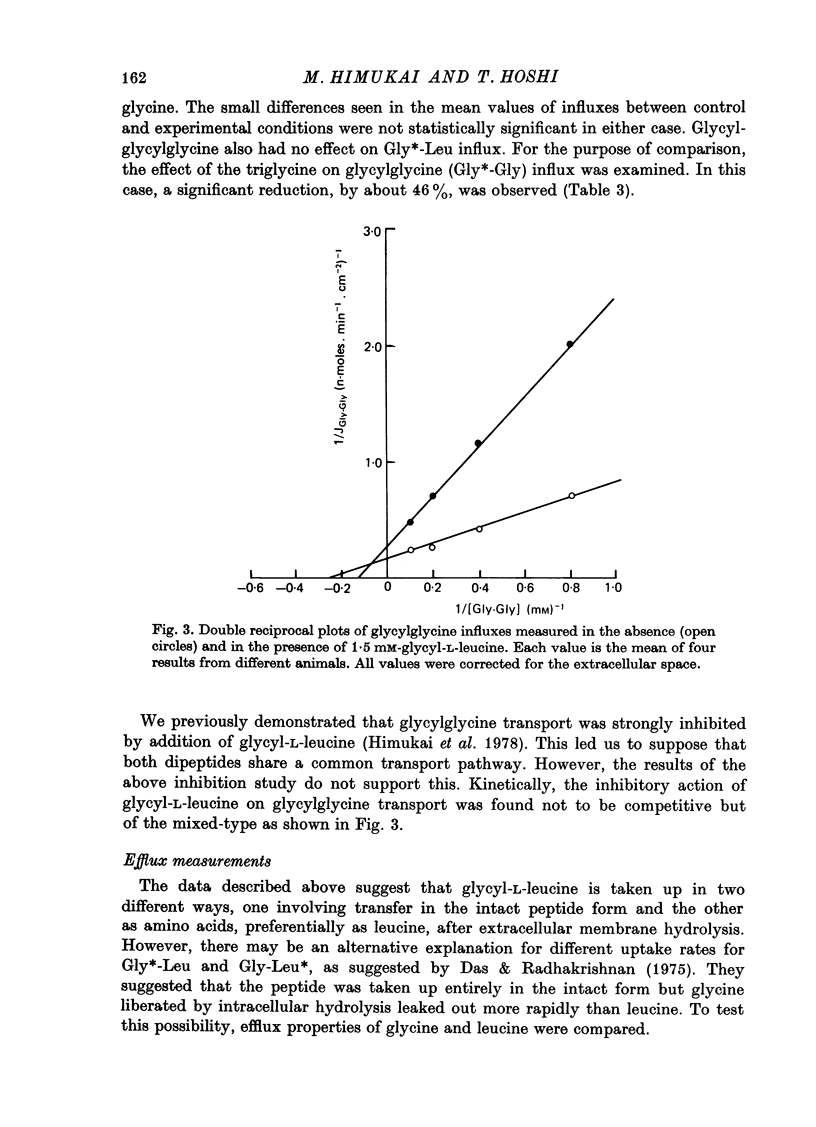
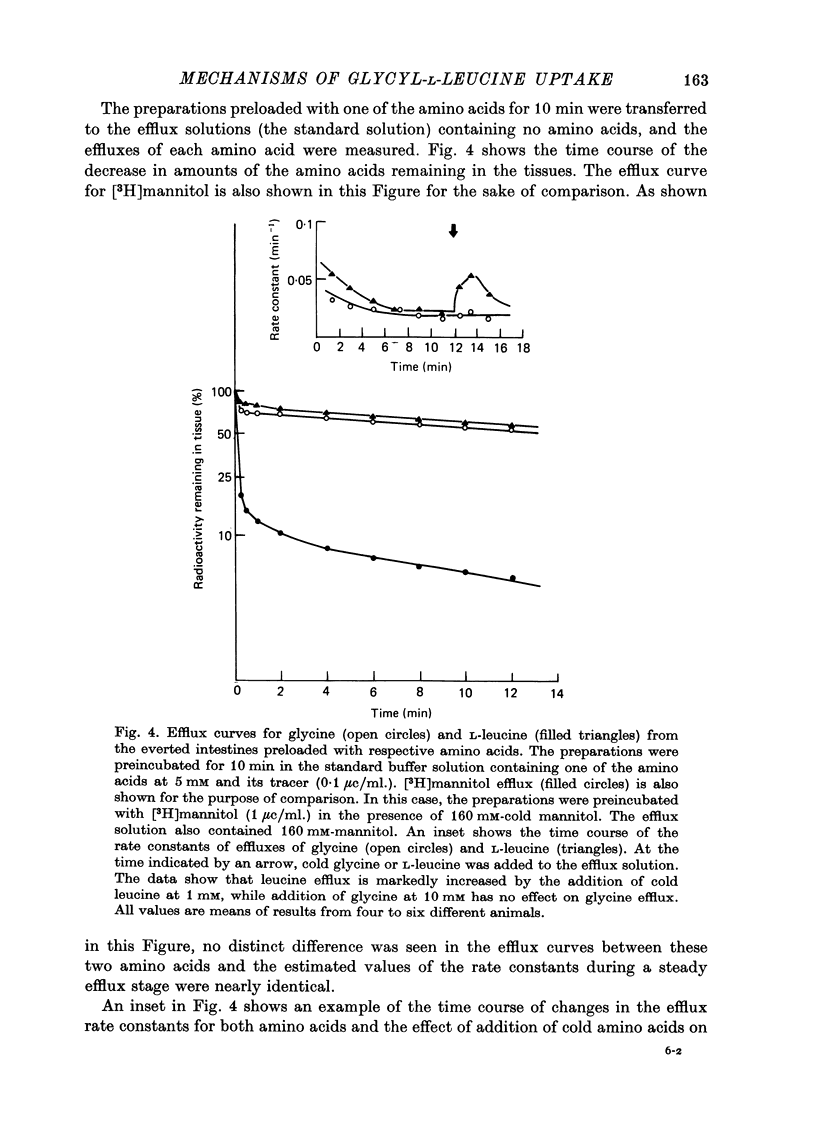
