Abstract
1. In the isolated frog spinal cord repetitive stimulation of a lumbar dorsal root produced a sustained negative potential recorded from an adjacent inactive dorsal root by sucrose gap techniques. This negative potential was followed by a positive potential, an indication that the dorsal root terminals were hyperpolarized. Increasing the duration of the tetanus applied to the active root increased the amplitude and duration of the after-hyperpolarization which could be up to 6 mV and 3 min respectively. 2. The hyperpolarization presumably reflected an increased rate of active sodium pumping. Since it was reversibly reduced by metabolic inhibitors (dinitrophenol, NaCN) and cooling (Q10, 2 . 6) it was clearly dependent upon intact metabolic activity. In addition, a variety of procedures used to inhibit sodium pumps (including application of ouabain, elimination of potassium from the superfusate, and partial substitution of lithium for sodium ions) significantly and reversibly decreased the potential. 3. The hyperpolarization was not dependent upon intact chemical synaptic transmission since it could survive prolonged immersion of the cord in Ringer solution containing manganese or magnesium ions. 4. It is suggested that the hyperpolarization of inactive fibres resulted from a decreased extracellular potassium concentration in the dorsal horn produced as a result of a pumping mechanism which extruded sodium and transported potassium inwards by dorsal root fibres directly activated by the tetanus.
Full text
PDF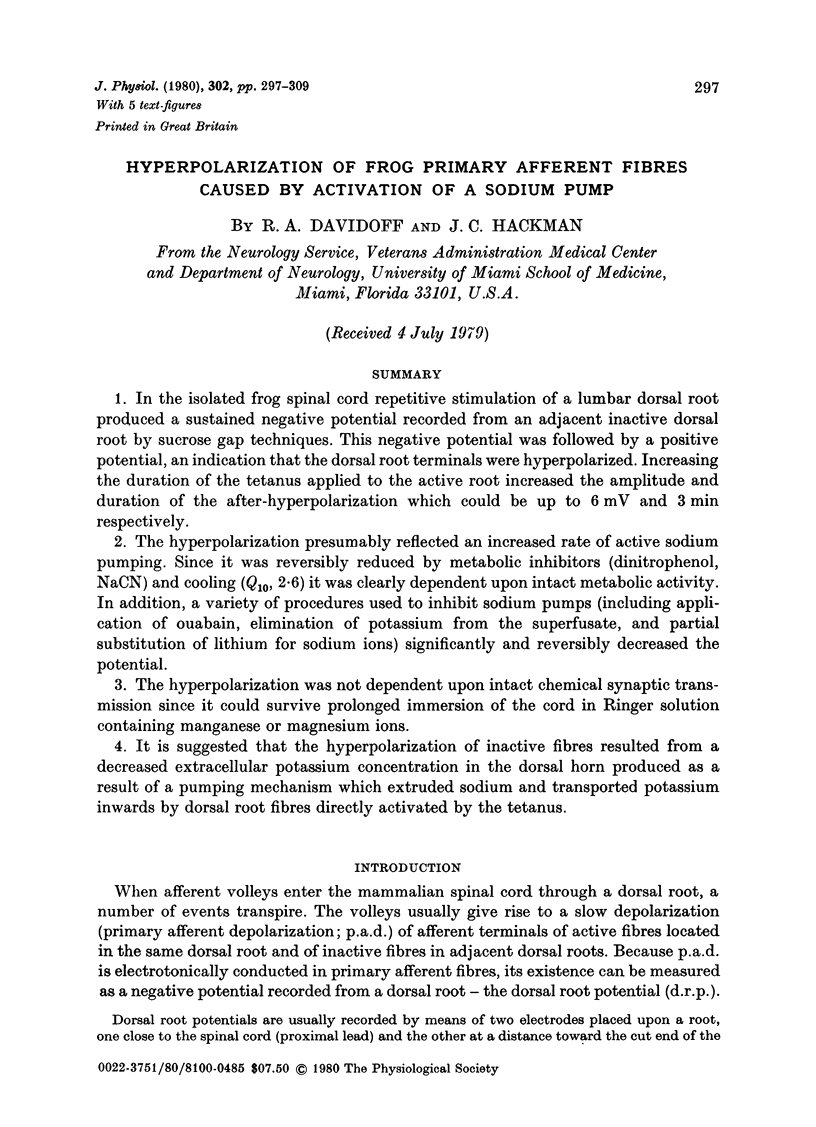
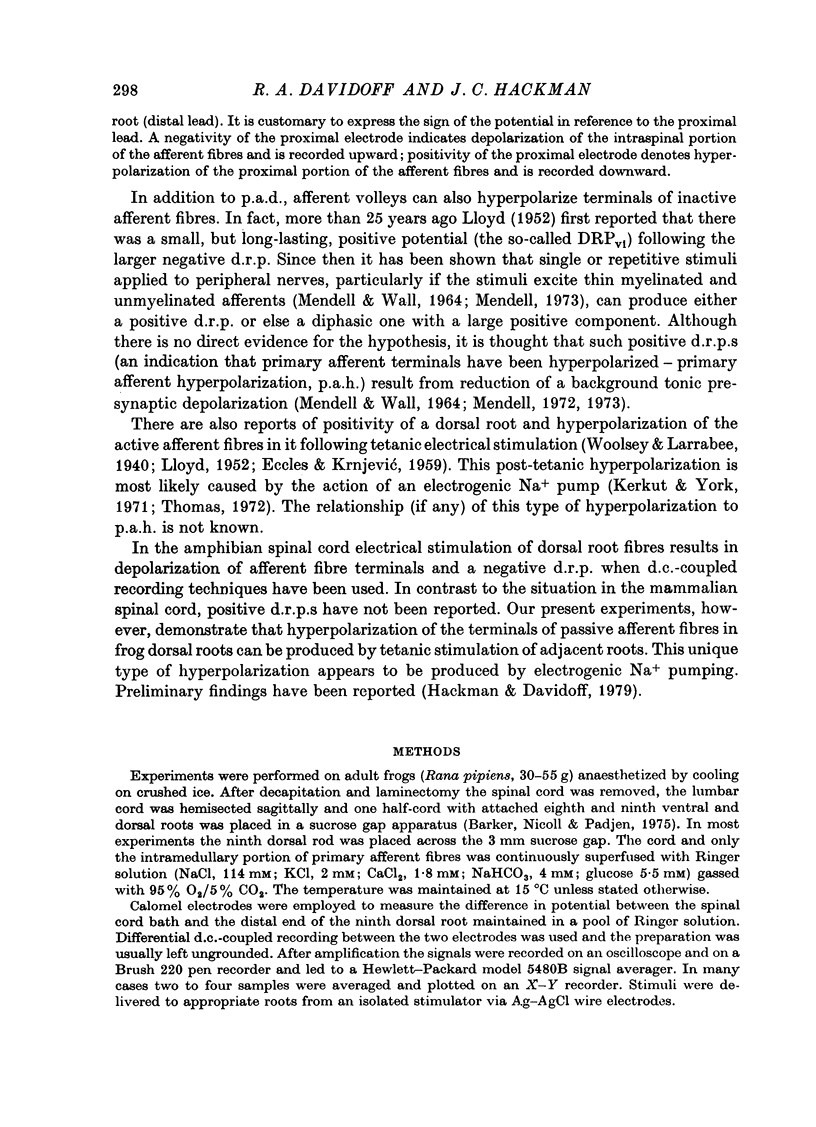
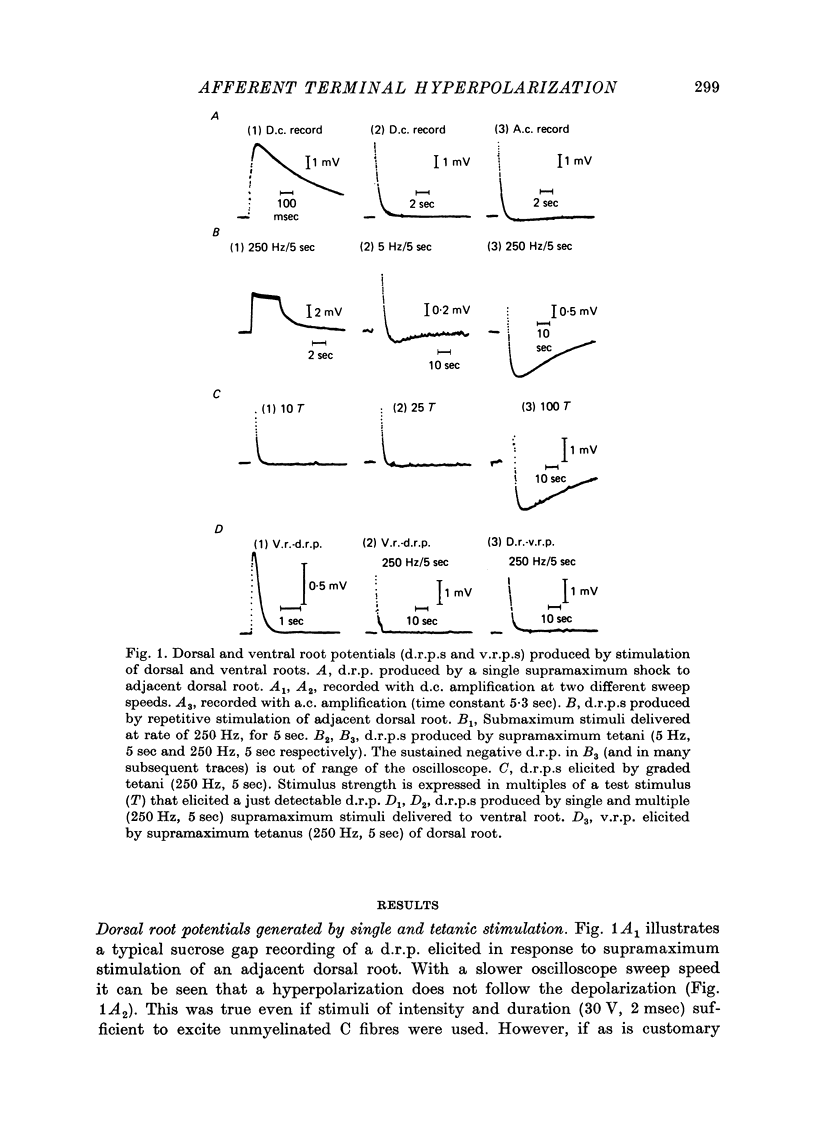
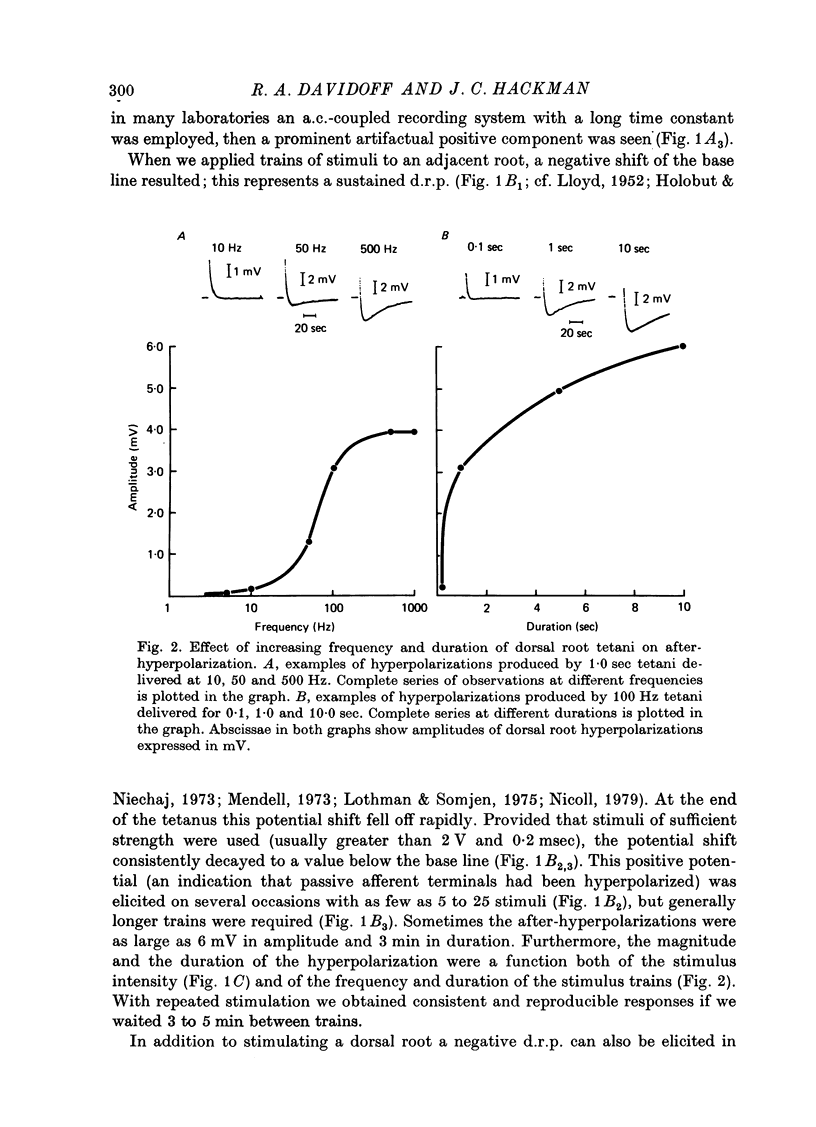
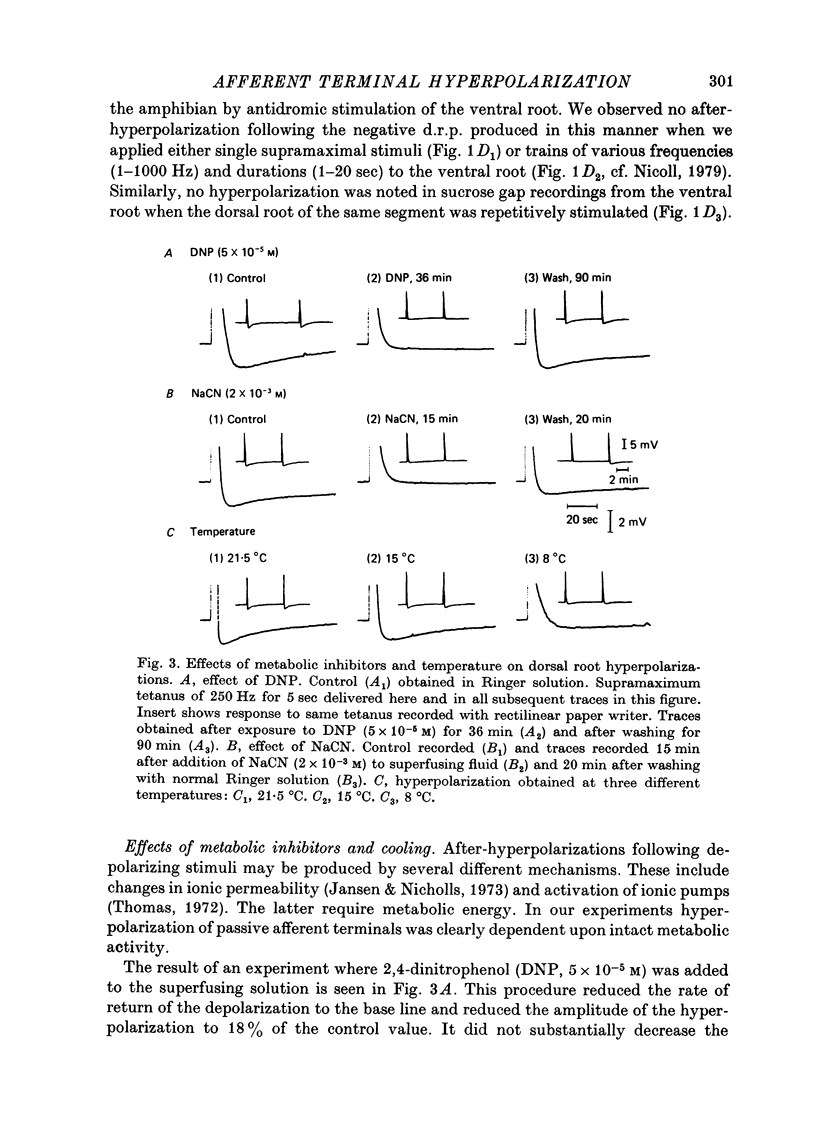
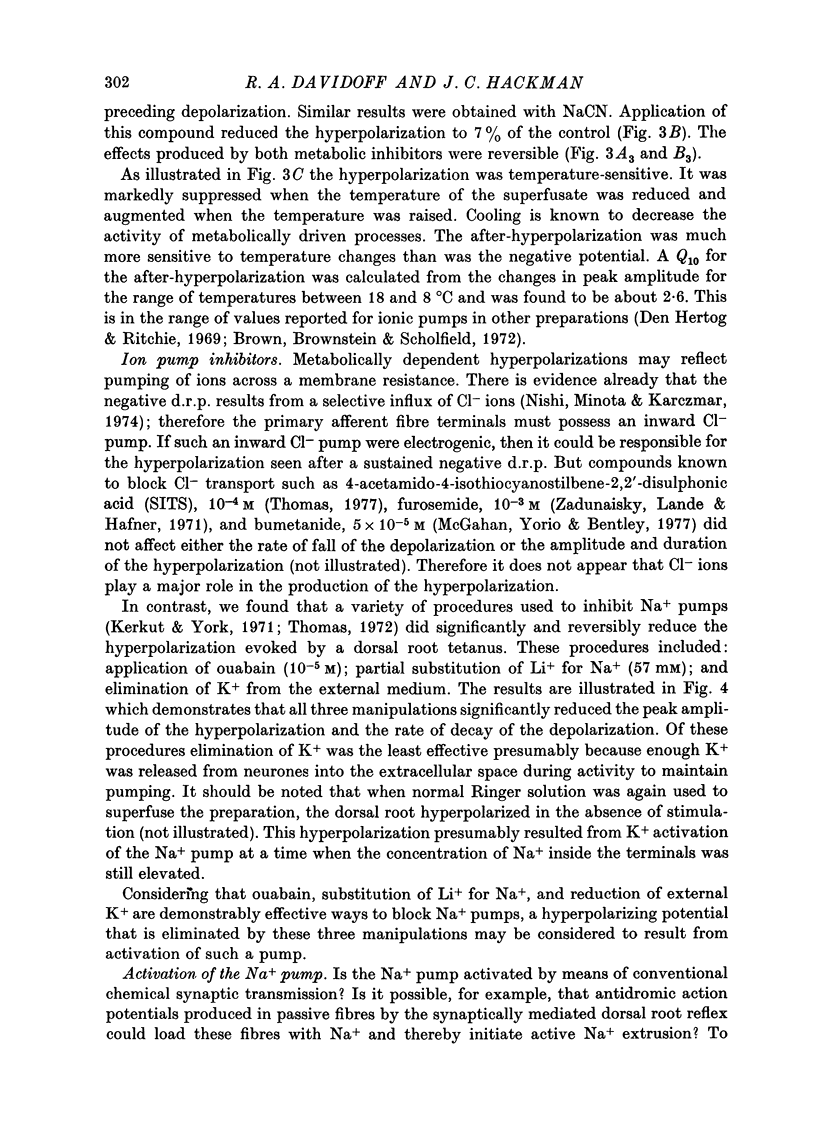
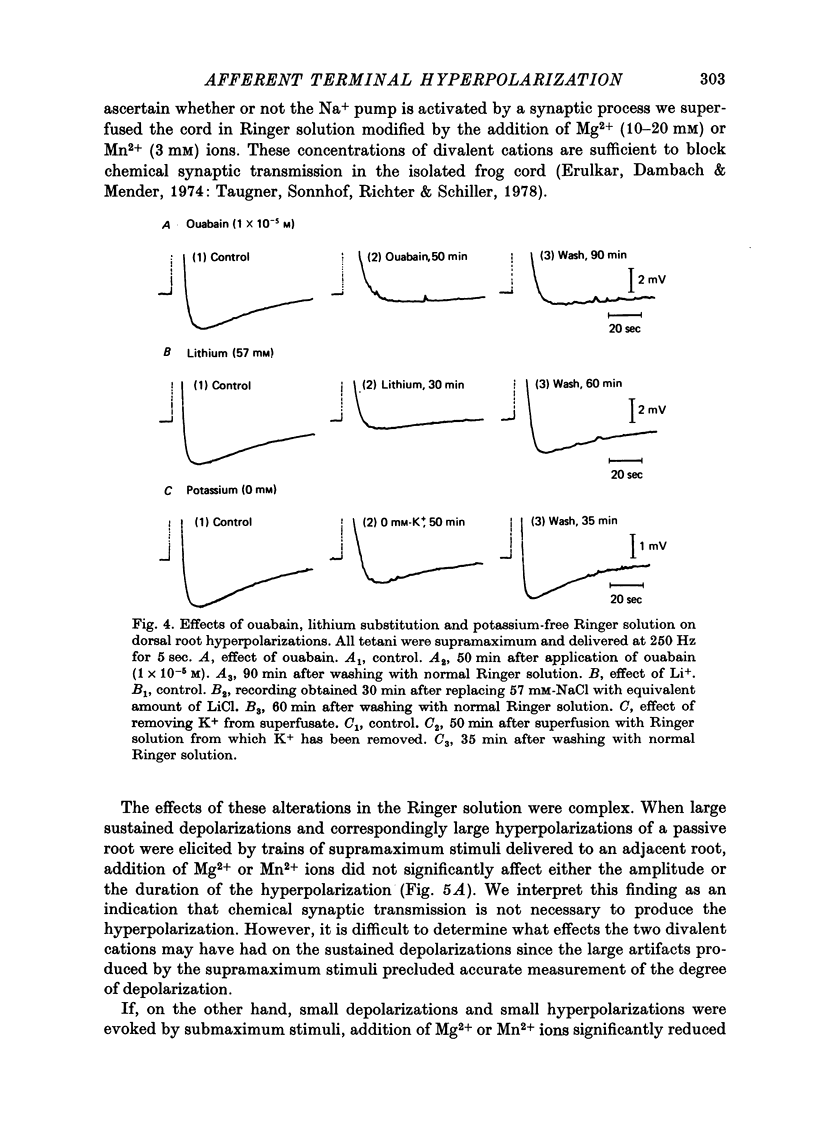
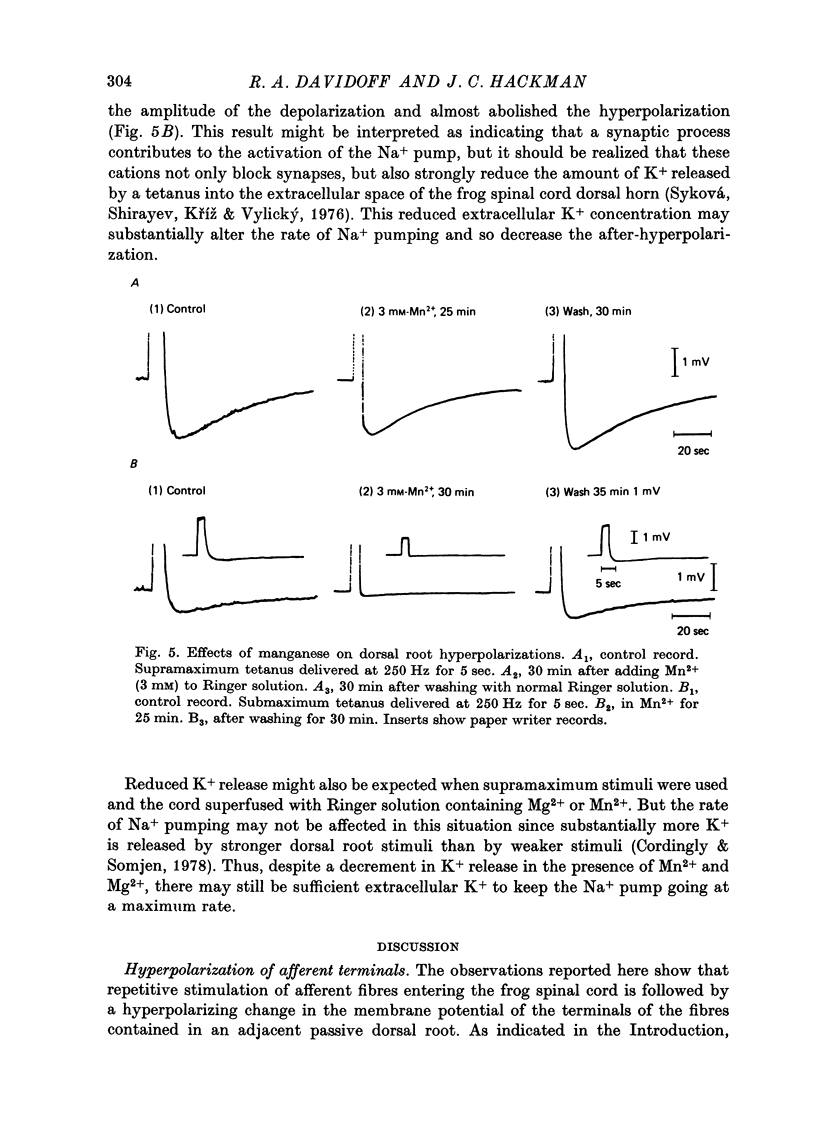
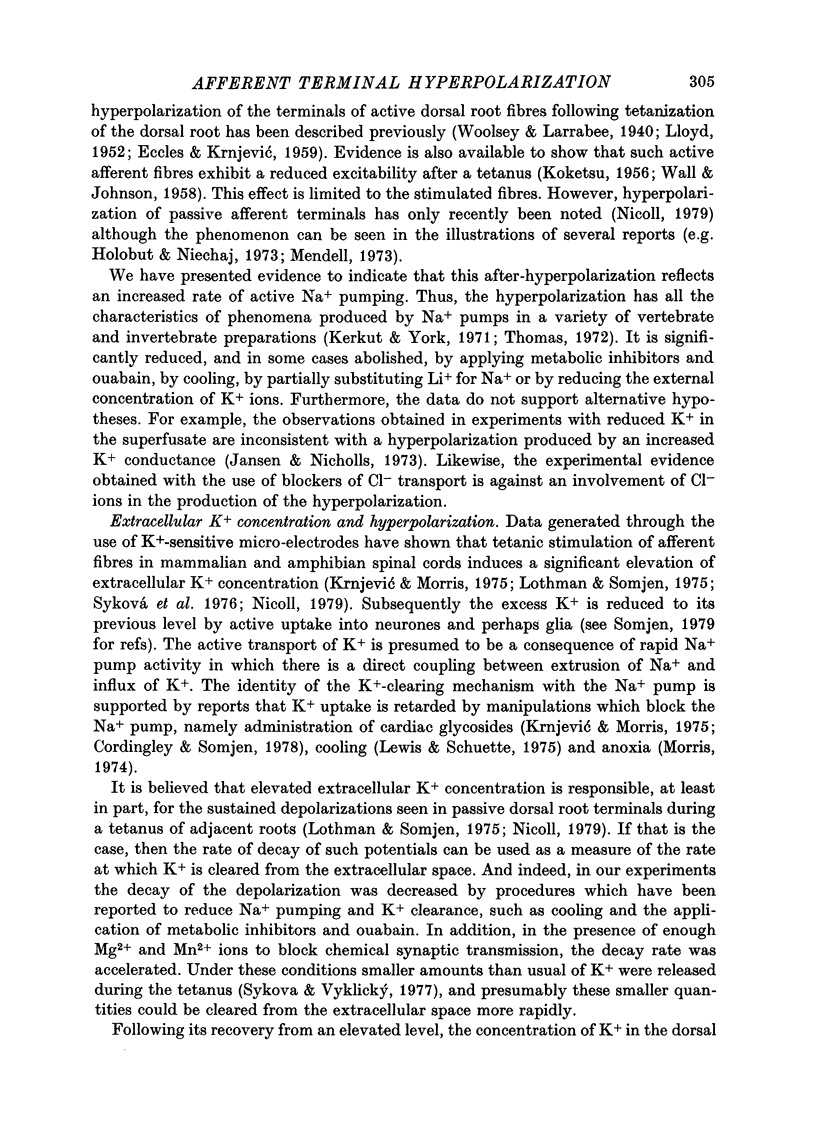
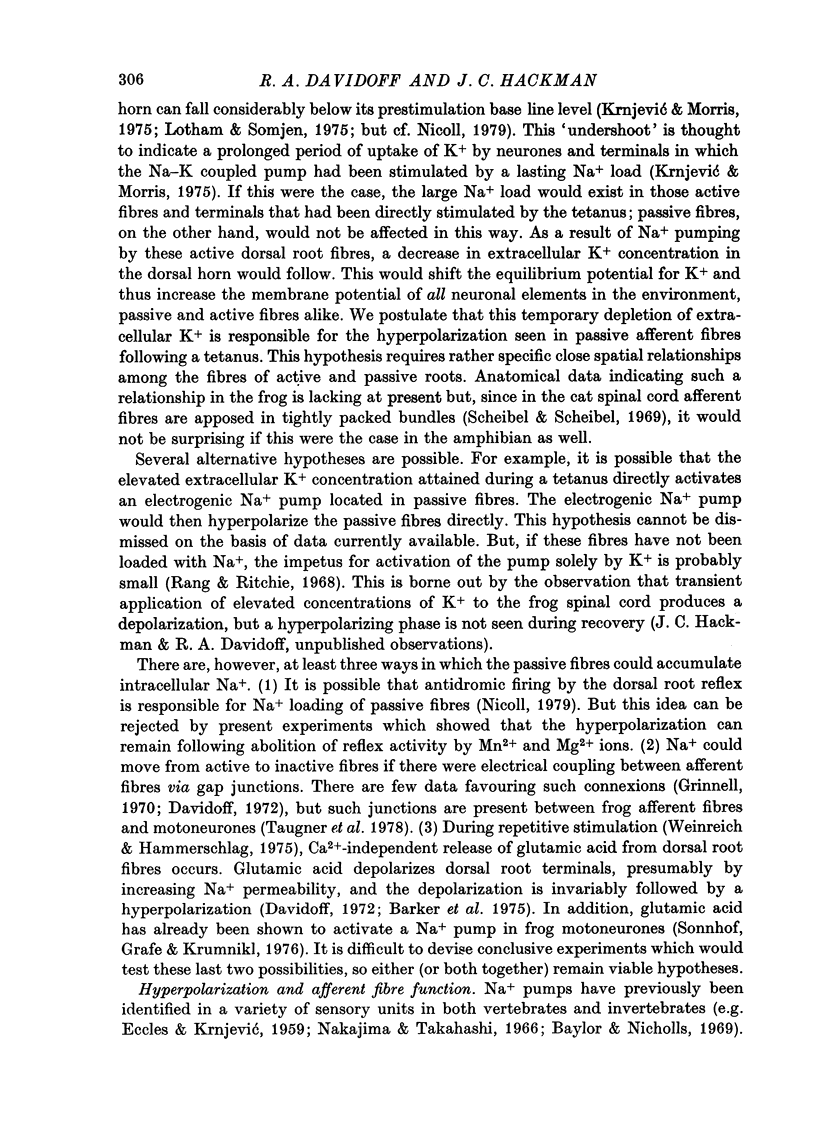
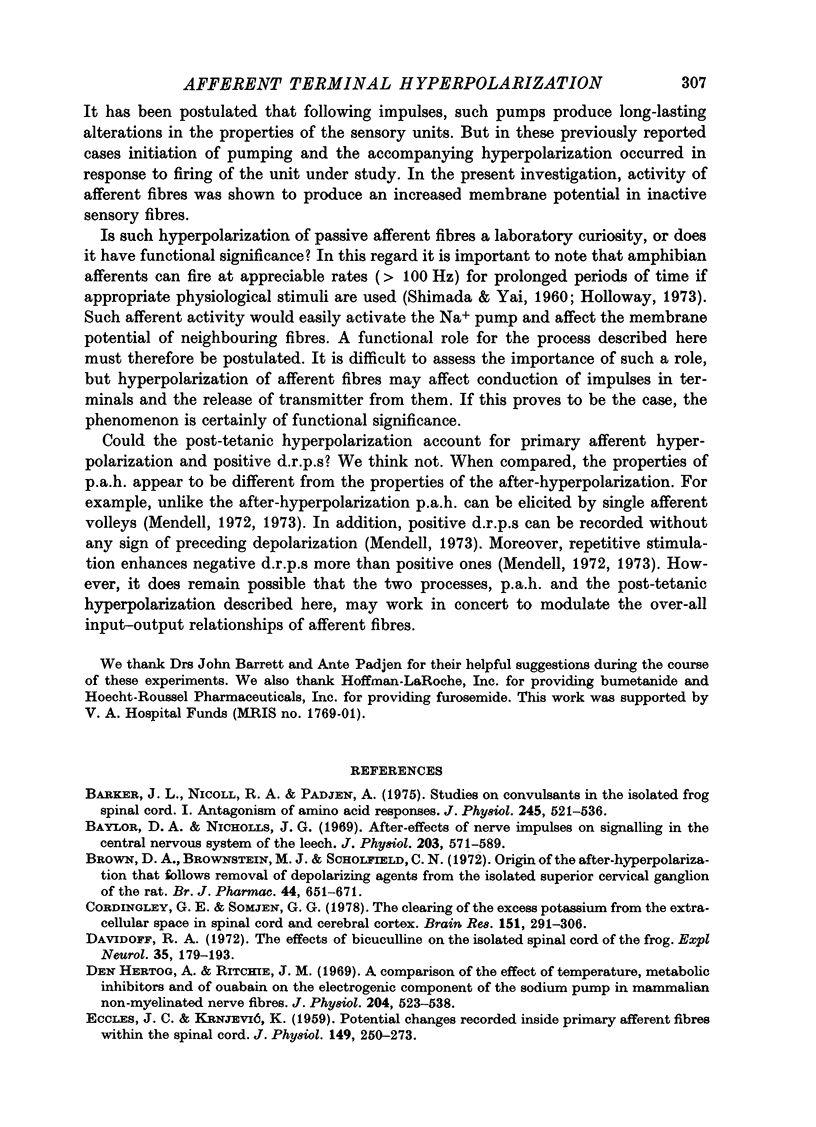
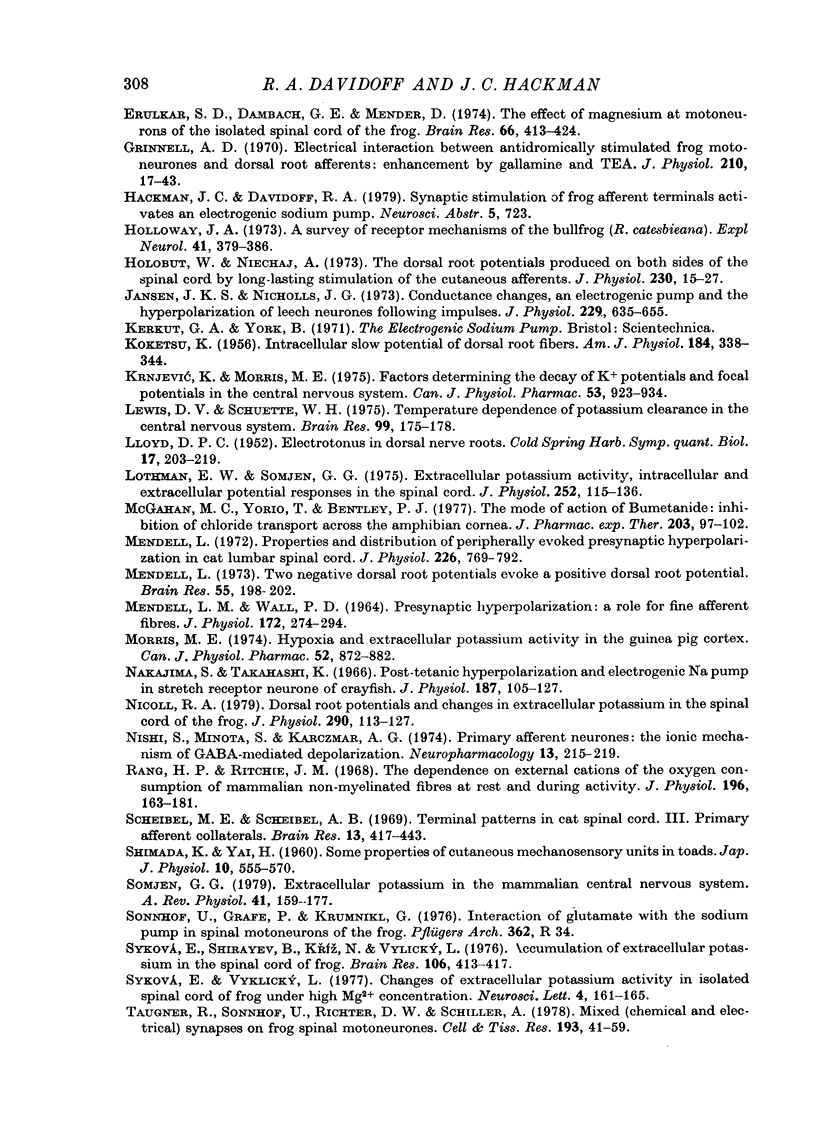
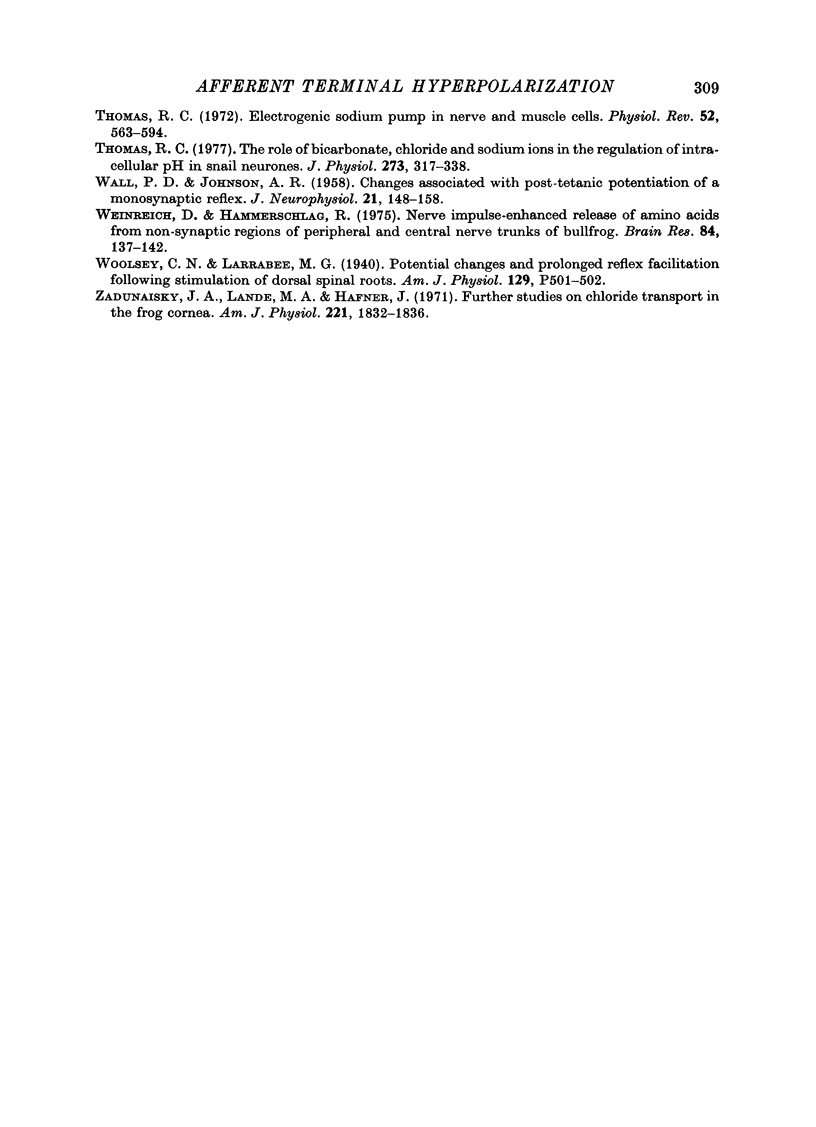
Selected References
These references are in PubMed. This may not be the complete list of references from this article.
- Barker J. L., Nicoll R. A., Padjen A. Studies on convulsants in the isolated frog spinal cord. I. Antagonism of amino acid responses. J Physiol. 1975 Mar;245(3):521–536. doi: 10.1113/jphysiol.1975.sp010859. [DOI] [PMC free article] [PubMed] [Google Scholar]
