Abstract
1. The electrical activity of Purkinje cells was studied in guinea-pig cerebellar slices in vitro. Intracellular recordings from Purkinje cell somata were obtained under direct vision, and antidromic, synaptic and direct electroresponsiveness was demonstrated. Synaptic potentials produced by the activation of the climbing fibre afferent could be reversed by direct membrane depolarization. 2. Input resistance of impaled neurones ranged from 10 to 19 M omega and demonstrated non-linearities in both hyperpolarizing and depolarizing directions. 3. Direct activation of a Purkinje cell indicated that repetitive firing of fast somatic spikes (s.s.) occurs, after a threshold, with a minimum spike frequency of about 30 spikes/sec, resembling the '2-class' response of crab nerve (Hodgkin, 1948). 4. As the amplitude of the stimulus was increased, a second form of electroresponsiveness characterized by depolarizing spike bursts (d.s.b.) was observed and was often accomppanied by momentary inactivation of the s.s. potentials. Upon application of tetrodotoxin (TTX) or removal of Na+ ions from the superfusion fluid, the s.s. potentials were abolished while the burst responses remained intact. However, Ca conductance blockers such as Co, Cd, Mn and D600, or the replacement of Ca by Mg, completely abolish d.s.b.s. 5. If Ca conductance was blocked, or Ca removed from the superfusion fluid without blockage of Na conductance, two types of Na-dependent electroresponsiveness were seen: (a) the s.s. potentials and (b) slow rising all-or-none responses which reached plateau at approximately -15 mV and could last for several seconds. These all-or-none