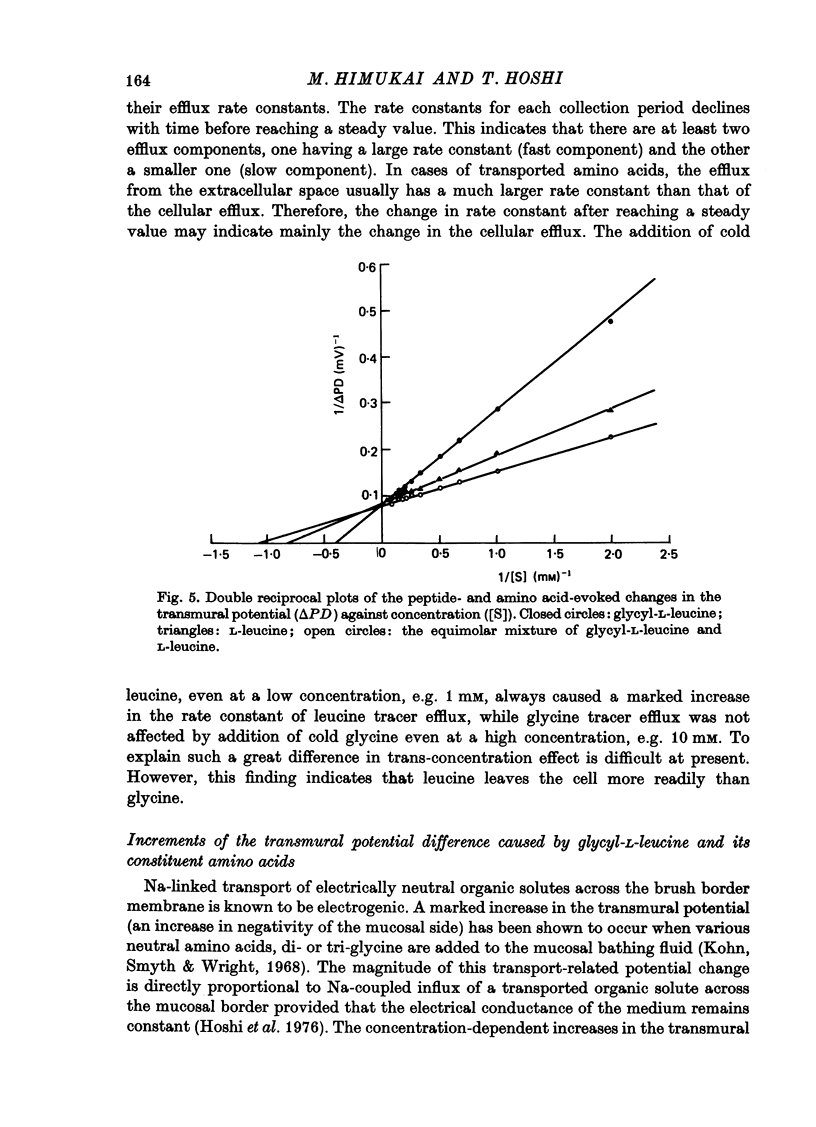
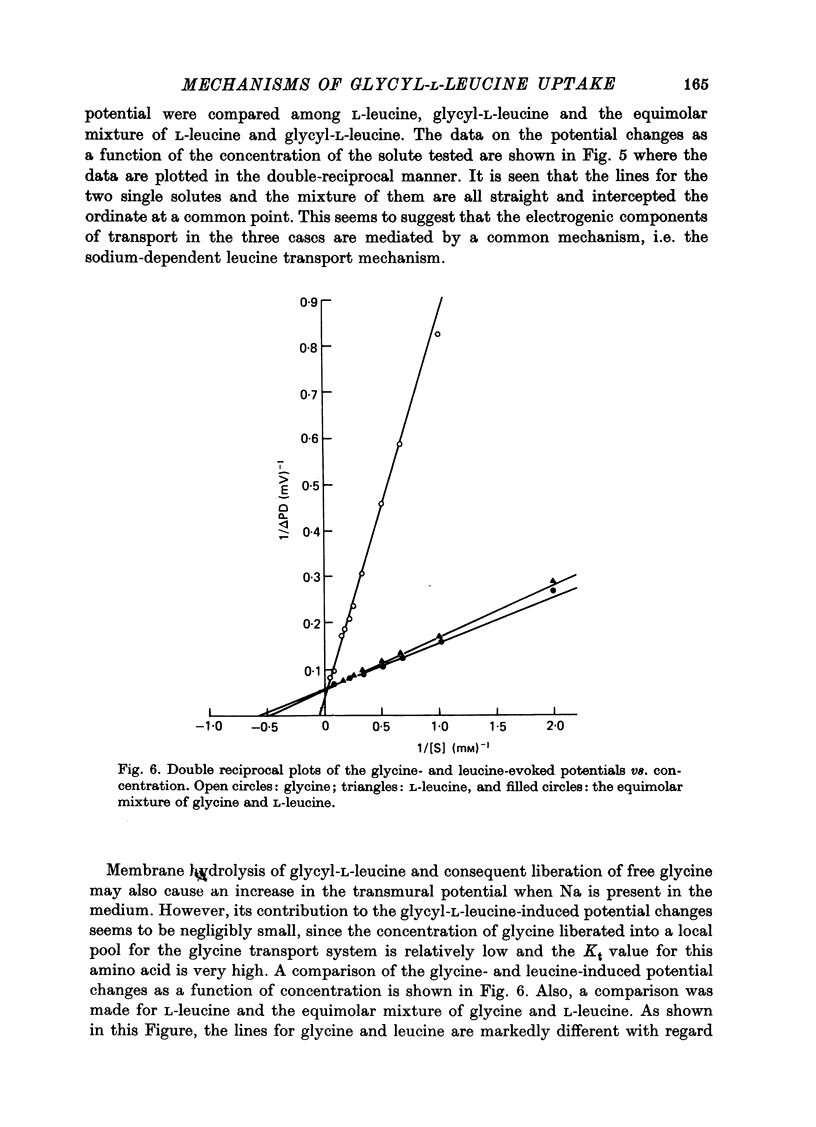