- Baylor D. A., Nicholls J. G. After-effects of nerve impulses on signalling in the central nervous system of the leech. J Physiol. 1969 Aug;203(3):571–589. doi: 10.1113/jphysiol.1969.sp008880. [DOI] [PMC free article] [PubMed] [Google Scholar]
- Brown D. A., Brownstein M. J., Scholfield C. N. Origin of the after-hyperpolarization that follows removal of depolarizing agents from the isolated superior cervical ganglion of the rat. Br J Pharmacol. 1972 Apr;44(4):651–671. doi: 10.1111/j.1476-5381.1972.tb07305.x. [DOI] [PMC free article] [PubMed] [Google Scholar]
- Cordingley G. E., Somjen G. G. The clearing of excess potassium from extracellular space in spinal cord and cerebral cortex. Brain Res. 1978 Aug 4;151(2):291–306. doi: 10.1016/0006-8993(78)90886-7. [DOI] [PubMed] [Google Scholar]
- Davidoff R. A. The effects of bicuculline on the isolated spinal cord of the frog. Exp Neurol. 1972 Apr;35(1):179–193. doi: 10.1016/0014-4886(72)90068-4. [DOI] [PubMed] [Google Scholar]
- ECCLES J. C., KRNJEVIC K. Potential changes recorded inside primary afferent fibres within the spinal cord. J Physiol. 1959 Dec;149:250–273. doi: 10.1113/jphysiol.1959.sp006338. [DOI] [PMC free article] [PubMed] [Google Scholar]