Na-dependent plateau depolarizations outlasted the stimulus and were accompanied by a large increase in membrane conductance. Within certain limits the rate of rise and amplitude of the plateau were independent of stimulus strength. The latency, however, was shortened as stimulus amplitude was increased. These potentials were blocked by TTX or by Na-free solutions. 6. Substitution of extracellular Ca by Ba or intracellular injection of tetraethylammonium generated prolonged action potentials lasting for several seconds and showing a plateau more ositive than those obtained in norrmal circumstances by either non-inactivating Na or Ca currents. 7. Spontaneous firing of the Purkinje cell was characterized by burst-like activity consisting of both s.s. and d.s.b. responses. Addition of TTX to the bath left the basic spontaneous activity and its frequency unaltered, indicating tha Ca spiking and Ca-dependent K conductance changes are the main events underlying this oscillatory behaviour. 8...
Full text
PDF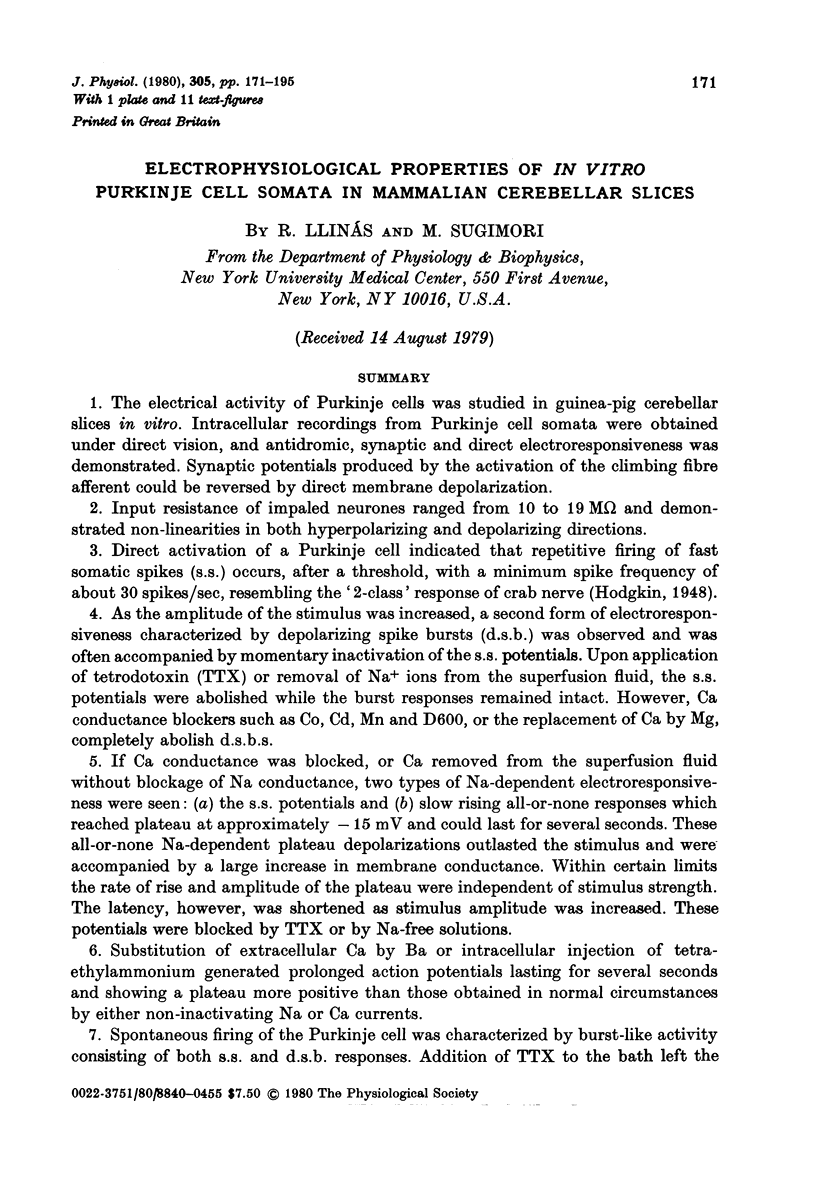
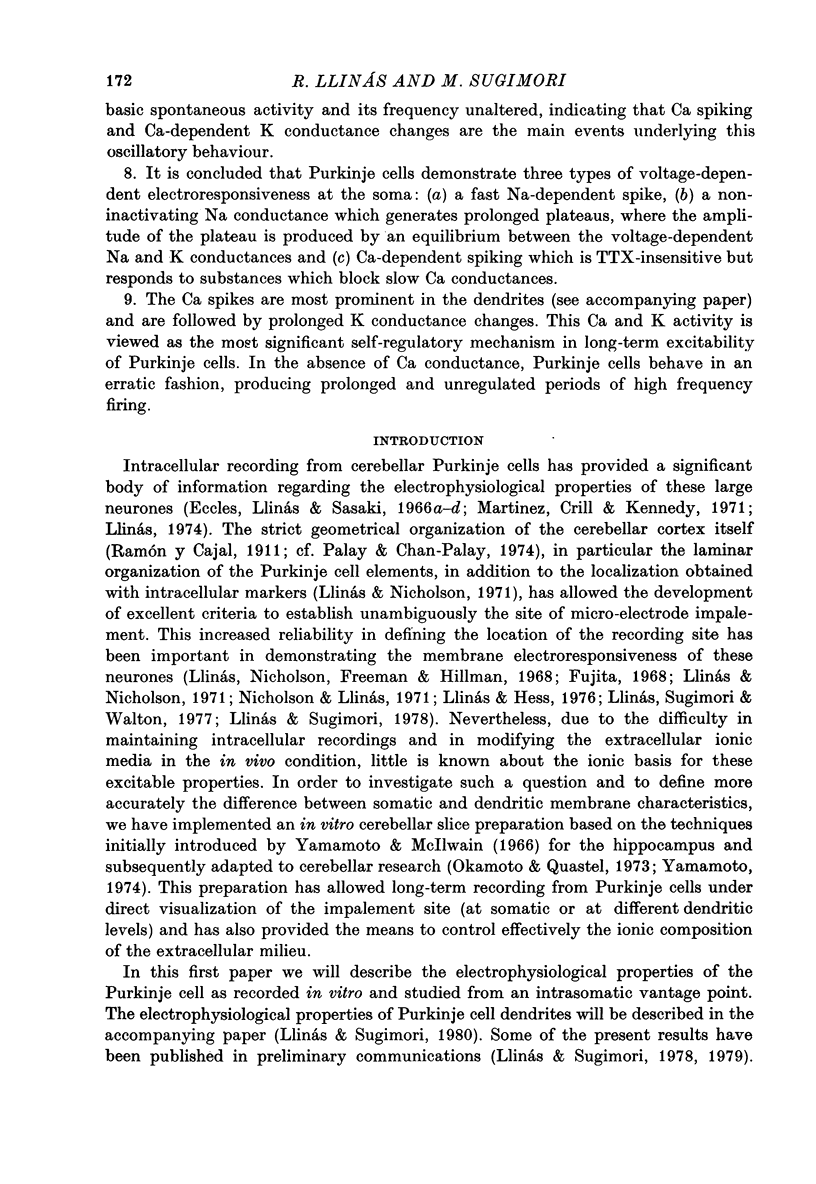