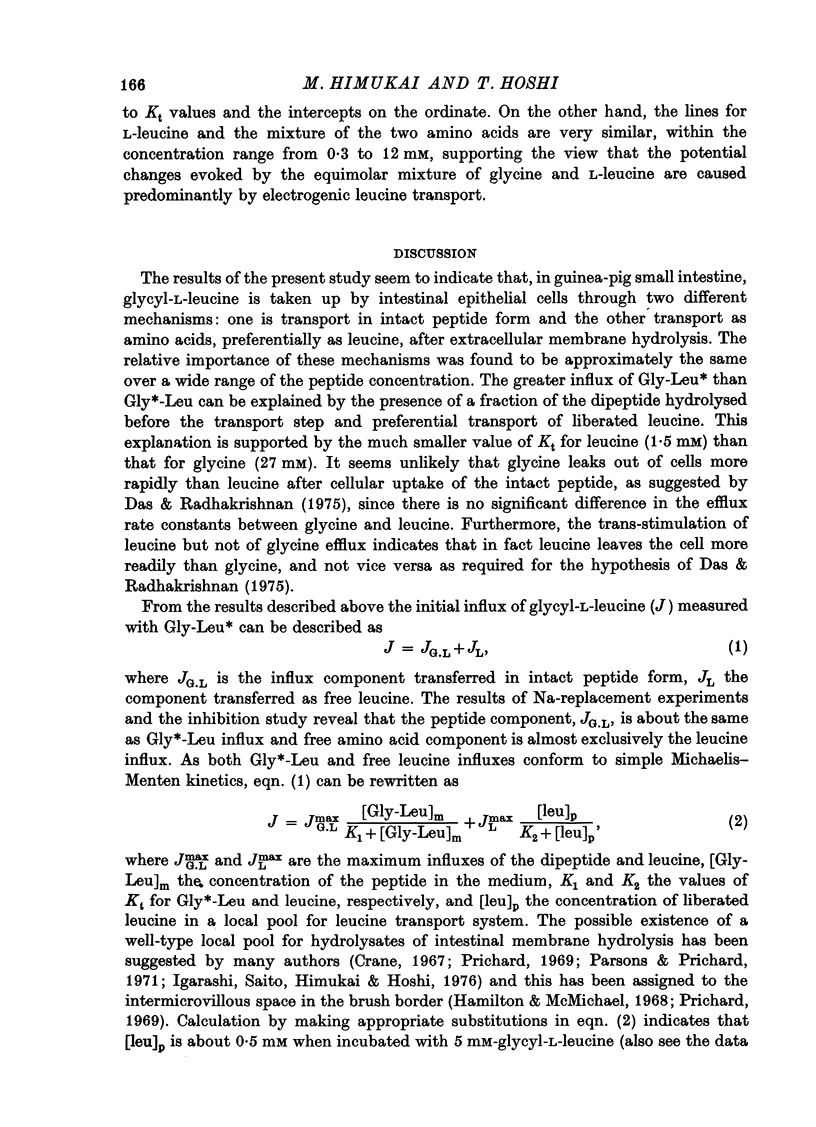