- Grinnell A. D. Electrical interaction between antidromically stimulated frog motoneurones and dorsal root afferents: enhancement by gallamine and TEA. J Physiol. 1970 Sep;210(1):17–43. doi: 10.1113/jphysiol.1970.sp009194. [DOI] [PMC free article] [PubMed] [Google Scholar]
- Holloway J. A. A survey of receptor mechanisms of the bullfrog. (R. catesbieana). Exp Neurol. 1973 Nov;41(2):379–386. doi: 10.1016/0014-4886(73)90279-3. [DOI] [PubMed] [Google Scholar]
- Holobut W., Niechaj A. The dorsal root potentials produced on both sides of the spinal cord by long-lasting stimulation of the cutaneous afferents. J Physiol. 1973 Apr;230(1):15–27. doi: 10.1113/jphysiol.1973.sp010172. [DOI] [PMC free article] [PubMed] [Google Scholar]
- Jansen J. K., Nicholls J. G. Conductance changes, an electrogenic pump and the hyperpolarization of leech neurones following impulses. J Physiol. 1973 Mar;229(3):635–655. doi: 10.1113/jphysiol.1973.sp010158. [DOI] [PMC free article] [PubMed] [Google Scholar]
- KOKETSU K. Intracellular slow potential of dorsal root fibers. Am J Physiol. 1956 Feb;184(2):338–344. doi: 10.1152/ajplegacy.1956.184.2.338. [DOI] [PubMed] [Google Scholar]
- Krnjević K., Morris M. E. Factors determining the decay of K+ potentials and focal potentials in the central nervous system. Can J Physiol Pharmacol. 1975 Oct;53(5):923–934. doi: 10.1139/y75-126. [DOI] [PubMed] [Google Scholar]