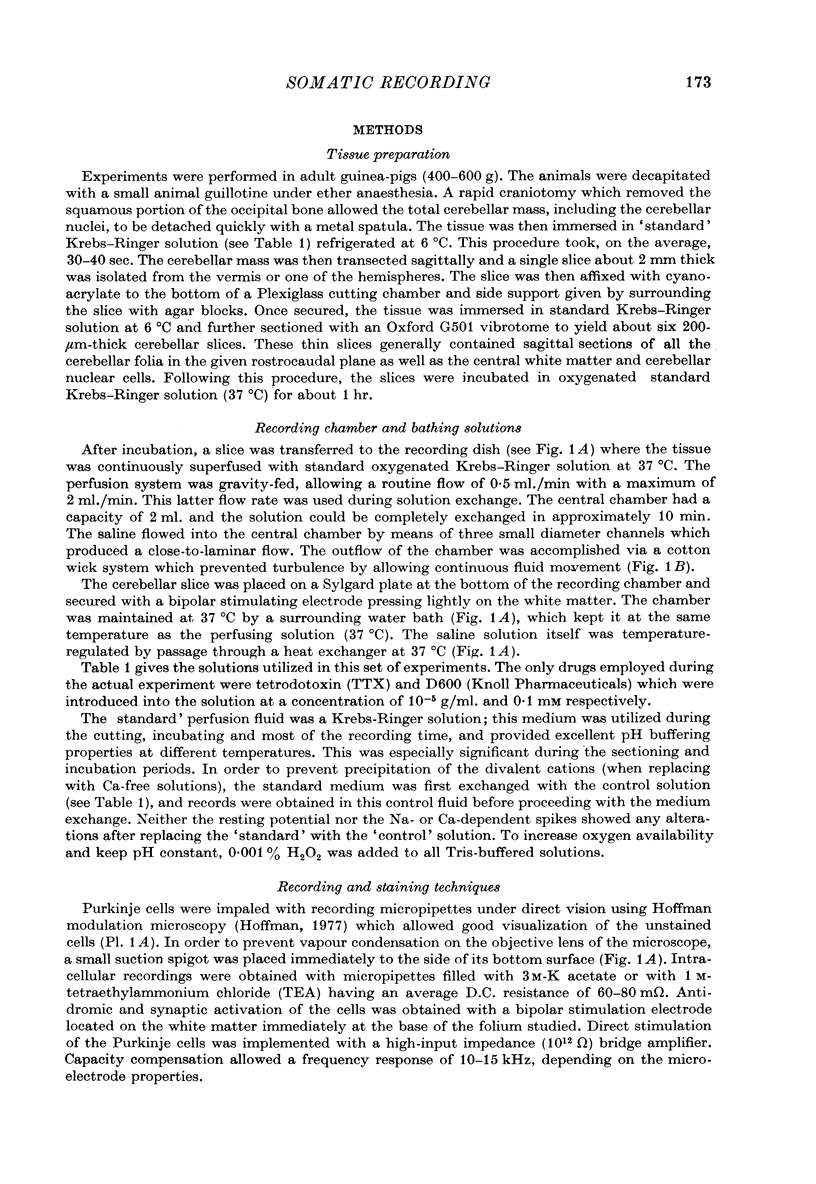
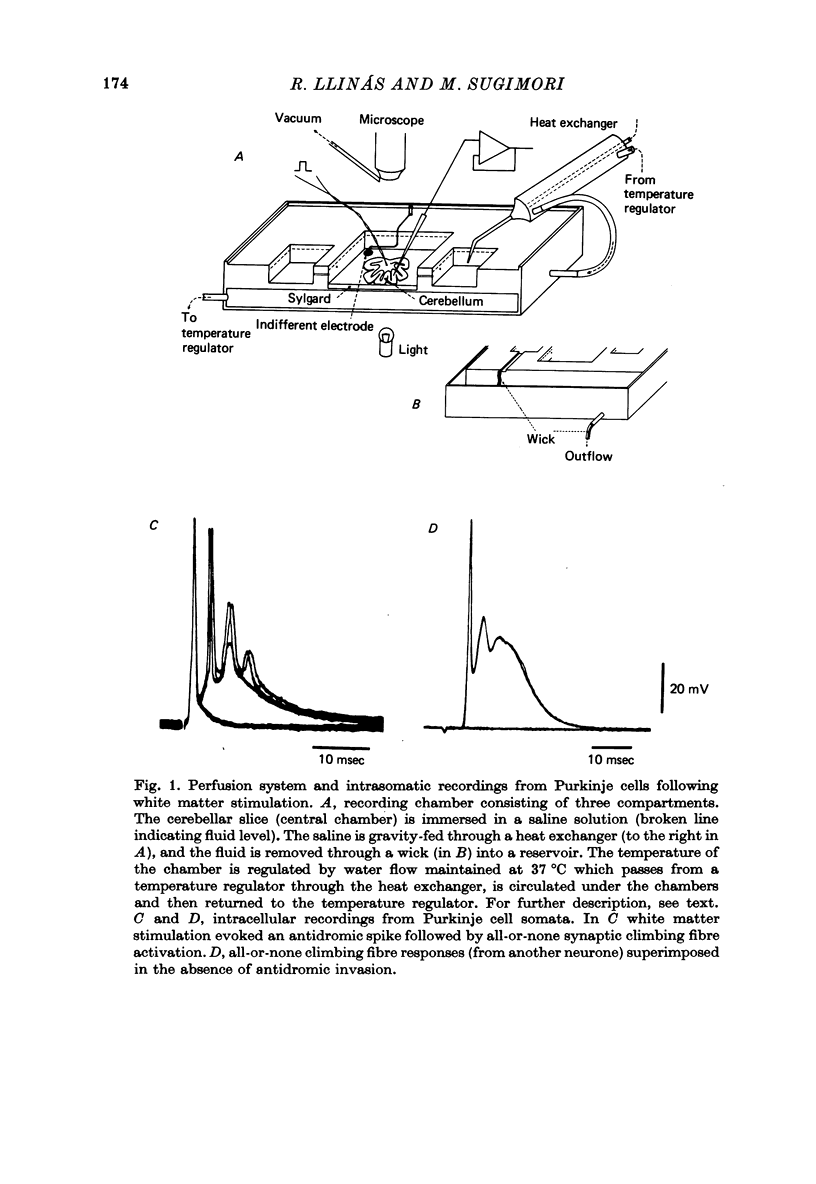
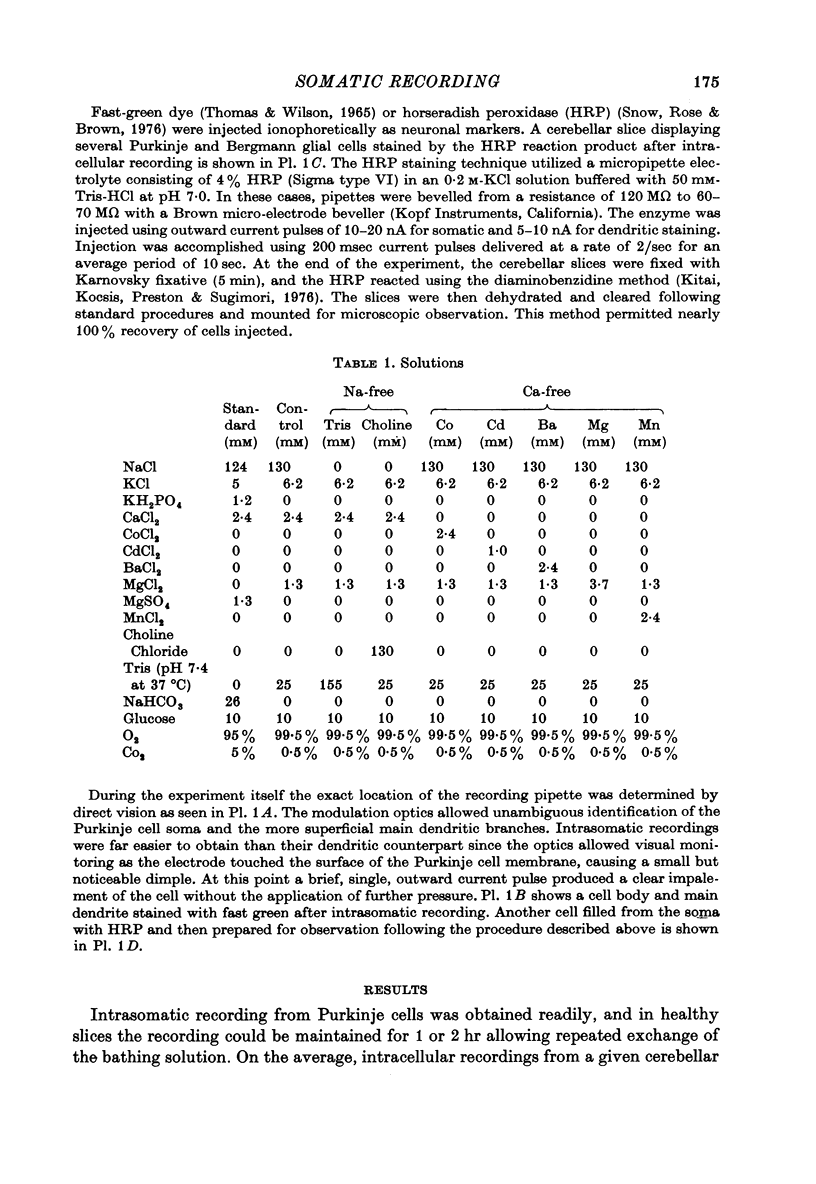
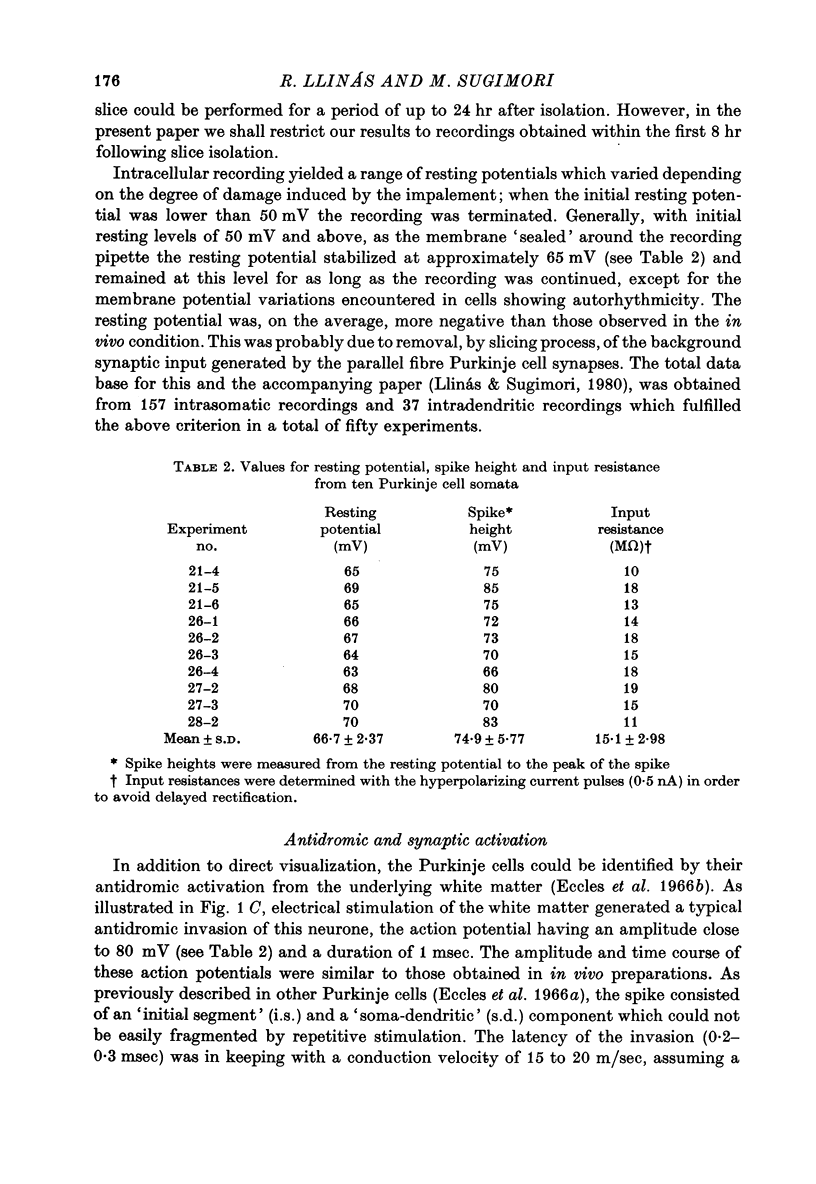
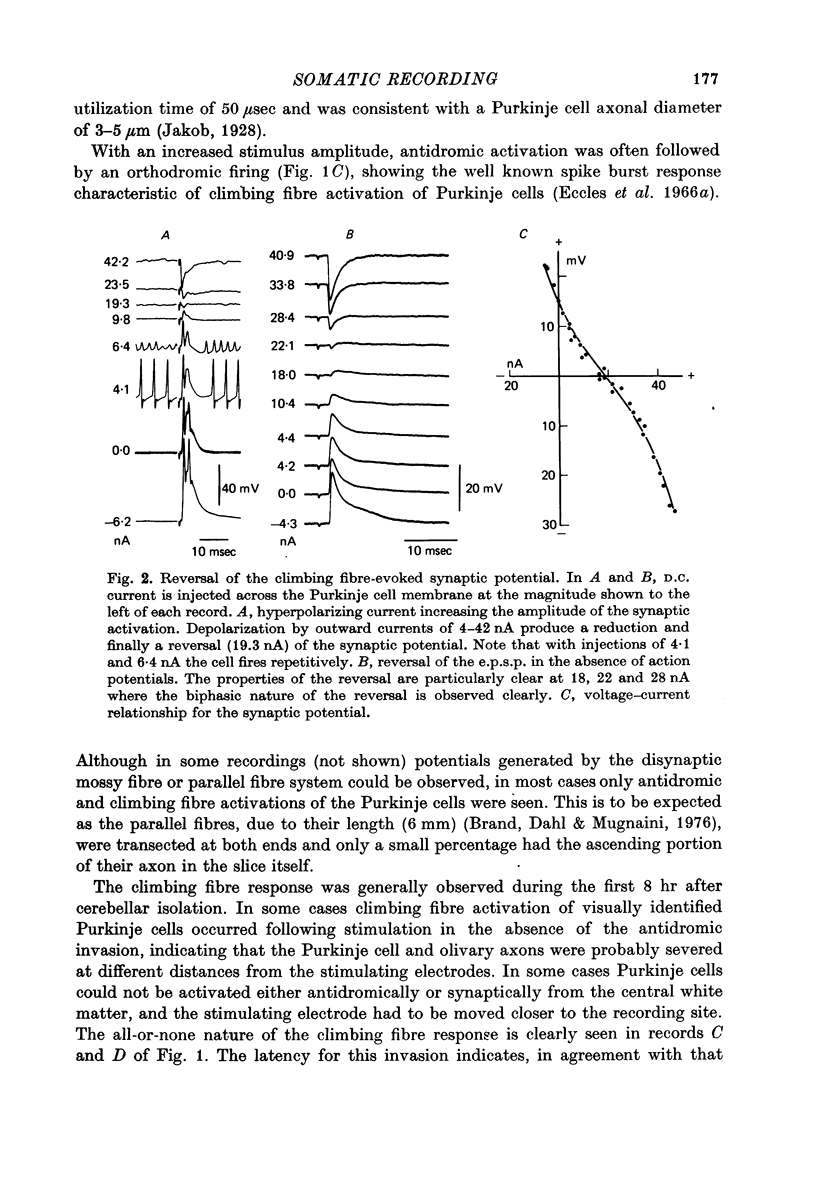
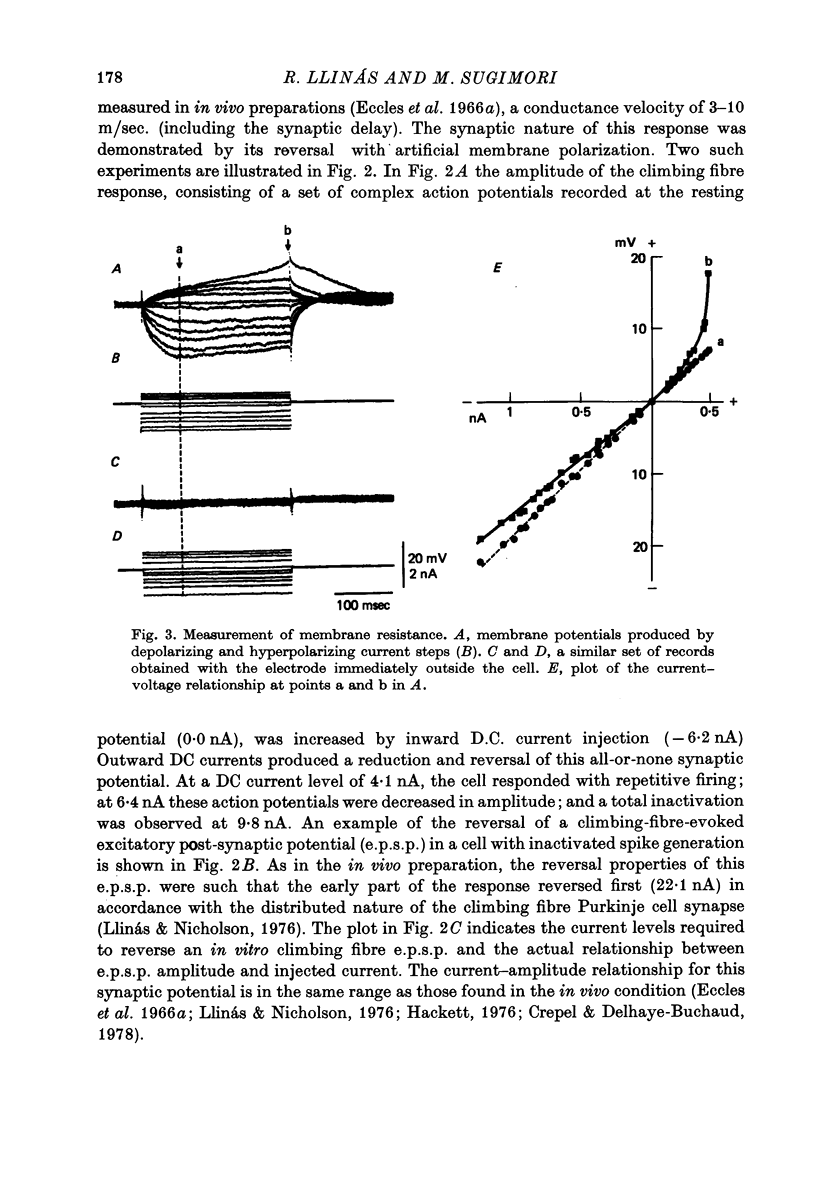
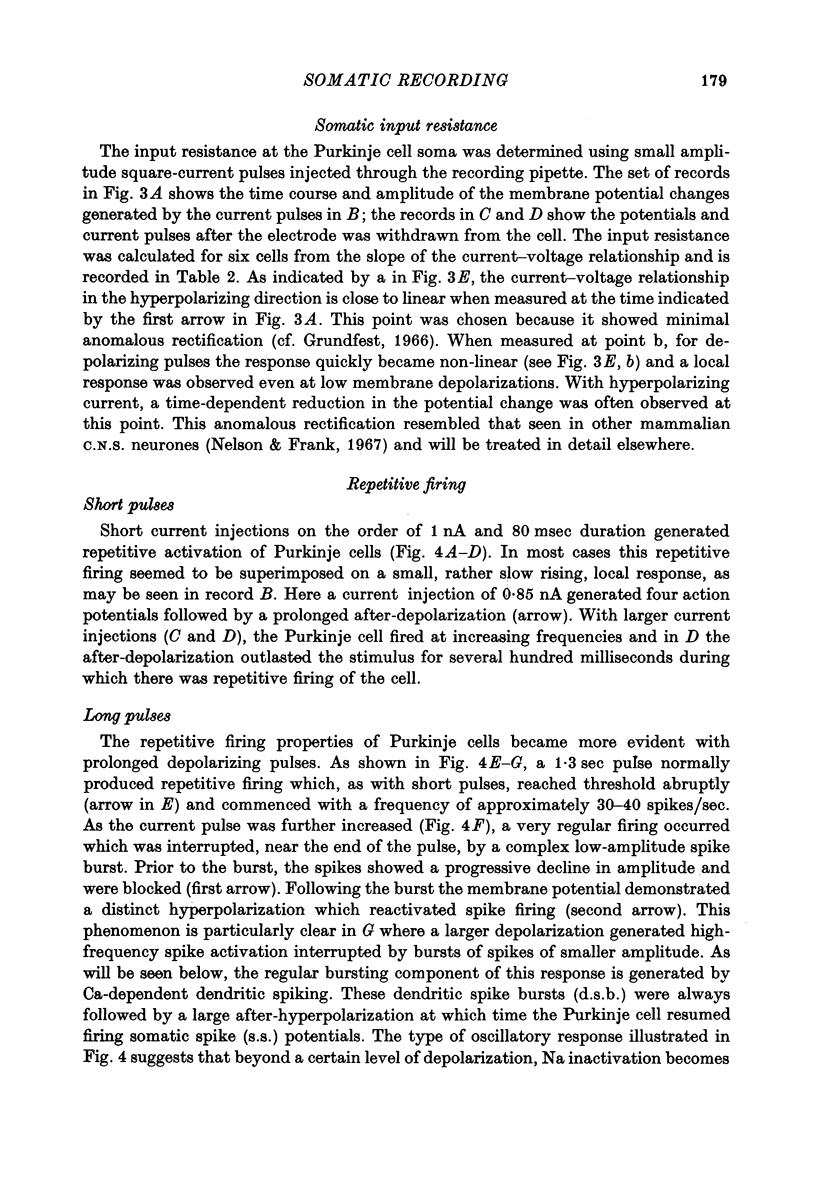
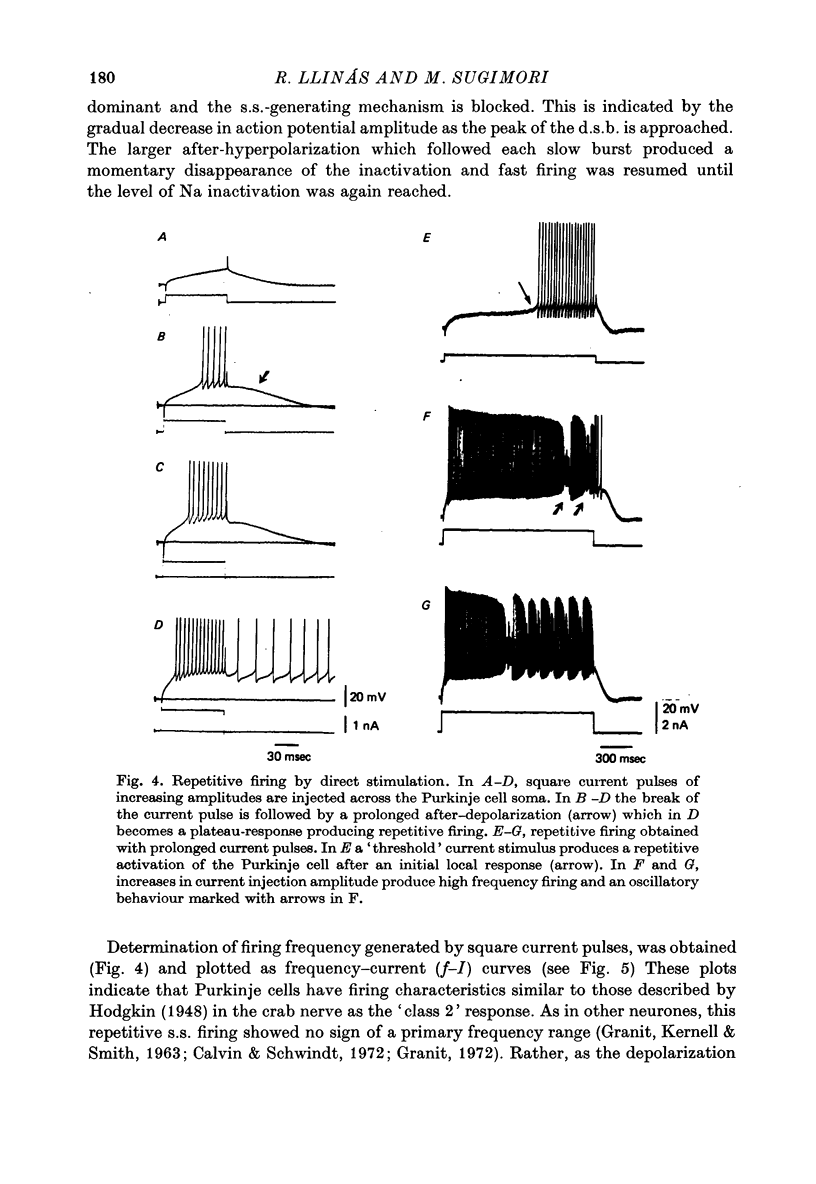
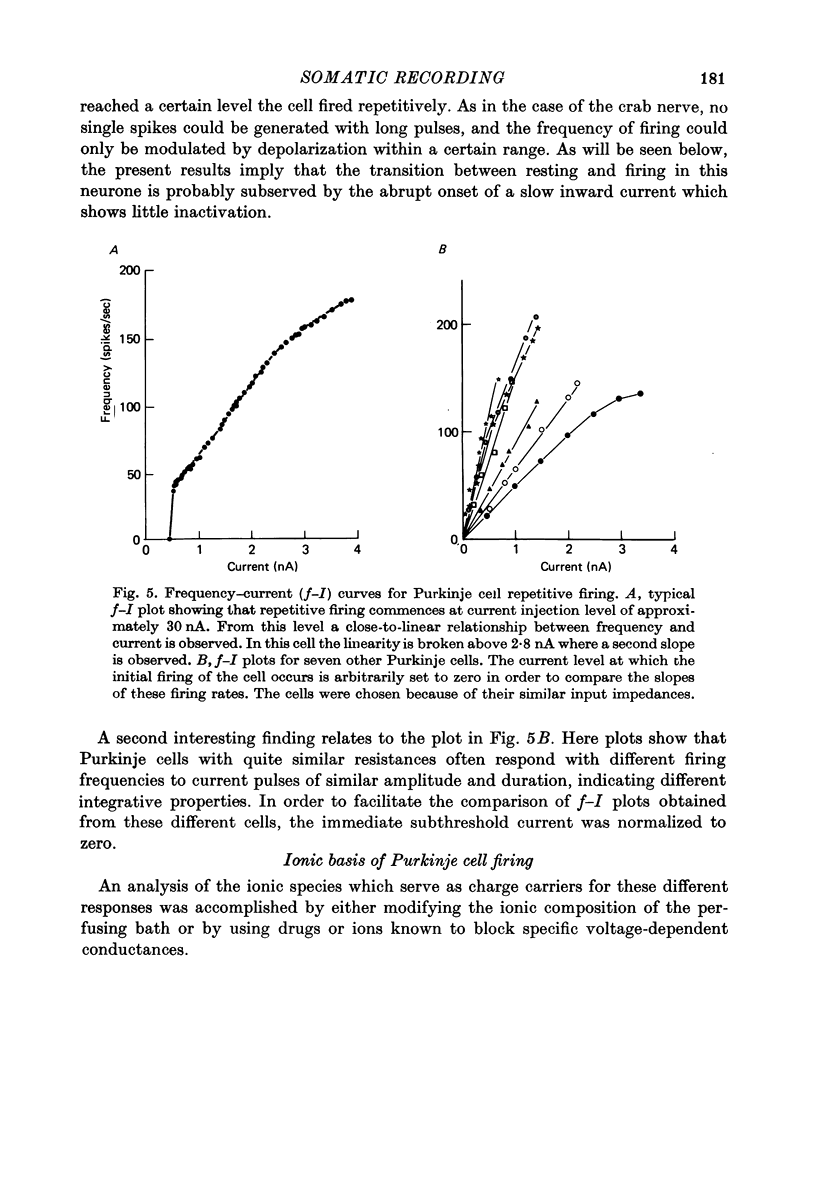
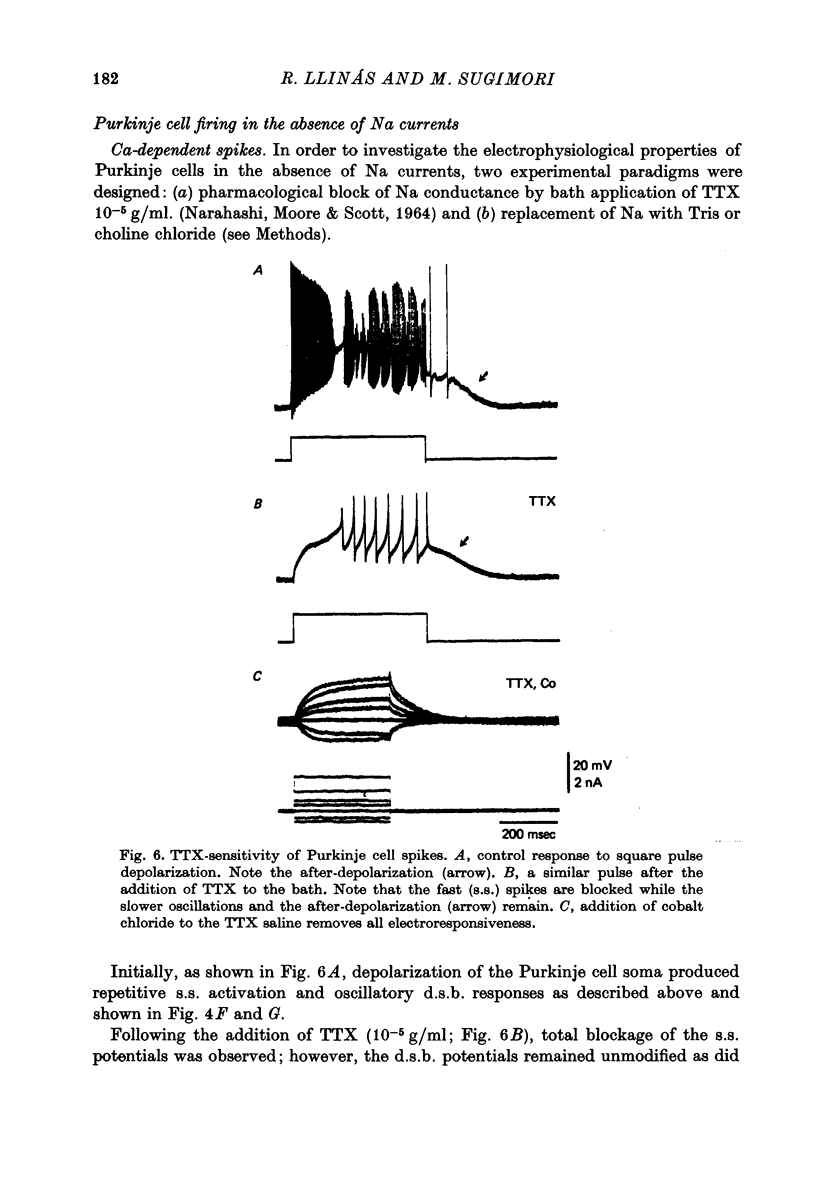
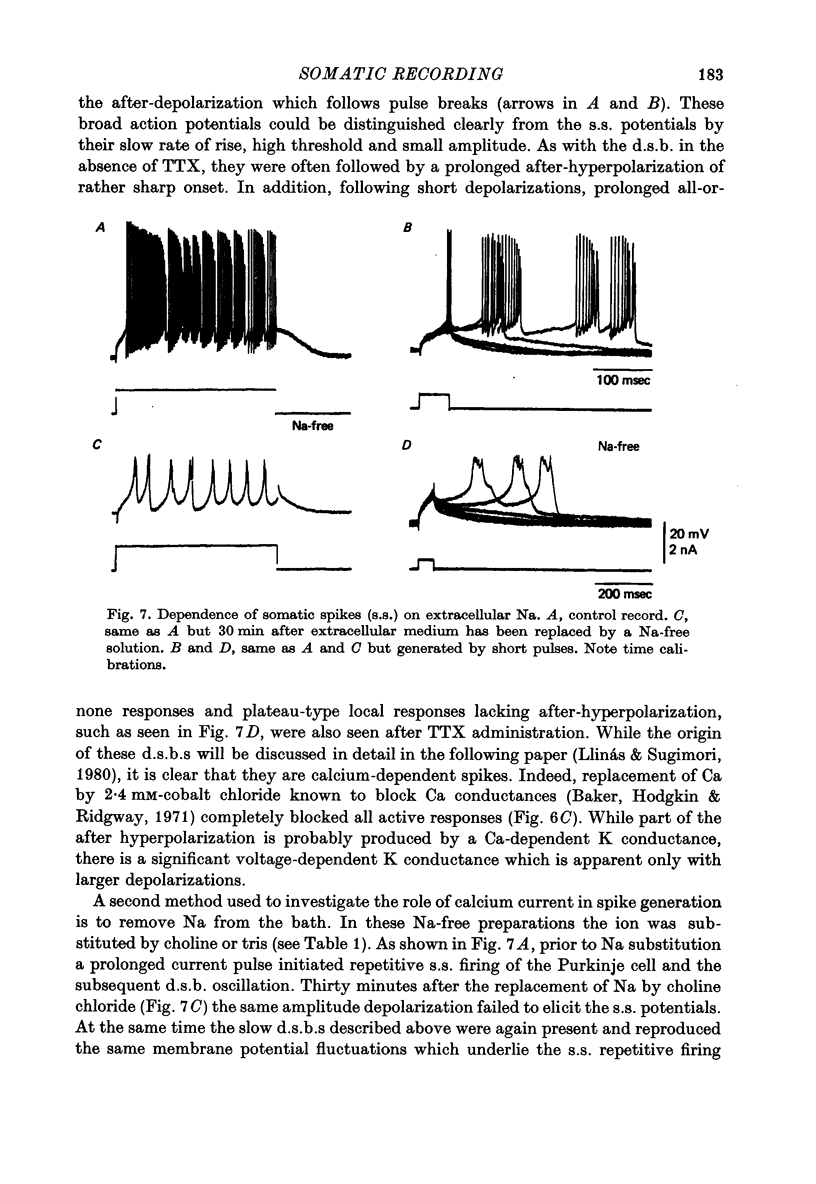
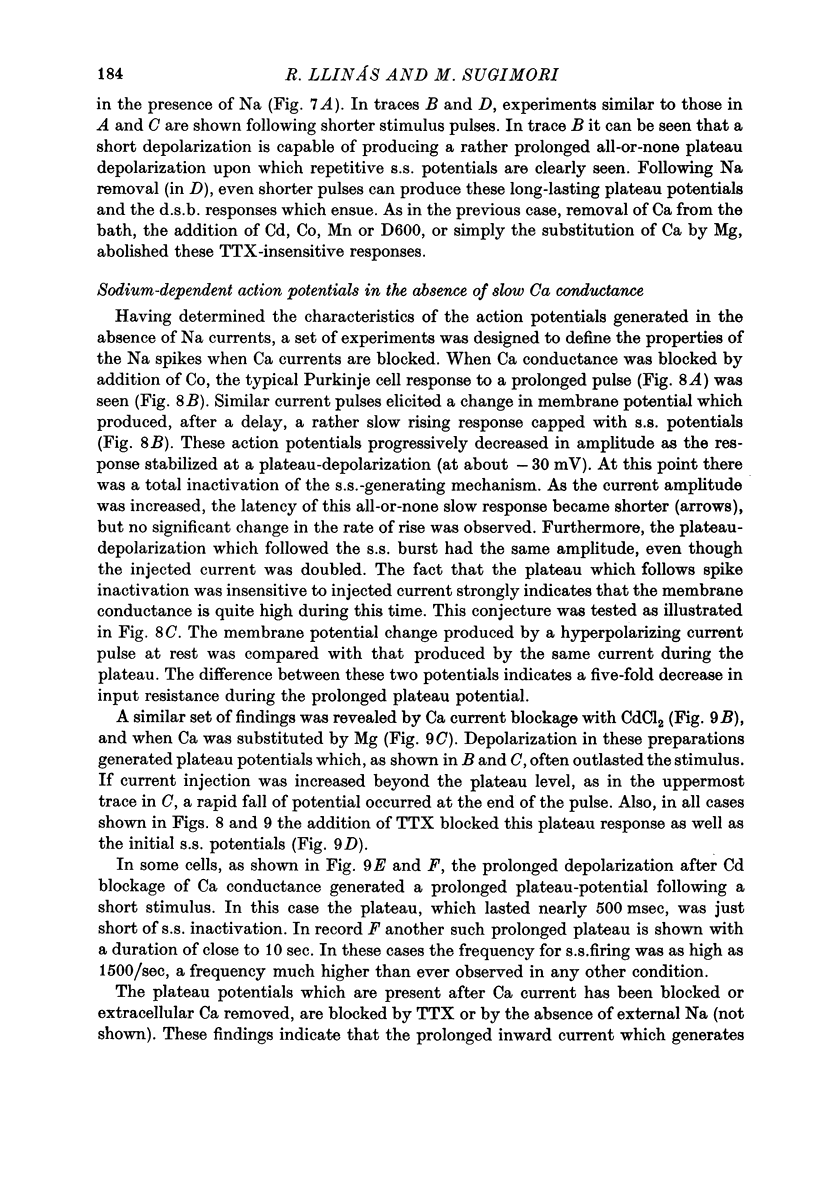
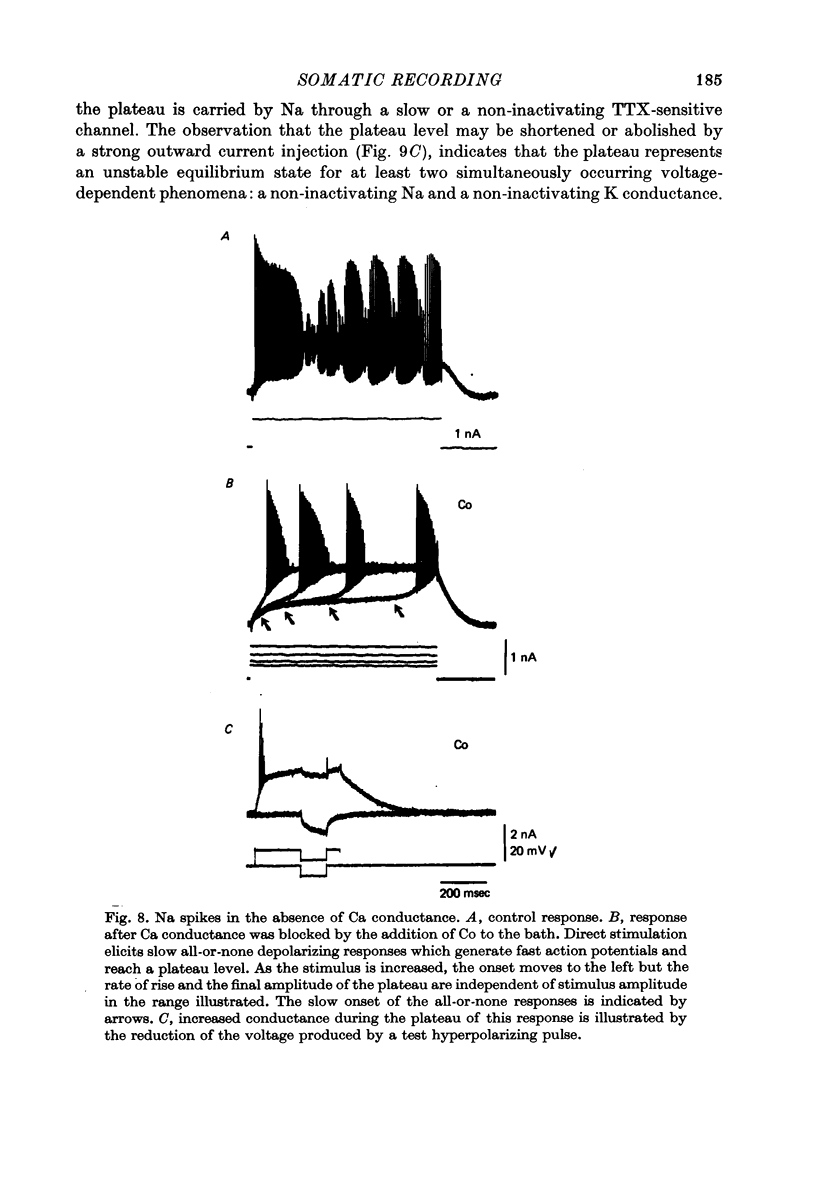
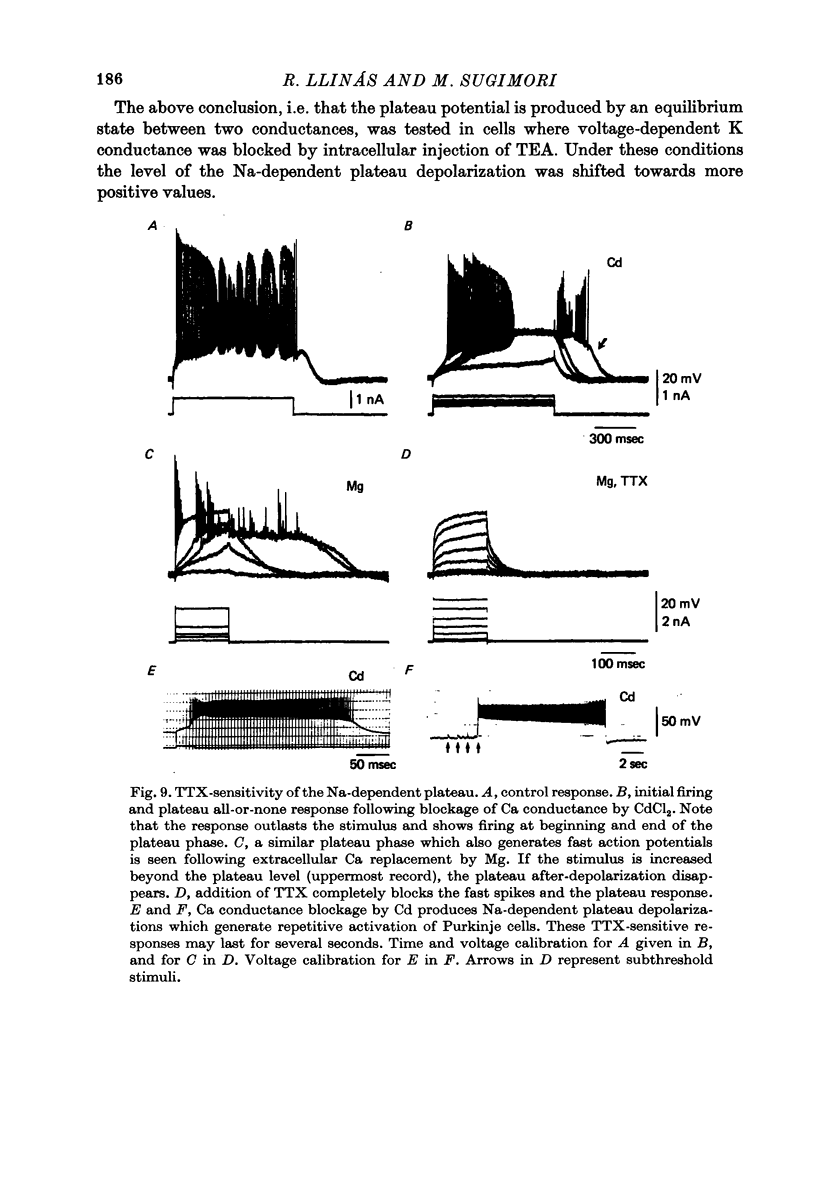