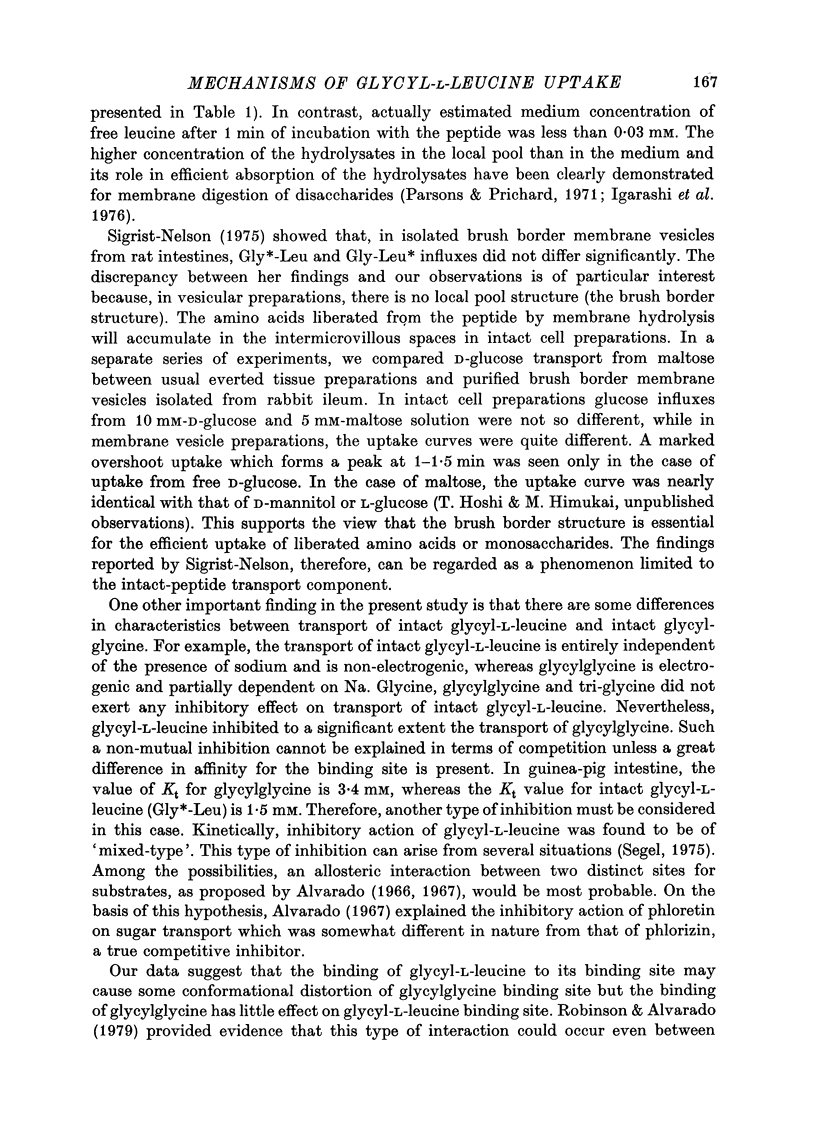
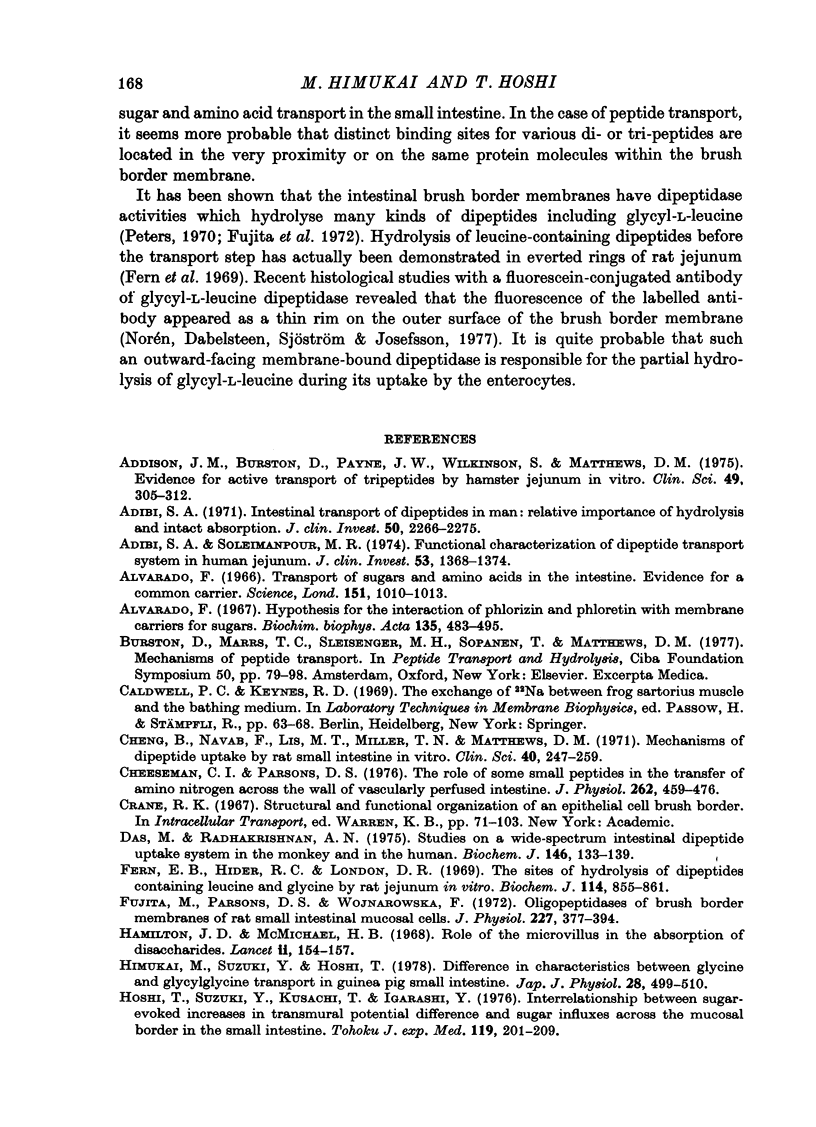