- LLOYD D. P. C. Electrotonus in dorsal nerve roots. Cold Spring Harb Symp Quant Biol. 1952;17:203–219. doi: 10.1101/sqb.1952.017.01.020. [DOI] [PubMed] [Google Scholar]
- Lewis D. V., Schuette W. H. Temperature dependence of potassium clearance in the central nervous system. Brain Res. 1975 Nov 28;99(1):175–178. doi: 10.1016/0006-8993(75)90623-x. [DOI] [PubMed] [Google Scholar]
- Lothman E. W., Somjen G. G. Extracellular potassium activity, intracellular and extracellular potential responses in the spinal cord. J Physiol. 1975 Oct;252(1):115–136. doi: 10.1113/jphysiol.1975.sp011137. [DOI] [PMC free article] [PubMed] [Google Scholar]
- MENDELL L. M., WALL P. D. PRESYNAPTIC HYPERPOLARIZATION: A ROLE FOR FINE AFFERENT FIBRES. J Physiol. 1964 Aug;172:274–294. doi: 10.1113/jphysiol.1964.sp007417. [DOI] [PMC free article] [PubMed] [Google Scholar]
- McGahan M. C., Yorio T., Bentley P. J. The mode of action of bumetanide: inhibition of chloride transport across the amphibian cornea. J Pharmacol Exp Ther. 1977 Oct;203(1):97–102. [PubMed] [Google Scholar]