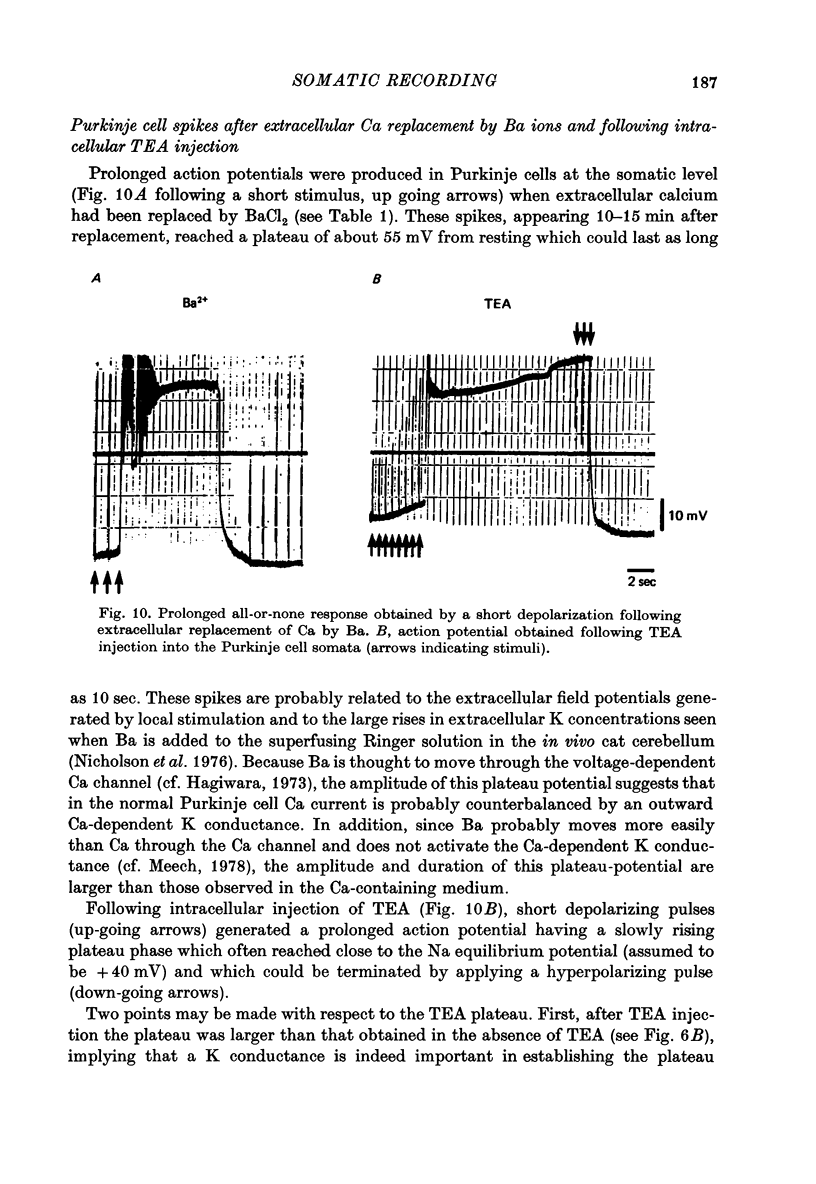
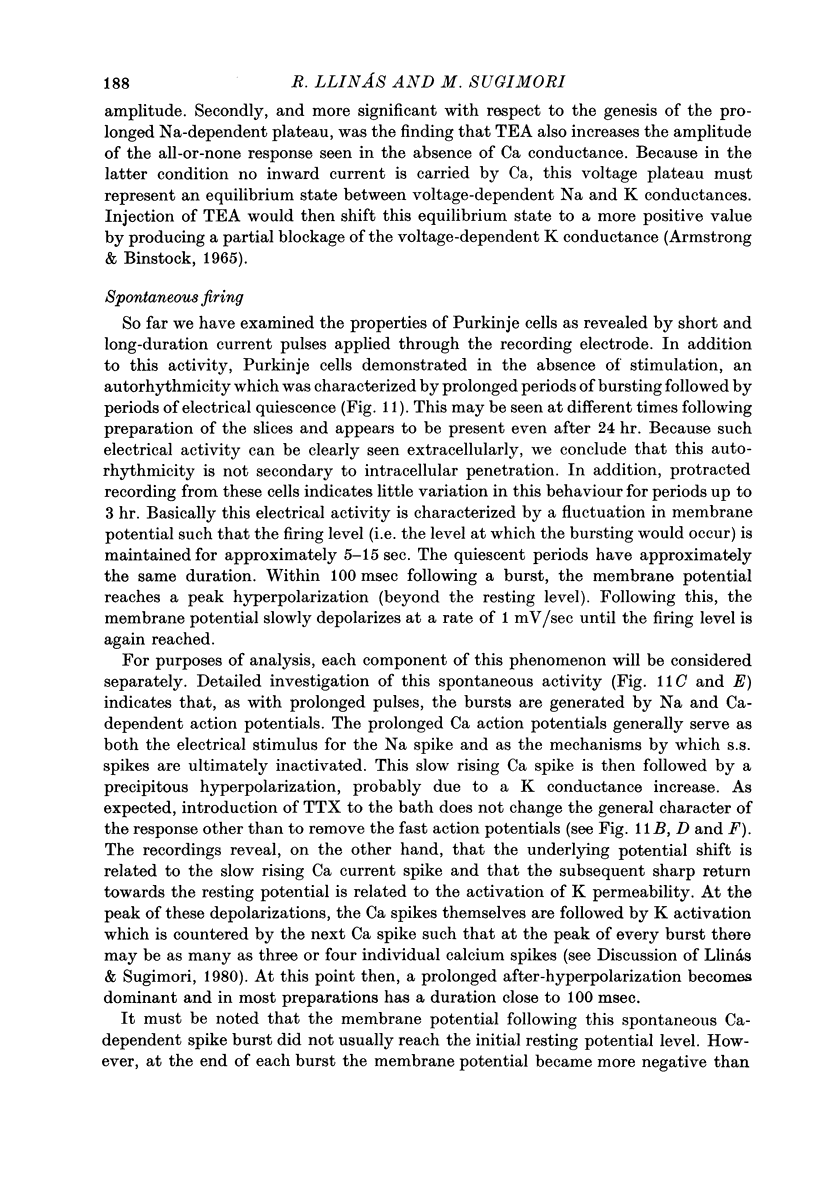
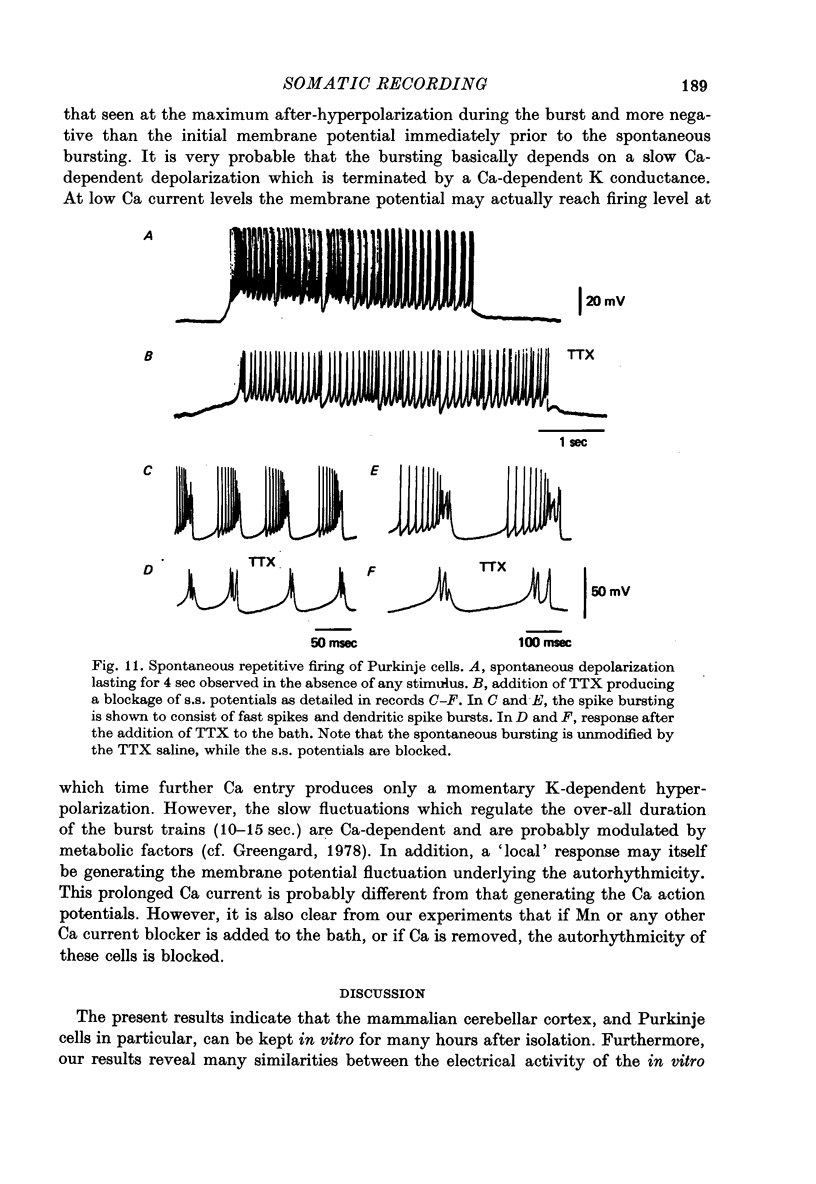
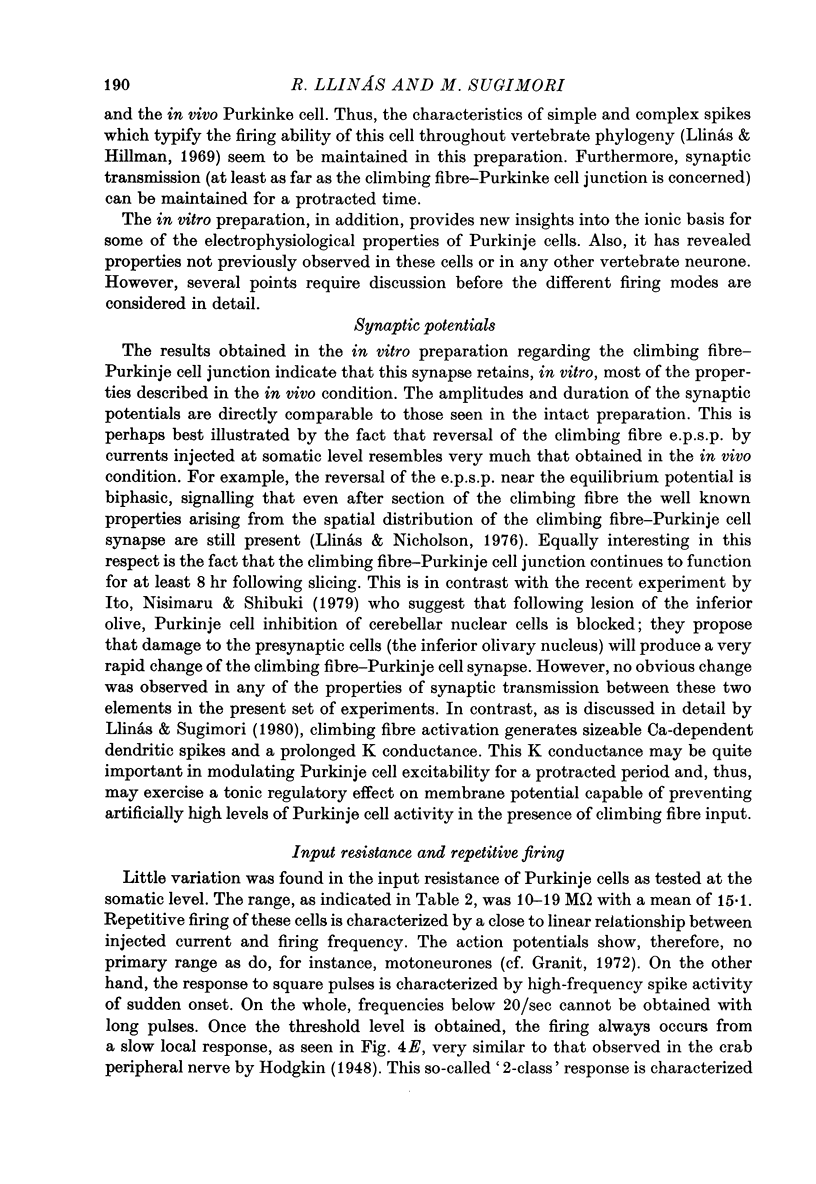
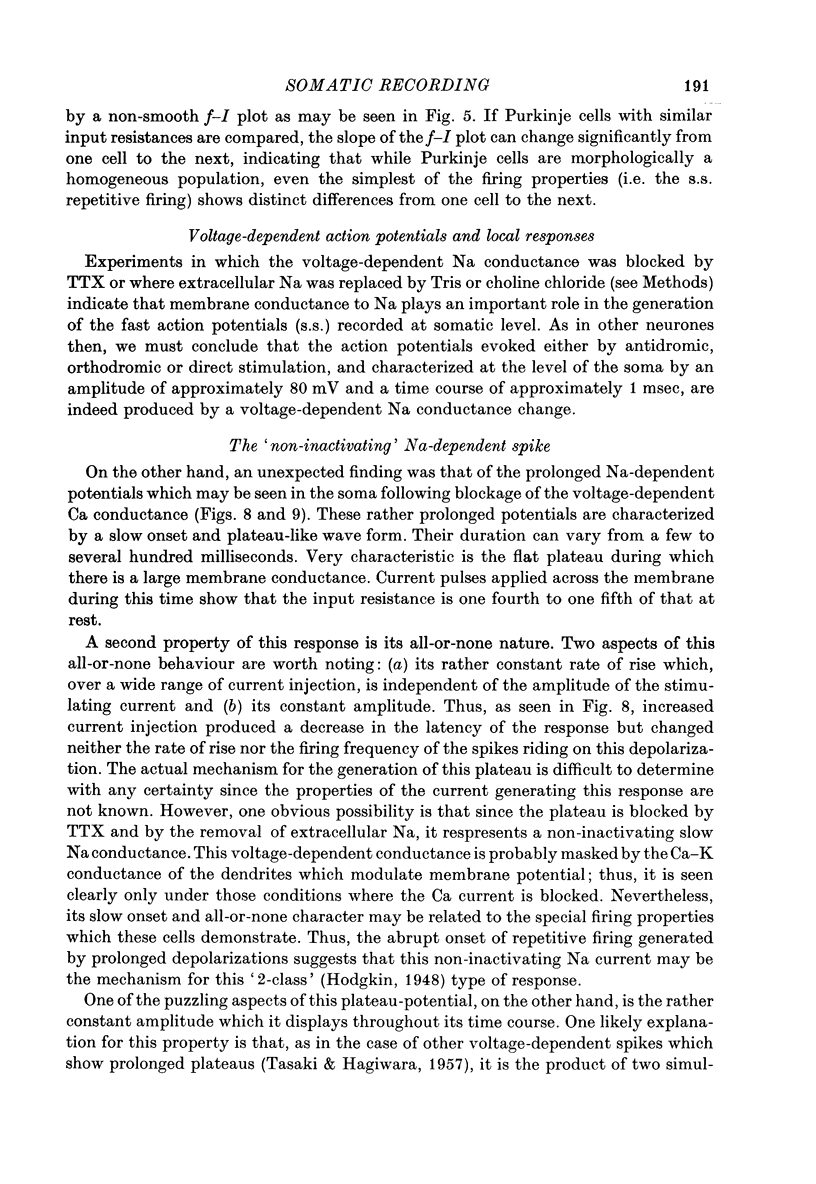
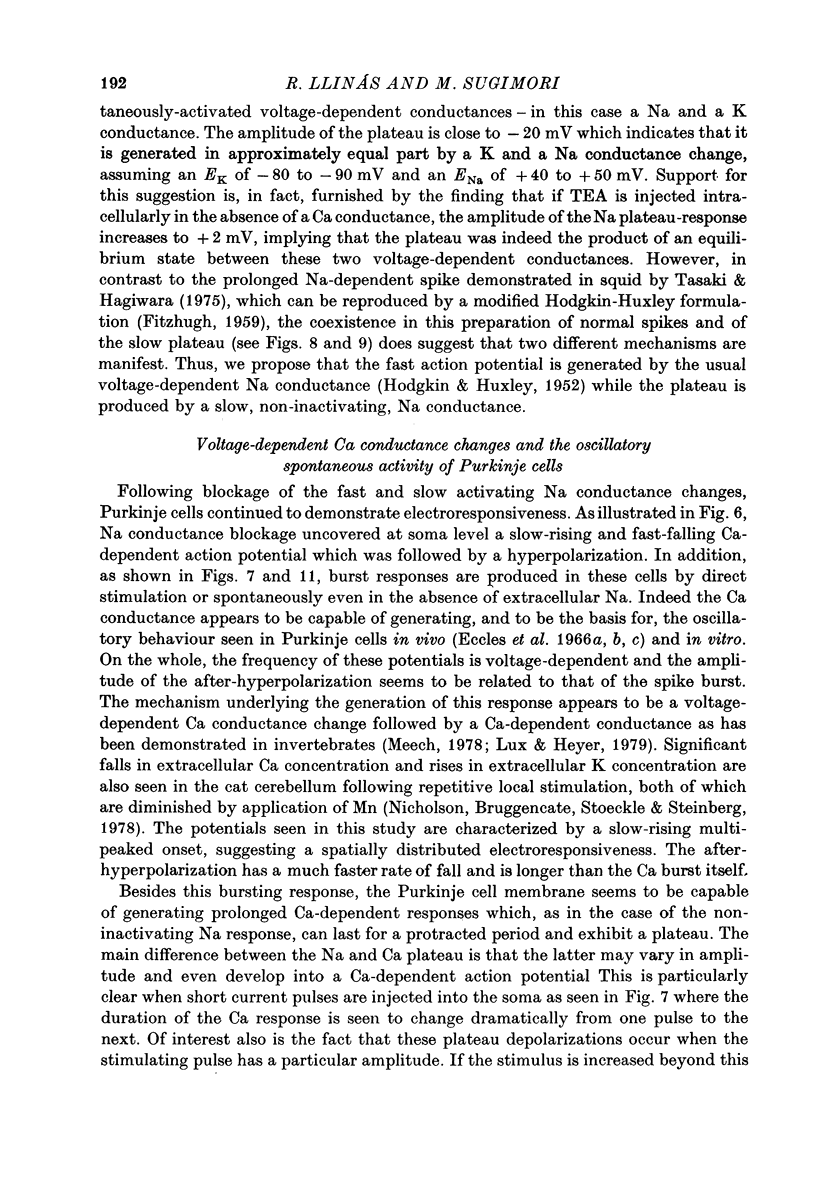
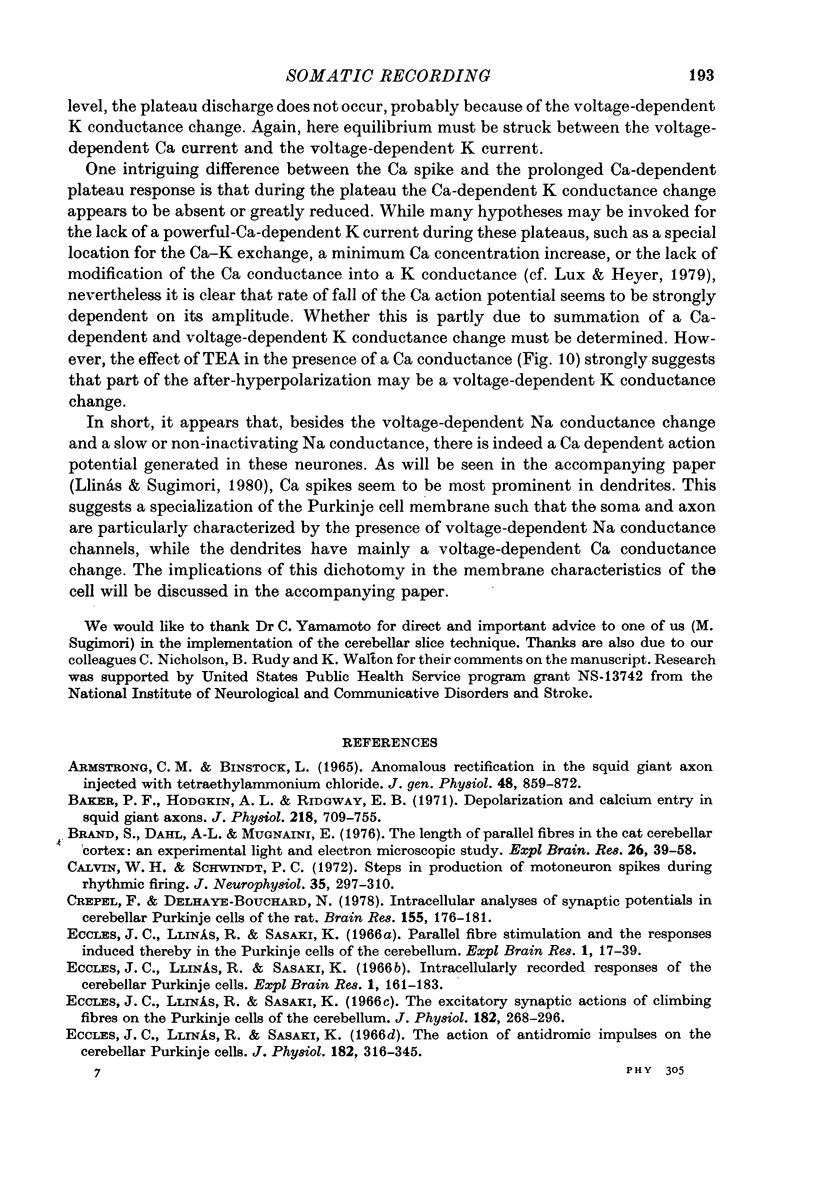
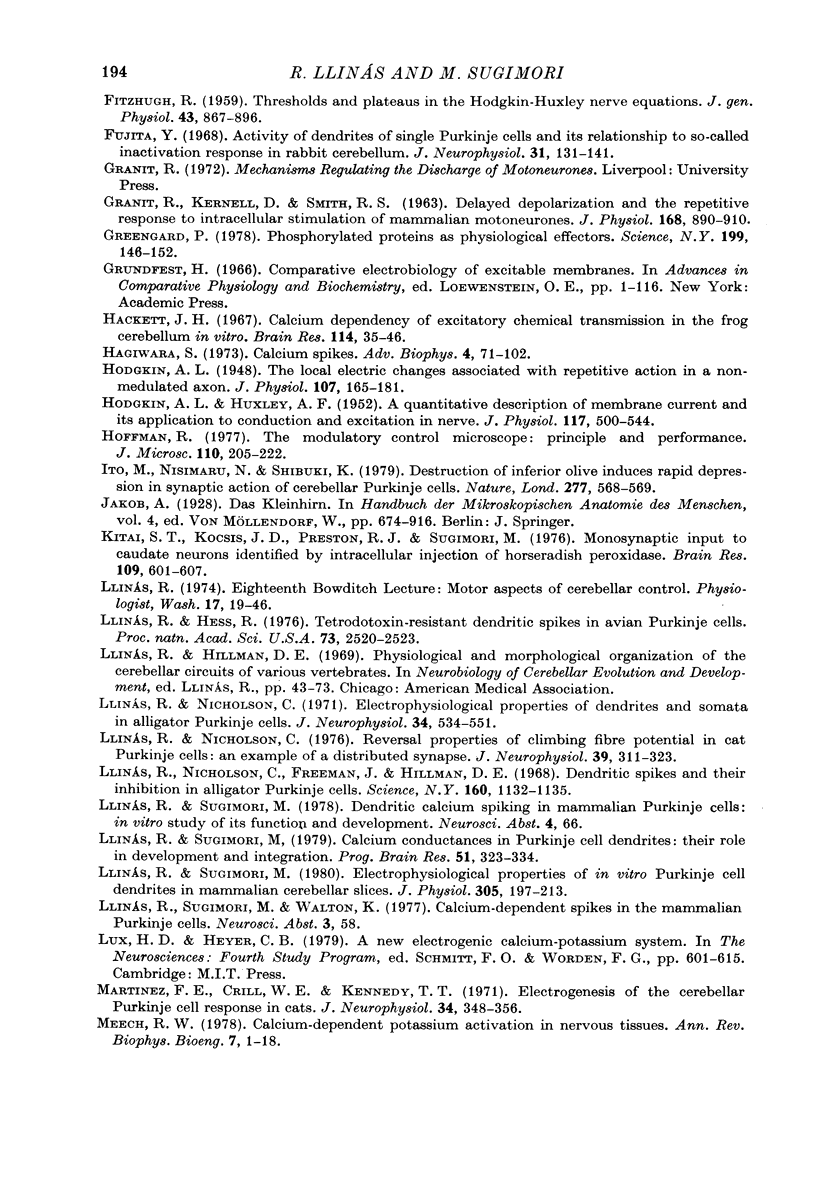
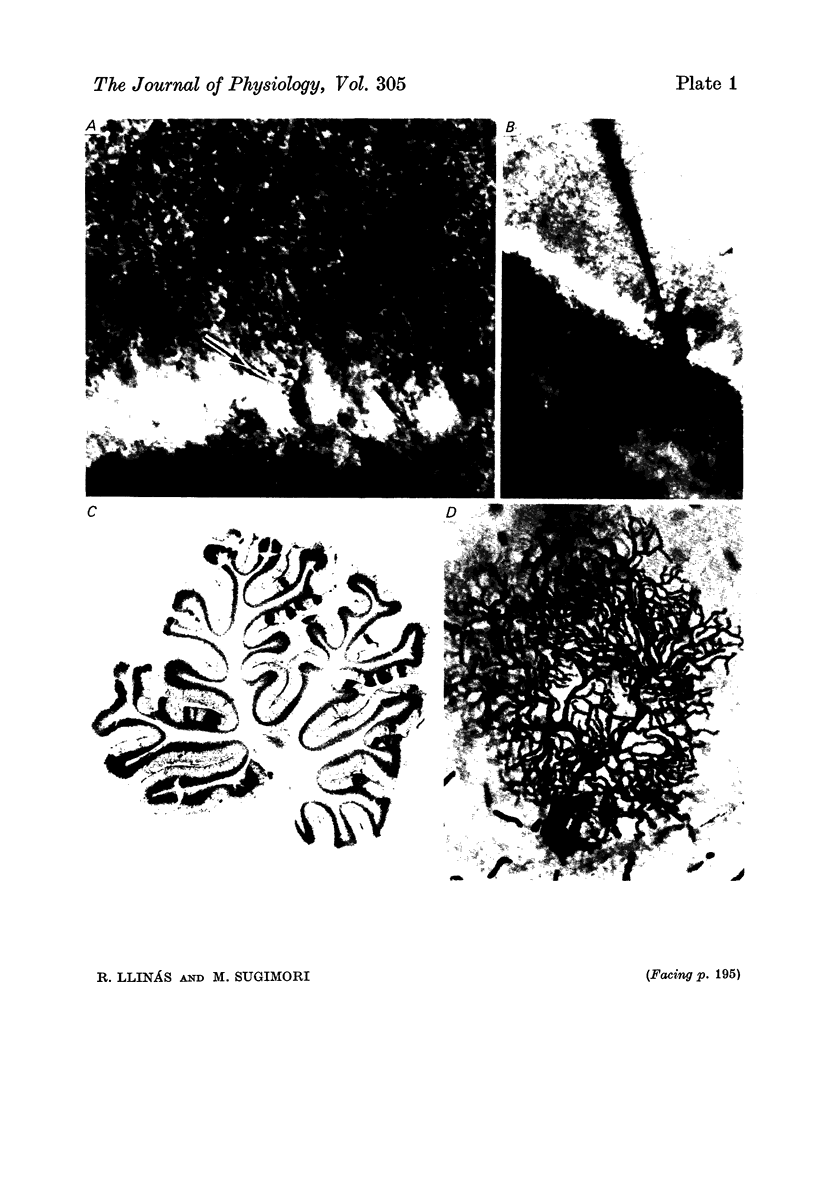
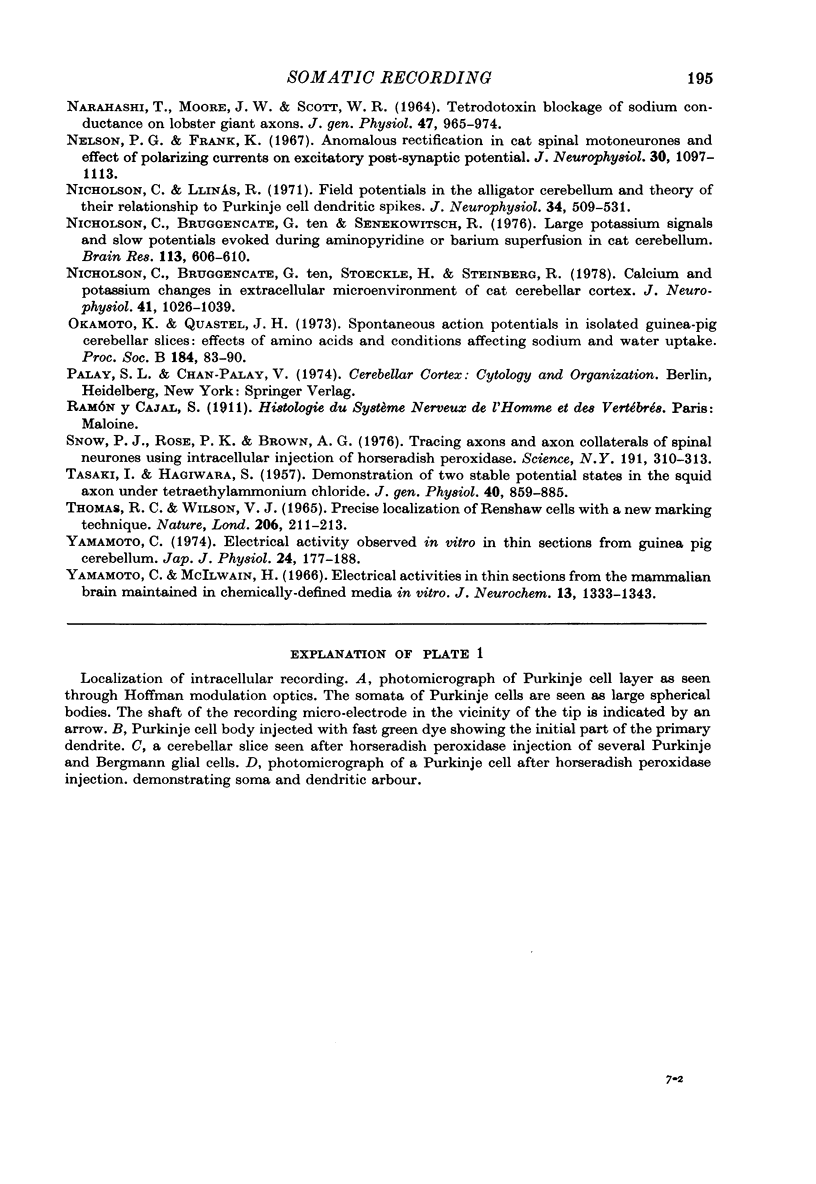
Images in this article
Selected References
These references are in PubMed. This may not be the complete list of references from this article.