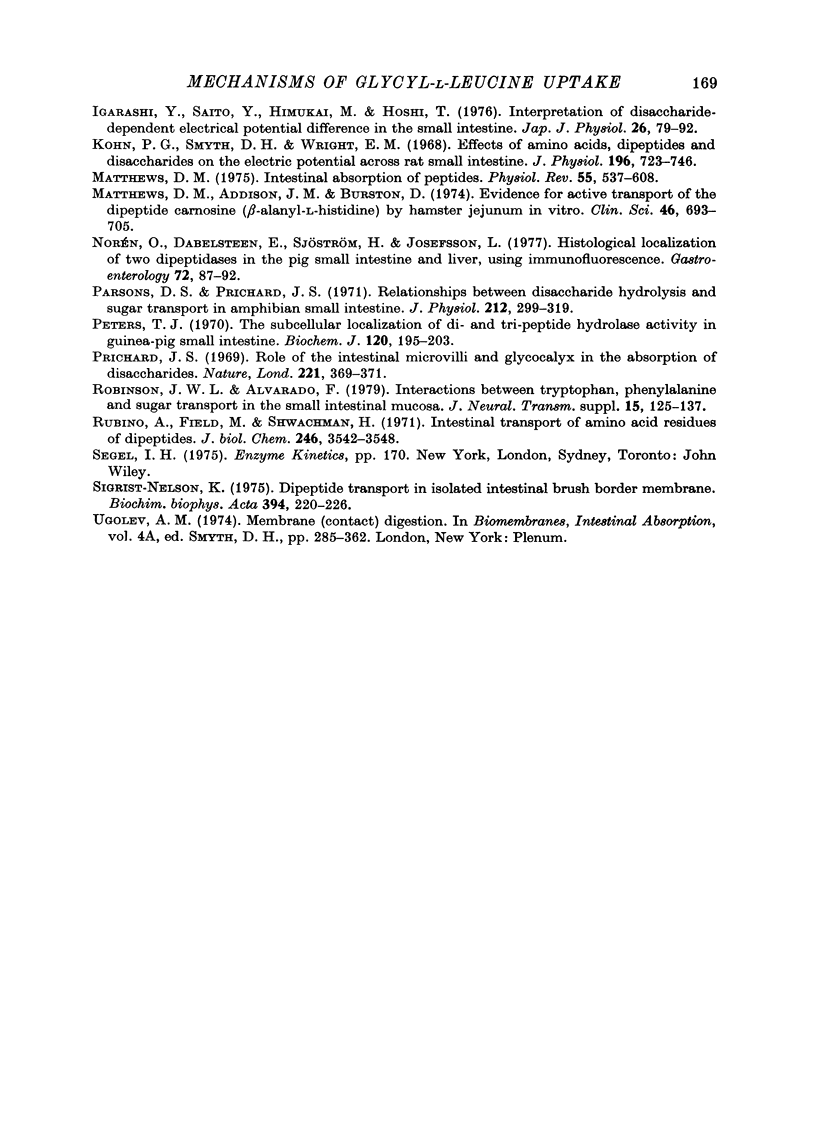
Selected References
These references are in PubMed. This may not be the complete list of references from this article.