- Mendell L. Properties and distribution of peripherally evoked presynaptic hyperpolarization in cat lumbar spinal cord. J Physiol. 1972 Nov;226(3):769–792. doi: 10.1113/jphysiol.1972.sp010009. [DOI] [PMC free article] [PubMed] [Google Scholar]
- Mendell L. Two negative dorsal root potentials evoke a positive dorsal root potential. Brain Res. 1973 May 30;55(1):198–202. doi: 10.1016/0006-8993(73)90501-5. [DOI] [PubMed] [Google Scholar]
- Morris M. E. Hypoxia and extracellular potassium activity in the guinea-pig cortex. Can J Physiol Pharmacol. 1974 Aug;52(4):872–882. doi: 10.1139/y74-111. [DOI] [PubMed] [Google Scholar]
- Nakajima S., Takahashi K. Post-tetanic hyperpolarization and electrogenic Na pump in stretch receptor neurone of crayfish. J Physiol. 1966 Nov;187(1):105–127. doi: 10.1113/jphysiol.1966.sp008078. [DOI] [PMC free article] [PubMed] [Google Scholar]
- Nicoll R. A. Dorsal root potentials and changes in extracellular potassium in the spinal cord of the frog. J Physiol. 1979 May;290(2):113–127. doi: 10.1113/jphysiol.1979.sp012763. [DOI] [PMC free article] [PubMed] [Google Scholar]
- Nishi S., Minota S., Karczmar A. G. Primary afferent neurones: the ionic mechanism of GABA-mediated depolarization. Neuropharmacology. 1974 Mar;13(3):215–219. doi: 10.1016/0028-3908(74)90110-5. [DOI] [PubMed] [Google Scholar]