- ARMSTRONG C. M., BINSTOCK L. ANOMALOUS RECTIFICATION IN THE SQUID GIANT AXON INJECTED WITH TETRAETHYLAMMONIUM CHLORIDE. J Gen Physiol. 1965 May;48:859–872. doi: 10.1085/jgp.48.5.859. [DOI] [PMC free article] [PubMed] [Google Scholar]
- Baker P. F., Hodgkin A. L., Ridgway E. B. Depolarization and calcium entry in squid giant axons. J Physiol. 1971 Nov;218(3):709–755. doi: 10.1113/jphysiol.1971.sp009641. [DOI] [PMC free article] [PubMed] [Google Scholar]
- Brand S., Dahl A. L., Mugnaini E. The length of parallel fibers in the cat cerebellar cortex. An experimental light and electron microscopic study. Exp Brain Res. 1976 Aug 27;26(1):39–58. doi: 10.1007/BF00235248. [DOI] [PubMed] [Google Scholar]
- Calvin W. H., Schwindt P. C. Steps in production of motoneuron spikes during rhythmic firing. J Neurophysiol. 1972 May;35(3):297–310. doi: 10.1152/jn.1972.35.3.297. [DOI] [PubMed] [Google Scholar]
- Crepel F., Delhaye-Bouchaud N. Intracellular analyses of synaptic potentials in cerebellar Purkinje cells of the rat. Brain Res. 1978 Oct 20;155(1):176–181. doi: 10.1016/0006-8993(78)90321-9. [DOI] [PubMed] [Google Scholar]
- Eccles J. C., Llinás R., Sasaki K. Intracellularly recorded responses of the cerebellar Purkinje cells. Exp Brain Res. 1966;1(2):161–183. doi: 10.1007/BF00236869. [DOI] [PubMed] [Google Scholar]
- Eccles J. C., Llinás R., Sasaki K. Parallel fibre stimulation and the responses induced thereby in the Purkinje cells of the cerebellum. Exp Brain Res. 1966;1(1):17–39. doi: 10.1007/BF00235207. [DOI] [PubMed] [Google Scholar]
- Eccles J. C., Llinás R., Sasaki K. The excitatory synaptic action of climbing fibres on the Purkinje cells of the cerebellum. J Physiol. 1966 Jan;182(2):268–296. doi: 10.1113/jphysiol.1966.sp007824. [DOI] [PMC free article] [PubMed] [Google Scholar]
- FITZHUGH R. Thresholds and plateaus in the Hodgkin-Huxley nerve equations. J Gen Physiol. 1960 May;43:867–896. doi: 10.1085/jgp.43.5.867. [DOI] [PMC free article] [PubMed] [Google Scholar]
- Fujita Y. Activity of dendrites of single Purkinje cells and its relationship to so-called inactivation response in rabbit cerebellum. J Neurophysiol. 1968 Mar;31(2):131–141. doi: 10.1152/jn.1968.31.2.131. [DOI] [PubMed] [Google Scholar]
- GRANIT R., KERNELL D., SMITH R. S. DELAYED DEPOLARIZATION AND THE REPETITIVE RESPONSE TO INTRACELLULAR STIMULATION OF MAMMALIAN MOTONEURONES. J Physiol. 1963 Oct;168:890–910. doi: 10.1113/jphysiol.1963.sp007229. [DOI] [PMC free article] [PubMed] [Google Scholar]
- Greengard P. Phosphorylated proteins as physiological effectors. Science. 1978 Jan 13;199(4325):146–152. doi: 10.1126/science.22932. [DOI] [PubMed] [Google Scholar]
- Grundfest H. Comparative electrobiology of excitable membranes. Adv Comp Physiol Biochem. 1966;2:1–116. doi: 10.1016/b978-0-12-395511-1.50006-8. [DOI] [PubMed] [Google Scholar]
- HODGKIN A. L., HUXLEY A. F. A quantitative description of membrane current and its application to conduction and excitation in nerve. J Physiol. 1952 Aug;117(4):500–544. doi: 10.1113/jphysiol.1952.sp004764. [DOI] [PMC free article] [PubMed] [Google Scholar]
- Hackett J. T. Calcium dependency of excitatory chemical synaptic transmission in the frog cerebellum in vitro. Brain Res. 1976 Sep 10;114(1):35–46. doi: 10.1016/0006-8993(76)91005-2. [DOI] [PubMed] [Google Scholar]
- Hagiwara S. Ca spike. Adv Biophys. 1973;4:71–102. [PubMed] [Google Scholar]
- Hodgkin A. L. The local electric changes associated with repetitive action in a non-medullated axon. J Physiol. 1948 Mar 15;107(2):165–181. doi: 10.1113/jphysiol.1948.sp004260. [DOI] [PMC free article] [PubMed] [Google Scholar]
- Ito M., Nisimaru N., Shibuki K. Destruction of inferior olive induces rapid depression in synaptic action of cerebellar Purkinje cells. Nature. 1979 Feb 15;277(5697):568–569. doi: 10.1038/277568a0. [DOI] [PubMed] [Google Scholar]
- Kitai S. T., Kocsis J. D., Preston R. J., Sugimori M. Monosynaptic inputs to caudate neurons identified by intracellular injection of horseradish peroxidase. Brain Res. 1976 Jun 18;109(3):601–606. doi: 10.1016/0006-8993(76)90039-1. [DOI] [PubMed] [Google Scholar]
- Llinas R., Nicholson C. Electrophysiological properties of dendrites and somata in alligator Purkinje cells. J Neurophysiol. 1971 Jul;34(4):532–551. doi: 10.1152/jn.1971.34.4.532. [DOI] [PubMed] [Google Scholar]
- Llinás R., Hess R. Tetrodotoxin-resistant dendritic spikes in avian Purkinje cells. Proc Natl Acad Sci U S A. 1976 Jul;73(7):2520–2523. doi: 10.1073/pnas.73.7.2520. [DOI] [PMC free article] [PubMed] [Google Scholar]
- Llinás R., Nicholson C., Freeman J. A., Hillman D. E. Dendritic spikes and their inhibition in alligator Purkinje cells. Science. 1968 Jun 7;160(3832):1132–1135. doi: 10.1126/science.160.3832.1132. [DOI] [PubMed] [Google Scholar]
- Llinás R., Nicholson C. Reversal properties of climbing fiber potential in cat Purkinje cells: an example of a distributed synapse. J Neurophysiol. 1976 Mar;39(2):311–323. doi: 10.1152/jn.1976.39.2.311. [DOI] [PubMed] [Google Scholar]
- Llinás R., Sugimori M. Calcium conductances in Purkinje cell dendrites: their role in development and integration. Prog Brain Res. 1979;51:323–334. doi: 10.1016/S0079-6123(08)61312-6. [DOI] [PubMed] [Google Scholar]
- Llinás R., Sugimori M. Electrophysiological properties of in vitro Purkinje cell dendrites in mammalian cerebellar slices. J Physiol. 1980 Aug;305:197–213. doi: 10.1113/jphysiol.1980.sp013358. [DOI] [PMC free article] [PubMed] [Google Scholar]
- Martinez F. E., Crill W. E., Kennedy T. T. Electrogenesis of cerebellar Purkinje cell responses in cats. J Neurophysiol. 1971 May;34(3):348–356. doi: 10.1152/jn.1971.34.3.348. [DOI] [PubMed] [Google Scholar]
- Meech R. W. Calcium-dependent potassium activation in nervous tissues. Annu Rev Biophys Bioeng. 1978;7:1–18. doi: 10.1146/annurev.bb.07.060178.000245. [DOI] [PubMed] [Google Scholar]
- NARAHASHI T., MOORE J. W., SCOTT W. R. TETRODOTOXIN BLOCKAGE OF SODIUM CONDUCTANCE INCREASE IN LOBSTER GIANT AXONS. J Gen Physiol. 1964 May;47:965–974. doi: 10.1085/jgp.47.5.965. [DOI] [PMC free article] [PubMed] [Google Scholar]
- Nelson P. G., Frank K. Anomalous rectification in cat spinal motoneurons and effect of polarizing currents on excitatory postsynaptic potential. J Neurophysiol. 1967 Sep;30(5):1097–1113. doi: 10.1152/jn.1967.30.5.1097. [DOI] [PubMed] [Google Scholar]
- Nicholson C., Bruggencate G. T., Senekowitsch R. Large potassium signals and slow potentials evoked during aminopyridine or barium superfusion in cat cerebellum. Brain Res. 1976 Sep 3;113(3):606–610. doi: 10.1016/0006-8993(76)90063-9. [DOI] [PubMed] [Google Scholar]
- Nicholson C., Llinas R. Field potentials in the alligator cerebellum and theory of their relationship to Purkinje cell dendritic spikes. J Neurophysiol. 1971 Jul;34(4):509–531. doi: 10.1152/jn.1971.34.4.509. [DOI] [PubMed] [Google Scholar]
- Nicholson C., ten Bruggencate G., Stöckle H., Steinberg R. Calcium and potassium changes in extracellular microenvironment of cat cerebellar cortex. J Neurophysiol. 1978 Jul;41(4):1026–1039. doi: 10.1152/jn.1978.41.4.1026. [DOI] [PubMed] [Google Scholar]
- Okamoto K., Quastel J. H. Spontaneous action potentials in isolated guinea-pig cerebellar slices: effects of amino acids and conditions affecting sodium and water uptake. Proc R Soc Lond B Biol Sci. 1973 Aug 31;184(1074):83–90. doi: 10.1098/rspb.1973.0032. [DOI] [PubMed] [Google Scholar]
- Snow P. J., Rose P. K., Brown A. G. Tracing axons and axon collaterals of spinal neurons using intracellular injection of horseradish peroxidase. Science. 1976 Jan 23;191(4224):312–313. doi: 10.1126/science.54936. [DOI] [PubMed] [Google Scholar]
- TASAKI I., HAGIWAR A. S. Demonstration of two stable potential states in the squid giant axon under tetraethylammonium chloride. J Gen Physiol. 1957 Jul 20;40(6):859–885. doi: 10.1085/jgp.40.6.859. [DOI] [PMC free article] [PubMed] [Google Scholar]
- Thomas R. C., Wilson V. J. Precise localization of Renshaw cells with a new marking technique. Nature. 1965 Apr 10;206(980):211–213. doi: 10.1038/206211b0. [DOI] [PubMed] [Google Scholar]
- Yamamoto C. Electrical activity observed in vitro in thin sections from guinea-pig cerebellum. Jpn J Physiol. 1974 Apr;24(2):177–188. doi: 10.2170/jjphysiol.24.177. [DOI] [PubMed] [Google Scholar]
- Yamamoto C., McIlwain H. Electrical activities in thin sections from the mammalian brain maintained in chemically-defined media in vitro. J Neurochem. 1966 Dec;13(12):1333–1343. doi: 10.1111/j.1471-4159.1966.tb04296.x. [DOI] [PubMed] [Google Scholar]