- Addison J. M., Burston D., Payne J. W., Wilkinson S., Matthews D. M. Evidence for active transport of tripeptides by hamster jejunum in vitro. Clin Sci Mol Med. 1975 Oct;49(4):305–312. doi: 10.1042/cs0490305. [DOI] [PubMed] [Google Scholar]
- Adibi S. A. Intestinal transport of dipeptides in man: relative importance of hydrolysis and intact absorption. J Clin Invest. 1971 Nov;50(11):2266–2275. doi: 10.1172/JCI106724. [DOI] [PMC free article] [PubMed] [Google Scholar]
- Adibi S. A., Soleimanpour M. R. Functional characterization of dipeptide transport system in human jejunum. J Clin Invest. 1974 May;53(5):1368–1374. doi: 10.1172/JCI107685. [DOI] [PMC free article] [PubMed] [Google Scholar]
- Alvarado F. Hypothesis for the interaction of phlorizin and phloretin with membrane carriers for sugars. Biochim Biophys Acta. 1967 Jul 3;135(3):483–495. doi: 10.1016/0005-2736(67)90038-7. [DOI] [PubMed] [Google Scholar]
- Alvarado F. Transport of sugars and amino acids in the intestine: evidence for a common carrier. Science. 1966 Feb 25;151(3713):1010–1013. doi: 10.1126/science.151.3713.1010. [DOI] [PubMed] [Google Scholar]
- Cheeseman C. I., Parsons D. S. The role of some small peptides in the transfer of amino nitrogen across the wall of vascularly perfused intestine. J Physiol. 1976 Nov;262(2):459–476. doi: 10.1113/jphysiol.1976.sp011605. [DOI] [PMC free article] [PubMed] [Google Scholar]
- Cheng B., Navab F., Lis M. T., Miller T. N., Matthews D. M. Mechanisms of dipeptide uptake by rat small intestine in vitro. Clin Sci. 1971 Mar;40(3):247–259. doi: 10.1042/cs0400247. [DOI] [PubMed] [Google Scholar]
- Das M., Radhakrishnan A. N. Studies on a wide-spectrum intestinal dipeptide uptake system in the monkey and in the human. Biochem J. 1975 Jan;146(1):133–139. doi: 10.1042/bj1460133. [DOI] [PMC free article] [PubMed] [Google Scholar]
- Fern E. B., Hider R. C., London D. R. The sites of hydrolysis of dipeptides containing leucine and glycine by rat jejunum in vitro. Biochem J. 1969 Oct;114(4):855–861. doi: 10.1042/bj1140855. [DOI] [PMC free article] [PubMed] [Google Scholar]
- Fujita M., Parsons D. S., Wojnarowska F. Oligopeptidases of brush border membranes of rat small intestinal mucosal cells. J Physiol. 1972 Dec;227(2):377–394. doi: 10.1113/jphysiol.1972.sp010038. [DOI] [PMC free article] [PubMed] [Google Scholar]
- Hamilton J. D., McMichael H. B. Role of the microvillus in the absorption of disaccharides. Lancet. 1968 Jul 20;2(7560):154–157. doi: 10.1016/s0140-6736(68)90429-7. [DOI] [PubMed] [Google Scholar]
- Hoshi T., Suzuki Y., Kusachi T., Igarashi Y. Interrelationship between sugar-evoked increases in transmural potential difference and sugar influxes across the mucosal border in the small intestine. Tohoku J Exp Med. 1976 Jul;119(3):201–209. doi: 10.1620/tjem.119.201. [DOI] [PubMed] [Google Scholar]