- Rang H. P., Ritchie J. M. The dependence on external cations of the oxygen consumption of mammalian non-myelinated fibres at rest and during activity. J Physiol. 1968 May;196(1):163–181. doi: 10.1113/jphysiol.1968.sp008501. [DOI] [PMC free article] [PubMed] [Google Scholar]
- Scheibel M. E., Scheibel A. B. Terminal patterns in cat spinal cord. 3. Primary afferent collaterals. Brain Res. 1969 May;13(3):417–443. doi: 10.1016/0006-8993(69)90258-3. [DOI] [PubMed] [Google Scholar]
- Somjen G. G. Extracellular potassium in the mammalian central nervous system. Annu Rev Physiol. 1979;41:159–177. doi: 10.1146/annurev.ph.41.030179.001111. [DOI] [PubMed] [Google Scholar]
- Syková E., Shirayev B., Kríz N., Vyklický L. Accumulation of extracellular potassium in the spinal cord of frog. Brain Res. 1976 Apr 23;106(2):413–417. doi: 10.1016/0006-8993(76)91039-8. [DOI] [PubMed] [Google Scholar]
- Taugner R., Sonnhof U., Richter D. W., Schiller A. Mixed (chemical and electrical) synapses on frog spinal motoneurons. Cell Tissue Res. 1978 Oct 6;193(1):41–59. doi: 10.1007/BF00221600. [DOI] [PubMed] [Google Scholar]
- Thomas R. C. Electrogenic sodium pump in nerve and muscle cells. Physiol Rev. 1972 Jul;52(3):563–594. doi: 10.1152/physrev.1972.52.3.563. [DOI] [PubMed] [Google Scholar]
- Thomas R. C. The role of bicarbonate, chloride and sodium ions in the regulation of intracellular pH in snail neurones. J Physiol. 1977 Dec;273(1):317–338. doi: 10.1113/jphysiol.1977.sp012096. [DOI] [PMC free article] [PubMed] [Google Scholar]
- WALL P. D., JOHNSON A. R. Changes associated with post-tetanic potentiation of a monosynaptic reflex. J Neurophysiol. 1958 Mar;21(2):148–158. doi: 10.1152/jn.1958.21.2.148. [DOI] [PubMed] [Google Scholar]
- Weinreich D., Hammerschlag R. Nerve impulse-enhanced release of amino acids from non-synaptic regions of peripheral and central nerve trunks of bullfrog. Brain Res. 1975 Jan 24;84(1):137–142. doi: 10.1016/0006-8993(75)90807-0. [DOI] [PubMed] [Google Scholar]
- Zadunaisky J. A., Lande M. A., Hafner J. Further studies on chloride transport in the frog cornea. Am J Physiol. 1971 Dec;221(6):1832–1836. doi: 10.1152/ajplegacy.1971.221.6.1832. [DOI] [PubMed] [Google Scholar]
- den Hertog A., Ritchie J. M. A comparison of the effect of temperature, metabolic inhibitors and of ouabain on the electrogenic componen of the sodium pump in mammalian non-myelinated nerve fibres. J Physiol. 1969 Oct;204(3):523–538. doi: 10.1113/jphysiol.1969.sp008929. [DOI] [PMC free article] [PubMed] [Google Scholar]