- Igarashi Y., Saito Y., Himukai M., Hoshi T. Interpretation of disaccharide-dependent electrical potential differences in the small intestine. Jpn J Physiol. 1976;26(1):79–92. doi: 10.2170/jjphysiol.26.79. [DOI] [PubMed] [Google Scholar]
- Kohn P. G., Smyth D. H., Wright E. M. Effects of amino acids, dipeptides and disaccharides on the electric potential across rat small intestine. J Physiol. 1968 Jun;196(3):723–746. doi: 10.1113/jphysiol.1968.sp008533. [DOI] [PMC free article] [PubMed] [Google Scholar]
- Matthews D. M., Addison J. M., Burston D. Evidence for active transport of the dipeptide carnosine (beta-alanyl-L-histidine) by hamster jejunum in vitro. Clin Sci Mol Med. 1974 Jun;46(6):693–705. doi: 10.1042/cs0460693. [DOI] [PubMed] [Google Scholar]
- Matthews D. M. Intestinal absorption of peptides. Physiol Rev. 1975 Oct;55(4):537–608. doi: 10.1152/physrev.1975.55.4.537. [DOI] [PubMed] [Google Scholar]
- Norén O., Dabelsteen E., Sjöström H., Josefsson L. Histological localization of two dipeptidases in the pig small intestine and liver, using immunofluorescence. Gastroenterology. 1977 Jan;72(1):87–92. [PubMed] [Google Scholar]
- Parson D. S., Prichard J. S. Relationships between disaccharide hydrolysis and sugar transport in amphibian small intestine. J Physiol. 1971 Jan;212(2):299–319. doi: 10.1113/jphysiol.1971.sp009326. [DOI] [PMC free article] [PubMed] [Google Scholar]
- Peters T. J. The subcellular localization of di- and tri-peptide hydrolase activity in guinea-pig small intestine. Biochem J. 1970 Nov;120(1):195–203. doi: 10.1042/bj1200195. [DOI] [PMC free article] [PubMed] [Google Scholar]
- Prichard J. S. Role of the intestinal microvilli and glycocalyx in the absorption of disaccharides. Nature. 1969 Jan 25;221(5178):369–371. doi: 10.1038/221369a0. [DOI] [PubMed] [Google Scholar]
- Robinson J. W., Alvarado F. Interactions between tryptophan, phenylalanine and sugar transport in the small intestinal mucosa. J Neural Transm Suppl. 1979;(15):125–137. doi: 10.1007/978-3-7091-2243-3_11. [DOI] [PubMed] [Google Scholar]
- Rubino A., Field M., Shwachman H. Intestinal transport of amino acid residues of dipeptides. I. Influx of the glycine residue of glycyl-L-proline across mucosal border. J Biol Chem. 1971 Jun 10;246(11):3542–3548. [PubMed] [Google Scholar]
- Sigrist-Nelson K. Dipeptide transport in isolated intestinal brush border membrane. Biochim Biophys Acta. 1975 Jun 25;394(2):220–226. doi: 10.1016/0005-2736(75)90260-6. [DOI] [PubMed] [Google Scholar]
- Ugolev A. M. Membrane (contact) digestion. Biomembranes. 1974;4A(0):285–362. [PubMed] [Google Scholar]